Implications
Fish welfare is important in two main contexts: laboratory research and aquaculture farms. Despite some common elements, these two contexts differ at the level of the dimensional scale, objectives, husbandry conditions, species and number of captive fish maintained. This review integrates the state of the art, critical considerations and perspectives concerning the main fish welfare issues with a particular focus on European directives, in the form of a single synthesis, which should be useful for both laboratory researchers and aquaculture staff.
Introduction
In recent years, teleost fish have been increasingly exploited as animal models for scientific research in both the biomedical and ecological fields, as they offer several practical advantages compared with mammals or other vertebrates (Schartl, Reference Schartl2014). Although molecular-based estimates of the actinopterygian evolutionary time-scale place the divergence of ray-finned fishes from the tetrapod lineage at ~400 million years ago (Hurley et al., Reference Hurley, Mueller, Dunn, Schmidt, Friedman, Ho, Prince, Yang, Thomas and Coates2007), there are enough commonalities between fish and mammals to justify research relevant to humans to be performed on fish (Schartl, Reference Schartl2014). In fact, despite their different physical appearance, teleosts, such as zebrafish, share multiple structural and physiological homologies with higher vertebrates such as humans, including cellular structure, organ anatomy, cognitive behaviours and genome homologies. A direct comparison between zebrafish and human protein-coding genes revealed that 71.4% of human genes have at least one zebrafish orthologue, and 69% of zebrafish genes have at least one human orthologue (Howe et al., Reference Howe, Clark, Torroja, Torrance, Berthelot, Muffato, Collins, Humphray, McLaren, Matthews, McLaren, Sealy, Caccamo, Churcher, Scott, Barrett, Koch, Rauch, White, Chow, Kilian, Quintais, Guerra-Assunção, Zhou, Gu, Yen, Vogel, Eyre, Redmond, Banerjee, Chi, Fu, Langley, Maguire, Laird, Lloyd, Kenyon, Donaldson, Sehra, Almeida-King, Loveland, Trevanion, Jones, Quail, Willey, Hunt, Burton, Sims, McLay, Plumb, Davis, Clee, Oliver, Clark, Riddle, Elliot, Threadgold, Harden, Ware, Begum, Mortimore, Kerry, Heath, Phillimore, Tracey, Corby, Dunn, Johnson, Wood, Clark, Pelan, Griffiths, Smith, Glithero, Howden, Barker, Lloyd, Stevens, Harley, Holt, Panagiotidis, Lovell, Beasley, Henderson, Gordon, Auger, Wright, Collins, Raisen, Dyer, Leung, Robertson, Ambridge, Leongamornlert, McGuire, Gilderthorp, Griffiths, Manthravadi, Nichol, Barker, Whitehead, Kay, Brown, Murnane, Gray, Humphries, Sycamore, Barker, Saunders, Wallis, Babbage, Hammond, Mashreghi-Mohammadi, Barr, Martin, Wray, Ellington, Matthews, Ellwood, Woodmansey, Clark, Cooper, Tromans, Grafham, Skuce, Pandian, Andrews, Harrison, Kimberley, Garnett, Fosker, Hall, Garner, Kelly, Bird, Palmer, Gehring, Berger, Dooley, Ersan-Ürün, Eser, Geiger, Geisler, Karotki, Kirn, Konantz, Konantz, Oberländer, Rudolph-Geiger, Teucke, Lanz, Raddatz, Osoegawa, Zhu, Rapp, Widaa, Langford, Yang, Schuster, Carter, Harrow, Ning, Herrero, Searle, Enright, Geisler, Plasterk, Lee, Westerfield, de Jong, Zon, Postlethwait, Nüsslein-Volhard, Hubbard, Roest Crollius, Rogers and Stemple2013). Interestingly, both coding and regulatory gene sequences are conserved between teleosts and mammals. In particular, sequences clustered around the developmental regulatory genes are conserved in so-called genomic regulatory blocks (Kikuta et al., Reference Kikuta, Fredman, Rinkwitz, Lenhard and Becker2007).
Teleost models have been utilized in many research fields by using various ‘omics’ approaches (Mushtaq et al., Reference Mushtaq, Verpoorte and Kim2013). Official European parliament reports have indicated that the number of fish used for research purposes in the European Union has increased in recent years. As a reference, in 2011 a total of 1 397 462 specimens were used, representing an increase of 28.54% compared with 2008 (European Parliament Report, 2013). Teleost fish as vertebrate models can also be effectively used in ecological studies aimed at assessing the potential effect of global warming and environmental pollution. In fact, a number of recent studies conducted to assess the possible effect of global warming on fish fauna have shown that an increase of water temperature impacts the health, behaviour and fitness of fish and fisheries (Malavasi et al., Reference Malavasi, Cipolato, Cioni, Torricelli, Alleva, Manciocco and Toni2013; Manciocco et al., Reference Manciocco, Toni, Tedesco, Malavasi, Alleva and Cioni2015) by increasing the absorption of toxic substances already present in the environment and by affecting immune activity, reproductive performance and egg survival (Manciocco et al., Reference Manciocco, Calamandrei and Alleva2014).
Aquaculture is another context where fish welfare has received increasing attention in recent decades. Aquaculture is not only a massive industrial activity that integrates human diet with high-quality food, but it also helps in restocking fish populations for both commercial and conservation purposes (Brown and Day, Reference Brown and Day2002). Considering this twofold goal, assessing fish welfare becomes of tantamount importance in aquaculture (Conte, Reference Conte2004), as farmed fish must be kept not only free of pain for ethical and practical concerns but also in conditions similar to their wild counterparts to guarantee the success of restocking plans (Huntingford et al., Reference Huntingford, Adams, Braithwaite, Kadri, Pottinger, Sandøe and Turnbull2006).
In Europe, the use of fish for human purpose is regulated by specific Directives and Recommendations based on the assumption that vertebrate animals should always be treated as sentient creatures and that their use in scientific procedures must be restricted to research areas that may ultimately benefit human or animal health or the environment (Nuffield Council on Bioethics, 2005).
The Directive 2010/63/EU (2010) on the protection of animals used for scientific purposes covers ‘vertebrate animals including cyclostomes, cephalopods […] as there is scientific evidence of their ability to experience pain, suffering, distress and lasting harm’. In addition, several official guidelines and other resources on the care and use of fish in biomedical research are available to ensure animal welfare and to minimize pain and suffering (for more details see the EU website at https://ec.europa.eu/food/animals/welfare_en). These Recommendations establish that all animals must be provided with accommodation, environments, food, water and care appropriate to their health and well-being. Moreover, the environmental conditions in which animals are bred must be checked daily and arrangements must be made to ensure that any defect or avoidable pain, suffering, distress or lasting harm discovered be eliminated as quickly as possible. Finally, animals must be transported in appropriate conditions.
Fish welfare in aquaculture can be evaluated using the freedoms proposed by the UK Farm Animal Welfare Council (1992), which states the five well-known animal freedoms: (1) freedom from hunger or thirst; (2) freedom from discomfort; (3) freedom from pain, injury or disease; (4) freedom to express normal behaviour; and (5) freedom from fear or distress. Moreover, in 2006 the Standing Committee of the European Convention on the Protection of Animals kept for Farming Purposes has provided a detailed Recommendation Concerning Farmed Fish (Council of Europe, 2006).
Assessing teleost fish welfare
Ensuring the welfare of fish kept in research laboratories and aquaculture farms is a complex issue due to the high number of species-specific factors and aspects that must be known, considered, set or monitored, including the physical–chemical parameters of water, welfare indicators, environmental complexity, stocking density and foraging and social behaviours of the animals.
According to Ashley (Reference Ashley2007), a definition of welfare is not only based on physical health but also on the lack of mental suffering. Therefore, the concept of welfare is wider than the simple concept of ‘wellness’, which refers more to physical health and the avoidance of prolonged stress. Stress can be defined as a condition in which homeostasis, that is the dynamic balance of the animal organism, is disturbed by intrinsic or extrinsic stimuli, commonly called stressors (Chrousos and Gold, Reference Chrousos and Gold1992). The stress response is an adaptive function and it does not necessarily signify suffering or poor welfare (Ashley, Reference Ashley2007).
To ensure the welfare of fish, it is first and foremost necessary to have adequate knowledge of the biology of the housed species and the appropriate equipment necessary to provide a suitable environment. Teleosts comprise more than 25 000 species, each with specific anatomical, physiological and behavioural characteristics. Therefore, a modus operandi that considers both the biodiversity of fish species and the peculiarity of each environmental context must be embraced.
Welfare indicators
To ensure the welfare of housed fish, it is necessary to have good indicators that should be readily and reliably recognizable and minimally invasive. Welfare indicators should include different aspects related to the behavioural and physiological performance of the captive individuals. Stress indicators can be considered a measure of poor welfare when stress is a prolonged and long-term response, causing maladaptive consequences (Ashley, Reference Ashley2007). Thus, health and stress avoidance contribute to welfare assessment, but positive welfare conditions are in turn a prerequisite for physical health and stress avoidance.
Freedom from hunger, pain, discomfort and fear can be assessed through metabolic, performance, anatomical, behavioural and physiological indicators. The assessment of animal welfare levels based on blood and tissue parameters necessarily requires capture and invasive sampling procedures. The development of physical and behavioural indicators allows the state of fish well-being to be assessed simply by observing the fish through non-invasive monitoring. The simplicity with which the fish can be observed varies between the laboratory and aquaculture contexts and depends on factors such as fish size, the type of tank or cage, water turbidity and depth, animal density and lighting. Currently, the use of remote-controlled underwater cameras, which can also be equipped with a zoom lens, allow for fish welfare to be monitored unobtrusively both in aquaria and aquaculture systems, and this monitoring further promotes the development and use of physical and behavioural parameters. The observation of animals without approaching the tank is particularly useful as observer proximity often leads to the acquisition of distorted and discordant information for the widespread shy disposition of fish (Toms et al., Reference Toms, Echevarria and Jouandot2010).
Physical indicators for fish welfare include eye and skin colour and changes (with darkening usually associated with disease), morphological alterations (such as bitten fins), reluctance to move, altered body posture, mucus production and opercular beat frequency. Behaviours that are generally recommended in the current literature to assess fish welfare in research and aquaculture consider the following main categories: (1) space use, habitat selection and structural complexity of the rearing environment; (2) foraging behaviour and altered food consumption; (3) aggression to conspecifics, especially in relation to stocking densities; and, (4) spatio-temporal patterns of behaviour, changes in the use of the water column, swimming activity (altered speed and direction), shoaling to escape predators and disruption of circadian rhythm (i.e. increased activity when animals should be inactive). Behavioural indicators have also been proposed as useful tools to assess the ‘wildness’ of farmed fish in the context of aquaculture programmes aimed at restocking (Malavasi et al., Reference Malavasi, Georgalas and Torricelli2009).
Chemical–physical characteristics of water
Fish, similar to all other organisms, are heavily influenced and dependent on the characteristics of their environment. The chemical–physical characteristics of water affect fish life significantly, mainly due to the intimate relationship between their bodily fluids and the water in the surrounding environment. Water parameters that may affect fish welfare include temperature, the concentration of dissolved gases (O2 and CO2), pH, salinity, the presence of the compounds ammonia and urea derived from natural metabolism and chemical pollutants of anthropogenic origin such as heavy metals and pesticides. Other factors, such as lighting, noise and vibrations are also crucial for fish welfare (for further details refer to Toni et al., Reference Toni, Angiulli, Malavasi, Alleva and Cioni2017). In a natural environment, fish can move searching for areas where the chemical–physical properties of water are more suitable. As this is not possible in animal husbandry and laboratory facilities, it is essential to keep the chemical–physical parameters of the water at levels consistent with optimal fish welfare. Constant and correct water parameters are also important for obtaining repeatable experimental data, as it is generally recognized that rearing and housing environment can affect their validity (Reinhardt, Reference Reinhardt2004).
Environmental complexity
Environmental complexity is a parameter that should be considered for the proper design of fish tanks and aquaculture systems. Natural underwater environments are extremely varied and offer places where animals can hide and feel safe, reducing stress and anxiety. Several lines of evidence support the idea that environmental enrichment can greatly enhance the welfare of animals kept in captivity (Simpson and Kelly, Reference Simpson and Kelly2011). With regards to fish, the available data suggest that the structural complexity of the rearing environment can alter fish behaviour and exert positive effects on their state of welfare and health by reducing the impact of stress (Pounder et al., Reference Pounder, Mitchell, Thomson, Pottinger, Buckley and Sneddon2016) with an effect comparable with that obtained with antidepressant and anxiolytic drug treatments (Giacomini et al., Reference Giacomini, Abreu, Zanandrea, Saibt, Friedrich, Koakoski, Gusso, Piato and Barcellos2016). Moreover, environmental enrichment can increase brain development, enhance cognitive abilities (Salvanes et al., Reference Salvanes, Moberg, Ebbesson, Nilsen, Jensen and Braithwaite2013) and improve the foraging skills (Rodewald et al., Reference Rodewald, Hyvärinen and Hirvonen2011) of farmed fish, simulating to some extent the spatial component of the ecological niche in the wild. Providing shelters in rearing tanks can, for example, enhance the use of the sheltering area in the tank. This should promote the aggregation of groups of familiar fish, spacing them through the physical barriers present in the shelter, thereby reducing competition, aggression and stress levels, as has been shown in wels catfish (Silurus glanis) (Slavík et al., Reference Slavík, Maciak and Horký2012). The introduction of shelters must be carefully evaluated and balanced with fish density and territoriality of the species, as little cover could cause competition and aggressive events leading to a reduction of the welfare level, but on the other hand, a higher number of shelters can increase competition in high-density situations and may also increase stress levels (Boerrigter et al., Reference Boerrigter, van den Bos, van de Vis, Spanings and Flik2015). Substrate characteristics and especially bottom colouration can improve fish welfare: blue and green–brown substrates lead to decreased aggression in Sparus aurata juveniles, and it has been suggested that the colour blue per se determines these effects (Batzina and Karakatsouli, Reference Batzina and Karakatsouli2014). Environmental enrichment should be evaluated for each species, considering the behavioural repertoire, the species-specific level of territoriality and aggression and the specific life stage reared to reverse the behavioural deficits caused by the lower level of structural complexity in artificial habitats (Huntingford et al., Reference Huntingford, Adams, Braithwaite, Kadri, Pottinger, Sandøe and Turnbull2006). To properly evaluate the type and level of enrichment, experiments based on comparisons between control groups and environmentally enriched groups should be conducted along with before and after enrichment comparisons.
Considering the effect enrichment has on the welfare of fish, environmental complexity must be carefully evaluated to guarantee comparable results in a research context. Although it is easy to reproduce a barrel aquarium perfectly, it may be more difficult to reproduce an enriched environment in which the number, size, shape, colour and position of elements could influence the experimental result. For this reason, researchers must carefully select the proper environmental enrichment in experimental planning. As noted by Näslund and Johnsson (Reference Näslund and Johnsson2016), some by-products of environmental enrichment could lead to adverse consequences for both aquaculture and research. Trade-offs between the potential welfare advantages and these problematic by-products should be carefully evaluated when assessing the type and level of environmental enrichment that should be adopted for welfare purposes in both contexts. As mentioned before, experiments designed to compare control groups with environmentally enriched groups should help to determine the effects of enrichment to apply enrichment protocols only when it is possible and when the effects of enrichment are shown to enhance the behavioural performance of captive fish.
Foraging behaviour, stocking density and social behaviour
Foraging behaviour, stocking density and social behaviour interact, which in turn determine levels of aggressive interactions, as the social systems of fish are often characterized by a combination of dominance hierarchy and territoriality. As aggressive behaviour demands high energy, the overly intensive aggressive behaviour may impair metabolic and immune functions, leading to unacceptable levels of stress (Ashley, Reference Ashley2007). Further, aggression is a key factor in determining territoriality and spatial behaviour, contributing to the inter-individual distance and the occurrence of physical contact and potential associated injuries (Huntingford et al., Reference Huntingford, Adams, Braithwaite, Kadri, Pottinger, Sandøe and Turnbull2006). For these reasons, these aspects must be considered in fish housing.
Foraging behaviour is one of the widest and most complex areas of investigation in this field; according to López-Olmeda et al. (Reference López-Olmeda, Noble and Sánchez-Vázquez2012) it is very difficult to develop a universal feeding strategy for all farmed fish species. Many species-specific factors are involved in the feeding strategies and tactics of fish, such as feeding rhythms, food ratio and feeding time. In general, a self-feeding system seems to improve fish welfare, allowing fish to choose their optimal feeding time and food ratio (López-Olmeda et al., Reference López-Olmeda, Noble and Sánchez-Vázquez2012).
Stocking density is another factor that requires careful consideration in both aquaculture and laboratory facilities. Spatial patterns of behaviour have often been tested in relation to stocking densities, showing that swimming activity may be a reliable indicator of stress, crowding averseness, lower growth rate and higher metabolic demand (Bégout Anras and Lagardère, Reference Bégout Anras and Lagardère2004; Kristiansen et al., Reference Kristiansen, Fernö, Holm, Privitera, Bakke and Fosseidengen2004; Uglem et al., Reference Uglem, Kjørsvik, Gruven and Lamberg2009; Laursen et al., Reference Laursen, Andersson, Silva, Petersson and Höglund2013). Understanding the spatial patterns of behaviour in rearing tanks is a research area that requires the development of sophisticated technological and statistical tools due to the complicated and chaotic patterns of fish aggregation.
Data on social systems and the foraging behaviour of the reared species should be integrated to properly calibrate the number and quality of individuals in relation to the available space and to modulate aggressive activity in captive conditions. The social systems of fish species are often based on social hierarchies, and the density of fish in relation to food distribution and quantity greatly affects the level of social interactions, which can in turn also affect swimming patterns. It is therefore clear that foraging behaviour and stocking density should be related to the social behaviour of each species to properly evaluate welfare (Ashley, Reference Ashley2007).
Trained staff and procedural protocols
Another critical point in preserving the quality of life of fish and guaranteeing the consistency and effectiveness of their welfare evaluation is to engage qualified staff who properly adopt welfare assessment protocols. This would ensure an appropriate environment and the use of husbandry procedures consistent with the physiological and psychological needs of the animals. Moreover, regular inspection of the adopted procedures should be conducted by competent institutions in the areas of concern.
Personnel should know the characteristics and the signs of suffering, stress or positive welfare of the species housed to be able to recognize the health status of animals, understand the significance of behavioural changes and appreciate the suitability of environments for animal welfare. To this end, a substantial period of training for those engaged in fish keeping is highly recommended in both laboratory and farming settings.
In both aquaculture and research, methodological concerns are of central importance, considering the need to develop standardized protocols that can be widely used by qualified operators around the world. It is commonly assumed that a welfare assessment protocol should include a minimum number of parameters necessary to rapidly and effectively detect adverse effects on animal life. The drafting of welfare assessment protocols requires the knowledge of biological and behavioural parameters of individual species to define good welfare standards. Generally, standard baseline requirements are drawn once fish are familiar with their accommodation and husbandry routines and behave according to a species-specific time budget. As many factors may impact fish living conditions and welfare assessment, it is essential to be aware of their effects and to conduct observations 1 or 2 h after stressful events such as transport, handling and husbandry procedures such as tank cleaning. Any dead or dying fish must be promptly removed from tanks to reduce the water pollution and the potential spread of diseases, to correctly euthanize dying animals, and finally to prevent adverse effects on the welfare of those remaining. The standardization of husbandry procedures can help reduce animal stress considering that fish have good learning and memory abilities (Brown, Reference Brown2015) and that they quickly become accustomed to cleaning operations no longer showing fear behaviour towards the plastic objects used to remove faeces from the tank bottom.
When fish are used as new animal models for research in pathology or mutagenesis, some effects can be completely unpredictable. In such cases, it is a good rule to carry out a pilot study during which many welfare indicators will be workable. Pilot studies not only provide information to define appropriate indicators but also help to refine experimentation, improving intervention points and humane endpoints. In addition, the timing of observations will depend on the nature of procedures, and more frequent monitoring is required when severe damage is expected.
Husbandry and care of fish
Several environmental parameters relevant to welfare assessment have been recognized by previous reviews (for recent work, see Toni et al., Reference Toni, Angiulli, Malavasi, Alleva and Cioni2017). All of these parameters can affect welfare in both research and aquaculture to a certain degree. These parameters can be subdivided into two main categories: abiotic factors, such as water quality, lighting and noise, and biotic factors, such as stocking density, environmental complexity, feeding and handling (Supplementary Table S1). The ease with which these parameters can be monitored, controlled, standardized and manipulated to achieve the proper levels of welfare differs markedly between laboratory research and aquaculture, mainly due to differences in dimensional scale.
Although there are different types of aquaculture systems (water- and land-based systems, recycling systems and integrated farming systems), we can generalize that aquaculture farms are designed to provide large volumes of water and mesocosm systems, whereas laboratory research is mostly based on microcosm experiments (experimental tanks).
As a rule, the use of large volumes of water helps to maintain greater stability and constancy of the physico-chemical parameters of the water. However, as the biological activity of the organisms influences these parameters in relation to their consumption of oxygen, the production of faeces and the excretion of metabolites, the ratio between the animal biomass and the volume of water is a key factor that must be considered. Moreover, the use of large volumes of water makes it more expensive to vary the physico-chemical parameters of the water when those of the water source are not optimal for the farmed species.
Thus, risk assessments of the variation in physico-chemical parameters of water and the mitigation procedures of such variations can be different between aquaculture industries that provide large amounts of water and laboratory facilities that often work with small water volumes in re-circulating closed systems (Supplementary Table S1). A common need for both contexts is the increase of knowledge on the specific requirements for individual species to assess optimal and threshold levels for each parameter.
European Directive 2010/63/EU (2010) includes a paragraph dedicated to fish and focuses on aspects to be considered for fish welfare. Similarly, the European Recommendation concerning farmed fish, adopted by the Standing Committee of the European Convention for the protection of animals kept for farming purposes, provides practical indications to aquaculturists on how to manage these animals correctly to guarantee them an appropriate level of well-being.
As far as teleost fish are concerned, it must be emphasized that the number of extant species is far greater than that of all other vertebrates and that teleosts are adjusted to different environments for temperature, salinity and lighting. This makes it difficult to provide generalized parameters that are valuable for all fish species. Therefore, the European norms must be applied with an accompanying thorough knowledge of physiology and ecological adjustments necessary for each species bred.
Environmental parameters in European research papers
The reproducibility of scientific results is only possible by precisely replicating the experimental conditions used, and this is especially true for experiments conducted on animals as evidence shows that environmental conditions can affect many physiological parameters including metabolism and neurochemistry. Housing conditions thus constitute important experimental parameters that authors of scientific publications should provide in detail. Directive 2010/63/EU (2010), in defining the parameters and environmental conditions to be monitored, not only provides guidance to ensure animal welfare but also suggests which housing parameters must be clearly indicated to allow experimental reproducibility.
A survey commissioned several years ago by the National Centre for the Replacement, Refinement and Reduction of Animals in Research (NC3Rs) showed that only 59% of the articles assessed stated the number and characteristics of the animals used. Following this study, the Animal Research: Reporting of In Vivo Experiments (ARRIVE) guidelines were developed in 2010 to improve the standard of reporting of research using animals (Kilkenny et al., Reference Kilkenny, Browne, Cuthill, Emerson and Altman2010). By the end of January 2016, more than 600 journals had endorsed the ARRIVE guidelines. However, little improvement in the quality of reporting has been shown by a recent comparison of papers published before and after the issuance of the ARRIVE guidelines, suggesting that authors, referees and editors are generally ignoring these recommendations (Baker et al., Reference Baker, Lidster, Sottomayor and Amor2014; Cressey, Reference Cressey2016).
We conducted a literature review in April 2016 to more thoroughly evaluate precisely which parameters described by Directive 2010/63/EU (2010) are being reported in scientific papers. We used the search engine Scopus (https://www.scopus.com) and entered the following expression search string ‘TITLE-ABS-KEY (fish species) AND ALL (tank) OR (aquaria) AND DOCTYPE (ar) AND DOCTYPE (ar) AND PUBYEAR=2014 OR 2015’ to select papers published in 2014 and 2015 where the keywords ‘tank’ or ‘aquaria’ were used. These keywords were chosen to select studies carried out in laboratory conditions and not in natural environments. Among the publications found, only works authored by European researchers were selected. The selection of research papers published in 2014 and 2015 would ensure that experimental activities were carried out in accordance with Directive 2010/63/EU (2010). A total of 82 articles were selected, and information reported in both the ‘Material and methods’ and the ‘Supplementary material’ sections was analysed for parameters related to housing conditions. In all, 24 of these studies were on Danio rerio, 16 on S. aurata, 15 on Dicentrarchus labrax, two on both S. aurata and D. labrax, 20 on Salmo salar and five on Cyprinus carpio. The results, summarized in Table 1, show that the range of parameters considered by the European Directive (2010) are not always reported. None of the papers provide information about environmental noise, despite the possibility of this parameter negatively affecting fish welfare and behaviour. Indications concerning fish handling are also rare. In particular, information about transfer procedures from the home tank to the experimental tank is usually scarce, despite that this can cause abrasions to the fish body and that prolonged permanence of the animal outside the water environment has repercussions on both fish health and the quality of experimental results. Few studies reported parameters of environmental complexity, although some evidence suggests that an enriched environment has a positive impact on fish health, even modifying the expression of neurotrophic factors. Moreover, the physical and chemical parameters of the water, such as the amount of nitrogen compounds, pH, and the level of oxygen saturation, are often lacking. Information on lighting conditions was provided in a number of papers, even if they mostly refer to the circadian rhythm rather than to the source of lighting, the light intensity and the wavelength used. In contrast, the density of fish in tanks, the type of food provided, water temperature and the general characteristics of the tanks were generally provided in almost all of the papers.
Table 1 Number (n) and percentage (%) of publications authored by European researchers published in 2014 and 2015 that provide information on the specific environmental parameter listed by Directive 2010/63/EU (2010) focused on Danio rerio, Sparus aurata, Dicentrarchus labrax, Salmo salar and Cyprinus carpio

Housing conditions adopted in the different works, even when they refer to the same species, vary greatly, as shown in Table 2. This reduces the possibility of comparing and integrating data from different laboratories, as variations in environmental conditions can strongly affect the animal status and, therefore, the experimental results. For example, the wide variation in the chemical and physical properties of the water, such as temperature and oxygen saturation, is striking, as these conditions strongly influence fish metabolism at both cellular and systemic levels.
Table 2 Range of environmental parameters values declared in publications authored by European researchers published in 2014 and 2015 that provide information on the specific environmental parameter listed by Directive 2010/63/EU (2010) focused on Danio rerio, Sparus aurata, Dicentrarchus labrax, Salmo salar and Cyprinus carpio
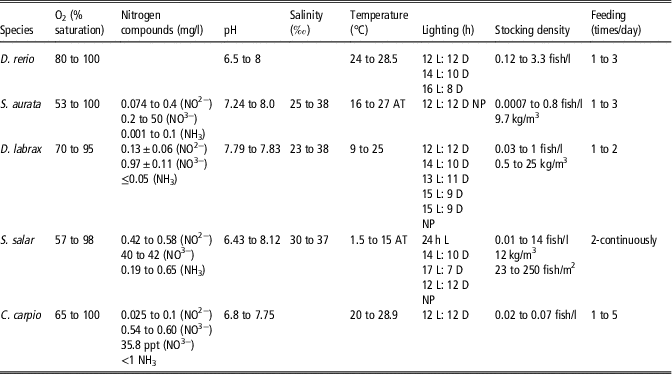
h=hour; L=light; D=dark; AT=ambient temperature; NP=not present.
The present literature review shows that, even when individual research groups have adopted the European Directive (2010) and the ARRIVE guidelines (Table 3), the information on housing conditions provided in the published papers is generally too incomplete to allow for a full assessment of animal health and the reproducibility of the experiment.
Table 3 References of scientific publications authored by European researchers published in 2014 and 2015 that provide information on the specific environmental parameter listed by Directive 2010/63/EU (2010) focused on Danio rerio, Sparus aurata, Dicentrarchus labrax, Salmo salar and Cyprinus carpio
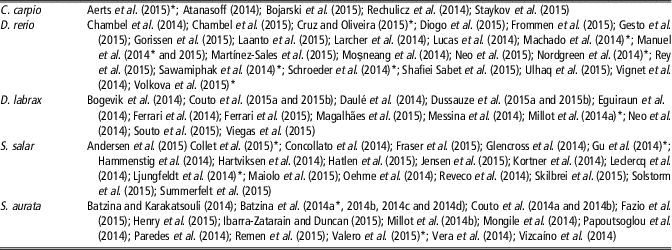
Extended references are reported in Supplementary Material S1.
* Papers published in scientific journals endorsing the Animal Research: Reporting of In Vivo Experiments guideline.
Discussion
Fish are an important biological resource for humanity as food through fisheries and aquaculture that allow for sustainable production and are useful study models for scientific research. Fish housing requires attention to aspects related to animal welfare and health. The adoption of welfare protocols in an attempt to reduce the overall stress level in fish can enhance the success of experiments by avoiding procedural bias due to unwanted behavioural and physiological alteration of the study subject, and can also improve the profitability of aquaculture, increasing growth performance, flesh quality, fish health and reproductive success. The best approach to welfare assessment depends on factors such as species, type of housing and the number of animals involved. In the scientific field, the nature of the research should also be considered, and protocols for monitoring fish welfare should be provided within individual projects (Hawkins et al., Reference Hawkins, Morton, Burman, Dennison, Honess, Jennings, Lane, Middleton, Roughan, Wells and Westwood2011).
The European Directive 2010/63 (2010) and Recommendation on farmed fish are important steps forward to ensure the welfare of fish used for scientific purposes and commercial activity. However, despite these efforts, information provided about fish sometimes appears too generic and concise to serve as a useful tool to guarantee fish health. The wide biological diversity of fish species and their different physiological and behavioural needs are scarcely considered. Annex III of Directive 2010/63/EU (2010), for example, contains very little consideration of species-specific features and does not even provide specific instructions for each species nor reference documents on which laboratories can rely. These are severe limits to the applicability of the Directive itself. Indeed, if compared with the guidelines previously provided in Recommendation 2007/526/CE, the actual Directive appears even more concise and lacking contextualization.
Another limitation of the current Directive 2010/63/EU (2010) is the lack of guidance on how to measure the chemical and physical parameters of interest, which makes the standardization of procedures for animal housing among different laboratories more difficult. This appears in contrast with the concept of harmonization in the care and use of laboratory animals. Harmonization of procedures would make results obtained by different laboratories comparable, thus allowing for the reduction of the number of experimental animals in each lab. Moreover, harmonization procedures in animal care, as encouraged by the present Directive (2010), are needed to facilitate the establishment of sharing programmes for organs and tissues of animals killed in accordance with the principle of Reduction (Russell and Burch, Reference Russell and Burch1959). A detailed protocol indicating precise guidelines for the proper maintenance and breeding has been provided for zebrafish (D. rerio) (Avdesh et al., Reference Avdesh, Chen, Martin-Iverson, Mondal, Ong, Rainey-Smith, Taddei, Lardelli, Groth, Verdile and Martins2012), the most widely used teleost animal model. The development of such protocols should also be encouraged for other fish species or groups of species, as these may help researchers in the process of harmonization and standardization of procedures.
Similar considerations also apply to aquaculture, where the need for more detailed guidelines in the assessment of fish welfare is equally urgent. Finally, to improve the quality of interventions aimed at ensuring fish welfare in both the research and aquaculture context, a large amount of experimental research is still needed.
According to the present work and previous review articles on this issue (Huntingford et al., Reference Huntingford, Adams, Braithwaite, Kadri, Pottinger, Sandøe and Turnbull2006; Ashley, Reference Ashley2007), welfare indicators cannot be easily defined. Indicators may be based on health status, stress response and behavioural performance and an array of indicators is often used, whereas integrated indicators based on standardized procedures are rarely found in the current literature.
Integrated welfare indicators that can be easily used and compared by laboratory and aquaculture operators must be developed to assess fish welfare conditions effectively and to improve the quality of husbandry practices not only from a productive point of view but, also from a more holistic, ecological and ethical perspective. In addition, although some recent studies have indicated significant effects of slaughter and pre-slaughter practices on flesh quality (Poli et al., Reference Poli, Parisi, Scappini and Zampacavallo2005; Lefevre et al., Reference Lefevre, Cos, Pottinger and Bugeon2016), information on how animal wellness can affect the organoleptic and nutritional properties of the fish flesh are needed to improve housing conditions in aquaculture farms. The main difficulties in achieving these goals are due to the great level of both inter- and intra-specific variation characterizing the teleost group, as this complicates the development of standardized protocols. Behavioural studies and related technologies are, perhaps, one of the most developed research areas that can provide the most effective tools for welfare assessment, but even in this field additional scientific research remains necessary to allow for the widest implementation of its results.
As the welfare of animals housed in captivity can only be maintained by knowing the characteristics and needs of the specific species, it is necessary to conduct new research and pilot studies aimed at characterizing the chosen species when detailed information is not available. An increase in scientific studies on teleost fish would raise the number of species that can be farmed in aquaculture centres or used as study models in scientific research. It is important that scientific papers reporting the results of studies on fish should provide a precise description of the housing conditions to be truly informative and useful, and in this sense, it is desirable that editors of scientific journals rigorously endorse the ARRIVE guidelines and require, according to a common form, more detailed information regarding the essential parameters indicated by the European Directive to ensure animal welfare.
Conclusions
The fish housing implemented in both aquaculture and in scientific research requires attention to the assessment of fish welfare. However, this is a complex issue due to the high number of environmental factors to be considered, the shortage of welfare indicators and the high number of teleost species. European Directive 2010/63 (2010) and Recommendations have been established to ensure optimal conditions for farmed and laboratory animals. Although European legislation is a useful and informative tool, further and more in-depth research is needed to bridge the cognitive gaps in the abovementioned areas. It is crucial to produce global legislation ensuring fish welfare in the contexts of both research and aquaculture that prevent different countries from applying different animal treatment procedures.
Acknowledgements
The authors thank Dr Roberto Serrai for the language revision and Dr Augusto Vitale for the valuable assistance in manuscript revision. The English editing was performed by the American Journal Experts service. This study was supported by FIRB Futuro in Ricerca 2012 (RBFR12QW4I_002) and by Sapienza University of Rome (Progetti di Ricerca 2016).
Declaration of interest
None.
Ethics statement
None.
Software and data repository resources
None.
Supplementary Material
To view supplementary material for this article, please visit https://doi.org/10.1017/S1751731118000940