Serotonin (5-HT) is known to play an important role in a wide variety of functions, including mood, anxiety, aggression, sleep, appetite and sexual function, although the precise details of the mechanisms involved in these processes have not been established clearly. The situation has been hampered by the fact that until very recently it has not been possible to measure brain 5-HT directly, which means that we have had to rely on evidence from indirect sources. Over the past 10 years, neurotransmitter depletion paradigms have provided another means of examining the systems involved, and tryptophan (TRP) depletion in particular has emerged as an important tool for investigating 5-HT function. This review discusses the evolution of the technique of TRP depletion, the effects that it produces in healthy volunteers and patients suffering from different disorders, and what this tells us about the role of 5-HT in the pathogenesis and treatment of several psychiatric conditions.
THE TECHNIQUE OF TRYPTOPHAN DEPLETION
The aim of TRP depletion is to lower brain 5-HT by depleting the body of its amino acid precursor TRP.
Figure 1 illustrates the processes involved in the transport of TRP into the brain and its synthesis into 5-HT. A key factor in this is the level of TRP in plasma, which depends on the balance between the dietary intake of TRP and its removal from the plasma as a result of protein synthesis. Most of the TRP in plasma is protein-bound, with only about 5% being left free and available for transport into the central nervous system (CNS). It is this free TRP that is transported into the brain across the blood—brain barrier by an active protein shuttle for which five other large neutral amino acids (LNAAs: valine, leucine, isoleucine, phenylalanine and tyrosine) also compete. Once in the brain, TRP can take part in 5-HT synthesis, which is a two-step process. Tryptophan is first converted into 5-hydroxytryptophan (5-HTP) by the enzyme tryptophan hydroxylase; 5-HTP is then decarboxylated by the enzyme aromatic acid decarboxylase to 5-hydroxytryptamine (5-HT). Tryptophan hydroxylase is only about 50% saturated in the CNS, which means that the rate of 5-HT synthesis is dependent on the availability of its substrate, free plasma TRP. This is therefore the rate-limiting step.
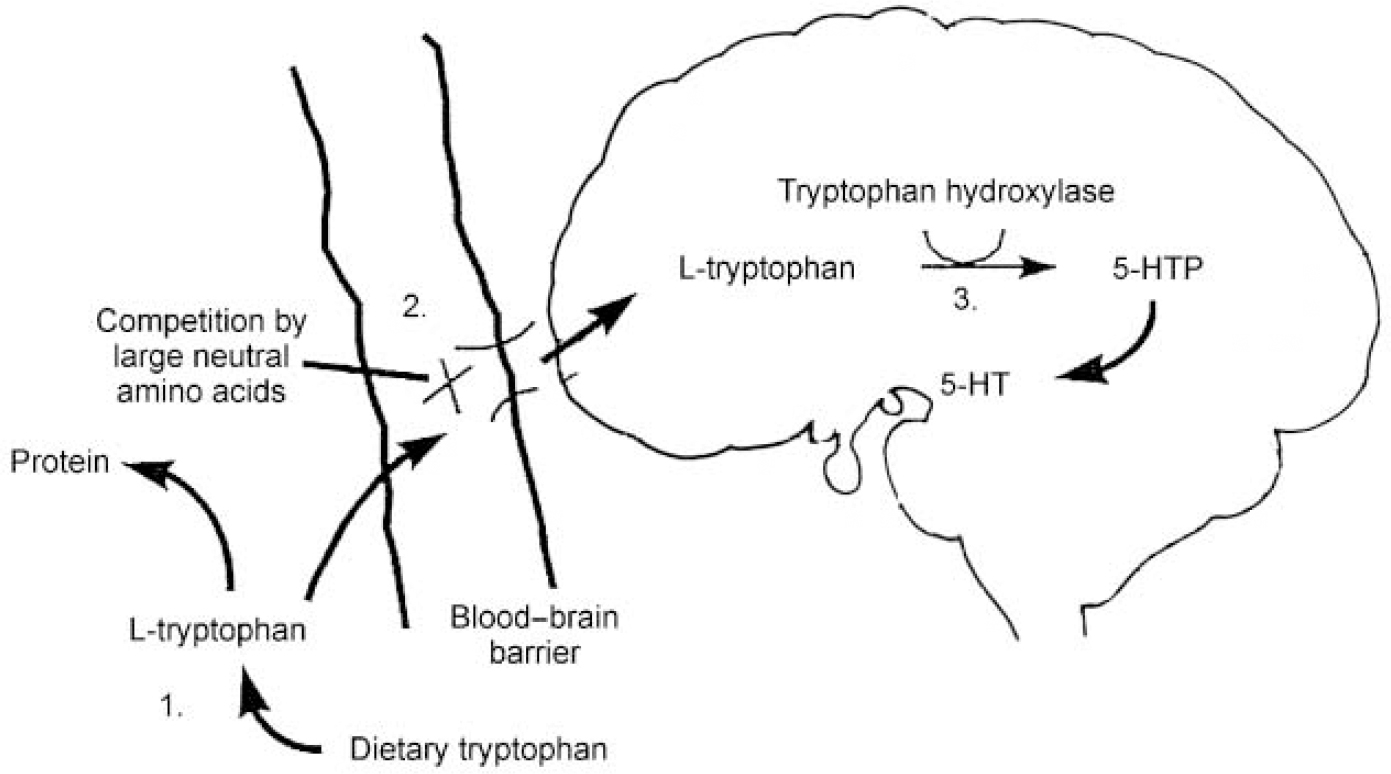
Fig. 1 Points at which serotonin (5-HT) synthesis may be controlled: (1) tryptophan availability: dietary restriction; (2) active transport: competition for the large neutral amino acid protein shuttle; (3) synthesis: inhibition of tryptophan hydroxylase. Best tryptophan depletion results are achieved using a combination of control at points 1 and 2. 5-HTP, 5-hydroxytryptophan.
From this description and Fig. 1 it can be seen that three factors are important in determining the rate of 5-HT synthesis: the total amount of free plasma TRP; how much of that free TRP crosses the blood—brain barrier; and the activity of the tryptophan hydroxylase enzyme. Synthesis of 5-HT can be influenced by interfering with any or all of these factors. Early studies in the 1970s inhibited tryptophan hydroxylase using the drug parachlorophenylalanine and reported that this produced a relapse of depressive symptoms in patients who had previously responded to treatment with imipramine and tranylcypromine. Parachlorophenylalanine is, however, too toxic for ethical use in human subjects today, which has meant that interest has focused on techniques affecting the first two factors. As discussed above, free plasma TRP levels vary with the amount of dietary TRP and the rate of protein synthesis. Completely removing TRP from the diet reduces plasma TRP by only 15-20% and has few behavioural consequences. A much bigger fall in plasma TRP can be achieved by giving subjects an amino acid load that does not contain TRP, in the form of a drink. This has two effects: it stimulates protein synthesis in the liver, which uses up plasma TRP; and the amino acids that are given include the other LNAAs, which compete with TRP for transport across the blood—brain barrier and thus restrict the entry of TRP into the brain. Techniques that influence both of these factors have been shown to produce maximal brain TRP depletion: that is, using a combination of a low-protein (low-TRP) diet and a TRP-deficient protein load containing large amounts of the other LNAAs (reviewed in Reference Reilly, McTavish and YoungReilly et al, 1997).
The TRP depletion paradigm is based on the hypothesis that reducing plasma TRP produces a consequent reduction in brain 5-HT synthesis and release. This has been confirmed in animals, with a large body of preclinical data showing that depletion of TRP specifically reduces brain 5-HT content and function. Ingestion of TRP-free amino acid mixtures in laboratory animals leads to extremely rapid changes in plasma TRP and brain 5-HT content, with maximal reductions of brain 5-HT occurring within 2 h. It also alters behavioural indices of 5-HT function (increasing pain sensitivity, acoustic startle, motor activity and aggression, and reducing rapid eye movement (REM) sleep), effects that are reversed once brain TRP is restored. In monkeys, TRP depletion has similar effects, decreasing plasma TRP and cerebrospinal fluid (CSF) TRP and 5-hydroxyindoleacetic acid (5-HIAA).
In humans, the effects of TRP depletion on brain 5-HT have been more difficult to establish. Neuroendocrine studies have shown that TRP depletion produces postsynaptic receptor supersensitivity, presumably secondary to reduced synaptic availability of 5-HT. Recent studies in healthy volunteers involving continuous CSF sampling have shown that TRP depletion reduces central TRP and 5-HIAA levels by 80-90% and 24-40%, respectively (Reference Carpenter, Anderson and PeltonCarpenter et al, 1998; Reference Williams, Shoaf and HommerWilliams et al, 1999). The time course of these responses was also slightly delayed compared with the plasma findings, with the nadir for CSF TRP being 7-10 h after TRP depletion and even longer (12-14 h) for 5-HIAA. One study of the rate of 5-HT synthesis in vivo using positron emission tomography (PET) has reported that TRP depletion reduces the rate of synthesis by up to 40% of baseline values (Reference Nishizawa, Benkelfat and YoungNishizawa et al, 1997).
Although the details vary, a common procedure for TRP depletion involves two test days about 1 week apart in a double-blind, crossover design. On both occasions subjects follow a low-TRP (160 mg/day) diet for 24 h prior to the test day, followed by an overnight fast extending throughout the test day. On one test day, subjects consume a drink containing a 100-g load of 15 amino acids (including the LNAAs described above) that does not contain TRP, and on the other they have a nutritionally balanced drink that does contain TRP. With this technique, free (and total) plasma TRP levels are reduced by about 80% 5-7 h after the depleting drink (Reference Delgado, Charney and PriceDelgado et al, 1990; Reference Williams, Shoaf and HommerWilliams et al, 1999). Behavioural measures are recorded throughout the test day, with peak effects usually being seen 5-7 h after TRP depletion (i.e. at the point of maximum depletion of plasma TRP).
CLINICAL EFFECTS OF TRYPTOPHAN DEPLETION
Healthy volunteers
The known link between 5-HT and affect led to the early studies of TRP depletion in healthy volunteers, focusing particularly on mood effects. They used male subjects exclusively and showed that TRP depletion produced significant mood lowering (although never amounting to clinical depression) in these groups (Reference Young, Smith and PihlYoung et al, 1985; Reference Smith, Clifford and HockneySmith et al, 1987). Subsequent studies, however, have tended to report that TRP depletion results in no mood effects (Reference Benkelfat, Ellenbogen and DeanBenkelfat et al, 1994; Reference Carpenter, Anderson and PeltonCarpenter et al, 1998; Reference Knott, Howson and PeruginiKnott et al, 1999). The discrepancy between these findings is probably explained by differences in the baseline mood states of subjects in the studies: those that reported a mood-lowering effect used volunteers with mean baseline depression scores at the upper end of the normal range, whereas the more recent negative findings were obtained with fully euthymic subjects. Studies that have included women have reported a gender difference, with a tendency for TRP depletion to produce mood lowering in healthy women but not men, despite similar degrees of plasma TRP depletion. This suggests that women may be more predisposed to the effects of TRP depletion than men. This is interesting given the increased incidence of depression in women and evidence from PET imaging studies suggesting that there may be gender differences in 5-HT metabolism with TRP depletion producing greater biochemical effects in women than in men (Reference Nishizawa, Benkelfat and YoungNishizawa et al, 1997).
An increased vulnerability to mood alteration during TRP depletion has been reported in male and female euthymic subjects who have a family history of affective illness (Reference Benkelfat, Ellenbogen and DeanBenkelfat et al, 1994; Reference Klaassen, Riedel and van SomerenKlaassen et al, 1999), although this finding has not been replicated by all studies (Reference Ellenbogen, Young and DeanEllenbogen et al, 1999). This suggests that a subgroup of patients might have a trait that makes them particularly vulnerable to alterations in 5-HT function. It also fits with clinical experience that a family history of affective disorder is a predisposing factor for depression.
Studies examining the effects of TRP depletion on anxiety symptoms in healthy volunteers have reported no significant effects on anxiety (Reference Klaassen, Klumperbeek and DeutzKlaassen et al, 1998). It does, however, produce an exacerbation in anxiety (although never amounting to panic) when TRP depletion is combined with pharmacological panicogenic challenges such as yohimbine (Reference Goddard, Charney and GermineGoddard et al, 1995) and CO2 (Reference Klaassen, Klumperbeek and DeutzKlaassen et al, 1998), which are known to induce panic symptoms in patients with untreated panic disorder.
Other studies that have focused on the memory and cognitive effects of TRP depletion in human subjects have shown that TRP depletion specifically impairs long-term memory formation and interferes with the process of memory consolidation (Reference Schmitt, Jorissen and SobczakSchmitt et al, 2000). Tryptophan depletion has also been reported to result in an improvement in measures of focused attention (Reference Schmitt, Jorissen and SobczakSchmitt et al, 2000), which may be a result of the inhibitory effect of 5-HT on other neurotransmitters, particularly those involved with attentional processes (noradrenaline and acetylcholine). Interestingly, TRP depletion does not produce changes in other measures of frontal functioning (Reference Schmitt, Jorissen and SobczakSchmitt et al, 2000).
The role of 5-HT in aggressive behaviour has been the subject of much research. Decreased central 5-HT function has been implicated in impulsive aggressive acts, including violent offending, arson and suicide. Animal studies have confirmed these findings and have shown that reducing 5-HT function increases measures of aggression. Studies in humans have shown that in healthy volunteers TRP depletion produces a marked rise in the ratings of aggression during provocation in subjects with high-trait aggression but has little effect in those with low-trait aggression (Reference Bjork, Dougherty and MoellerBjork et al, 2000).
The role of 5-HT in sleep is complex. It increases the number of wakings during the night and results in changes to sleep architecture, i.e. the suppression of REM and slow-wave sleep. A large body of work has examined the sleep findings seen in depression. The classical abnormalities reported include measures of sleep continuity (difficulty getting off to sleep, waking during the night and waking early in the morning) and architecture (reduced REM latency and an increased duration of the first REM period). It was hypothesised that TRP depletion in healthy volunteers would produce a reduction in 5-HT function that would be reflected by changes to the sleep electroencephalogram (EEG), possibly producing changes similar to those seen in patients with depression. One study reported findings consistent with this, that is, reduced REM latency after TRP depletion (Reference Bhatti, Gillin and SeifritzBhatti et al, 1998), although another study reported no change to REM latency or amount (Reference Voderholzer, Hornyak and ThielVoderholzer et al, 1998).
Depression (see Table 1)
Most of the studies of TRP depletion have been in the field of depression. This reflects the intense interest over the past 30 years in the involvement of 5-HT in the pathogenesis and treatment of affective disorders. At present, the evidence from untreated patients suggests that 5-HT function is reduced in depression. The evidence from treated patients suggests that antidepressants work in depression by increasing 5-HT and/or noradrenaline neurotransmission. Despite this knowledge, the site of the abnormality/abnormalities causing the 5-HT dysfunction in depression and the precise mechanisms of action of antidepressants have not been established. The hypotheses include factors such as the level of TRP availability, the rate of 5-HT synthesis, reuptake or metabolism, the functioning of pre- or post-synaptic 5-HT receptors and an altered interaction between 5-HT and other neurotransmitter systems. Tryptophan depletion provides a way of testing some of these theories.
Table 1 Effects of tryptophan depletion in depression

Mood state | Subjects (men, women) | Findings | Reference |
---|---|---|---|
Untreated depression | 43 (21, 22) | No mood effects | Delgado et al (Reference Delgado, Price and Miller1994) |
22 (6, 16) | No mood effects | Price et al (Reference Price, Matison and McDougle1997) | |
38 (17, 21) | No mood effects | Price et al (Reference Price, Matison and McDougle1998) | |
Remitted on antidepressants | 21 (8, 13) (on SSRIs, TCAs, MAOIs, lithium) | 14/21 relapsed | Delgado et al (Reference Delgado, Charney and Price1990) |
20 (11, 9) (on citalopram) | 5/12 relapsed with TRP depletion | Åberg-Wistedt et al (Reference Åberg-Wistedt, Hasselmark and Stain-Malmgren1998) | |
0/8 relapsed with no TRP depletion | |||
21 (11, 10) (on SSRIs) | 7/21 relapsed | Bremner et al (Reference Bremner, Innis and Salomon1997) | |
15 (9, 6) (on desipramine) | 8/15 relapsed | Delgado et al (Reference Delgado, Miller and Salomon1999) | |
15 (9, 6) (on fluvoxamine) | 1/15 relapsed | ||
Remitted on no medication | 15 (all women) | 10/15 relapsed | Smith et al (Reference Smith, Fairburn and Cowen1997) |
14 (5, 9) | No mood lowering | Leyton et al (Reference Leyton, Young and Blier1997) | |
12 (4, 8) | Mood lowering | Moreno et al (Reference Moreno, Gelenberg and Heninger1999) | |
12 controls (4, 8) | No mood lowering |
The effects of TRP depletion in untreated depression were somewhat unexpected. It was hypothesised that if decreased 5-HT function were associated with depression, then TRP depletion would lead to a transient worsening of symptoms. In a seminal study, 43 patients with untreated depression underwent TRP depletion (Reference Delgado, Price and MillerDelgado et al, 1994). This was found to result in no further mood lowering over the course of the day but, interestingly, a bimodal response the next day, with 37% describing an improvement in symptoms and 23% a worsening. The lack of effect of TRP depletion in causing a worsening of depressive symptoms in untreated patients has been replicated by two other studies (Price et al, Reference Price, Matison and McDougle1997, Reference Price, Matison and McDougle1998). One explanation for this is that 5-HT function is already so diminished that further lowering has little effect on symptom exacerbation. It may be, however, that deficient 5-HT function is not the primary or sole cause of depression and may be the result of dysfunction in regions or circuits in the brain that are modulated by 5-HT. This would mean that altering 5-HT function by itself would not be expected to produce immediate effects. The improvement in symptoms on the day after TRP depletion has been replicated by one study (Reference Price, Matison and McDouglePrice et al, 1998). The explanation for this is that TRP depletion results in an up-regulation of post-synaptic 5-HT receptors because of the decreased release of 5-HT at the synapse. When 5-HT levels are restored (by the next day), the net effect is an enhancement of 5-HT function, resulting in the improvement of symptoms.
The role of 5-HT in the mechanism of action of antidepressants in depression has been examined extensively using TRP depletion. The hypothesis for these studies was that if, as proposed, a time-dependent process of neuronal adaptation led to an enhancement of 5-HT neurotransmission after long-term antidepressant treatment, then acute depletion would be expected to rapidly reverse the beneficial effects. This has now been tested in multiple studies that have examined the effects of TRP depletion in patients who had responded to antidepressant treatments (particularly serotonergic antidepressants) (Table 1) (Reference Delgado, Charney and PriceDelgado et al, 1990; Reference Bremner, Innis and SalomonBremner et al, 1997; Reference Åberg-Wistedt, Hasselmark and Stain-MalmgrenÅberg-Wistedt et al, 1998; Reference Leyton, Young and PihlLeyton et al, 2000). These showed that rapid TRP depletion led to a return of depressive symptoms in patients who had responded to treatment with antidepressants. Interestingly, the content of the depressive symptomatology was often very similar to the original presentation of the illness and typically occurred about 4-6 h after the depleting drink (i.e. at the time of peak depletion of plasma TRP).
The rates of relapse from these studies (as measured by an increase in depressive symptoms on the Hamilton Rating Scale for Depression, HRSD; Reference HamiltonHamilton, 1960) were consistently higher for those on serotonergic drugs compared with those receiving more noradrenergic agents. One study that specifically examined this difference reported that 53% of patients who had responded to fluoxetine relapsed compared with 7% of patients who had responded to treatment with desipramine (Reference Delgado, Miller and SalomonDelgado et al, 1999). In contrast, catecholamine depletion studies (which use α -methyl-p-tyrosine to block the enzyme tyrosine hydoxylase and thus the synthesis of noradrenaline and dopamine) result in a similar transient depressive relapse in patients who had been treated successfully with noradrenergic drugs but not in those on serotonergic antidepressants (Reference Miller, Delgado and SalomonMiller et al, 1996). This suggests that increasing 5-HT or noradrenaline neurotransmission is important for the specific function of different antidepressants.
The rates of relapse for patients on selective serotonin reuptake inhibitors (SSRIs) ranged in the different studies from 80% (Reference Delgado, Price and MillerDelgado et al, 1991) in patients remitted for at least 2 weeks, to only 30% (Reference Bremner, Innis and SalomonBremner et al, 1997) in patients remitted for an average of 45 weeks. This suggests that the TRP depletion-induced relapse may be more likely in recently remitted patients compared with those who have been treated for longer periods of time. It may be that increasing synaptic 5-HT is only the first step in a cascade of events that lead to adaptive changes in 5-HT neurons, and that disrupting 5-HT function at this later stage has less effect.
The time course of the effects of depletion paradigms (i.e. over hours) implies that synaptic availability of 5-HT is more important than post-synaptic receptor effects, which would not be expected to change as rapidly. This is quite a major shift in thinking, because previous teaching has suggested that the delay in the clinical effect of antidepressants is explained by changes to postsynaptic receptors and other processes downstream of the synapse.
Two studies have used PET imaging to examine the effects of TRP depletion in remitted patients. Both reported that TRP depletion resulted in a decrease in brain metabolism in the areas of the brain implicated in the pathogenesis of depression (i.e. dorsolateral prefrontal and orbitofrontal cortex, amygdala and thalamus) (Reference Bremner, Innis and SalomonBremner et al, 1997; Reference Morris, Smith and CowenMorris et al, 1999). Interestingly, in one study changes were seen only in 7/21 patients and were limited to those who relapsed as a result of TRP depletion (Reference Bremner, Innis and SalomonBremner et al, 1997).
Patients who have had previous episodes of depression are known to be vulnerable to further episodes and it might be expected that TRP depletion in this group would result in relapse of depressive symptoms. Three studies have examined this specifically, using subjects with a past history of depression who at the time were well and on no medication (Reference Leyton, Young and BlierLeyton et al, 1997; Reference Smith, Fairburn and CowenSmith et al, 1997; Reference Moreno, Gelenberg and HeningerMoreno et al, 1999). Two of the three studies reported greater rates of depressive relapse after TRP depletion than after the control drink (Reference Smith, Fairburn and CowenSmith et al, 1997; Reference Moreno, Gelenberg and HeningerMoreno et al, 1999). These findings imply that deficient 5-HT function may play a part in triggering depressive episodes in patients with a vulnerability or susceptibility to changes in 5-HT function.
The sleep effects of TRP depletion in patients with remitted depression are interesting. One study has reported that although none of a group of ten men remitted for 2-13 months relapsed during TRP depletion, their sleep EEGs did regain some of the abnormalities characteristic of depression (i.e. reduced sleep and REM latencies and increased REM percentage and density) (Reference Moore, Gillin and BhattiMoore et al, 1998). This suggests that sleep may be a sensitive index of TRP levels.
Seasonal affective disorder (SAD) is a subtype of mood disorder characterised by recurrent major depressive episodes that occur regularly in the autumn and winter and respond to light therapy. The pathophysiology of SAD and the mechanism of action of light therapy are still poorly understood, but several lines of evidence suggest that 5-HT may be important (Reference Joseph-Vanderpool, Jacobsen and MurphyJoseph-Vanderpool et al, 1993). The results of TRP depletion in this group are similar to those reported with depression. Untreated patients are the least vulnerable to the mood-lowering effects of TRP depletion (Reference Neumeister, Praschak and HesselmannNeumeister et al, 1997b ). Patients with SAD who have been treated and are in remission after light therapy are likely to relapse after TRP depletion (Reference Lam, Zis and GrewalLam et al, 1996; Reference Neumeister, Praschak and HesselmannNeumeister et al, 1997a ). Long-term remitted patients produce mixed responses to TRP depletion, with one study reporting relapse rates of 73% (Reference Neumeister, Praschak and HesselmannNeumeister et al, 1998) and another reporting no effect on symptoms (Reference Lam, Bowering and TamLam et al, 2000).
Anxiety disorders
Serotonin neurotransmission is thought to play a central role in panic and anxiety in general. Perhaps the strongest evidence comes from treatment studies, which have shown that many of the disorders, including panic, social phobia and obsessive—compulsive disorder (OCD), are amenable to treatment with serotonergic drugs such as the SSRIs (discussed by Reference Bell and NuttBell & Nutt, 1998).
Most TRP depletion studies in anxiety have been performed in panic disorder and OCD, although there are ongoing studies in social phobia and generalised anxiety disorder.
Panic disorder
Patients with untreated panic disorder experience panic symptoms in response to panicogenic challenges (e.g. lactate, CO2, cholecystokinin-4, yohimbine or flumazenil), a reaction that is blocked by effective antidepressant treatment of the condition. Studies consistently show that in untreated patients TRP depletion alone produces no exacerbation of anxiety (Reference Miller, Deakin and AndersonMiller et al, 2000). However, when TRP depletion is combined with a panicogenic challenge it tends to result in an increase in anxiety and an increased rate of panic attacks (Reference Miller, Deakin and AndersonMiller et al, 2000), although one study reported a reduction in the expected number of panic attacks compared with normal controls (Reference Schruers, Klaassen and PolsSchruers et al, 2000). We have reported recently on the effects of TRP depletion and a flumazenil challenge in patients who have responded to treatment with SSRIs (Reference Nutt, Forshall and BellNutt et al, 1999). We showed that 5/8 patients panicked in response to flumazenil when they were TRP depleted, compared with 0/8 on the control day. This suggests that enhanced synaptic 5-HT neurotransmission is important in maintaining the response to SSRIs in this condition; and when this is reduced (by TRP depletion), panic results (a finding similar to that reported in depression).
Obsessive—compulsive disorder
Serotonergic tricyclic antidepressants and SSRIs have been used successfully in the treatment of OCD. It was hypothesised that, as with depression and panic disorder, TRP depletion might be expected to exacerbate OCD in this group of patients. Four studies have examined the effects of TRP depletion in OCD in remitted patients either taking medication (Reference Barr, Goodman and McDouglieBarr et al, 1994) or remitted and drug-free (Reference Smeraldi, Diaferia and ErzegovesiSmeraldi et al, 1997; Reference Huwig-Poppe, Voderholzer and BackhausHuwig-Poppe et al, 1999). These have all shown that TRP depletion produces no effect on OCD or Tourette symptoms, although some mood-lowering changes were reported. The difference in this response compared with the findings in depression and panic disorder suggests that the treatment of OCD may be less dependent on the synaptic availability of 5-HT than these other conditions, and more dependent on changes further downstream. Alternatively, it may be a reflection of the design of these studies, which did not combine TRP depletion with a challenge (as used in the panic disorder studies); and it may be that if this were done, TRP depletion would produce a relapse in symptoms.
Eating disorders
There is considerable evidence for a role for 5-HT in the control of feeding behaviour, with a reduction in 5-HT function being shown to lead to impaired satiety and weight gain in animals. Dieting is known to lower 5-HT function in healthy women (Reference Anderson, Parry-Billings and NewsholmeAnderson et al, 1990) and to increase their prolactin responses to TRP (implying post-synaptic supersensitivity as a result of reduced synaptic availability). It has also been reported that women with a personal history of depression become more depressed during dieting than those without (Reference Smith, Fairburn and CowenSmith et al, 1999).
Bulimia nervosa has been linked with lowered 5-HT function. Studies in acutely ill untreated subjects with bulimia nervosa found increases in subjective ratings of the fear of fatness and binge eating as a result of TRP depletion (Reference Kaye, Gendall and FernstromKaye et al, 2000). A similar study in a group of ten patients in remission from the condition reported that TRP depletion produced an increase in the symptoms of fear of fatness, fear of losing control over eating and an increase in the urge to eat (Reference Smith, Fairburn and CowenSmith et al, 1999). In both untreated and treated patients with bulimia nervosa and those in remission, there was a vulnerability to the mood-lowering effects of TRP depletion.
To date, no TRP depletion studies have been performed in anorexia nervosa.
Other disorders
Dementia
Studies that examined the effects of TRP depletion on memory and related cognitive processes have provided some evidence that reducing 5-HT function through this technique impairs memory consolidation. This may be of particular relevance for disorders in which a deficit of 5-HT function is known to be linked with cognitive effects (e.g. Alzheimer's disease, where diminished 5-HT levels were found, at post-mortem). In a study that examined the effects of TRP depletion in patients with Alzheimer's disease, a significant impairment in cognitive functioning was reported, again suggesting that compromised serotonergic function (in combination with cholinergic deficit) may make an important contribution to the cognitive decline seen in this group of patients (Reference Porter, Lunn and WalkerPorter et al, 2000).
Bipolar disorder
A role for serotonin in mania has been hypothesised (Reference Yatham, Shiah and LamYatham et al, 1999), although to date no TRP depletion studies have been performed in patients with acute mania. In patients recovered from bipolar disorder, TRP depletion has been reported to have either no effect (Reference Cassidy, Murry and CarrollCassidy et al, 1998) or to cause a slight but insignificant (due to small sample size) relapse of mania (Reference Cappiello, Sernyak and MalisonCappiello et al, 1997).
Schizophrenia
There is some evidence that 5-HT may be at least partially responsible for the negative symptoms of schizophrenia. The effects of TRP depletion in schizophrenia, however, appear to be quite complex. In one study, TRP depletion exacerbated depressive symptoms and had no effects on positive or negative psychotic symptoms (Reference D'Souza, Gil and Abi-DraghjamD'Souza et al, 1994), whereas in another study it worsened negative symptoms (Reference Sharma, Shapiro and KamathSharma et al, 1997). In a recent study of neuroleptictreated patients with schizophrenia, TRP depletion resulted in an improvement on two measures of cognition (Speed and Comprehension of Language Processing and Wisconsin Card Sorting Test; Reference Golightly, Lloyd and HobsonGolightly et al, 1999).
DISCUSSION
Tryptophan depletion provides a way of examining the role of 5-HT in the pathophysiology of several psychiatric disorders and the mechanism of action of medication (antidepressants in particular). The responses produced by TRP depletion in different patient groups give us some information about the role of 5-HT in these disorders. Many questions remain unanswered but TRP depletion does provide an interesting and usable tool with which to examine the involvement of 5-HT in these conditions.
Clinical Implications and Limitations
CLINICAL IMPLICATIONS
-
▪ Findings that tryptophan (TRP) depletion produces a relapse of symptoms in treated depression (and panic disorder) suggest that enhanced serotonin (5-HT) function is important in maintaining response to antidepressants.
-
▪ Findings that TRP depletion produces relapse or precipitates symptoms in patients with a personal or family history of affective disorders suggest that these subjects have a vulnerability to alterations in 5-HT function.
-
▪ Lack of effect of TRP depletion in untreated depression and panic disorder and in healthy volunteers implies that 5-HT dysfunction is not the primary or sole cause of these disorders.
LIMITATIONS
-
▪ We are not yet able to use TRP depletion as a tool to predict the likelihood of relapse when patients are taken off antidepressants.
-
▪ In humans it is difficult to establish the degree to which TRP depletion produces a reduction in brain 5-HT and the time course of this response.
-
▪ There are only a small number of studies in conditions other than depression, which limits the conclusions that can be made about these conditions.
ACKNOWLEDGEMENT
We thank Dr Spilios Argyropoulos for his help with revisions to this paper.
eLetters
No eLetters have been published for this article.