Introduction
Suggestions that the suite of platinum-group minerals (PGM) found in tropical alluvial PGM occurrences differ from the PGM encountered in conventional deposits with an igneous origin have become more convincing in recent years. For most of this time the evidence has been circumstantial, based on occurrence and apparent growth features seen under the microscope. Without experimental evidence for supergene PGM growth there was opportunity for controversy. There is abundant evidence that the PGM can be destroyed by weathering and the PGE taken into solution. But, can new PGM develop under surface conditions? Is PGM formation under surface conditions (‘neoformation’) ‘a scientifically untenable hypothesis’? The huge contribution of Frank Reith and his co-workers was to provide a viable mechanism to explain how PGM growth under surface conditions could occur (Reith et al., Reference Reith, Campbell, Ball, Pring and Southam2014, Reference Reith, Zammit, Shar, Etschmann, Bottrill, Southam, Ta, Kilburn, Oberthür, Bail and Brugger2016). The geochemical cycle is illustrated by Fig. 1a. We address the questions “What is our current understanding?” and “What could be the trend of future studies?”

Fig. 1. PGM geochemistry: (a) the natural PGE cycle (after Reith et al., Reference Reith, Campbell, Ball, Pring and Southam2014). The numbers are an estimate of the quantities involved in grams; (b) the principal changes of Pt-rich PGM from pristine sulfide ores through the oxidised zone to rivers (after Oberthür, Reference Oberthür2018), where appropriate * indicates Pt–Pd–Bi-tellurides or Fe, Mn, Co oxides/hydroxides; and (c) the variation of Pt/Pd from source rocks through soils to natural waters (after Bowles et al., Reference Bowles, Giże and Cowden1994a).
What is our current understanding?
Although the considerable difference in grain size between tropical alluvial occurrences and primary deposits is the most obvious feature there are other significant details. Overall the differences noted by Bowles et al. (Reference Bowles, Suárez, Prichard and Fisher2013, Reference Bowles, Suárez, Prichard and Fisher2018a,Reference Bowles, Suárez, Prichard and Fisherc), Oberthür et al. (Reference Oberthür, Weiser, Melcher, Gast and Wörhl2013), Oberthür (Reference Oberthür2018) and Cabral et al. (Reference Cabral, Zack, König, Eickmann, Kwitko-Ribeiro, Tupinambá and Lehmann2019) are:
Assemblage: there is a distinctive difference between the PGM assemblage in the fresh rocks and the alluvium. PGE-sulfides, abundant in the fresh rocks, are less abundant in the streams whereas Pt–Fe alloys form a much larger proportion of the alluvial mineral suite (Fig. 1b).
Chemical composition: supergene Pt nuggets can be enriched in Se and Hg with S/Se > 1 indicative of Se recycling in the supergene environment. The Pt-nuggets (rather than Pt–Fe alloys) contain less Fe, Cu, Sn and Te compared with primary examples. They show fractionated PGE patterns with enhanced Pd but with much reduced Ru, Rh, Os and Ir contents.
Palladium: palladium-bearing minerals present in the fresh rocks are not generally encountered in the alluvium and there is a significant loss of Pd relative to Pt with distance from the source. Low Pt/Pd ratios in the host rocks rise considerably in the alluvial deposits demonstrating the greater mobility of Pd in solution (Fig. 1c). Inclusions within alluvial nuggets can contain minute Pd-bearing phases.
Copper: some Pt–Fe alloys in the placers contain a small proportion of Cu (0.3‒2.7 wt.%) that increases the compositional variability and degree of disorder. Tulameenite, hongshiite and native Cu are present and appear to be a part of an involvement of Cu in which some Pt–Fe nuggets are replaced by hongshiite at the edges (Fig. 2). Apart from the presence of smaller grains of tulameenite, these features are not encountered in unweathered igneous rocks.
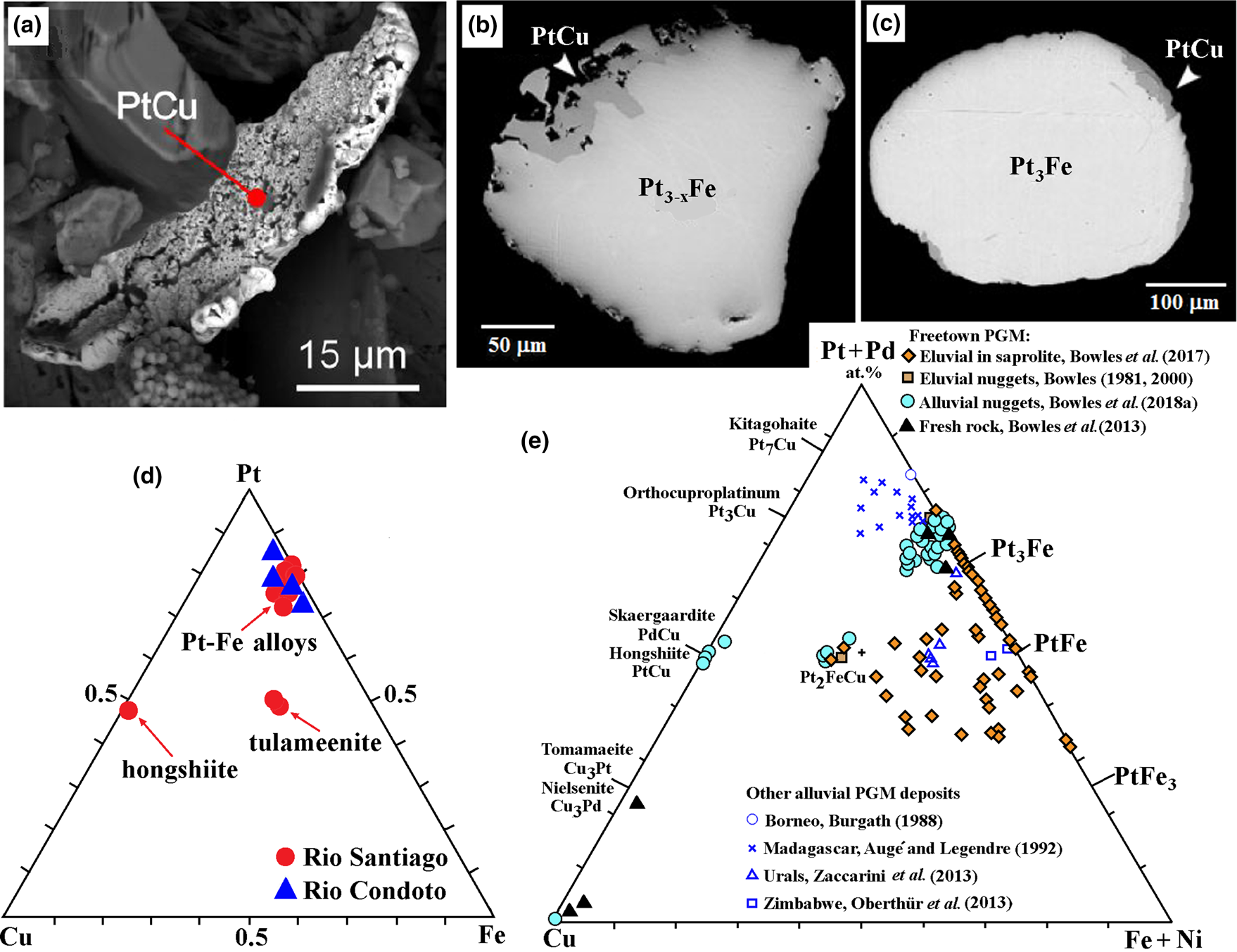
Fig. 2. Cu alloys in association with Pt–Fe (back-scattered electron images): (a) hongshiite coating alluvial Pt–Fe, Rio Santiago, Ecuador (reproduced from Aiglsperger et al., Reference Aiglsperger, Proenza, López and Arboleda2017, with permission of the Sociedad Española de Mineralogía); (b) and (c) rim alteration of Pt–Fe to PtCu in polished section, Big Water, Freetown Peninsula, Sierra Leone (reproduced from Bowles et al., Reference Bowles, Suárez, Prichard and Fisher2018a with permission of the Mineralogical Society of Great Britain and Ireland); (d) Pt–Fe–Cu diagram showing the compositions of Cu-bearing Pt alloys from the Rio Santiago, Ecuador and the Rio Condoto, Colombia (after Aiglsperger et al., Reference Aiglsperger, Proenza, López and Arboleda2017); and (e) eluvial and alluvial PGM from the Mateki (eluvial) and Big Water (alluvial) area, Freetown Peninsula, Sierra Leone compared with other examples from the literature (after Bowles et al., Reference Bowles, Suárez, Prichard and Fisher2018a, including data from Bowles, Reference Bowles1981, Reference Bowles2000; Bowles et al., Reference Bowles, Suárez, Prichard and Fisher2013, Reference Bowles, Suárez, Prichard and Fisher2017, Reference Bowles, Suárez, Prichard and Fisher2018a; Burgath, Reference Burgath, Prichard, Potts, Bowles and Cribb1988; Augé and Legendre, Reference Augé and Legendre1992; Zaccarini et al., Reference Zaccarini, Pushkarev, Garuti, Krause, Dvornik, Stanley and Bindi2013 and Oberthür et al., Reference Oberthür, Weiser, Melcher, Gast and Wörhl2013).
Oxides: weathering of PGM in the host rocks produces PGE oxides and hydroxides as a transitional stage in the alteration of the primary PGM. Their textures and locations at grain boundaries indicate that they are the result of alteration of the primary PGM and the mobility of the PGE (Fig. 3). Often the PGE oxides and hydroxides are intergrown with Fe oxides and hydroxides. Examples of intergrowths of unoxidised PGM and Fe oxides are also known and it can be difficult to distinguish intergrowths containing PGE oxides from those containing unoxidised PGM when the intergrowths occur on the very small scale. Larger examples of Pd oxide, associated with hematite, occur at Gongo Soco, Brazil (Cabral and Lehmann, Reference Cabral and Lehmann2003).

Fig. 3. Weathered and disordered PGM (back-scattered electron images). (a) Sponge texture Pt–Fe intergrown with Fe hydroxide, Hartley Mine, Great Dyke, Zimbabwe (reproduced from Oberthür, Reference Oberthür2018). (b) to (e) Weathered rocks in saprolite, Mateki, Freetown Peninsula, Sierra Leone (reproduced from Bowles et al., Reference Bowles, Suárez, Prichard and Fisher2017): (b) cooperite in pentlandite altering to produce flame-like textures; (c) and (d) cooperite at the edge of chalcopyrite altered to give an effervescent outgrowth of Pt–Fe and Pt oxide intergrown with Fe oxides/hydroxides; and (e) cooperite altered to produce a Pt–Fe reaction front. (f) PGE oxide containing ~50% Pt, the spongy areas contain the least Pd (1–4%) and the most O (8–15%) Nurali chromitite, Southern Urals, Russia (reproduced from Zaccarini et al., Reference Zaccarini, Garuti, Bakker and Pushkarev2015); (g) porous native Pt, Makwiro River, Zimbabwe (reproduced from Oberthür et al., Reference Oberthür, Weiser and Gast2003); (h) cooperite surrounded by porous Pt–Fe–O alteration; (i) ordered and disordered Pt–Fe alloys, Mateki, Freetown Peninsula, Sierra Leone (reproduced from Bowles et al., Reference Bowles, Suárez, Prichard and Fisher2017, including data from Bowles, Reference Bowles1981, Reference Bowles2000; Bowles et al., Reference Bowles, Suárez, Prichard and Fisher2013; Oberthür et al., Reference Oberthür, Weiser, Melcher, Gast and Wörhl2013 and Zaccarini et al., Reference Zaccarini, Pushkarev, Garuti, Krause, Dvornik, Stanley and Bindi2013).
Disordering: the Pt–Fe alloys in the weathered rocks show an almost continuous range of composition from Pt3Fe to PtFe and nearly to PtFe3, indicating varying degrees of disordering due to low-temperature alteration (Fig. 3i). The alluvial Pt–Fe nuggets are closer to Pt3Fe.
Size: there is often an order of three magnitude difference in size between the PGM seen in the fresh rocks (typically 0.1 to 10 μm) compared with the larger alluvial mineral suite (0.5 to 5 mm) although large PGM derived from the host rock as a resistate phase have also been reported (e.g. Oberthür, Reference Oberthür2018).
Delicate crystals: the delicate and skeletal nuggets suggest a local origin; damaged nuggets have had a longer residence time in the rivers.
Well preserved crystal forms: some PGM show nearly perfect crystal faces with sharp inter-facial angles. These include edges and corners that have not been damaged by movement in a stream (Fig. 4a,b,f).

Fig. 4. PGM overgrowths and epitaxial growth (back-scattered electron images): (a) to (c) over-plating of erlichmanite over a resorbed surface, Guma Water, Freetown Peninsula, Sierra Leone, (reproduced from Bowles, Reference Bowles1986, with permission of the Society of Economic Geologists); (d) secondary colloform zonation and over-plating of erlichmanite Guma Water, Freetown Peninsula, Sierra Leone, Os-rich regions are lighter in colour, less Os-rich regions appear as tones of grey (redrawn from Hattori et al., Reference Hattori, Cabri and Hart1991); (e) arborescent Pt–Pd grain, Pt-rich areas shown as white, Pd-rich areas in grey from Córrego Bom Sucesso, Minas Gerais, Brazil (reproduced from Cabral et al., Reference Cabral, Beaudoin, Choquette, Lehmann and Polônia2007); (f) Os–Ir alloy platelets formed simultaneously with Pt–Fe alloy Guma Water, Freetown Peninsula, Sierra Leone (reproduced from Bowles, Reference Bowles1995, with permission of the BRGM); and (g) laurite epitaxial growth on isoferroplatinum showing the shared surface, Big Water, Freetown Peninsula, Sierra Leone (reproduced from Bowles et al., Reference Bowles, Suárez, Prichard and Fisher2018a).
Overgrowths of PGM on PGM: there are textures indicative of resorption of the PGM surface and then regrowth, the regrown surfaces forming the nearly perfect crystal faces (Fig. 4a–c).
Internal zonation: primary igneous compositional zonation is known to occur, but the convoluted patterns of zonation seen in some alluvial grains is comparable with that described as due to secondary formation in other ore mineral occurrences (Fig. 4d).
Inclusions: silicate inclusions with mineralogy comparable to the host rock can be considered as inclusions of primary material. Inclusions of PGM filling negative crystal voids clearly post-date their host and those present in alluvial PGM indicate continuing PGE development.
Organic coating: the coating of organic material on the nuggets is evidence of an association between the nuggets and organic material that may indicate involvement of organic material or bacterial action during formation of the nuggets. Alluvial PGM from Big Water, Freetown Peninsula, Sierra Leone have a green or green–brown rather slimy organic coating that requires immersion in acid to remove it. PGM from Córrego Bom Sucesso, Minas Gerais, Brazil (Fig. 5a,b) and the Wilson River, Tasmania, Australia (Fig. 5c,d) are covered by a bacterial biofilm comparable to those produced in the laboratory (Fig. 5e,f). Organic material present in PGE-bearing soil from the forest floor (Fig. 5g) appears to differ from that normally encountered (Fig. 5h) and the PGE may act as a catalyst during breakdown of the organic compounds (Fig. 5i).
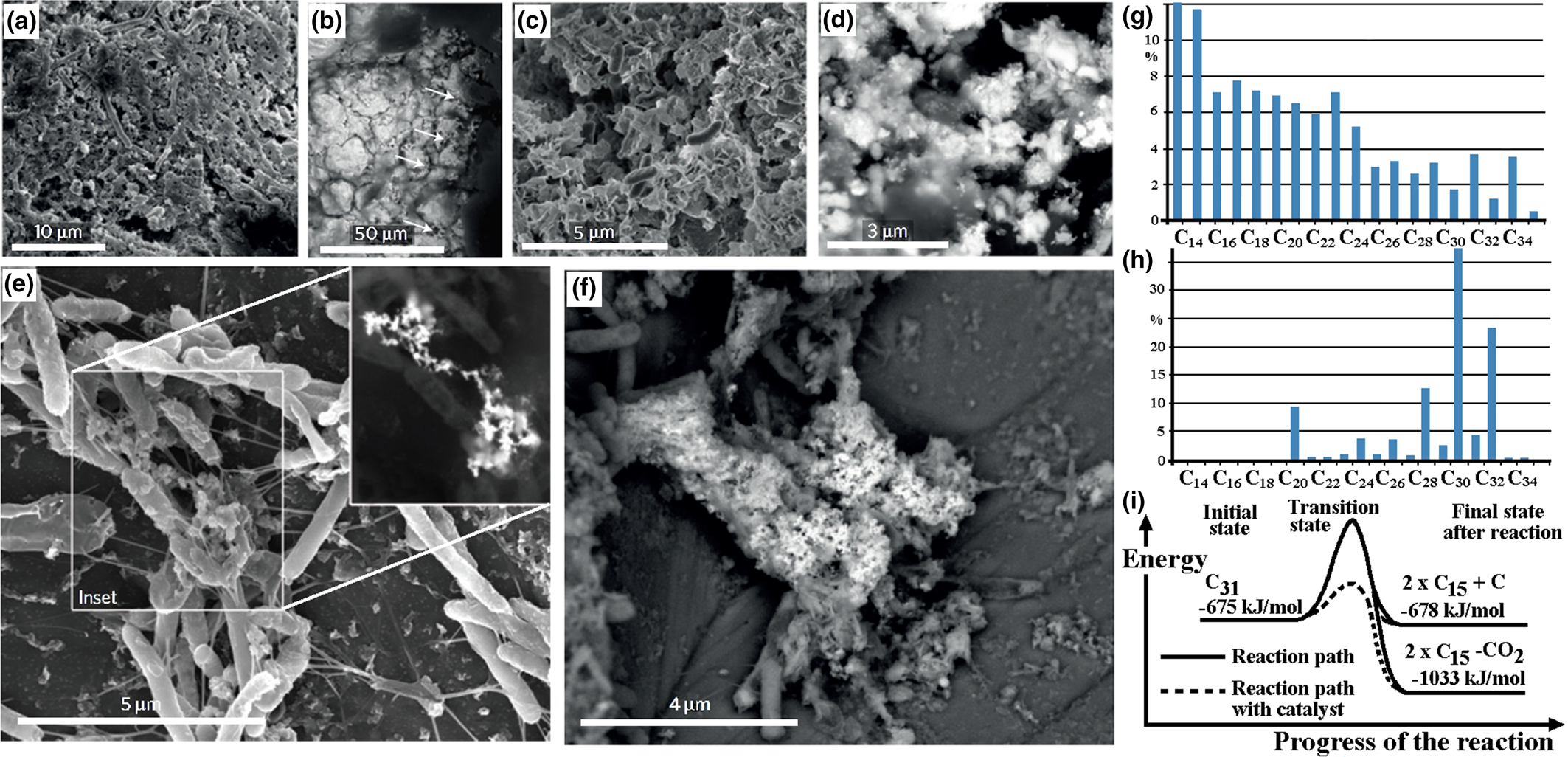
Fig. 5. The organic-PGM association: (a) secondary electron image of a bacterial biofilm covering a botryoidal Pt–Pd grain from Córrego Bom Sucesso, Minas Gerais, Brazil; (b) back-scattered electron image of (a) containing nano-crystalline Pt (arrowed); (c) secondary electron image of bacterial cells with nano-aggregates of Ir; (d) back-scattered electron image of (c) showing polymorphic biofilm layers coating Ir–Os grains from the Wilson River, Tasmania, Australia; (e) secondary electron image of Pt nano-particles formed by bacterial activity of C. metallidurans in column experiments with (inset) back-scattered electron image of the Pt nano-particles; (f) secondary electron image showing the subsequent formation of larger Pt aggregates with biofilms – (a) to (f) reproduced from Reith et al. (Reference Reith, Zammit, Shar, Etschmann, Bottrill, Southam, Ta, Kilburn, Oberthür, Bail and Brugger2016); (g) the n-alkane (C14 to C32) content of a soil sample from Mateki, Freetown Peninsula, Sierra Leone showing the bias towards the lighter C14 to C15 alkanes; (h) the n-alkane content of a non-PGE-bearing moist tropical forest soil at Debu, Cameroon showing the typical bias towards heavier C31 alkanes; (i) the reaction path for breakdown of C31 to C15 requires less energy in the presence of a Pt catalyst – (h) to (i) reproduced from Bowles et al. (Reference Bowles, Bowles and Giże2018b).
The eluvial regime
The PGM from the eluvial deposit in laterite at Yubdo, Ethiopia were examined by Augustithis (Reference Augustithis1965) and Ottemann and Augustithis (Reference Ottemann and Augustithis1967) who suggested that they may be secondary in origin. Other authors (Cabri and Harris, Reference Cabri and Harris1975; Cabri et al., Reference Cabri, Criddle, Laflamme, Bearne and Harris1981) took the opposing view, insisting that they are of magmatic origin.
Cousins and Kinlock (Reference Cousins and Kinlock1976, p. 1377) noted “recent alluvial platinoids … are predominantly alloys of the platinum-group elements … that show both rounded and irregular outlines, and … are much larger than known primary grains of platinoid minerals … features .. observable not only in alluvial grains but also in the eluvial grains from the Birbir deposit of Ethiopia. Zoning and inclusions are present. … The authors consider the possibility that the particles originated largely from the weathering and accretion of platinoids … and not altogether from the liberation of metallic platinoid particles contained in (the mother) rock.”
Studies of the PGE in solution (Fuchs and Rose, Reference Fuchs and Rose1974; Westland, Reference Westland and Cabri1981; Hodge et al., Reference Hodge, Stallard, Koide and Goldberg1985; Wood and Vlassopoulos, Reference Wood and Vlassopoulos1990) demonstrated that the PGE can move in solution in natural waters at ambient temperatures in much the same way that gold can be transported in laterites and ground water (Mann, Reference Mann1984). A significant feature is that Pd is retained in solution to a greater extent than Pt so that Pt tends to come out of solution fairly close to the source whereas Pd is carried much further away (Fig. 1c).
Description of the eluvial PGM from the Freetown Peninsula, Sierra Leone, showed “well preserved crystals of laurite-erlichmanite ((Os, Ru)S2), Os-Ir alloys and large nuggets of platinum-iron alloy present in laterites … notable for their very smooth crystal faces and sharp interfacial angles which have clearly not suffered abrasion” (Bowles, Reference Bowles1986). Pollett (Reference Pollett1931) noted the presence of large nuggets (one of 250 g) and the presence of delicate, dendritic growths. Some crystals show surface features consistent with resorption and, in some cases, subsequent regrowth or ‘over-plating’ (Fig. 4a–c).
In an attempt to rationalise these observations Bowles (Reference Bowles1986) considered the acidity (pH 3 to 5) and high Eh (0.4 to 1.0) of lateritic soils and showed that these conditions are comparable with those needed to take Pt and Pd into solution as chloride species. Chloride solutions appear to be a contributory factor in some occurrences and the association with Cu also appears relevant. In some deposits this leads to the presence of hongshiite (PtCu), which has only “ever been reported from … mafic-ultramafic rocks and associated placer deposits” (Kwitko et al., Reference Kwitko, Cabral, Lehmann, Laflamme, Cabri, Criddle and Galbiatti2002). These authors offered a hydrothermal reaction at 180°C, with a salinity of 15 wt.% NaCl equivalent between PtCl4 and CuCl2 in the presence of magnetite and water, that produced hematite as a pseudomorph after magnetite, with hongshiite formed on the mineral surface. Subsequent supergene leaching of Cu from the hongshiite left porous rims of nearly pure Pt. It was Na and Ca brines that were responsible for hydrothermal (86 ± 13°C) veins that host the Au–Pd mineralisation in the Permo–Triassic basins of southwest England (Shepherd et al., Reference Shepherd, Bouch, Gunn, McKervey, Naden, Scrivener, Styles and Large2005). Cabral et al. (Reference Cabral, Galbiatti, Kwitko-Ribeiro and Lehmann2008) examined fracturing of hongshiite that they considered “diagnostic of a low-temperature origin” that “adds further evidence for Pt mobility and concentration in non-magmatic systems”.
The occurrence at Yubdo, Ethiopia, needs some explanation. Cousins and Kinlock (Reference Cousins and Kinlock1976, using the name Birbir deposit) were correct to describe it as an eluvial deposit, although other reports describe it as alluvial. The Yubdo ultramafic body consists of a central core of dunite surrounded by a narrow peridotite with a larger outer rim of pyroxenite in the form that offers comparison with Alaskan-type intrusions (Belete et al., Reference Belete, Mogessie and Bowles2002; Jackson et al., Reference Jackson, Prichard, Fisher, Bowles and Belete2005; Jackson, Reference Jackson2006), although the interpretation that Yubdo and other bodies lying along a common suture are ophiolitic remnants is also possible (Blades et al., Reference Blades, Foden, Collins, Alemu and Woldetinsae2019). The composition of chromite from fresh dunite lies mainly in the Alaskan-type field, although there is significant overlap into the ophiolite field, especially with samples of altered dunite (Fig. 6a). Primary PGM occur within the chromite and these are Ir–Os alloys (Fig. 6b,c) and Pt–Fe alloy (Fig. 6d). They are less than 5 μm in size. The area is drained by the River Birbir on the eastern edge and its tributary the Allfe to the north (Fig. 6e).

Fig. 6. Yubdo, Ethiopia chromite and PGM occurrence: (a) chromite compositions; (b) Ir–Os alloy with minor Rh and Ru in chromite; (c) Os–Ir alloy with minor Ru and Fe in chromite; (d) Pt–Fe alloy in chromite; and (e) the River Allfe that crosses the Yubdo deposit. (a) to (d) reproduced from Jackson et al. (Reference Jackson, Prichard, Fisher, Bowles and Belete2005).
The whole body is weathered extensively to a depth exceeding 50 m (Fig. 7a); there is a thin soil on top containing rounded quartz pebbles carried from elsewhere and there are layers of iron-rich red laterite with yellow, less iron-rich material below that is known locally as ‘bonde’. Nearby, PGE-bearing laterite is overlain by Miocene lavas indicating that the weathering has occurred over a long period. Local workers use sluice boxes (Fig. 7b), followed by hand panning (Fig. 7c), to extract the eluvial PGM (Fig. 7d), which consists largely of Pt–Fe alloy with grains typically ~1 mm across and occasionally measuring 1 cm. Naturally laterite is washed into the drainage and the Ethiopian Geological Survey report alluvial occurrences in the River Birbir several km downstream (Tefera and Berhe, Reference Tefera and Berhe1987). Together with the alluvial-style method of extraction, this explains why the deposit has been described as alluvial, but clearly it is eluvial (Fig. 7). The workers do not take the laterite closest to the sluice boxes, but trek across the face of the workings to carry laterite from a level near the base of the red laterite that they have found by trial and error to be the most productive. An important feature for exploration is the existence of a bi-modal PGE distribution, small samples for geochemical analyses record PGE concentrations without recoverable PGM grains whilst larger, sluice box sampling, recovers only the PGM nuggets. It appears that the PGE are present both as nuggets and very finely distributed, as grain coatings or within oxide or hydroxide phases. The PGE content of the laterite should be regarded as the sum of the results of bulk sampling for nuggets and geochemical analyses of smaller samples.

Fig. 7. PGM occurrence at Yubdo, Ethiopia: (a) the pit at Yubdo; (b) sluice boxes in action; (c) the senior panner; (d) the final concentrate. (Photos JFWB, (a) is composed of 4 images and (b) of 2 images that have been assembled by computer).
The laterite profile at Yubdo is similar to other tropical lateritic occurrences, but it has been well exposed by the mining activity. The pit of the Ni mine at Cerro Matoso, Colombia, is also the result of mining activity (Tobón et al., Reference Tobón, Weber, Proenza, Aiglsperger, Betancur, Farré-de-Pablo, Ramírez and Pujol-Solà2020), and it shows a comparable profile with a cover of allochthonous soil over in situ red laterite with saprolite beneath (Fig. 8a,b). The saprolite shows significant leaching, but retains some structure of the primary peridotite of the Ure–Cerro Matoso–Planeta Rica peridotite occurrences in NW Colombia. The profile at Planeta Rica (~50 km north of Cerro Matoso) shows the same sequence and the concentration of the PGE in the laterite is evident from Fig. 8c. PGM recovered from a sample of this section, containing 259 ppb total PGE, are Pt–Ir–Fe–Ni alloy surrounded by iron oxides and hydroxides (Fig. 8d), show the effects of oxidation and are considered to be secondary.

Fig. 8. PGM occurrences northern Colombia (after Tobón et al., Reference Tobón, Weber, Proenza, Aiglsperger, Betancur, Farré-de-Pablo, Ramírez and Pujol-Solà2020); (a) laterite overlying saprolite at the Cerro Matoso pit; (b) the laterite profile; (c) PGE in a drill hole at Planeta Rica; (d) PGM near the saprolite–laterite boundary from the same drill core.
Concentration of the PGE in the upper layers of laterites is demonstrated by the examples described above. It is a feature of laterites elsewhere such as those in Cuba and the Dominican Republic (Aiglsperger et al., Reference Aiglsperger, Proenza, Lewis, Labrador, Svojtka, Rojas-Puró, Longo and Ďurišová2016), where the PGE (and in this case also the rare earth elements) increase in concentration in the upper part of the profile. The critical question is to distinguish between a purely resistate effect, whereby the PGM increase in proportion because the other rock components have been removed, and a solution–transport–redeposition effect where the concentration is due to precipitation from solution at horizons favoured by parameters such as Eh, pH, $f_{{\rm O}_ 2}$, reactions with organic material and bacterial action. It is important to distinguish between the results of bulk sampling that favour the nuggets and geochemical analyses of small samples from drill core that represent PGE on grain surfaces. In general, fresh rock contains identifiable PGM and only small quantities of PGE on grain surfaces. In the saprolite only relict PGM remain and few are recovered by bulk sampling, whilst the PGE reside mainly on grain surfaces and are seen in geochemical analyses of small samples. In the laterite there is a bimodal distribution with both nuggets and PGE on grain surfaces. This distribution from rock through saprolite to laterite favours the solution–transport–redeposition model.
The alluvial regime
In a stream, the pH will be close to neutral and the Eh normal. Turbulent water can be oxygenated, although reducing conditions can be found in the bottom sediment. The concentrations of PGE in solution, organic material and bacteria are very much diluted compared to those in a soil. The solution–transport–redeposition process must be confined mainly to the weathering soil profile (Fig. 9b). In a stream, PGE remaining in solution will mainly be carried away and any PGM are likely to be subjected to mechanical abrasion.

Fig. 9. Possible conditions for supergene PGM formation: (a) Eh–pH diagram for Pt in aqueous solution containing a mixed ligand system with S, Cl and N (after Reith et al., Reference Reith, Campbell, Ball, Pring and Southam2014), the shaded area represents the range of Eh, pH conditions encountered in natural waters, (b) hypothetical flow regime modelled on the profile between the Alako and Big Water, Freetown Peninsula, Sierra Leone (reproduced from Bowles et al., Reference Bowles, Suárez, Prichard and Fisher2018a with permission of the Mineralogical Society of Great Britain and Ireland), with PGE-bearing horizons B, C and D containing cooperite, isoferroplatinum, nielsenite, bowieite, tulameenite and laurite.
The role of organic materials
For many geologists, our practical experience of platinum is its use as a crucible – that is to make a container of a noble metal that is not affected by high temperature or the contents. In that context, platinum is regarded as immutable. However, the reaction of the PGE with organic materials requires a very different perspective. The ease with which platinum reacts with organic material has been known for a long time. When Zeise (Reference Zeise1831) wished to make a synthetic organometallic compound he chose Pt as the metal, knowing that it would cooperate. PGE-organic compounds have become a huge area of interest. Cisplatin (Pt(NH3)2Cl2) and later variants will, sadly, have impinged on many lives – it is used in chemotherapy for cancer treatment (Rosenberg et al., Reference Rosenberg, VanCamp, Trosko and Mansour1969). It attacks the fastest growing cells (tumours, hair and finger nails) and the later variants have been designed to reduce the side effects. There is current interest in organic-PGE reactions due to the presence of PGE from vehicle catalytic converters entering urban and rural drainage systems. The PGE can complex with inorganic ligands to form aqueous complexes that are transported easily and may enter the food chain. The bioavailability of the PGE, given the known toxic and allergenic effects of these complexes, especially PGE-chloride complexes, raises significant concerns (Colombo et al., Reference Colombo, Oates, Monhemius and Plant2008a,Reference Colombo, Monhemius and Plantb and references therein).
The reactions between the PGE and organic compounds in soils require much more study. Wood (Reference Wood1990) showed that fulvic and phthalic acid maintain Pt in solution over a wide pH range at room temperature, probably as a colloidal suspension. Experiments with Pt and Pd foils and synthetic PtS powder, reacting with humic and fulvic acid at room temperature for 393 days showed that both metals are taken into solution (Bowles et al., Reference Bowles, Giże and Cowden1994a,Reference Bowles, Giże, Vaughan and Norrisb, Reference Bowles, Giże, Vaughan and Norris1995; Bowles and Giże, Reference Bowles and Giże2005). Humic acid took up to 195 ppb Pt and 102 ppb Pd into solution from the metal foils and up to 271 ppb Pt from the PtS powder, with some variation depending on the source of the humic acid. Fulvic acid was less reactive (20 ppb Pt and 6 ppb Pd from the foils; and 14 ppb Pt from the PtS powder). These experiments were intended as a first attempt to ‘see if anything would happen’ – a surprise was that 8 ppb Pt and 6 ppb Pd were also taken into solution by de-ionised water that had been intended as a control, assuming it to be unreactive. Parallel experiments with PGE-bearing rock powders and polished sections were inconclusive, which was not surprising as the exposed mineral surface area was of the order of 105 less than the metal foils.
Cabral et al. (Reference Cabral, Beaudoin, Choquette, Lehmann and Polônia2007) described botryoidal Pt–Pd growths from Córrego Bom Sucesso, Minas Gerais, Brazil (Fig. 4e), for which they offered a $f_{{\rm O}_ 2}$ vs. pH diagram in explanation of the genetic environment to show in particular the solubility of Pd as Pd(OH)2– over a wide pH range. In addition to sulfide, oxide and chloride species, Reith et al. (Reference Reith, Campbell, Ball, Pring and Southam2014) added species containing NH3 to the Eh–pH diagram using data from Colombo et al. (Reference Colombo, Oates, Monhemius and Plant2008a), as shown in Fig. 9a. Although these are inorganic compounds (e.g. Pt(NH3)Cl3–, Pt(NH3)2(OH)2(aq), Pt(NH3)42+) this represents a significant step towards understanding the behaviour of organic species containing a NH3 component.
Reith et al. (Reference Reith, Zammit, Shar, Etschmann, Bottrill, Southam, Ta, Kilburn, Oberthür, Bail and Brugger2016) showed that alluvial PGM from Brazil, Australia and Colombia are covered with biofilms containing abundant PGE nanoparticles and microcrystalline aggregates and dominated by Proteobacteria closely related to species known to be metal-resistant (Fig. 5a–d). Some PGM contained C, N, S, I and Se suggestive of a biogenic origin. These authors showed that nanophase Pt formed when a metallophillic bacterium was incubated with a Pt-containing medium, suggesting that biofilms can precipitate metallic Pt from solution (Fig. 5e,f). They concluded that “biofilms are capable of forming or transforming platinum-group mineral grains and may play an important role for platinum-group element dispersion and re-concentration in surface environments.” This mechanism requires further study because some biofilms have a substrate-based nature that limits mass transfer. Concentric banding on Pt–Pd grains suggests that the grains are the result of direct precipitation from solution rather than being the product of biofilms; the biofilms may instead act as a catalyst (Cabral et al., Reference Cabral, Reith, Lehmann, Brugger, Meinhold, Tupinambá and Kwitko-Ribeiro2012).
Study of the organic content of PGE-bearing soil from the Freetown Peninsula (Bowles et al., Reference Bowles, Giże and Cowden1994a,Reference Bowles, Giże, Vaughan and Norrisb, Reference Bowles, Giże, Vaughan and Norris1995; Bowles and Giże, Reference Bowles and Giże2005) showed the presence of anomalous concentrations of long chain n-alkanes (CnH2n+2, with 14 > n > 22) that are not normally seen in soils (Fig. 5g,h). Longer chains (23 > n > 33) are considered to be the result of breakdown of leaf litter on the forest floor and are normally stable. Bowles et al. (Reference Bowles, Bowles and Giże2018b) found that the presence of the PGE (especially Pt) lowers the temperature at which oxidation of the longer (23 > n > 33) chains can occur and that reaction between the PGE and the n-alkanes distorts the organic molecules. This lowers their heat of formation making it more likely that they can breakdown (Fig. 5i).
The hydrological regime is significant. Within thick, in situ lateritic profiles such as those shown in Fig. 8, it is assumed that ground waters react with the primary rock to take the PGE into solution, the water rises through the saprolite and then, in the laterite, reaches a level where the conditions (Eh, pH, $f_{{\rm O}_ 2}$ and bacterial action) favour deposition and mineral growth. The conditions on the Freetown Peninsula, Sierra Leone offer a similar model that differs due to the extremely high rainfall, with only a thin soil cover over the saprolite on the hilltops and thicker laterite on the lower slopes. The PGE enter into solution within the saprolite, aided or catalysed by bacterial action at high Eh and
$f_{{\rm O}_ 2}$ with low pH. The ground water flow is down towards the streams at the foot of the hill and the conditions favouring deposition (low Eh and normal pH) occur in the laterite adjacent to the streams (Fig. 9b). The topography at Yubdo, Ethiopia, is such that there is a hill with thick laterite over the source rock with slopes down to the Rivers Birbir and Allfe, and it appears likely that deposition occurs both in the laterite over the source rock (as in Fig. 7) and adjacent to the rivers.
What could be the trend of future studies?
There are some questions that still need to be answered:
Why is there a variation in the PGE content of weathered soil profiles (as in Fig. 8c) with a marked concentration at a particular horizon? Is this concentration due to the PGM acting as resistate phases whilst the host rock is removed by weathering, or has there been growth of the PGM at a particular horizon due to favourable conditions?
What conditions lead to the destruction of primary PGM during weathering? How are the PGE transported in solution, what complexes are involved? To what extent can the PGE reside on grain surfaces?
What would be the favourable conditions for PGM growth in a weathering profile? What controls are offered by pH, Eh, organic species and bacterial action? What is the relative importance of these controls and does this vary with the hydrological regime?
Can the mechanism of PGM growth as a biofilm develop to form larger crystals or is it limited to a surface film?
Is the presence of long chain n-alkanes (CnH2n+2, with 14 > n > 22) in PGE-bearing soils connected with the presence of the PGE, or is there some other explanation? Are these long chain n-alkanes present in other PGE-bearing soils?
Acknowledgements
Visits to Yubdo, Ethiopia were only possible with significant cooperation from Ato Banti Tassisa and his team at Yubdo, Dr Kebede Belete and his team in Addis Ababa, Ato Getachew Tesfaye, Head of the Minerals Operations Department, Ministry of Mines and Energy and his officials in Addis Ababa, funding from Golden Prospect Mining Company in the UK, and the assistance of the late Prof. H.M. Prichard and Dr M.T. Jackson of Cardiff University. SSB received generous funding from the Department of Education, Universities and Research of the Basque Government (Refs. BFI-2011-254, IT762-13). We thank Stuart Mills as Principal Editor and an anonymous reviewer for their help and encouragement. We greatly appreciate the very constructive review provided by Alexandre Raphael Cabral.