Energy is a component of poultry diets, representing about half of the expenses that producers have to pay for broilers’ feed( Reference Cooke 1 ). At the same time, a part of this energy is wasted in the body by heat increment produced during the utilisation of different chemical components( Reference Noblet, Van Milgen and Dubois 2 ). As such, nutritionists are always looking for novel approaches to improve availability of energy for broiler chickens. One mechanism to store the flow of energy in the body within the cells is mediated through the formation of high-energy phosphate bonds( Reference Lemme, Ringel and Sterk 3 ). However, impaired bioenergetics is accompanied by low levels of creatine – a compound that plays a key role in the formation of phosphate-bound cellular energy( Reference Ostojic 4 ). Poultry are uricotelic animals, lacking enzymes to synthesise enough arginine de novo as a precursor for creatine( Reference Campbell 5 ). In addition, in average, 1·7 % of the total body creatine and phosphocreatine pool is irreversibly converted to creatinine and excreted in the urine, daily( Reference Wyss and Kaddurah-Daouk 6 ). Therefore, creatine replenishment from an exogenous source is necessary, particularly in younger than in adult animals( Reference Brosnan, Wijekoon and Warford-Woolgar 7 ). However, nowadays, diets of broilers are free of animal by-products, which reduces the chance of animals to obtain exogenous creatine from an all-vegetable feed( Reference Wallimann, Tokarska-Schlattner and Neumann 8 ).
Guanidinoacetic acid (GAA) is a readily available precursor for creatine, synthetised in the kidney from glycine and l-arginine by l-arginine:glycine amidinotransferase. In the second reaction, GAA forms creatine in the liver via the action of guanidinoacetate N-methyltransferase. The feed-grade GAA is provided commercially and has a better potential to be included in practical broilers’ diets because it is more stable and cost-efficient than creatine( Reference Baker 9 ). Supplementation of dietary GAA was shown to have sparing effects for arginine, which increase arginine availability in broiler chickens( Reference Baker 9 ). In addition, arginine has influenced the intestinal morphometric features( Reference Khajali, Moghaddam and Hassanpour 10 ); therefore, it may possible that dietary inclusion of GAA affects the intestinal digestibility and absorption of nutrients. In the recent works, GAA was found to be more efficient than dietary creatine for improving the muscular creatine stores in human( Reference Ostojic, Ostojic and Drid 11 ) and Yucatan miniature pigs( Reference McBreairty, Robinson and Furlong 12 ). Moreover, GAA enhances the ratio of muscular creatine and phosphocreatine to ATP in broiler chickens( Reference Lemme, Ringel and Sterk 3 , Reference Michiels, Maertens and Buyse 13 ). In addition, supplemental GAA had beneficial effects on growth performance of broilers( Reference Lemme, Ringel and Rostagno 14 ) and turkey( Reference Lemme, Gobbi and Helmbrecht 15 ), whereas the same effects were not observed in the other trials( Reference Michiels, Maertens and Buyse 13 , Reference Abudabos, Saleh and Lemme 16 , Reference Mousavi, Afsar and Lotfollahian 17 ). The impact of GAA on daily feed intake (DFI) of broilers is equivocal( Reference Michiels, Maertens and Buyse 13 , Reference Ringel, Lemme and Knox 18 , Reference Tossenberger, Rademacher and Németh 19 ); however, we expected that GAA inclusion in diets with lower energy content may improve overall body energy retention as fat (REf) or protein (REp), thereby contributing to a better understanding of energy utilisation in broiler chickens. Most researches investigating the effects of GAA have focused on growth performance or concentration of muscular energetic metabolites in broilers receiving diets containing various levels of apparent metabolisable energy (AME) contents, and less attention has been payed to effect of supplemental GAA on dietary net energy for production (NEp).
We hypothesised that supplementation of GAA in diets with different energy contents may influence energy utilisation, intestinal morphology and consequently growth performance of broiler chickens. Furthermore, we hypothesised that dietary inclusion of GAA could affect NEp in broiler chickens. Therefore, the current study was conducted to evaluate effects of GAA (0, 0·6 and 1·2 g/kg) and energy content of the diet (with or without energy reduction) on production performance, gut morphology, blood parameters and energy utilisation of broiler chickens using comparative slaughter technique.
Methods
All experimental procedures were evaluated and approved by the Institutional Animal Care and Ethics Committee of the Lorestan University.
Animals, diets and experiment design
Male broiler chickens (Ross 308), 390 d old, were purchased from a commercial hatchery and used in this experiment. Upon arrival, chicks were weighed, wing-banded and randomly assigned to the treatment groups so that the initial weights were similar among different treatment groups. Five replicate cages of thirteen chicks each were randomly allotted to six dietary treatments based on a factorial arrangement of treatments (2×3) in a completely randomised design across starter (1–15 d) and growing (15–35 d) periods. Experimental treatments included two basal diets with standard (STD; starter: 12·56 MJ/kg and grower: 12·97 MJ/kg) or reduction (LME; starter: 11·93 MJ/kg and grower: 12·33 MJ/kg) of AME requirement of broiler chickens (Ross 308) each supplemented with 0, 0·6 and 1·2 g/kg GAA (CreAMINO®; Evonik Degussa Gmbh) by replacing with an equal quantity of maize and soyabean meal. Diets were formulated to be iso-energetic and iso-nitrogenous and to meet or marginally exceed nutrient requirements provided by Ross manual (Aviagen( 20 ); Tables 1 and 2) across different periods. Birds had free access to feed and water throughout the experiment. All experimental diets were fed in mash form. The birds were reared in a power-ventilated broiler house equipped with battery cages (length 124 cm × width 65 cm). Birds’ density was 7·7 kg/m2 during days 1–15 of age and 23·4 kg/m2 during days 15–35 of age. The lighting programme consisted of 23 h light and 1 h darkness. The light was provided by incandescent bulbs, and the light intensity at bird level was 30 lux. Ambient temperature was kept at 31°C for days 1–3 and gradually decreased thereafter to 24°C by the end of the 3rd week. Relative humidity was maintained between 50 and 70 %.
Table 1 Dietary composition and nutrients during starter period
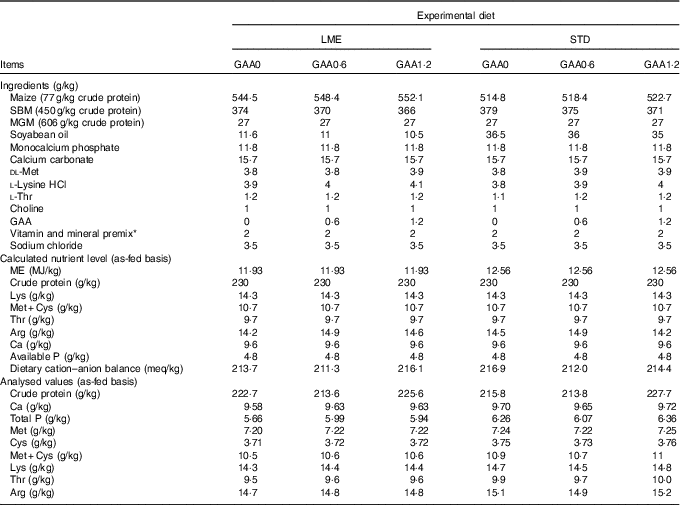
LME, basal diet with energy reduction below the level recommended by Ross 308 (2014) broiler manual (starter: 11·93 MJ/kg and grower: 12·33 MJ/kg); STD, basal diet with energy level recommended by Ross 308 (2014) broiler manual (starter: 12·56 MJ/kg and grower: 12·97 MJ/kg); GAA0, basal diet; GAA0·6, basal diet supplemented with 0·6 g/kg guanidinoacetic acid; GAA1·2, basal diet supplemented with 1·2 g/kg guanidinoacetic acid; SBM, soyabean meal; MGM, maize gluten meal; GAA, guanidinoacetic acid.
* Vitamin premix provided per kg of diet: vitamin A (retinol), 3600 μg; vitamin D3 (cholecalciferol), 125 μg; vitamin E (tocopheryl acetate), 63 mg; vitamin K3, 3·5 mg; thiamine, 3 mg; riboflavin, 7·5 mg; pantothenic acid, 18 mg; pyridoxine, 4·3 mg; cyanocobalamin, 0·017 mg; niacin, 65 mg; biotin, 0·3 mg; folic acid, 2 mg; choline chloride, 600 mg; antioxidant 100 mg; mineral premix provided per kg of diet: Fe (FeSO4.7H2O, 20·09 % Fe), 80 mg; Mn (MnSO4.H2O, 32·49 % Mn), 120 mg; Zn (ZnO, 80·35 % Zn), 110 mg; Cu (CuSO4.5H2O), 16 mg; iodine (KI, 58 % I), 1·3 mg; Se (NaSeO3, 45·56 % Se), 0·3 mg.
Table 2 Dietary composition and nutrients during growing period

LME, basal diet with energy reduction below the level recommended by Ross 308 (2014) broiler manual (starter: 11·93 MJ/kg and grower: 12·33 MJ/kg); STD, basal diet with energy level recommended by Ross 308 (2014) broiler manual (starter: 12·56 MJ/kg and grower: 12·97 MJ/kg); GAA0, basal diet; GAA0·6, basal diet supplemented with 0·6 g/kg guanidinoacetic acid; GAA1·2, basal diet supplemented with 1·2 g/kg guanidinoacetic acid; SBM, soyabean meal; GAA, guanidinoacetic acid.
* Vitamin premix provided per kg of diet: vitamin A (retinol), 3600 μg; vitamin D3 (cholecalciferol), 125 μg; vitamin E (tocopheryl acetate), 63 mg; vitamin K3, 3·5 mg; thiamine, 3 mg; riboflavin, 7·5 mg; pantothenic acid, 18 mg; pyridoxine, 4·3 mg; cyanocobalamin, 0·017 mg; niacin, 65 mg; biotin, 0·3 mg; folic acid, 2 mg; choline chloride, 600 mg; antioxidant 100 mg; mineral premix provided per kg of diet: Fe (FeSO4.7H2O, 20·09 % Fe), 80 mg; Mn (MnSO4.H2O, 32·49 % Mn), 120 mg; Zn (ZnO, 80·35 % Zn), 110 mg; Cu (CuSO4.5H2O), 16 mg; iodine (KI, 58 % I), 1·3 mg; Se (NaSeO3, 45·56 % Se), 0·3 mg.
Data collection and sampling
Feed intake and live body weight (BW) of chickens in each cage were recorded individually from 1 to 35 d, and DFI, daily weight gain (DWG) and feed conversion ratio (FCR; feed intake/weight gain) were calculated accordingly. On days 15 and 35 of the experiment, two birds close to the mean BW of cage were individually weighed and slaughtered. Carcass, breast, leg, abdominal fat and liver were collected, weighed and expressed as a percentage of BW.
At the beginning of the experiment (day 1), thirty randomly selected birds were killed by cervical dislocation and stored at −20°C pending carcass analyses . At day 8 post hatch, two birds from each cage (those with the closest BW to the cage average) were selected, weighed and transferred to one of thirty metabolic cages (length 60×width 30×height 30 cm) located in a temperature-controlled room with the ambient temperature of 24°C and relative humidity of 60 %. The average area, equivalent to that occupied by these two chicks in our main cages, was calculated and restricted by wire mesh for the remaining chicks in order to keep the stocking density of 7·7 kg/m2 per cage until day 15 of experiment. In metabolic cages, all birds had free access to the experimental diets for preliminary 4-d adaptation period followed by 3 d of excretion collection in triplicate. A source of acid-insoluble ash (AIA; Celite*545; Merck KGaA) was added to all diets (10 g/kg) as an indigestible marker to measure the AME of experimental diets during this period. Excreta were collected using trays located beneath each cage to determine AME. Contamination in excreta (e.g. feathers and skin debris) was carefully removed and the collected excreta per cage during the collection period were pooled and stored at −20 °C. Before analysis, samples of excreta were thawed and dried at 60°C for 48 h in a forced air oven, ground to pass through a 0·5-mm sieve and stored in airtight plastic containers. After collection period, all birds were weighed, euthanised by cervical dislocation and immediately frozen. A comparative slaughter technique was applied to determine retention of nutrients. In brief, the whole carcass of frozen birds (feathers, head, feet and all organs) was minced twice, and thoroughly mixed in a blender to obtain a homogeneous sample of approximately 200 g. The samples were freeze-dried and two sub-samples from each replicate were mixed after drying and ground together. Hence, chemical analyses were performed on one sample per cage. The same procedure was applied to the carcass of thirty birds taken at the initiation of the experiment. The data were used to determine the DM, carcass REf and REp in broiler chickens during days 1–15 of the experimental period.
Morphology of small intestine
Two birds from each cage were slaughtered on day 15, and segments of their small intestine were sampled from the midpoint of the duodenum (intestine from the gizzard to pancreatic and bile ducts) and jejunum (midway between the point of entry of the bile ducts and Meckel’s diverticulum). The intestinal samples were evaluated for the villus height, crypt depth, villus height:crypt depth ratio, villus width and villus surface area. Segments with 1·5-cm length were gently flushed twice with physiological saline solution (1 % NaCl) to remove intestinal contents and placed in 10 % formalin in 0·1 m phosphate buffer (pH=7·0) for fixation. The samples were processed for 24 h in a tissue processor with ethanol as dehydrant and were embedded in paraffin. Sections (5 μm) were made and stained with haematoxylin–eosin. An optical microscope (Olympus CX31) was used for morphological examination of small intestine samples. A total of ten intact, well-oriented villus–crypt units were selected for each intestinal cross section (three cross-sections/sample and thirty-six cross-sections/treatment, for a total of 360 measurements/treatment). Villus height (μm) was measured from the tip of the villus to the villus crypt junction, and crypt depth was defined as the depth of the invagination between two villi. Villus height:crypt depth ratio (V:C) was then calculated. Villus width was measured at the middle point of the villus. The formula for calculating villus surface area was 2π×(villus width/2)×villus height. The average of values for each cross section was used for data analysis.
Blood parameters
At 35 d of age, two birds from each pen were randomly selected for blood sampling via a wing vein. The serum was harvested by centrifuging the blood samples at 3000 g for 20 min at 4 °C using a centrifuge (Pars Azma), and stored at −20°C until required. We determined serum levels of total protein (TP), uric acid (UA) and creatinine using commercial kits (Pars Azmoon). All analyses were performed in duplicate.
Laboratory analyses
Before trial commencement, feed ingredients were analysed by Evonik Industries AG animal nutrition analytical laboratory for crude protein (AMINOProx®), amino acids (AMINONIR®), diethyl ether extract (AMINOProx®), DM (AMINOLab®) and total and phytate phosphorous (AMINOProx®) contents. Furthermore, dietary samples were analysed for the profile of amino acids by AMINOLab®. In addition, the experimental diets and the excreta were analysed for gross energy (GE) and AIA in order to determine dietary AME. The GE was determined using an adiabatic bomb calorimeter (Gallenkamp) standardised with benzoic acid. The AIA in feed and excreta was determined using the method of Vogtmann et al. ( Reference Vogtmann, Pfirter and Prabucki 21 ). The N contents of the feed and freeze-dried carcass samples were determined by the Kjeldahl method (984.13)( 22 ). The crude fat in the feed and carcass samples was extracted using soxhelt fat analysis according to the method 920.39 of Association of Official Analytical Chemists( 22 ).
Calculations
A series of calculations were carried out to calculate energy utilisation according to Olukosi et al. ( Reference Olukosi, Cowieson and Adeola 23 ) and Pirgozliev & Bedford( Reference Pirgozliev and Bedford 24 ). Dietary AME (MJ/kg) was calculated as follows:
AME (MJ/kg of diet)=GEf−(GEe×(AIAd)/(AIAe)),
where GEf is the GE (MJ/kg) of the feed, GEe is the GE (MJ/kg) of the excreta, AIAd is the AIA in the diets (%) and AIAe is the AIA in the excreta (%).
The total carcass GE retained in the body was calculated as the sum of the carcass REp and REf. The total carcass protein retention (CPr, g/bird) was calculated as follows:
CPr=(N15−N1)×6·25,
where N15 is the N (g) in chicken carcasses at 15 d old, N1 is the N (g) in chicken carcasses at the beginning of the experiment at 1 d old and 6·25 is the coefficient used to calculate the REp in the body.
The value of carcass GE retained as REp was calculated as follows:
REP=CPr × 23·6 MJ,
where CPr (kg) is multiplied by 23·6 MJ, the amount of energy in 1 kg of protein.
The total carcass fat retention (CFr, g/bird) was calculated similar to CPr as follows:
CFr=(F15−F1),
where F15 is the fat (g) in chicken carcasses at 15 d old; F1 the fat (g) in chicken carcasses at the beginning of the experiment at 1 d old.
The value of the carcass REf was calculated as follows:
REf=CFr×39·12 MJ,
where CFr (kg) is multiplied by 39·12 MJ, the amount of energy in 1 kg of fat.
Total energy retained in the carcass (REc) was calculated as follows:
REc (MJ)=(REp + REf).
NEp (MJ/kg) was calculated using the following equation:
NEp (MJ/ kg)=(REc)/FI,
where FI is the average amount of feed (kg/bird) consumed from 1 d old until day 15.
The efficiency of AME used for energy retention (Kre) was calculated as the REc divided by AME intake.
Kre=REc/AME intake,
where AME intake is the FI (kg/bird) for the experimental period multiplied by determined metabolisable energy (MJ/kg) of the diets.
Total heat production (HPt) of birds from 1 d old to 15 days old consisting energy for tissue retention, maintenance and heat increment of production was calculated as follows:

Heat production per kg of feed intake (HPf, MJ/kg feed intake) was calculated as follows:
HPf (MJ/kg feed intake)=(HPt)/FI,
where HPt is the total heat production of the birds from 1 d old to 15 d old (MJ), and FI (kg) consumed. The NEp:HPf ratio describes the relative efficiency of the use of metabolisable energy between body energy retention and heat production, implicit that a more efficient split in energy towards production rather than heat increment is related to a higher ratio.
Statistical analysis
Data were analysed as a 2×3 factorial arrangement based on a completely randomised design using the GLM procedure of SAS 9.2 (SAS Institute Inc.) according to the following model:

where µ is the overall mean, A i is the fixed effect of dietary energy contents (STD and LME), B j is the fixed effects of dietary GAA supplementation (0, 0·6 and 1·2 g/kg), AB ij is the interaction of dietary energy contents and GAA supplementation and e ij is the random residual error. Performance and energy utilisation data were analysed considering all birds in a cage as an experimental unit. Because of a possible influence of variation in AME intake on energy utilisation response criteria, the AME intake was used as a covariate in the analysis of energy utilisation. When a significant F-test was detected (P<0·05), corresponding means were separated by Tukey’s test, and the interaction between treatments was analysed using an least square means test adjusted for Tukey’s test. Pearson’s correlation coefficient was employed using CORR procedure of SAS to determine any correlation between growth performance and energy utilisation data. Whenever the interaction effects of main factors were significant, the main effects were not further discussed. For all statistical analyses, significance was declared at P≤0·05 and trends at P≤0·10, unless otherwise stated. Specific orthogonal contrasts were used to test linear and quadratic effects of treatments.
Results
Growth performance and carcass measurements
There was a linear interaction between dietary energy contents and GAA level during 1–15, 15–35 and 1–35 days of age on DWG of broilers (Table 3; P<0·001). In all noted periods, reduction of dietary energy from STD diet to LME significantly decreased DWG of broilers, which was ameliorated by 6·1 % after supplementing with 1·2 g/kg dietary GAA (P<0·05). Additionally, DWG increased numerically by 5·2 % when 0·6 g/kg GAA was added to the LME diet across the entire rearing period compared with the birds fed non-supplemented STD diet (P<0·1). A linear interaction of dietary energy content × GAA was observed for FCR in growing (P<0·014) and whole rearing periods (P<0·001), where the FCR impaired with consumption of LME feed, but reversed by dietary supplementation with 0·6 g/kg or 1·2 g/kg GAA (P<0·05). Dietary energy level interacted with GAA (quadratic; P<0·001) for the DFI during starter period so that the feed consumption was greater in broiler chickens fed STD diet supplemented with 1·2 g/kg GAA than those fed LME diet supplemented with 0·6 g/kg GAA (P<0·05).
Table 3 Effects of dietary treatments on the performance of broiler chickens at different ages

GAA, guanidinoacetic acid; STD, basal diet with energy level recommended by Ross 308 (2014) broiler manual (starter: 12·56 MJ/kg and grower: 12·97 MJ/kg); LME, basal diet with energy reduction below the level recommended by Ross 308 (2014) broiler manual (starter: 11·93 MJ/kg and grower: 12·33 MJ/kg).
a,b,c,d Values in the same column with unlike superscript letters were significantly different (P<0·05).
On day 35 of age, the abdominal fat was significantly greater in birds fed STD diet containing 1·2 g/kg GAA than those fed LME diets without any supplemental GAA (online Supplementary Table S1; P<0·05). Other carcass traits were neither affected by experimental diets nor by age (15 or 35 d of age).
Metabolism of energy and dietary apparent metabolisable energy
There were linear dietary energy content × GAA interactions for CFr (P<0·001; Table 5), REc (P=0·0063; Table 5), REf (P<0·001; Table 4), NEp (P=0·020; Table 5), HPt (P=0·004; Table 5), HPf (P=0·009; Table 5) and NEp:HPf (P=0·010; Table 4). In addition, a quadratic interaction was observed for dietary AME (P=0·006; Table 4). Birds fed low AME diets with no supplemental or 0·6 g/kg GAA had significantly lower CFr, REf and REc than the chickens of other groups (P<0·05; Table 4). However, this trend was compensated by dietary supplementation of LME diet with 1·2 g/kg GAA (P<0·05; Table 4). On the other hand, dietary inclusion of GAA in STD diets did not affect the carcass energy retention. The kre tended to increase by 2·97 % when the LME diet was supplemented with 1·2 g/kg GAA (P<0·1; Table 4). The NEp content of basal LME diet decreased compared with the STD diet, but it was retrieved when the diet was supplemented with 1·2 g/kg of GAA (P<0·05; Table 4). Further, supplementing STD diet with 0·6 g/kg GAA tended to enhance its NEp content by 4·4 % (P<0·1; Table 4). Although HPt was not affected by the GAA supplementation in STD diets, it was remarkably increased in broilers fed LME diets supplemented with 1·2 g/kg GAA (P<0·05; Table 4). Moreover, HPf was lower in broilers fed LME diet with no supplemental GAA or with 1·2 g/kg supplemental GAA compared with those fed STD diets (P<0·05; Table 4).
Table 4 Effects of dietary treatments on energy utilisation

GAA, guanidinoacetic acid; CPr, retained carcass protein (g/bird); CFr, retained carcass fat (g/bird); REc, total carcass energy retained in a bird from 0 to 15 d of age; REp, carcass gross energy retained as carcass protein; REf, carcass gross energy retained as carcass fat; Kre, efficiency of dietary apparent metabolisable energy retention; NEp, net energy for production (carcass energy retained per kg feed intake); HPt, total heat production from 0 to 15 d of age; HPf (MJ), heat production per kg feed intake; STD, basal diet with energy level recommended by Ross 308 (2014) broiler manual (starter: 12·56 MJ/kg and grower: 12·97 MJ/kg); LME, basal diet with energy reduction below the level recommended by Ross 308 (2014) broiler manual (starter: 11·93 MJ/kg and grower: 12·33 MJ/kg).
a,b Values in the same column with unlike superscript letters were significantly different (P<0·05).
Table 5 Correlations between growth performance and energy utilisation on day 15 of age

AME, apparent metabolisable energy; REp, carcass gross energy retained as carcass protein; REf, carcass gross energy retained as carcass fat; REc, total carcass energy retained in a bird from 0 to 15 d of age; NEp, net energy for production (carcass energy retained per kg feed intake); HPt, total heat production from 0 to 15 d of age; HPf (MJ), heat production per kg feed intake; Kre, efficiency of dietary apparent metabolisable energy retention; DWG, daily weight gain; DFI, daily feed intake; FCR, feed conversion ratio.
Morphology of small intestine
Irrespective of GAA supplementation, reducing energy content of diet decreased villus height (quadratic; P<0·001; online Supplementary Table S2) and crypt depth (quadratic; P=0·002; online Supplementary Table S2). However, the V:C was not affected by energy content of diets (online Supplementary Table S2). The intestinal morphometric features in the duodenum were not affected by dietary treatments (online Supplementary Table S2). Moreover, energy content and GAA levels did not interact significantly for the intestinal morphometric features (online Supplementary Table S2).
Correlations
Table 5 presents the Pearson’s correlations between growth performance and energy utilisation parameters in broiler chickens. There was a positive and significant correlation between DWG and dietary NEp during 1–15 d of age (r 0·493; P=0·005), but during the same period DWG was not correlated to AME of diets. A negative correlation was found between FCR and NEp (r −0·591; P<0·001), but not with dietary AME across the starter period.
Blood parameters
An interaction of GAA supplementation and dietary energy level influenced serum concentration of TP (P=0·049; Table 6), UA (P=0·048; Table 6) and creatinine (P=0·049; Table 6). The greatest concentration of serum TP was observed in birds fed LME diet supplemented with 1·2 g/kg GAA, which was significantly greater than those fed STD diet without GAA supplementation (P<0·05). The serum concentration of UA was highest when broilers received the basal LME diet that was greater than the birds fed STD diets or fed STD feed supplemented with 1·2 g/kg GAA (P<0·05). Chickens subjected to LME feed supplemented with 1·2 g/kg GAA had the maximum concentration of serum creatinine, particularly compared with broilers fed basal STD diet (P<0·05).
Table 6 Effects of dietary treatments on the blood parameters of broiler chickens

GAA, guanidinoacetic acid; TP, total protein; UA, uric acid; STD, basal diet with energy level recommended by Ross 308 (2014) broiler manual (starter: 12·56 MJ/kg and grower: 12·97 MJ/kg); LME, basal diet with energy reduction below the level recommended by Ross 308 (2014) broiler manual (starter: 11·93 MJ/kg and grower: 12·33 MJ/kg).
a,b Values in the same column with unlike superscript letters were significantly different (P<0·05).
Discussion
In the present experiment, diets were formulated with two different energy contents and supplemented with graded levels of GAA in order to study productive performance and energy utilisation in broiler chickens in response to GAA addition. Dietary supplementation with 1·2 g/kg GAA elevated the compromised growth, and improved the FCR of birds fed LME diets without GAA inclusion. Effects of GAA on the growth performance was likely owing to its key role as a creatine precursor and subsequently its ability to increase the muscular creatine and ATP stores( Reference Lemme, Ringel and Sterk 3 ). Creatine plays the role of a carrier in the form of phosphocreatine, enhancing the energy delivery to high demanding energy tissues such as muscles and heart( Reference Wyss and Kaddurah-Daouk 6 ). It seems that the improving effects of GAA are more prominent in low-energy and animal-product-free diets where the energy would be limiting for growth of broiler chickens with high-energy demands. These findings were consistent with the results of Lemme et al. ( Reference Lemme, Ringel and Rostagno 14 ) who observed an improvement in growth of male and female broilers fed all vegetable diets supplemented with 0·6 or 1·2 g/kg of GAA. Similarly, feeding turkeys with vegetable-based diets supplemented with 0·8 g/kg GAA increased DWG across 1–63 d of age( Reference Lemme, Gobbi and Helmbrecht 15 ). Moreover, there are some trials reporting the beneficial effect of GAA on FCR of broilers, whereas their DWG was not significantly affected( Reference Michiels, Maertens and Buyse 13 , Reference Abudabos, Saleh and Lemme 16 , Reference Mousavi, Afsar and Lotfollahian 17 ). Data on feed consumption showed a reduction in birds fed LME diet supplemented with 0·6 g/kg GAA during the starter period; however, this parameter did not change in birds subjected to the other levels of energy or GAA supplementation. As such, a comparative slaughter technique was used during the starter period to clarify the context of energy utilisation, particularly in the early stages of chick’s life.
Results showed that energy was deposited in the carcass of birds mainly as protein than fat during the early stages of chick’s life. Generally, broiler chickens proportionally gain more carcass protein than fat in their early stage of growth, which is supported by previous reports( Reference Pirgozliev and Bedford 24 , Reference Jamroz, Wiliczkiewicz and Wertelecki 25 ). Improvements in energy utilisation depend upon the enhancement in nutrient and energy availability, leading to deposition of carcass energy in forms of fat and protein( Reference Olukosi, Cowieson and Adeola 23 ). Indeed, lower DWG, CFr, REc and abdominal fat in broilers fed LME diet could be owing to their reduced energy utilisation as the measured dietary NEp value was lower in these birds. In this regard, supplemental GAA successfully ameliorated the reduced growth and carcass energy retention of birds fed LME diet, suggesting the efficacy of this compound to improve energy utilisation in broiler chickens. Further, GAA supplementation in STD diets did not affect the body energy retention, providing the evidence that GAA works better in low-energy diets. This is against the previous reports in which researchers failed to detect any interaction between GAA inclusion and dietary energy content on the growth of broilers( Reference Abudabos, Saleh and Lemme 16 , Reference Mousavi, Afsar and Lotfollahian 17 ).
In the present experiment, NEp for LME feed containing 1·2 g/kg GAA was greater than the same diet lacking supplemental GAA, which was in line with their estimated higher HPt, REc, Kre and consequently DWG. However, dietary AME was not affected in response to dietary supplementation with GAA. In this regard, we found that DWG of broilers was positively correlated to dietary NEp, but not to AME. In other words, the dietary AME was not affected in response to dietary supplementation with GAA compared with NEp. Beneficial effects of GAA on NEp could be attributed to increased buffering capacity for ATP by phosphocreatine hydrolysis in muscles, consistent with the idea that GAA increases muscle growth or work; therefore, chickens spent more energy towards growth rather than maintenance. In this study, we did not measure the effect of GAA on net energy for maintenance; therefore, further investigations are warranted.
The HPt decreased in chickens that received LME diets, but was elevated by dietary inclusion of 1·2 g/kg GAA. It has been reported that a higher plane of nutrition leads to a greater heat production( Reference Johnson 26 ). Hence, lower HPt in LME diets was due to decreased dietary energy intake through the energy sources of dietary ingredients. Energy expenditure comprises tissue respiration and energy-dependent nutrient transportation such as Na-K ATPase( Reference Olukosi, Cowieson and Adeola 23 ). Furthermore, organs such as liver, gastrointestinal tract and pancreas are involved in almost 30 % of fasting heat production( Reference Spratt, McBride and Bayley 27 ). Experimental evidence has shown that GAA may affect the proportional weight of the liver( Reference Abudabos, Saleh and Lemme 16 , Reference Mousavi, Afsar and Lotfollahian 17 ). Because liver weight in the current trial was not changed in response to the inclusion of GAA, it is likely that higher heat production in broilers given GAA was due to the increased energy expenditure associated with maintenance of skeletal muscles and fat, taking into consideration that heat production for muscle deposition is more than fat( Reference Close 28 ). This speculation seems plausible as dietary supplementation of LME diet with 1·2 g/kg GAA increased energy REf and REp in broilers’ carcass.
Morphology of certain segments of small intestine was studied to evaluate whether dietary supplementation with GAA affects intestinal digestibility of nutrients. Although the impact of arginine as a precursor for GAA and creatine was evaluated previously( Reference Khajali, Moghaddam and Hassanpour 10 ), there is a dearth of reports linking the effects of GAA in diets with reduction of energy content on the intestinal morphology. Our results indicated that gut morphometrical attributes were not changed in the birds fed GAA-added diets. Therefore, it could be postulated that GAA supplementation in the diet may have no effect on intestinal digestibility of nutrients in broiler chickens. We found that reduction in energy content of diet decreased villus height and crypt depth in the jejunum. A deeper intestinal crypt is a criterion for greater tissue turnover and the need for new tissue, which increases the demand for maintenance energy( Reference Choct 29 ). Therefore, the lower depth of crypt and height of villi when birds subjected to lower energy diets may stand to reason that epithelial cells of intestine encountered with the lack of energy required for their proliferation. Analogous to our data, Fleming et al. ( Reference Fleming, Youngman and Ames 30 ) found that proliferation of epithelial cells in the intestine of young male rats decreased by lowering the energy intake. Moreover, Ale Saheb Fosoul et al. ( Reference Ale Saheb Fosoul, Toghyani and Gheisari 31 ) reported the anatomical variations in the small intestine of broiler chicken given diets with alternating energy contents.
UA is the final and main poultry nitrogenous waste product( Reference Harr 32 ). Reduction in serum concentration of UA in the present experiment agreed with studies in which reduction of dietary energy to protein ratio increased plasma concentration of UA in broiler chickens( Reference Rosebrough, McMurtry and Vasilatos-Younken 33 , Reference Rosebrough, McMurtry and Vasilatos-Younken 34 ). Moreover, dietary energy restriction has been shown to increase the plasma UA( Reference Chen, Guo and Huang 35 ). Indeed, any changes in protein metabolism could be reflected as altered blood concentration of UA( Reference Rajman, Juráni and Lamošová 36 ). High levels of serum UA is likely associated with utilisation of structural proteins as energy sources when animals encounter energy restriction. In the present study, serum UA decreased marginally after supplementing LME diet with GAA, supporting the idea that GAA supplementation improves protein and energy utilisation. Besides, serum concentration of TP was the greatest in chickens fed LME diet supplemented with 1·2 g/kg GAA, which further supports the idea that GAA supplementation improves protein utilisation in broiler chickens specifically when energy is limited in their diet. Creatinine is a product of metabolic breakdown of phosphocreatinine in skeletal muscle, and its blood level is proportional to muscle mass( Reference Wyss and Kaddurah-Daouk 6 ). Serum concentration of creatinine increased in response to feeding broiler chickens with LME diets with or without 1·2 g/kg supplemental GAA. In this respect, Kubíková et al. ( Reference Kubíková, Výboh and Košťál 37 ) observed an increase in plasma creatinine in response to feed restriction in broiler breeders. Moreover, muscular concentration of creatinine increased after supplementing diets with graded levels of GAA in broiler chickens( Reference Lemme, Ringel and Rostagno 14 ).
In conclusion, feeding diets supplemented with 1·2 g/kg GAA could decrease the adverse effects of dietary energy reduction on performance of broiler chickens across different age periods specifically in early stage of growth. Such a beneficial effect of supplemental GAA cannot be attributed to improved DFI or intestinal digestibility. It seems that enhancement in buffering capacity for ATP in the muscles exerted by supplemental GAA affected the metabolism of energy in broiler chickens fed diets with energy reduction. In this respect, retained energy in carcass increased during 1–15 d of age owing to improved energy utilisation by GAA in LME diets. In addition, effects of dietary energy reduction and GAA inclusion was reflected in blood-related parameters. The growth performance of broilers was more sensitive to NEp of diet than to AME. Additionally, effects of GAA on NEp was more pronounced in diets with lower energy content.
Acknowledgement
Authors greatly acknowledge the Evonik Degussa representative in Iran for supplying GAA.
This research was financially supported by Lorestan University (grant no. 12236-42).
S. S. A. S. F. contributed to the project idea, design and execution of the study. A. A. was in charge of project idea and writing the manuscript. A. G. participated in project implementation and H. K. was involved in manuscript revision.
None of the authors has any conflicts of interest to declare.
Supplementary material
For supplementary material/s referred to in this article, please visit https://doi.10.1017/S0007114517003701