2076 results in Statistical Physics
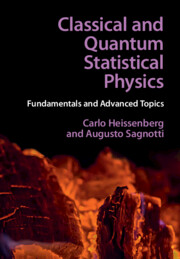
Classical and Quantum Statistical Physics
- Fundamentals and Advanced Topics
-
- Published online:
- 06 January 2022
- Print publication:
- 20 January 2022
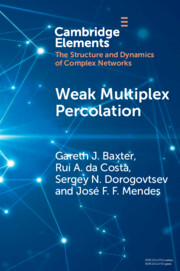
Weak Multiplex Percolation
-
- Published online:
- 21 December 2021
- Print publication:
- 27 January 2022
-
- Element
- Export citation
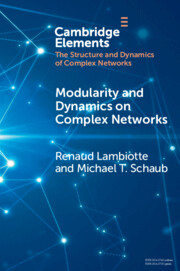
Modularity and Dynamics on Complex Networks
-
- Published online:
- 21 December 2021
- Print publication:
- 03 February 2022
-
- Element
- Export citation
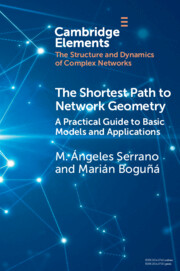
The Shortest Path to Network Geometry
- A Practical Guide to Basic Models and Applications
-
- Published online:
- 02 December 2021
- Print publication:
- 06 January 2022
-
- Element
- Export citation
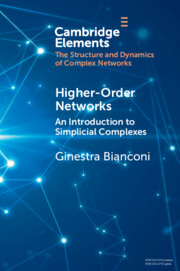
Higher-Order Networks
-
- Published online:
- 23 November 2021
- Print publication:
- 23 December 2021
-
- Element
- Export citation
5 - Perceptrons
- from Part II - Supervised Learning
-
- Book:
- Machine Learning with Neural Networks
- Published online:
- 14 October 2021
- Print publication:
- 28 October 2021, pp 75-95
-
- Chapter
- Export citation
Part I - Hopfield Networks
-
- Book:
- Machine Learning with Neural Networks
- Published online:
- 14 October 2021
- Print publication:
- 28 October 2021, pp 13-14
-
- Chapter
- Export citation
3 - Stochastic Hopfield Networks
- from Part I - Hopfield Networks
-
- Book:
- Machine Learning with Neural Networks
- Published online:
- 14 October 2021
- Print publication:
- 28 October 2021, pp 34-51
-
- Chapter
- Export citation
11 - Reinforcement Learning
- from Part III - Learning without Labels
-
- Book:
- Machine Learning with Neural Networks
- Published online:
- 14 October 2021
- Print publication:
- 28 October 2021, pp 206-226
-
- Chapter
- Export citation
Part II - Supervised Learning
-
- Book:
- Machine Learning with Neural Networks
- Published online:
- 14 October 2021
- Print publication:
- 28 October 2021, pp 73-74
-
- Chapter
- Export citation
7 - Deep Learning
- from Part II - Supervised Learning
-
- Book:
- Machine Learning with Neural Networks
- Published online:
- 14 October 2021
- Print publication:
- 28 October 2021, pp 114-140
-
- Chapter
- Export citation
Bibliography
-
- Book:
- Machine Learning with Neural Networks
- Published online:
- 14 October 2021
- Print publication:
- 28 October 2021, pp 227-237
-
- Chapter
- Export citation
Series page
-
- Book:
- Machine Learning with Neural Networks
- Published online:
- 14 October 2021
- Print publication:
- 28 October 2021, pp 250-250
-
- Chapter
- Export citation
8 - Convolutional Networks
- from Part II - Supervised Learning
-
- Book:
- Machine Learning with Neural Networks
- Published online:
- 14 October 2021
- Print publication:
- 28 October 2021, pp 141-156
-
- Chapter
- Export citation
Frontmatter
-
- Book:
- Machine Learning with Neural Networks
- Published online:
- 14 October 2021
- Print publication:
- 28 October 2021, pp i-iv
-
- Chapter
- Export citation
9 - Supervised Recurrent Networks
- from Part II - Supervised Learning
-
- Book:
- Machine Learning with Neural Networks
- Published online:
- 14 October 2021
- Print publication:
- 28 October 2021, pp 157-176
-
- Chapter
- Export citation
2 - Deterministic Hopfield Networks
- from Part I - Hopfield Networks
-
- Book:
- Machine Learning with Neural Networks
- Published online:
- 14 October 2021
- Print publication:
- 28 October 2021, pp 15-33
-
- Chapter
- Export citation
Contents
-
- Book:
- Machine Learning with Neural Networks
- Published online:
- 14 October 2021
- Print publication:
- 28 October 2021, pp v-viii
-
- Chapter
- Export citation
10 - Unsupervised Learning
- from Part III - Learning without Labels
-
- Book:
- Machine Learning with Neural Networks
- Published online:
- 14 October 2021
- Print publication:
- 28 October 2021, pp 179-205
-
- Chapter
- Export citation
Acknowledgements
-
- Book:
- Machine Learning with Neural Networks
- Published online:
- 14 October 2021
- Print publication:
- 28 October 2021, pp ix-x
-
- Chapter
- Export citation