All environments contain hidden hazards to humans. When a population moves to an allochthonous place either by choice or by force, it faces the challenge of adapting to a new ecological niche. Thus, understanding the environment's role in the biocultural evolution and health consequences of ancient population mobility is of paramount importance.
During the expansion of Tawantisuyo, the Incas occupied a variety of macro-ecological zones, from jungles to the vast and inhospitable Atacama Desert, the focus of this study. To help impose, maintain, expand, and consolidate the empire's ideology; transmit their way of life, cultivation methods, and strategies; and gain tribute for the state, among other activities, the Inca rulers not only mobilized their armies but also moved entire communities, called mitimaes or mitmaqkuna, to very distant places (Horta Reference Horta2015; Noack Reference Noack2018). Some groups were displaced from their native regions as punishment for opposing the Inca. In the Quechua language, mitmaqkuna means “upstart man” or “settled in a place different from his place of origin” (González-Holguín Reference González-Holguín1952 [1608]:140). The mitimaes fulfilled strategic military, sociocultural, and religious functions, depending on the empire's needs. For instance, they were tasked with spreading the Quechua language and customs or taking care of the huacas (shrines) and important sanctuaries. Productive mitimaes were charged with incentivizing, improving, or producing goods for the state (Horta Reference Horta2015; Murra Reference Murra1972; Noack Reference Noack2018). Although mitimaes may have been dependent on a curaca (chief), ultimately they functioned exclusively for the benefit of the Inca state (Noack Reference Noack2018).
Thus, various populations of mitimaes were relocated to places far away from Cusco or their ancestral locations. Forced to experience this diaspora, the populations had to carry out the activities entrusted to them by the Inca while adapting to the challenges in their new ecological and geographic environment. In this article, we ask whether bioarchaeological evidence of mitimaes can be found in extreme northern Chile. If so, what was their primary role, and how did the new environment affect their health and productivity?
One of the challenges faced by new arrivals to extreme northern Chile would have been water poisoning caused by the high amount of arsenic present in local rivers (Álvarez Reference Álvarez2014; Arriaza, Amarasiriwardena, et al. Reference Arriaza, Huaman, Villanueva, Tornero and Aravena2018; Arenas Reference Arenas H.2016; Bundschuh et al. Reference Bundschuh, Nicolli, Blanco, Blarasin, Farías, Cumbal, Cornejo, Bundschuh, Carrera and Litter2008; Figueroa Reference Figueroa2001), which led to a condition known as chronic regional endemic hydroarsenicism (CREHA; Litter and Bundschuh Reference Litter and Bundschuh2010). The Atacama Desert is rich in minerals that, when mixed with water, are incorporated into the food chain and eventually consumed by humans, causing severe health problems (Hopenhayn et al. Reference Hopenhayn, Ferreccio, Browning, Huang, Peralta, Gibb and Hertz-Picciotto2003; Hopenhayn-Rich et al. Reference Hopenhayn-Rich, Browning, Hertz-Picciotto, Ferreccio, Peralta and Gibb2000; Tondel et al. Reference Tondel, Rahman, Magnuson, Chowdhury, Faruquee and Ahmad1999). Therefore, the ancient populations that settled in these places, particularly those who relocated to them, very likely suffered from water poisoning, the effects of which ranged from stomachaches to various types of cancer.
Since ancient times, poisoning by natural polycontaminants (e.g., arsenic, boron, and lithium) has occurred through the ingestion of water or of contaminated products (e.g., plants, animals) that consume the same water sources, thereby incorporating these invisible polycontaminants into their food chain (Arriaza et al. Reference Arriaza, Dulasiri Amarasiriwardena, Vivien Standen, Bartkus and Bandak2010; Byrne et al. Reference Byrne, Amarasiriwardena, Bandak, Bartkus, Kane, Jones, Yáñez, Arriaza and Cornejo2010; Figueroa et al. Reference Figueroa, Razmilic, Allison and González1988; Swift et al. Reference Swift, Cupper, Greig, Westaway, Carter, Santoro, Wood, Jacobsen and Bertuch2015). Of these elements, arsenic is the most toxic, and its levels in water sources vary regionally. Water in the Camarones River reaches an annual arsenic (As) average of 1,000 μg/L. Thus, considering the endemic nature of this contamination, arseniasis would have affected the daily lives of those who had not been previously exposed or who were more susceptible to this toxic element, exacting a toll on their subsistence and health. If mitimaes were relocated to Camarones, they would have been exposed to the health risk of arsenic poisoning.
It should be noted that mitimaes populations retained their ethnic origins, kinship ties, ancestral customs, clothing, and headdresses while holding economic obligations to their curacas (Horta Reference Horta2015; Noack Reference Noack2018). Maintaining their identity served to make them identifiable by the authorities and differentiated them from the locals, making them discoverable in the bioarchaeological record. This is especially true in places such as the Atacama Desert, where the excellent preservation of bioarchaeological remains has facilitated the study of grave goods and deceased individuals.
The coexistence of multiethnic groups in territories in northern Chile during the Late Intermediate period (ca. AD 1000–1450) has been postulated based on ethnographic and archaeological records (Hidalgo Reference Hidalgo and Hidalgo2004; Hidalgo and Focacci Reference Hidalgo and Focacci1986). Rostworowski de Diez Canseco (Reference Rostworowski de Diez Canseco1986:129; translation by the authors) notes, “During the Late Horizon, in each of the valleys within the Colesuyu area, there were innumerable curacazgos of diverse extensions. . . . Based on archival information, the main fishermen settlements in Moquegua, Tacna, Arica, and south Atacama were established on the coast and at the mouth of rivers, forming particular villages led by their own chiefs.”
The influence of the Inca state during the Late Horizon (ca. AD 1450–1550) and the evidence of new populations in the extreme north of Chile are revealed there by the network of roads (capac ñan), resting places (tambos), storage deposits (colcas), and administrative and housing centers; for example, at sites AZ-15 and Saguara (Santoro and Muñoz Reference Santoro and Muñoz1981; Schiappacasse and Niemeyer Reference Schiappacasse and Niemeyer1989, Reference Schiappacasse and Niemeyer2002) and at cemeteries such as Chaca 5 and PLM-6 (Horta Reference Horta2000, Reference Horta2011a, Reference Horta2015). The AZ-15 village and Chaca 5 cemetery provide evidence for foreign populations that moved to the Azapa and Vitor Valleys, respectively, during the Inca period.
Other sites with similar ceramic and cultural characteristics include PLM-6 (on the Arica coast), where radiocarbon dates of hair combs from this site range from the Late period (cal AD 1520–1570) to Spanish contact (Arriaza et al. Reference Arriaza, Standen, Heukelbach, Cassman and Olivares2014). CAM-9 (a coastal site at Camarones Cove), although with fewer goods, is also associated with the Inca period (Horta Reference Horta2011a, Reference Horta2011b, Reference Horta2015; Santoro et al. Reference Santoro, Romero, Standen, Valenzuela and Topic2009). Based on this evidence, the populations of these sites correspond to Altiplanic colonies that settled in different ecological zones, including the lowland and coastal valleys. In contrast, Cassman (Reference Cassman2000), who studied Arica textiles from the AZ-140 and AZ-71 valley sites, as well as the PLM-9 coastal sites, which range from AD 900 to 1400, argues that mortuary textiles did not support a multiethnic hypothesis for these sites and that many textiles were frequently repaired.
Murra (Reference Murra1972) suggests that different ethnic groups exploited different ecological areas to locate complementary resources; however, the available water quality must have constrained their quality of life and the volume of resources produced. Proving the existence of multiethnic groups or even of a foreign group is a challenge, particularly if they vary regionally across time and space. In this sense, if nonlocal groups were present in the Arica area, then it is worth investigating how they were affected by the local environment.
Yet, different lines of evidence suggest the presence of nonlocal populations in various Andean regions, including northern Chile during the Inca Horizon. Such evidence raises several questions: What kind of foreign populations (e.g., military or productive) would be present in the archaeological record? What is the bioanthropological evidence? And how were these displaced populations affected when they faced new ecological niches? Undoubtedly, the first generation of relocated populations suffered greatly after leaving behind their natural habitat, food, families, and customs. This was no minor problem, considering that individuals and populations faced different potential risks in each environment, some of which were visible and others invisible to the human eye, such as water poisoning. Exposure to environmental stressors present in new niches is an important factor that affects daily life, as well as economic and social activities (Bigham and Lee Reference Bigham and Lee2014). Of these challenges in northern Chile, water quality is the most serious stressor and needs to be examined when discussing ancient health and socioeconomic dimensions of Andean populations that settled in allochthonous places.
Before relocating, ancestral populations had likely already experienced an adaptation process in their original environments. In some of the environments where the Inca relocated new populations, they unknowingly were exposed to arsenic-laden water. In this study, we both summarize previous publications regarding arseniasis in the CAM-9 population and provide new analytical arsenic data for this site, proposing that CAM-9 represents a nonlocal population. We integrate archaeological and bioarchaeological data to (1) deepen understanding of the environment's role in the biocultural evolution of translocated populations to new ecological niches, (2) investigate how the new environment could have affected the productivity level of the relocated populations, and (3) shed light on adaptation processes experienced by different human groups in the past that faced hidden natural contaminants such as arsenic.
Study Background
Natural Environmental Pollution
The waters in northern Chile contain very high levels of natural contaminants (Bundschuh et al. Reference Bundschuh, Nicolli, Blanco, Blarasin, Farías, Cumbal, Cornejo, Bundschuh, Carrera and Litter2008; Echeverría et al. Reference Echeverría, Niemeyer, Muñoz and Uribe2018; Figueroa Reference Figueroa2001; Yáñez et al. Reference Yáñez, Mansilla, Paola Santander, Fierro, Cornejo, Barnes and Amarasiriwardena2015). Arsenic (As), boron (B), and lithium (Li) are found at concentrations that exceed 10–100 times the standard concentrations recommended by the World Health Organization (WHO) for human consumption. Of these three eco-pollutants, As is the most harmful to humans. It is found in both surface water and groundwater, and its concentration varies depending on the geographic area. For example, the waters in the San José River (Azapa) are of better quality than those in the Lluta and Camarones Rivers (Figueroa Reference Figueroa2001). In some places, such as Quebrada de Camarones, As concentrations average 1,000 μg/L—one hundred times more than the standard limit proposed by the WHO, which recommends that As in drinking water should not exceed 10 μg/L (Bundschuh et al. Reference Bundschuh, Nicolli, Blanco, Blarasin, Farías, Cumbal, Cornejo, Bundschuh, Carrera and Litter2008; Figueroa Reference Figueroa2001; WHO Reference WHO2003, Reference WHO2008). Chronic regional endemic hydroarsenicism affects many populations (Litter and Bundschuh Reference Litter and Bundschuh2010) and presents a mosaic-type spatial distribution; that is, nearby communities can have different levels of natural contamination (Arriaza, Amarasiriwardena, et al. Reference Arriaza, Amarasiriwardena, Standen, Yáñez, Van Hoesen and Figueroa2018; Swift et al. Reference Swift, Cupper, Greig, Westaway, Carter, Santoro, Wood, Jacobsen and Bertuch2015). Therefore, water quality is of utmost importance when discussing the possible paleopathological, epidemiological, dietary, and social dynamics that confronted the ancient populations in this arid area of the continent. Álvarez (Reference Álvarez2014) postulates that the Incas preferred rivers with brackish water, because the abundance of water year-round allowed for better management of their maize crops in the Locumba, Sama, Lluta, Camarones, and Loa Valleys. The arsenic water in Lluta and Camarones presented a great health risk when consumed on a recurrent basis.
Several epidemiological studies have demonstrated that the deterioration of individual health in As-contaminated environments is directly proportional to the As concentration in the water, years of continuous exposure, accumulation in the individual, and age of the individual (Ahmad et al. Reference Ahmad, Salim Sayed, Barua, Khan, Faruquee, Jalil, Abdul Hadi and Talukder2001; Bundschuh et al. Reference Bundschuh, Nicolli, Blanco, Blarasin, Farías, Cumbal, Cornejo, Bundschuh, Carrera and Litter2008; Castro de Esparza Reference de Esparza and Luisa2004; Figueroa Reference Figueroa2001; Hopenhayn et al. Reference Hopenhayn, Ferreccio, Browning, Huang, Peralta, Gibb and Hertz-Picciotto2003; McClintock et al. Reference McClintock, Chen, Bundschuh, Oliver, Navoni, Olmos, Lepori, Ahsan and Parvez2012; Tondel et al. Reference Tondel, Rahman, Magnuson, Chowdhury, Faruquee and Ahmad1999; WHO Reference WHO2003, Reference WHO2008). Epidemiological studies also indicate that CREHA causes acute and chronic health problems, such as retarded growth and development in children, skin lesions, increased perinatal and infant mortality, congenital anomalies, and several types of cancer (Hopenhayn et al. Reference Hopenhayn, Ferreccio, Browning, Huang, Peralta, Gibb and Hertz-Picciotto2003; Hopenhayn-Rich et al. Reference Hopenhayn-Rich, Browning, Hertz-Picciotto, Ferreccio, Peralta and Gibb2000; Tondel et al. Reference Tondel, Rahman, Magnuson, Chowdhury, Faruquee and Ahmad1999).
The As in water originates from geomorphology and volcanoes in the area. After mixing with water, it cannot be easily perceived, because it is colorless, odorless, and tasteless; that is, it is invisible to the senses. Studies of populations that inhabited the Atacama Desert must consider such contamination, especially considering the poor water quality in the water sources (Figueroa Reference Figueroa2001), the limited number of rivers (which have a low flow that concentrates minerals), and the paucity of other water sources (wells and springs) available. The first populations that explored prehistoric regions sporadically (those with rotational mobility) and those that settled permanently must have adapted to harsh living conditions in an environment with scarce water sources, which were not always of good quality. We are what we consume; therefore, studying the presence of chronic arsenic poisoning in ancient populations is important in determining the effects of prolonged exposure to endemic contaminants (Figure 1).

Figure 1. General diagram of overexposure to arsenic.
The CAM- 9 Site
The CAM-9 site is located approximately 100 km south of Arica City in the Quebrada de Camarones. It is situated on the periphery (to the southeast) of a large shell deposit, located on the southern terrace of the ravine and adjacent to CAM-8, a Late Intermediate period site (ca. AD 1400), and to CAM-14 and CAM-17, both Archaic sites. The CAM-9 site is contiguous to the coast and the mouth of the Camarones River, where its ancient inhabitants could plant crops, consume river water, and supplement their subsistence with extractions from nearby coastal resources (Figures 2 and 3). Although plants are good arsenic bioaccumulators (Ruiz and Amienta Reference Ruiz and Armienta2012), it is likely that the daily amount of arsenical water ingested significantly outweighed the quantity of arsenic ingested per day from plant or animal foods. Yet, the consumption of edible plants irrigated with arsenical water was likely another source of arsenic contamination (Arriaza et al. Reference Arriaza, Dulasiri Amarasiriwardena, Vivien Standen, Bartkus and Bandak2010; Cornejo et al. Reference Cornejo, Lienqueo, Arriaza, Acarapi and Arenas2008).

Figure 2. Map illustrating the location of the archaeological sites mentioned.

Figure 3. Surrounding area of CAM-9 site and terrace, showing the fertility of the river delta (photograph by Carlos Chow). (Color online)
Dating to the Inca period, the CAM-9 site shows evidence of a mixed economy, which included hunting, fishing, and gathering; growing crops, including maize; and using farming tools. Vessels in the form of bowls and aryballoi were also found at the site (Muñoz Reference Muñoz1989; Schiappacasse and Niemeyer Reference Schiappacasse and Niemeyer1989). Radiocarbon dates place the site between AD 1320 and 1680 (Catalán Reference Catalán2008; Figueroa et al. Reference Figueroa, Razmilic, Allison and González1988; Table 1). According to Muñoz (Reference Muñoz1989:103), an Altiplanic influence is suggested by the ceramics found at CAM-9, which were similar to the chilpe and saxamar styles. Based on radiocarbon dates, this pottery was attributed to the highland valleys of Arica and Camarones between AD 1220 and 1400. He suggests that there was interaction between coastal groups and Altiplanic populations, as well as an Inca population dedicated to agricultural and maritime exploitation, in CAM-9.
Table 1. Radiocarbon Dates of the CAM-9 Site.

CAM-9 has been excavated twice. It was excavated in the early 1960s by a team from the Regional Museum of Arica (led by Percy Dauelsberg), who excavated the majority of the funerary contexts, leaving the bodies in situ. In 1985, Percy Dauelsberg and Vivien Standen of the University of Tarapacá excavated the site again, recovering bodies that had been left in situ and other contexts that had not been disturbed. They uncovered 41 tombs, located in pits with individual burials (Carmona Reference Carmona2004). Of these individuals, 20 were male, 7 were female, and in 14 cases, sex was undetermined (Muñoz Reference Muñoz1989). The most frequent offerings were hunting and fishing artifacts (e.g., harpoons and lithic weights), textiles (wool bags), food remains, and symbolic ritual objects.
The Inca tombs found at the CAM-9 site resembled circular or oval pits whose diameters ranged from 30 to 90 cm, with some exceeding 100 cm. The bodies were adorned with thick braids, some in the form of a set of small braids at the back of their heads and others with lateral braids. The bodies were placed in a squatting position and then wrapped from head to toe in bichrome camelid wool blankets (brown with some striped decorations on both sides) to form a burial bundle (Figure 4). The bundles were buried at a depth of 40–80 cm. In this period, the bodies and bundles were always painted red, the blankets had red lateral stripes, and some elements of the offering that accompanied the deceased were also painted red (Ulloa et al. Reference Ulloa, Standen and Gavilán2000). A headdress (Figure 5), possibly made with Otaria sp. filaments (fastened with wool at its base) or with seabird feathers, was placed at the head level. Some of these headdresses were accompanied by a row of projectile points. In other cases, cephalic ornaments were simpler, and wool yarn was placed as a headband.
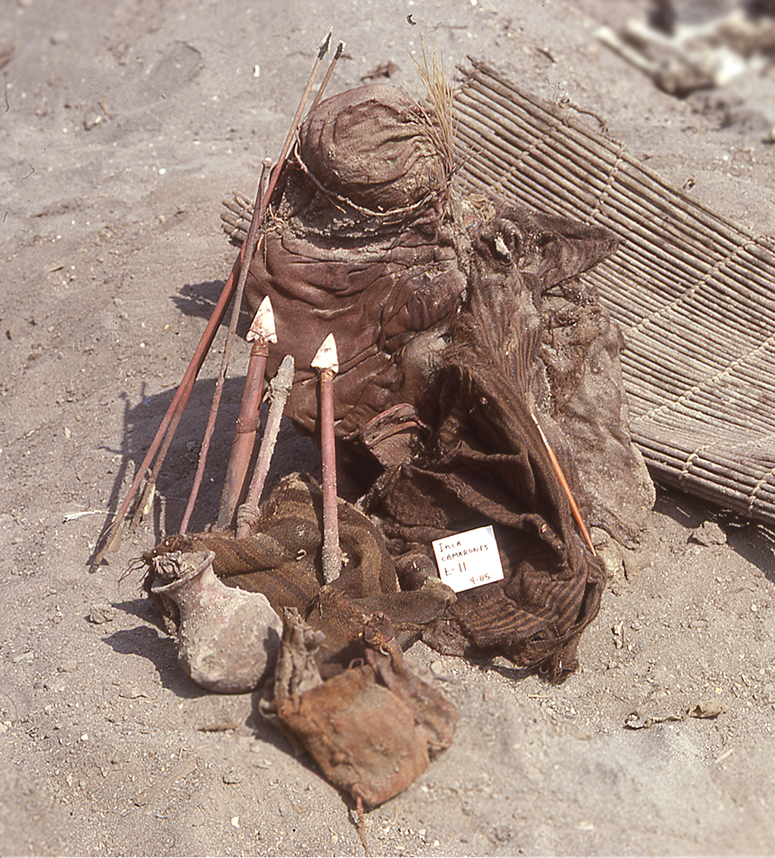
Figure 4. Complete undisturbed tomb, with its grave goods (harpoons, shafts, bags, and a vessel) and cephalic headdress possibly made of Otaria sp. filaments (CAM-9 Tomb 11) (photograph by Vivien Standen). (Color online)
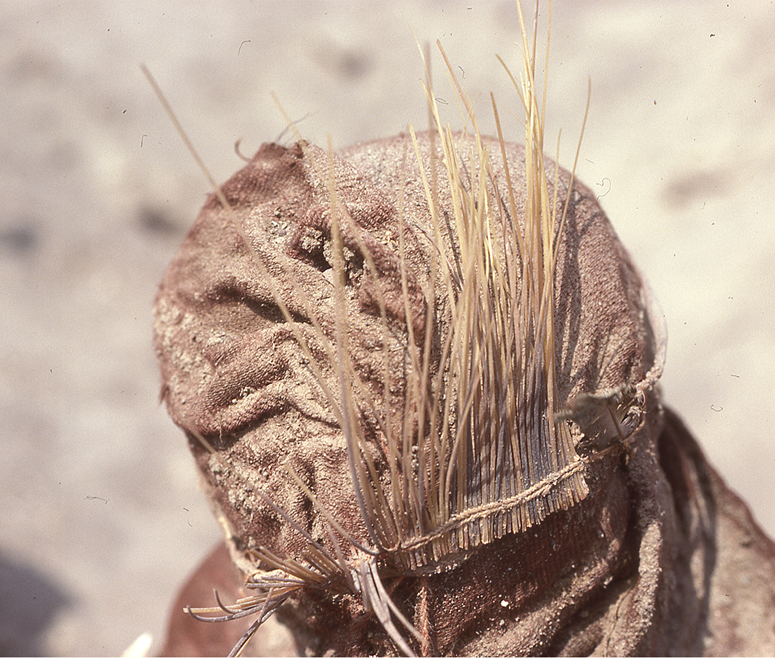
Figure 5. Detail of headdress made of possible Otaria sp. filaments (CAM-9 Tomb 11) (photograph by Vivien Standen). (Color online)
Horta (Reference Horta2015:390) stated that CAM-9 was a camanchaca (coastal fishing) population: “regarding the aforementioned cemeteries for the relocated population, I have concluded that, on the contrary, the population of Playa Miller 6 and Camarones 9 would have been camanchacas and would have survived as transculturated camanchacas until posthispanic times” (translation by the authors). She also argued that the main activity they engaged in was hunting sea lions and cetaceans (Horta Reference Horta2015:334).
Previous Bioanthropological Studies in CAM-9
Figueroa and colleagues (Reference Figueroa, Razmilic, Allison and González1988) reported on 31 unbundled and autopsied bodies from Camarones to quantify the degree of As intoxication in various tissues (mainly soft). They found that 84% of the bodies exhibited skin lesions and that the analyzed organs far exceeded the As intake values established as normal, finding values that were 342 (kidneys), 113 (liver), 44 (skin), 34.4 (hair), 29.3 (nails), and 13 times (bones) the recommended norm. This concentration of As retained in different body tissues indicates that the ancient inhabitants of CAM-9 consumed high levels of As, especially from geologically contaminated water. They found that skin lesions correlated positively with age: the older the individual, the higher the number of skin lesions present. In contrast, studies focusing on the skin of individuals from Azapa archaeological sites, such as AZ-14 (N = 13), AZ-71 (N = 12), and AZ-140 (N = 19), did not exhibit signs of hyperkeratosis or skin lesions that could be associated with arseniasis (Arriaza Reference Arriaza2021).
Another study found that individuals from CAM-9 had a significantly higher frequency of spina bifida occulta in the sacrum—a defect of multifactorial and environmental origin associated with the interruption of the neural tube closure—than did the ancient populations of Arica (Lluta 54 and Azapa 140 sites combined) with values of 13.5% and 2.4%, respectively (Silva et al. Reference Silva, Arriaza and Standen2010). The CAM-9 population had spina bifida occulta values that were nearly six times higher than those of the Lluta and Arica Valleys; this reflected greater environmental stress, because inorganic As crosses the placental barrier and directly causes the development of congenital malformations, such as spina bifida. In addition, studies of As levels in the hair of mummies from Atacama in northern Chile, using laser ablation mass spectrometry, revealed that these levels were much higher in the coastal populations than in the valleys (Arriaza et al. Reference Arriaza, Dulasiri Amarasiriwardena, Vivien Standen, Bartkus and Bandak2010). Of the four CAM-9 individuals reported, all had values greater than 1 μg/g (the norm for hair), and two reached levels around 27 and 47 times this value. From a comparative regional perspective, Echeverría and colleagues (Reference Echeverría, Niemeyer, Muñoz and Uribe2018) quantified As in hair (AsH) from the agroceramic period of northern Chile (Pica-8, Topater, and San Pedro de Atacama). They found significant differences in AsH between groups but no clear association with water sources. Interestingly a few individuals from Pica-8 and Topater presented extremely high AsH values. Echeverría and coauthors (Reference Echeverría, Niemeyer, Muñoz and Uribe2018) argued that they were nonlocal individuals who had presumably used rich As resources. In addition, Swift and colleagues (Reference Swift, Cupper, Greig, Westaway, Carter, Santoro, Wood, Jacobsen and Bertuch2015) reported on 21 precontact skeletal coastal samples from Caleta Vitor (about 52 km north of Camarones) that presented 33% arseniasis (>1 ppm), demonstrating the continuing risk of arsenic poisoning over several millennia of occupation at one site (ca. 3900–500 cal BP). Even though their sample size was small, Swift and colleagues (Reference Swift, Cupper, Greig, Westaway, Carter, Santoro, Wood, Jacobsen and Bertuch2015) stated that Tiwanaku through to Inca cases presented much higher As concentrations values (mean of 2.736 ppm).
At CAM-9, other bioarchaeological data indicate that the site had a mixed economy (Arriaza, Huaman, et al. Reference Arriaza, Amarasiriwardena, Standen, Yáñez, Van Hoesen and Figueroa2018). Analysis of dentition revealed a high percentage of caries, dental calculus, and plant consumption (e.g., Zea mays, Phaseolous sp., among others). In addition, paleopathological studies of 17 skulls of CAM-9 children revealed that 65% had mild to moderate crib lesions (Brito Reference Brito2020), reflecting health problems that were most likely products of anemia and poor water quality. Analysis of the presence of bony growth in the ear canals (external auditory exostosis) in 58.3% (7 of 12) of the CAM-9 cases suggests the intensive practice of maritime activities. In addition, these auditory exostoses are larger than those of individuals in other coastal populations in the region, most likely reflecting the continuous exploitation of coastal resources (Standen et al. Reference Standen, Arriaza and Santoro1997).
Thus, various studies conducted on the CAM-9 population reveal the presence of pathology associated with a high concentration of arsenic. However, these analyses do not delve into the characteristics of this population or the causes of their arsenicism. Our study provides new analytical data arguing that the CAM-9 population is a foreign group and therefore susceptible to arsenic.
Materials and Methods
Bioarchaeological Sample
To contextualize the archaeological samples, we reviewed archival records of the Museo Arqueológico de la Universidad de Tarapacá in San Miguel de Azapa (MASMA) and related published papers (e.g., Figueroa Reference Figueroa2001; Figueroa et al. Reference Figueroa, Razmilic, Allison and González1988). MASMA also houses the skeletons and soft tissue of many of the mummies autopsied during the 1980s. In this study, we sampled 16 of these skeletal remains for chemical analysis. We knew the sex of most of these remains because the external sex organs remained intact. When soft tissue was not present, we estimated their sex using standard protocols for pelvic and cranial characteristics (Buikstra and Ubelaker Reference Buikstra and Ubelaker1994). Eleven individuals were female, and five were males. Age was determined by observing the stage of development of dental eruption and bone growth (e.g., epiphyseal fusion stages) and by using standard scoring of the pubic symphysis (Brooks and Suchey Reference Brooks and Suchey1990) for younger people and the auricular surface for adults (Lovejoy et al. Reference Lovejoy, Meindl, Pryzbeck and Mensforth1985). Following Buikstra and Ubelaker (Reference Buikstra and Ubelaker1994), we grouped the 16 individuals into these age categories: four were adolescents (12–20 years), three were young adults (20–35 years), seven were middle adults (35–50 years), and two were older adults (50+ years).
Diagenesis
Previous studies using ancient samples from the Atacama Desert indicate that diagenetic contamination is absent or minimal in mummy tissues due to the absence of rain (Byrne et al. Reference Byrne, Amarasiriwardena, Bandak, Bartkus, Kane, Jones, Yáñez, Arriaza and Cornejo2010; Kakoulli et al. Reference Kakoulli, Prikhodko, Fischer, Cilluffo, Uribe, Bechtel, Fakra and Marcus2014); the clothing and wrappings of Andean mummies also protected buried bodies from harsh environments. The mummy bundles in CAM-9 were found on the dry sand slopes of the Atacama Desert, away from the local shallow rivers. The Camarones River is seasonal, exoreic, and has a low annual flow; for example, in 2018 it had a water flow of 1.0 m3/s (DGA 2019). Therefore, it likely did not affect this archaeological site or its remains. In addition, the prevalence of salts in the soil of the region prevents the proliferation of microorganisms that degrade collagen, thus contributing to keeping the bone matrix of bioarchaeological samples intact (Barrientos et al. Reference Barrientos, Sarmiento and Galligani2016) and allowing various archaeometric studies to be conducted.
Chemical Analysis of the Bioanthropological Samples
We followed protocols of the analytical chemistry laboratory at the Universidad de Tarapacá in Arica to analyze the samples.
First, each sample was cleaned mechanically and with a no. 21 sterile scalpel, and all external organic and inorganic material present in the remains of the ribs sampled were removed. A minimum of 1 g of clean bone sample was obtained. Second, clean bone pieces for each sample were fragmented and reduced to <0.5 mm particle sizes in an analytically clean and dry porcelain mortar. They were then stored in clean, dry, and sealed bottles with identification labels.
Third, the ground rib bone samples were subjected to chemical dissolution. Chemical digestion, in a mixture of acids (nitric and hydrochloric), allows the solubilization of minerals, such as apatite, hydroxyapatite, and fluorapatite, and the larger fraction of the bone. The acid mixture facilitates the release and transformation of As from the bone to a chemical compound, such as the acid arsenate anion, H2AsO4-; this is an arsenical chemical species that is soluble in the aqueous and acid medium, representing, to a maximum degree, the total As present in the rib bone.
In a 100 mL Pyrex beaker, 1 g ± 1 × 10–4 g of the ground rib bone sample was weighed, to which we added 5 mL of fuming hydrochloric acid 37% (w/w) for analysis of EMSURE® ACS, ISO, and REAG. Ph Eur; we then added 2 mL of nitric acid 65% (w/w) for analysis of EMSURE® ISO. This heterogeneous mixture was placed in a vessel covered with a convex Teflon plate, 70 mm in diameter. It was heated on a heating plate in a semi-closed system until the solid material was boiling and totally dissolved and the organic matter obtained partial oxidation. This process was completed when NOx gas, which is brownish in color, was emitted.
The resulting solution was diluted in a beaker, using 10 mL of demineralized water, free of As, and then brought to a slow boil again for two to three minutes. It was then allowed to cool to 20°C–21°C, and the solution was subsequently increased to 25 mL using demineralized water. The resulting solution was then homogenized, filtered through ADVANTEC 5 B quantitative ash-free filter paper with a diameter of 110 mm, placed over a Pyrex analytical funnel, and transferred to a hermetically sealed and properly identified polypropylene bottle. The digested sample was used in this state for As quantification.
At this stage, the As concentration in the rib sample solution, which contained the original As in the transformed sample, was measured using the arsenamine hydride (AsH3) gas-generation methodology, and calculated by the stoichiometry of the respective chemical reaction. For this purpose, we used a continuous transport system of arsenic gas that was connected first to the quantification instrument (atomic absorption spectrophotometer with hydride generation, EAA/GH) and then to an atomization system in a quartz cell heated with an air and acetylene flame. The instrument's software, which was calibrated using standards of known As concentrations, enabled us to obtain calibration parameters that established the degrees of precision and sensitivity of the measurement, as well as the As concentration data in the observed or measured solution. The concentration data obtained from each sample are related to the quantitative data from the digestion solution achieved and the mass of the initial solid sample. The As concentration in the rib sample was thus obtained, which can be expressed in units such as mg As/kg of rib or μg As/g of rib or simply ppm; that is, part of As per million parts of rib.
The quantification analytical procedure was conducted on the solubilized rib sample in an aliquot in a volumetric flask of a defined total volume and conditioned to a concentration of 1 M HCl and of potassium iodide (KI) at 1% mass/volume. The total mixture was homogenized and absorbed simultaneously into the Agilent Technologies VGA 77 continuous flow system through line 1. This was conducted in parallel with the absorption of a 5 M hydrochloric acid (HCl) solution through line 2 and of a reducing reagent, sodium borohydride (NaBH4) of concentration 0.6% mass/volume in sodium hydroxide 0.5% mass/volume. The reagent mixture transformed the As element in the solution into the gaseous arsenamine or As hydride (AsH3), a gas phase that is transported by the nitrogen gas in the system, with a continuous flow, to the atomization cell of the Agilent Technologies 240FS AA atomic absorption instrument, where the As concentration was quantified.
The different instrumental quantification operations were achieved based on the instrumental conditions shown in Table 2.
Table 2. Equipment Specifications.

Statistical Analysis
We first calculated z-values to exclude outliers or extreme cases (z > 3), keeping 99.7% of the most representative cases. Then, we conducted a descriptive analysis of the As concentrations in the rib samples and a statistical contrast of means in the sex variable using Student's t-test. Analysis of the presence of arsenic in ribs according to age ranges using the Shapiro-Wilk test showed that they were not normally distributed. Consequently, the Kruskal-Wallis test was performed, which determined no significant differences between the groups.
To establish a comparative reference standard, we gathered data on current As concentrations in the water sources found in the three main valleys of the northern zone of Chile (Table 3) and the maximum values of As in the human bone matrix, according to global health organizations, such as WHO.
Table 3. Summary of Arsenic Concentration Levels in the Main Water Sources of Northern and Central Chile.

Sources: Compiled from Cornejo Reference Cornejo2004; Ferreccio et al. Reference Ferreccio, González, Milosavjlevic, Marshall, Sancha and Smith2000; Figueroa Reference Figueroa2001; NCh 2005; Niemeyer Reference Niemeyer1980; Schull et al.Reference Schull, Blago Razmilic, González, Schull and Rothhammer1990; WHO Reference WHO2006; Yáñez et al. Reference Yáñez, Fierro, Mansilla, Figueroa, Cornejo and Barnes2005.
Results
The overall sample (N = 16; Table 4) showed a median of 2.2 μg/g for As in bones (mean = 3.4, SD = 3.1, minimum = 0.2, maximum = 9.3). However, in 12 individuals (75%), the presence of As in the bone matrix was above the level recommended by WHO (1 μg/g); this increased these individuals’ median to 3.6 μg/g (mean = 4.3. SD = 3.0, minimum = 1.4, maximum = 9.3). Both males and females were affected, and no cases of extreme outliers (z > 3) or significant differences between sexes were observed (t = 2.78 [2 tails], p = 0.19). In the sample, individuals in all the age categories showed As median values higher than the recommended 1 μg/g: adolescents = 2.6 μg/g, young adults = 8.8 μg/g, middle adults = 1.4 μg/g, and older adults = 5.2 μg/g. Three individuals—one young female, one older female, and one young male—had levels about nine times higher than the WHO recommended values (Table 4).
Table 4. Arsenic Values Obtained for the Analyzed Mummies.

Discussion
Previous archaeological analyses, such as studies on headdresses (Horta Reference Horta2000, Reference Horta2011a), support the presence of a foreign fishing population in Camarones Cove at the end of the Late Intermediate period (ca. AD 1000–1450). Similarly, Muñoz (Reference Muñoz1989:95) states, “The late settlement of the Desembocadura del Río Camarones is framed within this same population structure; that is, groups with a wide domain of the sea and that work on the land, interacting with populations linked to a pre-Inca and later Inca Altiplanic tradition” (translation by the authors). In addition, coastal technological elements—such as rafts, capacacho baskets, harpoon holders, copper hooks, and harpoons with copper barbs for fishing—and fishing for deep-sea species would have caused an increase in surplus (e.g., dried fish), facilitating exchange with inland populations (Horta Reference Horta2000, Reference Horta2011b; Llagostera Reference Llagostera1990; Muñoz Reference Muñoz1989; Núñez 1986). Therefore, a nonlocal population likely inhabited the Camarones coast, was assigned to carry out productive tasks, and suffered the consequences of a quietly hidden toxic environment. This hostile environment was paradoxically near a river mouth that stood out due to the beauty of its landscape, with a large wetland and abundance of birds and coastal fauna.
Populations that are suddenly exposed to highly arsenical waters are at high risk of arseniasis. Two epidemiological examples validate this point. Millions of people in many districts of Bangladesh have become poisoned since the 1990s due to the use of deep tube wells containing levels of arsenic of 100 μg/L (Ahmad et al. Reference Ahmad, Salim Sayed, Barua, Khan, Faruquee, Jalil, Abdul Hadi and Talukder2001; Alam et al. Reference Alam, Allinson, Stagnitti, Tanaka and Westbrooke2002). In the second case, a massive intoxication occurred in Antofagasta City in Chile in the 1960s when mining activities led to a shortage of freshwater. The Toconce River, which contains 800 μg/L of arsenic, was connected to the main street water system. Soon after, people began to experience multiple health problems linked to arsenic in the water (Arriaza and Galaz-Mandakovic Reference Arriaza and Galaz-Mandakovic2020; Hopenhayn et al. Reference Hopenhayn, Ferreccio, Browning, Huang, Peralta, Gibb and Hertz-Picciotto2003; Hopenhayn-Rich et al. Reference Hopenhayn-Rich, Browning, Hertz-Picciotto, Ferreccio, Peralta and Gibb2000).
Similarly, foreign populations without previous adaptation to high levels of As and who settle in an arsenical area to exploit its natural resources face a potential health risk by exposing themselves to a new, naturally contaminated environment. In our study, 75% of the individuals buried in CAM-9 presented As values in their ribs that were higher than the norm. Arsenic bone values were 3.6 times higher (median) than the recommended limit, with some reaching up to nine times higher on average (Table 4). In addition, individuals in every age category were significantly affected, ranging between an average As bone value of 2.5 and 7.3 times above the recommended values. Archaeometric analyses of different types of matrices (e.g., hair, bone, and soft tissue) demonstrate that ancient populations of CAM-9 were significantly exposed to As, showing various manifestations of arseniasis at the somatic level independent of sex and age (Arriaza et al. Reference Arriaza, Dulasiri Amarasiriwardena, Vivien Standen, Bartkus and Bandak2010; Figueroa Reference Figueroa2001; Figueroa et al. Reference Figueroa, Razmilic, Allison and González1988). Unfortunately, there were no satisfactory bioanthropological collections available from Camarones to test the complete cultural sequence of human adaptation and exposure to As.
In addition to high levels of arsenic in bones, macroscopic skin lesions observed in CAM-9 in previous studies (Figueroa et al. Reference Figueroa, Razmilic, Allison and González1988) are relevant because they provide a visible sign of a somatic alteration with arsenical etiology. Similarly, the bodies in CAM-9 presented very high concentrations of As in their organs, with average values between three and 342 times above the norm, depending on the analyzed tissue (Figueroa et al. Reference Figueroa, Razmilic, Allison and González1988). This evidence, in conjunction with the high prevalence of spina bifida occulta in CAM-9 individuals (Silva et al. Reference Silva, Arriaza and Standen2010), reinforces the hypothesis that the population of CAM-9 was exposed for the first time to an arsenical environment and suffered from severe arseniasis after settling in the Camarones Valley and drinking the highly contaminated waters. The most plausible explanation is that this population had no natural resistance to As and may represent a new population (mitimaes) that originated from less contaminated environments. In other words, they did not have the genetic makeup to tolerate or eliminate the As, leading to many health problems.
Increasingly, research demonstrates that after multiple generations have lived in arsenic-rich environments, adaptation to this poisonous element occurs. For instance, modern populations from northern Chile (Camarones) and Argentina (San Antonio de los Cobres, SAC) that have been exposed to arsenical environments demonstrate genetic variants (AS3MT) that help them methylate and excrete As: they have adapted to this invisible environmental toxin and can ingest it without experiencing negative consequences to their health (Apata et al. Reference Apata, Arriaza, Llop and Moraga2017; Eichstaedt et al. Reference Eichstaedt, Antao, Cardona, Pagani, Kivisilda and Mormina2015; Schlebusch et al. Reference Schlebusch, Lewis, Vahter, Engström, Tito, Obregón-Tito and Huerta2013). According to Yáñez and colleagues (Reference Yáñez, Mansilla, Paola Santander, Fierro, Cornejo, Barnes and Amarasiriwardena2015:7), “It appears that exposure to higher concentrations of arsenic (V) would eventually enhance the methylation capability by the liver leading into a more efficient elimination of ingested arsenic body burden through urine.” In addition, several studies conducted in modern populations from Camarones and SAC showed that the AS3MT enzyme plays an important role in the elimination of the ingested toxic As (Apata et al. Reference Apata, Arriaza, Llop and Moraga2017; Eichstaedt et al. Reference Eichstaedt, Antao, Cardona, Pagani, Kivisilda and Mormina2015; Schlebusch et al. Reference Schlebusch, Lewis, Vahter, Engström, Tito, Obregón-Tito and Huerta2013; Vicuña et al. Reference Vicuña, Fernandez, Vial, Valdebenito, Chaparro, Espinoza, Annemarie Ziegler and Eyheramendy2019). Approximately 68% of the modern people of Camarones and SAC carry such a protected variant, specifically the CTA haplotype (Apata et al. Reference Apata, Arriaza, Llop and Moraga2017; Schlebusch et al. Reference Schlebusch, Lewis, Vahter, Engström, Tito, Obregón-Tito and Huerta2013).
If, indeed, the samples recovered from CAM-9 represent newcomers to the region who suffered from arseniasis, then future ancient DNA studies should demonstrate that CAM-9 individuals carried a low percentage of the AS3MT protective variant. In addition, the origin of the CAM-9 population remains to be determined in future studies: Were they members of Altiplanic groups, or did they come from coastal areas (e.g., from Arica or southern Peru) and were then transferred to Camarones? Similarly, how the CAM-9 population was integrated into nearby sites in the same valley, such as CAM-12 (north slope of the Camarones River mouth) and Saguara (inland of Camarones valley), should be investigated.
The arsenicism (skin lesions, high systemic intoxication, etc.) observed in the CAM-9 individuals most likely affected their daily lives. The CREHA experienced by this displaced population probably carried aspects of emotional burden and stigma for those chronically affected. Highly visible skin lesions, together with other systemic arsenic-related pathologies, may have reduced the desirability of affected individuals, minimizing their reproductive and labor success. Ethnographic data show that women from Bangladesh affected with skin lesions due to hydroarsenic poisoning are socially very vulnerable and are often stigmatized or abandoned by their husbands. In addition, some affected young women are unable to marry (Alam et al. Reference Alam, Allinson, Stagnitti, Tanaka and Westbrooke2002; Hassan et al. Reference Hassan, Atkins and Dunn2005). One could speculate that similar problems or some social stigma occurred among heavily affected individuals from CAM-9.
In addition, the various types of symptoms and pathology produced by CREHA must have affected productivity and their tribute to the Inca in communities associated with the CAM-9 cemetery. Carmona (Reference Carmona2004) shows that in CAM-9 most of the shirts were repaired and reused, illustrating a difficult life. These data suggest a biologically and socially affected population.
Conclusions
CAM-9 represents the final period of the precontact sequence of fishermen from the extreme north of Chile. Based on the bioarchaeological data presented, we posit that it housed a population of mitimaes newly settled in an arsenic-laden environment. In addition, their grave goods suggest that the Inca moved the CAM-9 population to this coastal environment to exploit marine resources. The high concentrations of As found in various tissues of CAM-9 mummies confirm that it represents an allochthonous population that was not previously exposed to high As contents. Therefore, arsenic-laden water must have significantly affected both the health of the population and their assigned functions and productive capacity. However, the biogeographic origin of CAM-9, whether coastal or highland, is unresolved.
We conclude that environments in the past that seemed paradisiacal to settle in because of their abundance of exploitable natural resources instead presented hidden and lethal risks. These environments subjected their inhabitants to a process of adaptation that had biological, cultural, and economic costs. Consequently, when discussing cultural trajectories of ancient populations, it is of vital importance to incorporate the potential inorganic hazards of a given environment. This study highlights the importance of natural water pollution in the diaspora of the Andean populations; it generates new debates and proposals on the role of natural contamination and its consequences in the appropriation of space for human settlement, in both favorable and unfavorable conditions, while exploiting multiple ecological niches in different cultural periods. This study is relevant to all regions of the world that present ecotoxic loads, such as hydroarsenicism, as well as in the human diaspora at different moments of prehistory and universal history.
Acknowledgments
We are grateful for the funding of Fondecyt 1170120 and 1210036. We acknowledge the support of the Museo Arqueológico San Miguel de Azapa, the Chemistry Department, and the Instituto de Alta Investigación, all from the Universidad de Tarapacá, Arica, for the execution of this project. We thank Camila Contreras, Natalia Aravena, Anita Flores, and Susana Monsalve for their laboratory collaboration and Arnoldo Vizcarra for assistance with the preparation of figures. Our gratitude to the anonymous reviewers for their insightful comments and suggestions.
Data Availability Statement
The data that support the findings of this study are available from the corresponding author on request.
Competing Interests
The authors declare none.