Childhood asthma and other allergy-related diseases pose a substantial health burden in developed countries( Reference Asher, Montefort and Bjorksten 1 ). Fetal life is a critical time period of susceptibility during which a nutritional stressor can affect the development and maturation of respiratory and immune systems, and thus have irreversible influences on the development of allergy( Reference Devereux 2 ). In this context, it has been hypothesised that a lower intake of n-3 relative to n-6 PUFA might play a role in the aetiology of allergy-related diseases( Reference Black and Sharpe 3 ). PUFA of n-6 and n-3 families can be readily transferred across the placenta( Reference Haggarty 4 ), and have been shown in animal and in vitro studies to exert immunomodulatory effects( Reference Prescott and Dunstan 5 , Reference Calder, Kremmyda and Vlachava 6 ). Specifically, n-6 PUFA have been suggested to promote inflammation and increase allergic-disease activity, whereas n-3 PUFA seem to have an opposite effect( Reference Prescott and Dunstan 5 , Reference Calder, Kremmyda and Vlachava 6 ).
However, reports from human studies do not conclusively support that an increase in n-3 PUFA status, a decrease in n-6 PUFA status or both in the prenatal period is an effective strategy to prevent allergy-related symptoms in childhood. A 2016 systematic review and meta-analysis of prospective cohort studies assessing maternal fish or n-3 long-chain PUFA intake during pregnancy and of trials assessing fish-oil supplementation reported that the hypothesis linking maternal n-3 PUFA intake to childhood allergic disease cannot unequivocally be confirmed or rejected( Reference Best, Gold and Kennedy 7 ). Two subsequent trials on fish-oil supplementation also showed discrepant results, with reports of null( Reference Best, Sullivan and Palmer 8 ) or beneficial (i.e. lower incidence) effects( Reference Bisgaard, Stokholm and Chawes 9 ). Similarly, findings from studies assessing prenatal PUFA biomarker concentrations, which constitute accurate surrogates of fetal exposure( Reference Hornstra 10 ), have produced mixed results. The British Southampton Women’s Survey and Avon Longitudinal Study of Parents and Children (ALSPAC) studies reported lower rates of childhood wheeze symptoms with higher prenatal n-3 PUFA concentration( Reference Pike, Calder and Inskip 11 ) or a lower n-6:n-3 fatty acid ratio( Reference Newson, Shaheen and Henderson 12 ). In contrast, the Dutch Generation R study showed maternal concentration of n-6 PUFA, but not of n-3 PUFA levels, to be associated with decreased asthma risk in childhood( Reference Rucci, den Dekker and de Jongste 13 ), whereas other studies in the Netherlands, Germany and Singapore found no associations between any PUFA and child asthma or wheeze symptoms( Reference Notenboom, Mommers and Jansen 14 – Reference Yu, Chan and Calder 16 ). A lower risk of childhood eczema was shown with increasing cord blood n-3 PUFA concentration in the Spanish INMA cohort( Reference Montes, Chisaguano and Castellote 17 ). The Generation R found a higher eczema risk with higher maternal n-6 PUFA concentration( Reference Rucci, den Dekker and de Jongste 13 ), whereas the Dutch KOALA study reported a lower risk with increasing n-6:n-3 fatty acid ratio( Reference Notenboom, Mommers and Jansen 14 ). Other studies showed weak or null associations of prenatal PUFA concentrations with rates of childhood eczema( Reference Newson, Shaheen and Henderson 12 , Reference Standl, Demmelmair and Koletzko 15 , Reference Yu, Chan and Calder 16 ). Only a few studies have specifically examined childhood rhinitis and found no associations with prenatal PUFA concentrations( Reference Notenboom, Mommers and Jansen 14 – Reference Yu, Chan and Calder 16 ).
Contradictory findings of prior studies may at least partly be explained by heterogeneity in exposure and health outcome definitions, and differences in adjustment for confounding variables. Moreover, most of the trials were prone to methodologic limitations, such as selective reporting and absence of blinding of participants or personnel. Given the substantial health burden of allergic diseases, it is important to better characterise the potential role that PUFA may play in the development of asthma and other allergy-related diseases. Pooled analyses of diverse populations constitute an important step towards more generalisable conclusions. Hence, in the present study, we harmonised and pooled individual data of two cohorts from West and South Europe to assess whether n-3 and n-6 PUFA concentrations in cord blood phospholipids, reflecting fetal exposure in late pregnancy, are associated with symptoms of wheeze, asthma, rhinitis and eczema in children aged 6–7 years.
Methods
Study population
The Maastricht Essential Fatty Acid Birth (MEFAB, www.mefab.org) cohort( Reference van der Wurff, de Groot and Stratakis 18 ) enrolled 1334 pregnant women at the time of their first antenatal visit in three maternity clinics in the province of Limburg, the Netherlands, between 1989 and 1995. Inclusion criteria for participation in the study were as follows: gestational age <16 weeks, diastolic blood pressure <90 mmHg and no cardiovascular, neurological, renal or metabolic disorder. A total of 1203 women with singleton pregnancies were followed up until delivery, and 1008 them provided umbilical cord blood samples. Between 1997 and 2000, when children were 7 years of age (mean age 7·3 (sd 0·3) years), we conducted a follow-up evaluation; eligibility criteria included childbirth date before 1994, singleton pregnancy and availability of cord blood fatty acid measurements. Among 750 eligible children, 306 participated in the follow-up study, during which information on allergy-disease symptoms was obtained for 293 of them. A flow chart of the MEFAB participants is shown in online supplementary Fig. S1.
The RHEA Mother–Child Study in Crete (Greece, www.rhea.gr)( Reference Chatzi, Plana and Daraki 19 ) enrolled pregnant women during 2007–2008 at the time of the first major ultrasound examination at 10–13 weeks of gestation if they were resident at the prefecture of Heraklion, >16 years of age and without communication handicaps. During the study period, 1610 pregnant women agreed to participate and 1363 singleton pregnancies were followed up until delivery. A random subgroup of 500 umbilical cord blood samples was essayed for fatty acid levels in RHEA. For the child’s follow-up, the mothers were contacted at 9 months (n 1223), 4 years (n 873) and 6 years of age (n 608); sample sizes correspond to singleton children. In the present analysis, we included 228 children aged 9 months (mean age 9·1 (sd 1·9) months), 222 aged 4 years (4·2 (sd 0·2) years) and 213 aged 6 years (6·6 (sd 0·3) years) with available information on fatty acid exposure and allergy-related symptoms. A flow chart of the RHEA participants is shown in the online Supplementary Fig. S2.
In both cohorts, participants’ parents or legal guardians provided written informed consent. The study was conducted according to the guidelines laid down in the Declaration of Helsinki. All procedures involving human subjects were approved by the local authorised institutional review boards (Medical Ethics Committee of the University Hospital Maastricht and the University of Maastricht, the Netherlands, for the MEFAB cohort, and the review board of the University Hospital in Heraklion, Crete, Greece for the RHEA cohort).
PUFA analysis
In the MEFAB birth cohort, blood samples from the umbilical vein of infants were collected immediately after delivery in EDTA-containing tubes. Plasma was separated from blood cells by centrifugation and stored in aliquots at −80°C until analysis. Methods of fatty acid analysis and the phospholipid PUFA identified in MEFAB have been described in detail elsewhere( Reference Al, Vanhouwelingen and Kester 20 ).
In the RHEA birth cohort, umbilical cord blood samples were collected at delivery in 10-ml BD gel separator vacutainers. Serum was separated within 2 h after collection using centrifugation and stored in 0·5-ml aliquots at –80°C until assayed. The analysis of phospholipid fatty acids, including PUFA, was conducted in the Environmental Chemical Processes Laboratory (University of Crete, Greece) following a standard methodology, as described by Glaser et al.( Reference Glaser, Demmelmair and Koletzko 21 ). In brief, a fatty acid methyl ester (FAME) sample was obtained using direct in situ transesterification. The individual FAME were quantified by GC with flame ionisation detection. GC analysis was carried out on a Shimadzu-2010 chromatograph equipped with AOC-20i auto-injector (Shimadzu Europe). Separation of serum phospholipid FAME was performed on a SP-2560-fused silica capillary column (100 m×0·25 mm internal diameter, 0·2μm film thickness; Supelco) using He as the carrier gas at a constant flow of 1·1 ml/min. The injector port was maintained at 250°C and 1·6 μl sample volumes were injected at a split ratio of 1:20. The GC oven initial temperature was 130°C, and then increased at a rate of 4°C/min to 170°C and then at a rate of 2°C/min to 210°C and retained for 20 min. Detector temperature was kept at 260°C. Individual FAME peaks were identified by comparison of their retention times with SUPELCO 37 Component FAME mix (Supelco).
PUFA composition of plasma phospholipids is similar to that of serum phospholipids, and slight differences in the analytical methods used for fatty acid analysis in serum and plasma do not appear to affect the way that PUFA composition can be interpreted( Reference Hodson, Skeaff and Fielding 22 , Reference Stark, Van Elswyk and Higgins 23 ).
For the present analysis, our primary exposures of interest were the dietary essential n-3 PUFA precursor, α-linolenic acid (ALA, 18 : 3n-3), the sum of its major biologically active metabolic products EPA (20 : 5n-3) and DHA (DHA, 22 : 6n-3)( Reference Calder 24 ), and the sum of total n-3 fatty acids assayed in each cohort; the dietary essential n-6 PUFA precursor, linoleic acid (LA, 18 : 2n-6), its major metabolic product arachidonic acid (AA, 20 : 4n-6)( Reference Schmitz and Ecker 25 ) and the sum of total n-6 fatty acids assayed; and the total n-3:n-6 PUFA ratio.
Secondary exposures of interest were intermediate metabolic products in the biosynthetic pathway of PUFA that have been previously associated with pregnancy and child allergy-related outcomes including the n-3 docosapentaenoic acid (DPA, 22 : 5n-3), the n-6 γ-linolenic acid (GLA, 18 : 3n-6), dihomo-γ-linolenic acid (DGLA, 20 : 3n-6) and osbond acid (22 : 5n-6)( Reference Rucci, den Dekker and de Jongste 13 , Reference Hornstra 26 , Reference de Vries, Gielen and Rizopoulos 27 ). Individual fatty acid measurements were expressed as weight percentage of total fatty acids present in the chromatogram (wt%).
Childhood allergy-related outcomes
In both cohorts, information on wheeze, asthma, rhinitis and eczema occurrence was obtained by questionnaires adapted from the International Study on Asthma and Allergy in Childhood (ISAAC)( Reference Asher, Keil and Anderson 28 ). We defined current wheeze as the presence of any episode of wheezing or whistling in the chest in the past 12 months. Asthma ever was defined as ever-reported doctor diagnosis of asthma, whereas current asthma was defined as a positive answer to both ever-reported diagnosis of asthma and presence of wheezing or whistling in the chest in the past 12 months( Reference Pearce, Ait-Khaled and Beasley 29 ). We defined current rhinitis as the presence of sneezing or a runny or blocked nose in the past 12 months without common cold or flu( Reference Bjorksten, Clayton and Ellwood 30 ). We defined current eczema as the presence of an itchy rash in the past 12 months that affected any of the following places: the folds of the elbows, behind the knees, in front of the ankles, under the buttocks or around the neck, ears or eyes( Reference Pinart, Benet and Annesi-Maesano 31 ). For the MEFAB cohort, we used available information on allergy-related outcomes at the age of 7 years. For the RHEA cohort, we used information on wheeze symptoms at three time points (at 9 months, 4 years and 6 years), and information on asthma, rhinitis and eczema at 6 years. Although in RHEA there was available information on asthma, rhinitis and eczema at 4 years of age, we did not include these outcomes in the current analysis owing to very small numbers.
Covariates
We defined important confounders or effect modifiers as similarly as possible among the cohorts. Information on maternal weight and height at study entry, which were used to calculate maternal BMI (kg/m2), gestational weight gain from study entry until birth (kg), maternal smoking during pregnancy (yes/no), maternal age at delivery (in years), delivery type (vaginal delivery or caesarean section), parity (primiparous or multiparous), birth weight (g), gestational age (weeks) and child sex (male or female) was collected by means of self-administered questionnaires or hospital records. Information on breast-feeding (months), number of siblings (0, 1, 2, or ≥3), parental education (cohort-specific definitions of low, medium or high according to the highest completed education level of either parent) and history of parent atopy (presence of asthma, rhinitis or eczema to either one or both parents; yes/no) was obtained through self-administered questionnaires. At 6–7 years of age, child weight and height were measured according to standard procedures in both cohorts, and BMI (kg/m2) was calculated. In RHEA, we had available information on Mediterranean diet adherence in pregnancy calculated with an a priori defined score using a validated FFQ( Reference Chatzi, Torrent and Romieu 32 ). In MEFAB, we also collected fasting child blood samples at the age of outcome assessment and measured plasma levels of PUFA with standard procedures as described previously( Reference Rump, Popp-Snijders and Heine 33 ).
Statistical analysis
Characteristics of the study population were determined using descriptive analysis. Differences in the baseline characteristics between children included the present analysis and those excluded were studied using Pearson’s χ 2 test for categorical variables and Student’s t test for continuous ones.
We calculated standardised z scores for concentrations of all PUFA in each cohort to enable the comparison and pooling of cohort-specific effect estimates. We used multivariable generalised linear models for binary outcomes (with log link, Poisson distribution and robust variance estimator)( Reference Zou 34 ) to estimate relative risks (RR) and 95 % CI for the associations of each PUFA exposure with the outcomes of interest. We detected no departures from linearity in the exposure–outcome associations using a Wald test with the STATA command nlcheck( Reference Ben 35 ). We conducted cohort-specific analyses. We also performed pooled analyses by including a cohort indicator variable in the models and tested for heterogeneity in the associations between the two cohorts by examining the interaction between PUFA concentrations and cohort.
Following a Directed Acyclic Graph approach (online Supplementary Fig. S3), we selected the following variables for model adjustment: maternal age, maternal BMI at study entry, gestational weight gain, maternal smoking during pregnancy, parental education, parity, parent atopy, child sex and age at outcome assessment. We did not include birth weight or gestational age in our main models, as they might be in the intermediate pathway between prenatal PUFA levels and child allergy-related outcomes.
To further assess our research hypothesis, we conducted additional analyses using different levels of available information within each cohort. First, we assessed the extent to which child plasma PUFA levels measured at the age of outcome assessment could explain the observed associations by further adjusting for them in the models for MEFAB and evaluating whether they changed the effect estimates. Second, we conducted further adjustment for Mediterranean diet adherence in pregnancy in the RHEA models. Third, we examined associations between the primary PUFA of interest and current wheeze symptoms at 9 months and 4 years of age in the RHEA birth cohort.
To increase sample size (precision) and reduce bias due to missing data, we imputed missing covariate data. Using multiple imputations with chained equations (MICE), we generated fifty imputed data sets for each cohort( Reference Rubin 36 , Reference White, Royston and Wood 37 ). The imputation model included exposures, outcomes, confounders, as well as additional auxiliary variables such as maternal origin, mode of delivery, pregnancy outcomes, breast-feeding duration, sibship size and child BMI. In analytic models, we combined estimates from the imputed data sets with the use of Rubin’s rules( Reference Rubin 36 ).
Sensitivity analyses were performed to assess the robustness of our results. We repeated the analyses by including only those participants with no missing covariate data (complete case analysis). We made further adjustment for BMI at the age of outcome assessment to assess the extent to which child adiposity could influence the observed associations. We also repeated the analyses after including birth weight and gestational age in the multivariable models. Finally, we examined effect measure modification by child sex (boy v. girl)( Reference Almqvist, Worm and Leynaert 38 ) and parent atopy (yes v. no)( Reference Nurmatov, Nwaru and Devereux 39 , Reference Duchen and Bjorksten 40 ) in the associations of the total n-3:n-6 PUFA ratio with child outcomes by assessing both multiplicative and additive interaction measures between the potential effect modifier (one at a time) and the exposure( Reference Knol, van der Tweel and Grobbee 41 , Reference VanderWeele and Knol 42 ). Statistical significance for effect modification was defined as P<0·10.
Our study including 506 participants had 80 % power at a 5 % significance level to detect an effect size of as small as 48 % for risk estimates of current asthma (the outcome with the lowest prevalence in the pooled data set; 4·6 %) per sd increase in fatty acid concentrations, which is similar to those reported in previous studies( Reference Rucci, den Dekker and de Jongste 13 – Reference Standl, Demmelmair and Koletzko 15 ). Power analysis was conducted using GPower version 3.1( Reference Faul, Erdfelder and Buchner 43 ). All other analyses were conducted with STATA version 13.0 (StataCorp).
Results
Participant characteristics
In the pooled data set, mothers had a mean age at delivery of 29·8 (sd 4·5) years. Of all parents included in this analysis, 37% had a high education level. The total ratio of boys to girls was 1·29. Participants’ characteristics of the separate cohorts are presented in Table 1. In non-response analysis, we saw that, compared with children without follow-up measurements, those with follow-up data had mothers who were more likely to be older at delivery and multiparous in MEFAB, whereas in RHEA children with follow-up data were more likely to be male (online Supplementary Table S1).
Table 1 Parental and child characteristics in Maastricht Essential Fatty Acid Birth (MEFAB) and RHEA Mother–Child birth cohorts (Mean values and standard deviations; numbers and percentages)
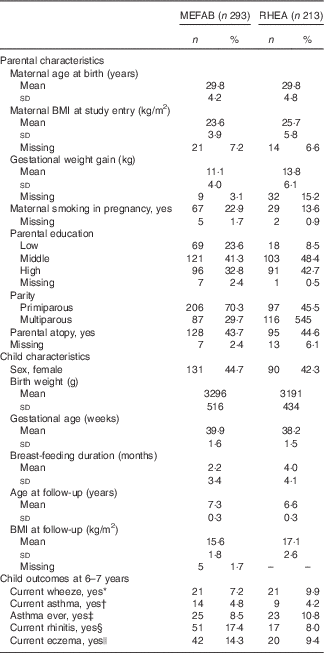
* Current wheeze was defined as the presence of wheezing or whistling in the chest in the past 12 months.
† Current asthma was defined as a positive answer to both ever-reported doctor diagnosis of asthma and presence of wheezing or whistling in the chest in the past 12 months.
‡ Asthma ever was defined as ever-reported doctor diagnosis of asthma.
§ Current rhinitis was defined as the presence of sneezing or a runny or blocked nose in the past 12 months without common cold or flu.
|| Current eczema was defined as the presence of an itchy rash in the past 12 months that affected any of the following places: the folds of the elbows, behind the knees, in front of the ankles, under the buttocks or around the neck, ears or eyes.
Table 2 shows the distribution of the cord blood phospholipid PUFA measured in each cohort. Mean concentrations of n-3 and n-6 PUFA in MEFAB were 6·89 (sd 1·59) and 32·16 (sd 1·69) wt%, respectively; the corresponding values for RHEA were 5·38 (sd 1·12) and 32·06 (sd 2·35) wt%.
Table 2 Distribution of cord blood phospholipid PUFA levels in Maastricht Essential Fatty Acid Birth (MEFAB) and RHEA Mother–Child birth cohorts (Mean values and standard deviations)
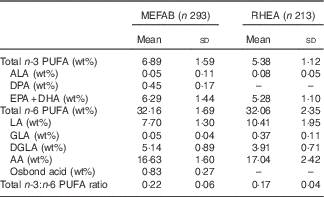
AA, arachidonic acid (20 : 4n-6); ALA, α-linolenic acid (18 : 3n-3); DGLA, dihomo-γ-linolenic acid (20 : 3n-6); DHA, 22 : 6n-3; DPA, docosapentaenoic acid (22 : 5n-3); EPA, 20 : 5n-3; GLA, γ-linolenic acid (18 : 3n-6); LA, linoleic acid (18 : 2n-6); osbond acid, 22 : 5n-6; total n-3 (n-6) PUFA, the sum of n-3 (n-6) PUFA present in the chromatogram; wt%, weight percentage of total fatty acids measured.
Primary/pooled analyses
Interaction P values between PUFA concentrations and cohort were indicative of no significant heterogeneity between cohorts for most exposure–outcome associations, although the magnitude and precision of some effect estimates differed slightly (Table 3 and online Supplementary Tables S2 and S3).
Table 3 Associations of cord blood phospholipid PUFA levels with current wheeze and asthma at 6–7 years of age in Maastricht Essential Fatty Acid Birth (MEFAB) and RHEA Mother–Child cohorts, separately and in pooled analysisFootnote ‡ (Relative risks (RR) and 95 % confidence intervals)
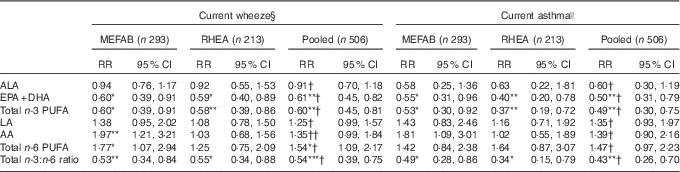
AA, arachidonic acid (20 : 4n-6); ALA, α-linolenic acid (18 : 3n-3); DHA, 22 : 6n-3; EPA, 20 : 5n-3; LA, linoleic acid (18 : 2n-6); total n-3 (n-6) PUFA, the sum of n-3 (n-6) PUFA present in the chromatogram.
P value for PUFA exposure–outcome association: * P<0·05, ** P<0·01, *** P<0·001.
P value for PUFA exposure–cohort interaction: † P for interaction >0·10, †† P for interaction=0·06.
‡ RR and 95 % CI were calculated using generalised linear models for binary outcomes (modified Poisson) adjusted for maternal age, maternal BMI at study entry, gestational weight gain, maternal smoking during pregnancy, parity, parental education, parent atopy, child age at outcome assessment, child sex and for the pooled estimate a cohort indicator. Effect estimates correspond to a standard deviation score increase in PUFA and to a unit increase in the total n-3:n-6 ratio.
§ Current wheeze was defined as the presence of wheezing or whistling in the chest in the past 12 months.
|| Current asthma was defined as a positive answer to both ever-reported doctor diagnosis of asthma and the presence of wheezing or whistling in the chest in the past 12 months.
In pooled analyses, we found that higher total n-3 PUFA concentration was associated with a lower risk of current wheeze, whereas an inverse association was observed for total n-6 PUFA (pooled RR 0·60; 95 % CI 0·45, 0·81 and 1·54; 95 % CI 1·09, 2·17 per sd increase in n-3 and n-6 PUFA, respectively). Accordingly, a higher ratio of total n-3:n-6 fatty acids at birth was associated with a lower risk of current wheeze (pooled RR 0·54; 95 % CI 0·39, 0·75 per unit increase) (Table 3). When we examined current asthma, we found a lower risk with higher n-3 PUFA concentration (pooled RR 0·49; 95 % CI 0·30, 0·75) and a higher n-3:n-6 fatty acid ratio (pooled RR 0·43; 95 % CI 0·26, 0·70) (Table 3). Associations for ever-reported doctor diagnosis of asthma were in the same direction and magnitude (online Supplementary Table S2). No associations with current rhinitis and eczema symptoms at 6–7 years were found for total n-6 PUFA and n-3 PUFA concentrations, and the n-3:n-6 ratio (online Supplementary Table S3).
In individual fatty acid analyses, we found EPA+DHA concentration in cord blood to be associated with decreased risk of both current wheeze and current asthma (pooled RR 0·61; 95 % CI 0·45, 0·82 and 0·50; 95 % CI 0·31, 0·79 per sd increase in EPA+DHA, respectively) (Table 3). Similarly, a protective association was observed between EPA+DHA and risk of ever asthma (online Supplementary Table S2). No associations with any of the outcomes examined were found for the n-3 ALA, and the n-6 LA and AA in the pooled data set (Table 3 and online Supplementary Tables S2 and S3). Results from the crude models adjusted only for age and sex did not materially differ from those of the main adjusted models (online Supplementary Table S4).
Secondary analyses
In secondary analyses, we found no associations of the n-3 DPA and n-6 GLA, DGLA and osbond acid with allergy-related outcomes (online Supplementary Tables S5 and S6). In addition, inclusion of child plasma PUFA concentrations at the age of outcome assessment in MEFAB models did not modify the direction and size of the effect estimates (data not shown). In RHEA, results remained similar when we further adjusted for maternal Mediterranean diet adherence in pregnancy (data not shown). We found no associations with current wheeze symptoms in the other time points in RHEA, although a trend towards a reduction in wheeze risk with higher concentration of n-3 PUFA, and especially EPA+DHA, and a higher n-3:n-6 ratio was observed with increasing child age from 9 months to 6 years (Fig. 1 and online Supplementary Table S7).

Fig. 1 Associations of cord blood phospholipid PUFA concentrations with current wheeze symptoms at 9 months, 4 years and 6 years of age in the RHEA Mother–Child cohort. Values are relative risks and their 95 % CI calculated using generalised linear models for binary outcomes (modified Poisson). Models were adjusted for maternal age, maternal BMI at study entry, gestational weight gain, maternal smoking during pregnancy, parity, parental education, parent atopy, child age at outcome assessment and sex. Effect estimates correspond to a standard deviation score (SDS) increase in total n-3 (n-6) PUFA and to a unit increase in the total n-3:n-6 ratio. Current wheeze was defined as presence of wheezing or whistling in the chest since birth at 9 months of age (n/N 62/228) and in the past 12 months at 4 years (n/N 10/222) and 6 years of age (n/N 21/213). Total n-3 (n-6) PUFA, the sum of n-3 (n-6) PUFA present in the chromatogram. ,
and
, Effect estimate of each time point. Vertical bars represent 95% confidence intervals.
Sensitivity analyses
The effect estimates did not materially change in complete case analysis or when additional adjustment was made for child BMI (data not shown). Effects estimates also remained similar after including birth weight and gestational age in multivariable models (online Supplementary Table S8). We found no evidence of effect measure modification by parent atopy for any of the outcomes of interest (online Supplementary Table S9). There was some evidence of effect measure modification by female sex on the multiplicative scale for the association between the n-3:n-6 PUFA ratio and current rhinitis symptoms (pooled RR 0·57; 95 % CI 0·32, 1·01; P=0·06) (online Supplementary Table S10). However, no effect modification by child sex on either multiplicative or additive scale was observed for any of the other outcomes of interest (online Supplementary Table S10).
Discussion
In this analysis of prospective data of two birth cohorts from West and South Europe with different sociodemographic characteristics, we found that higher concentrations of n-3 PUFA, and especially of EPA and DHA, in cord blood were associated with reduced risk of current wheeze symptoms and asthma at 6–7 years of age. Higher cord blood n-6 PUFA concentrations were associated with a higher risk of current wheeze. We also observed a reduced risk of both current wheeze and asthma with increasing n-3:n-6 fatty acid ratio. The results were fairly homogeneous across the cohorts after controlling for a variety of confounders, and remained robust in the pooled and sensitivity analyses.
We measured cord blood phospholipid PUFA concentrations, which reflect recent maternal dietary intake at a time frame of 2–4 weeks( Reference Hodson, Skeaff and Fielding 22 ), as well as the level of fetal demand, and selectivity and efficiency of placental transfer( Reference Haggarty 4 ). Hence, they constitute good surrogates of fetal exposure in late pregnancy. The timing and biomarker used for assessing fetal exposure in our study are of high relevance as they coincide with the period during which there is an exponential increase in the rate of fetal fat accretion( Reference Haggarty 4 ).
Fetal life is a critical time period of susceptibility during which nutritional exposures could have irreversible long-term influences on airway and immune function( Reference Devereux 44 ). The n-6 and n-3 fatty acids have been proposed to exert immune-regulatory effects through involvement in several pathways. Animal and in vitro studies have shown that n-6 PUFA, as substrate for eicosanoid synthesis, can promote inflammation, favour T-helper cell type 2-driven immune responses and enhance the synthesis of Ig E antibodies( Reference Calder, Kremmyda and Vlachava 6 ). On the contrary, n-3 PUFA have been suggested to exert a protective effect by competing with the n-6 fatty acids for incorporation into cell membranes and for metabolism by enzymes of eicosanoid synthesis( Reference Calder, Kremmyda and Vlachava 6 ). In addition, n-3 long-chain PUFA have been shown in vitro to alter the expression of inflammatory genes by modifying transcription factor activation, inhibit T-cell signalling through alteration of membrane lipid rafts, as well as to give rise to a family of anti-inflammatory mediators termed resolvins( Reference Calder, Krauss-Etschmann and de Jong 45 ).
Reports from human trials cannot unequivocally confirm or reject a beneficial effect of n-3 EPA and DHA supplementation in fetal life on allergy in childhood( Reference Best, Gold and Kennedy 7 , Reference Best, Sullivan and Palmer 8 , Reference Ramaswami, Serhan and Levy 46 ). Findings from longitudinal cohort studies on prenatal PUFA exposure and childhood asthma or other allergy symptoms have also been mixed, with reports of null( Reference Rucci, den Dekker and de Jongste 13 , Reference Standl, Demmelmair and Koletzko 15 , Reference Yu, Chan and Calder 16 ), beneficial (i.e. lower incidence)( Reference Pike, Calder and Inskip 11 , Reference Newson, Shaheen and Henderson 12 , Reference Montes, Chisaguano and Castellote 17 , Reference Lumia, Luukkainen and Tapanainen 47 , Reference Miyake, Sasaki and Tanaka 48 ) or even unfavourable( Reference Notenboom, Mommers and Jansen 14 ) associations with higher n-3 PUFA or decreasing n-6:n-3 fatty acid ratio. Inconsistencies in findings may partly arise from exposure profile heterogeneity (i.e. categorisation and timing of exposure), and differences in outcome definition and adjustment for confounding variables. In the present study, we used two independent cohorts for replication, and we followed a centralised statistical analysis following a consensus protocol with harmonised information on exposure variables, potential confounders and child allergy-related symptoms. We found broadly similar associations across the different cohorts, although there was some evidence for between-cohort heterogeneity for the risk estimates of current wheeze in relation to n-6 AA, for ever asthma in relation to n-3 ALA and for risk estimates of the n-6 GLA and DGLA. Such heterogeneity might have occurred owing to variability in the analytical methods used for cord blood fatty acid analysis and/or owing to differences in confounder patterns (e.g. differences in diet or lifestyle) between participating cohorts.
The EPA+DHA status in the Netherlands and Greece is characterised as very low-to-low, which is similar to most European and US populations following a Westernised diet( Reference Stark, Van Elswyk and Higgins 23 ). We found that 1 sd increase in cord blood concentrations of n-3 PUFA, and especially of EPA and DHA, was associated with lower risk for childhood symptoms of wheeze and asthma. An inverse association with childhood wheeze and asthma risk was also observed for the n-3:n-6 PUFA ratio, which was driven to a great extent by n-3 long-chain PUFA. We did not have information on dietary intake in late pregnancy so as to disentangle the amount of dietary PUFA needed in order to achieve an sd change in cord blood long-chain PUFA concentrations. This change is feasible and likely reflects a significant dietary modification( Reference Miles, Noakes and Kremmyda 49 ). Thus, a sizeable effect on wheeze and asthma symptoms could be achieved from dietary interventions leading to a marked increase in the n-3:n-6 PUFA ratio, and mainly in the intake of n-3 long-chain PUFA in late gestation. Indeed, in trials, a relation between fish-oil supplementation and effect becomes apparent at high doses, which are expected to significantly increase blood PUFA concentrations. In two trials conducted in Denmark, where population background levels of n-3 long-chain PUFA are higher compared with most European populations( Reference Stark, Van Elswyk and Higgins 23 ), supplementation with high doses of fish oil in late gestation (providing 2·4 g EPA+DHA/d from week 24 of pregnancy onwards( Reference Bisgaard, Stokholm and Chawes 9 ) or 2·7 g/d from week 30 of pregnancy onwards( Reference Olsen, Osterdal and Salvig 50 )) resulted in significant increases (>1 sd) in blood n-3 long-chain PUFA concentrations and lower risk of offspring wheeze and asthma compared with placebo. In terms of safety, high doses of n-3 long-chain PUFA up to 2·7 g/d in pregnancy through fish-oil supplementation have not been associated with serious adverse effects, such as bleeding complications( Reference Makrides, Duley and Olsen 51 ).
Our findings are partly in line with the initial Black and Sharpe hypothesis, suggesting that the balance between n-3 and n-6 PUFA might have implications in the development of asthma and other allergy-related symptoms( Reference Black and Sharpe 3 ). Despite the unifying hypotheses that have been developed to explain the common co-existence of allergic disease symptoms in a child( Reference Spergel and Paller 52 ), asthma, rhinitis and eczema represent different disease entities with different underlying mechanisms( Reference Martinez and Vercelli 53 – Reference Skoner 55 ). In the present study, we observed associations only for symptoms of wheeze and asthma, suggesting a specific effect of prenatal PUFA on airway development and function. Further observational and experimental studies using spirometry and markers of airway inflammation are needed for replication and to explore the long-term effects of prenatal PUFA exposure.
A recent systematic review highlighted the importance of planning a subgroup analysis by sex in studies assessing the health effects of perinatal PUFA( Reference Decsi and Kennedy 56 ). Sexual dimorphism in fatty acid metabolism has been suggested to exist owing to sex differences in rates of β-oxidation and fat mobilisation, and possible influences of sex hormones on the enzymatic synthesis of long-chain fatty acids( Reference Decsi and Kennedy 56 , Reference Childs, Romeu-Nadal and Burdge 57 ). A previous analysis in the Generation R reported no effect measure modification in the association between maternal PUFA concentrations with childhood atopic diseases by offspring sex( Reference Rucci, den Dekker and de Jongste 13 ). In our study, we found some evidence for effect measure modification by female sex on the multiplicative scale for the association between the ratio of total n-3:n-6 PUFA at birth with rhinitis symptoms in childhood. However, we did not observe any effect modification by sex for the other outcomes of interest. Thus, we treat this result with caution, as we cannot rule out the possibility that the observed effect modification for rhinitis is due to chance.
As in all observational studies, there is the possibility of unmeasured residual confounding. Although, in our analyses, we took into account a variety of maternal and child lifestyle characteristics, including Mediterranean diet adherence during pregnancy( Reference Chatzi, Torrent and Romieu 32 ) and child biomarker levels of PUFA, we cannot exclude the possibility that other differences in diet or lifestyle factors might have influenced the observed findings. However, we did not see strong evidence for sociodemographic confounding in our analyses. Similar to most cohort studies, a limitation of the present study is attrition. It is possible that loss to follow-up could have introduced bias. Nevertheless, many sociodemographic characteristics and the cord blood PUFA concentrations were similar in the study sample and in those excluded. Child allergic-disease symptoms were ascertained by symptom-based questionnaires, which could lead to outcome misclassification. However, both participating cohorts used questionnaires adapted from the ISAAC study( Reference Asher, Keil and Anderson 28 ). Moreover, to enhance asthma and eczema outcome accuracy, we used composite definitions that require many conditions to be met. Although assessment of several PUFA exposures and child outcomes might raise concern about multiple testing, in our opinion an application of correction for multiple comparisons might be inappropriate in this case given the high correlations among exposures and among outcomes( Reference Rothman 58 ).
In this pooled analysis of two cohorts from West and South Europe, we found that high concentrations of n-3 PUFA, particularly EPA and DHA, and a high n-3:n-6 ratio in cord blood were associated with reduced risk of current wheeze and asthma in mid-childhood. Our findings suggest that dietary interventions resulting in a marked increase in the dietary n-3:n-6 PUFA ratio, and mainly in the intake of n-3 long-chain PUFA in late gestation are likely to reduce the risk of asthma symptoms in mid-childhood.
Acknowledgements
The authors would like to thank Professor Emeritus Gerard Hornstra, who made the MEFAB study possible. The authors are also extremely grateful to the participants of the MEFAB and RHEA cohorts for their time and commitment.
MEFAB: The MEFAB cohort was financially supported by the Dutch Organization for Scientific Research (NWO, grant no. 904 62 186), and the University Hospital of Maastricht (Profilerings Fonds).
RHEA: The Mother–Child Cohort in Crete project was financially supported by European projects (EU FP6-003-Food-3-NewGeneris – contract no. 16320, EU FP6 STREP Hiwate– contract no. 36224, EU FP7 ENV.2007.1.2.2.2. Project No. 211250 Escape, EU FP7-2008-ENV-1.2.1.4 Envirogenomarkers contract no. 226756, EU FP7-HEALTH-2009-single stage CHICOS contract no. 241604, EU FP7 ENV.2008.1.2.1.6. proposal no. 226285 ENRIECO, EU-FP7, proposal no. 264357 MeDALL, EU- FP7- HEALTH-2012 proposal no. 308333 HELIX), and the Greek Ministry of Health (Program of Prevention of obesity and neurodevelopmental disorders in preschool children, in Heraklion district, Crete, Greece: 2011–2014; ‘Rhea Plus’: Prevention Program of Environmental Risk Factors for Reproductive Health, and Child Health: 2012–2015.
N. S., M. G., L. C. and M. P. Z. conceived the study and had primary responsibility for the final content; M. A. and E. G. S. conducted the assay of fatty acids for the RHEA cohort; all authors were involved in the design and planning of the study, and the data collection; N. S. and K. M. analysed data; N. S., M. G. and L. C. wrote the paper; and all authors critically reviewed and approved the final manuscript.
None of the authors has any conflicts of interest to declare.
Supplementary material
For supplementary material/s referred to in this article, please visit https://doi.org/10.1017/S0007114517003348
Target article
PUFA status at birth and allergy-related phenotypes in childhood: a pooled analysis of the Maastricht Essential Fatty Acid Birth (MEFAB) and RHEA birth cohorts
Related commentaries (1)
Invited commentary in response to PUFA status at birth and allergy-related phenotypes in childhood: a pooled analysis of the Maastricht Essential Fatty Acid Birth and Rhea birth cohorts