Introduction
A rich assemblage of isolated body fossils and rare associated skeletal remains of small-bodied ornithopods has been recovered from Early Cretaceous rocks of the Australian-Antarctic rift system, strata of which crop out in sea cliffs and wave-cut shore platforms along the southern coast of Victoria, southeastern Australia (Fig. 1.1, 1.2). Four small-bodied ornithopods have been named from this region, including Atlascopcosaurus loadsi Rich and Rich, Reference Rich and Rich1989, Leaellynasaura amicagraphica Rich and Rich, Reference Rich and Rich1989, and Diluvicursor pickeringi Herne et al., Reference Herne, Tait, Weisbecker, Hall, Nair, Cleeland and Salisbury2018, all of which are from the lower Albian of the Eumeralla Formation in the Otway Basin (Fig. 1.2); and Qantassaurus intrepidus Rich and Vickers-Rich, Reference Rich and Vickers-Rich1999 from the Wonthaggi Formation in the Gippsland Basin (Fig. 1.2), which has been considered Valanginian–middle Barremian in age (Wagstaff and McEwen Mason, Reference Wagstaff and McEwen Mason1989). Of these four taxa, Atlascopcosaurus loadsi, L. amicagraphica, and Q. intrepidus are known from craniodental remains, whereas Diluvicursor pickeringi is known from a partial postcranium.

Figure 1. Maps of Australia, southern Victoria and Gondwana: (1) present-day eastern Australia indicating region of interest; (2) inset from (1) showing upper Barremian–lower Albian ornithopod localities and associated geology; (3) reconstruction of Gondwana during the late Barremian (~ 125 Ma) using GPlates (www.gplates.org). Dashed lines in (2) indicate basin boundaries. Geological information in (2) based on Bryan et al. (Reference Bryan, Constantine, Stephens, Ewart, Schon and Parianos1997, Reference Bryan, Ewart, Stephens, Parianos and Downes2000). V-shaped symbols in (3) indicate direction and position of plate subduction, based on Wandres and Bradshaw (Reference Wandres and Bradshaw2005). Australian paleoshoreline in (3) based on Heine et al. (Reference Heine, Yeo and Müller2015). Dashed arrows in (2–3) indicate paleoflow direction. AAR = Australian-Antarctic rift; AF = Africa; AN = Antarctica; AU = Australia; I = India; EF = Eumeralla Formation; ES = epeiric Eromanga Sea (in region of Eromanga Basin); ETRW = Eric the Red West; M = Madagascar; NC = New Caledonia; NZ = New Zealand; SA = South America; VHFT2 = Victorian Hypsilophodontid Femur Type 2; VOPC1 = Victorian ornithopod postcranium 1 (NMV P185992/P185993); VOPC2 = Victorian ornithopod postcranium 2 (NMV P186047); W = Whitsunday Large Siliceous Igneous Province (Bryan et al., Reference Bryan, Constantine, Stephens, Ewart, Schon and Parianos1997); WF = Wonthaggi Formation.
In addition to the named Victorian taxa, several isolated femora from the Eumeralla and Wonthaggi formations were referred to Fulgurotherium australe von Huene, Reference von Huene1932 (Rich and Rich, Reference Rich and Rich1989; Rich and Vickers-Rich, Reference Rich and Vickers-Rich1999), a femoral-based taxon from the Albian Griman Creek Formation in the Lightning Ridge region of northern New South Wales (Molnar and Galton, Reference Molnar and Galton1986). However, Fulgurotherium australe has been reassessed as a nomen dubium (Agnolin et al., Reference Agnolin, Ezcurra, Pais and Salisbury2010). Another femur (NMV P156980) collected from Cape Paterson in the Wonthaggi Formation (Fig. 1.2), approximately double the size of the largest Victorian femora assigned to Fulgurotherium australe (see Rich and Vickers-Rich, Reference Rich and Vickers-Rich1999; Herne, Reference Herne2014), was informally termed ‘Victorian Hypsilophodontid Femur Type 2’ (Rich and Rich, Reference Rich and Rich1989).
Excavated at the Slippery Rock site at the fossil vertebrate locality of Dinosaur Cove (Fig. 1.2), the holotype of Leaellynasaura amicagraphica (NMV P185991) comprises a left-side cheek fragment of a juvenile individual, including the maxilla (Rich and Rich, Reference Rich and Rich1989) but lacks a dentary. A cranial table (NMV P185990) and two partial postcranial specimens (NMV P185992, P185993; confirmed as belonging to a single individual; Herne, Reference Herne2009; Herne et al., Reference Herne, Tait and Salisbury2016, fig. 5) were originally referred to L. amicagraphica, as scattered parts of the holotypic individual (Rich and Rich, Reference Rich and Rich1989; Rich and Vickers-Rich, Reference Rich and Vickers-Rich2000). However, these referrals have also been questioned (Herne et al., Reference Herne, Tait and Salisbury2016). For this reason, the referrals of several isolated femora and another partial postcranium (NMV P186047) to L. amicagraphica from Dinosaur Cove (sensu Rich and Rich, Reference Rich and Rich1989; Rich and Vickers-Rich, Reference Rich and Vickers-Rich1999; Rich et al., Reference Rich, Galton and Vickers-Rich2010) have also been questioned (Herne et al., Reference Herne, Tait and Salisbury2016).
Discovered at the locality of Point Lewis in the Eumeralla Formation (Fig. 1.2), the holotype of Atlascopcosaurus loadsi (NMV P166409) consists of a partial left maxillary fragment. In addition to the Atlascopcosaurus loadsi holotype, another left maxillary fragment from Point Lewis (NMV P157390), as well as a left maxillary fragment (NMV P157970) and several isolated maxillary teeth from Dinosaur Cove, were also referred to the taxon. Two isolated dentary fragments (NMV P182967, P186847) and two isolated dentary teeth (notably NMV P177934) were also referred to Atlascopcosaurus loadsi (Rich and Rich, Reference Rich and Rich1989; see also Herne et al., Reference Herne, Tait and Salisbury2016). However, because none of these Atlascopcosaurus loadsi-referred dentary and dentary tooth specimens were found in association with a maxilla, we consider these assignments inconclusive.
Discovered at the locality of Eric the Red West in the Eumeralla Formation (Fig. 1.2), Diluvicursor pickeringi comprises the holotypic partial hind-region postcranium (NMV P221080) and a referred, isolated caudal vertebra (Herne et al., Reference Herne, Tait, Weisbecker, Hall, Nair, Cleeland and Salisbury2018). With future discoveries, this taxon could be found synonymous with any one of the Victorian taxa previously named from craniodental remains. However, the significance of Diluvicursor pickeringi as a rare, articulated Australian ornithopod skeleton, clearly differing from two other partial postcrania from the Eumeralla Formation (i.e., NMV P185992/P185993, P186047), were considered by Herne et al. (Reference Herne, Tait, Weisbecker, Hall, Nair, Cleeland and Salisbury2018) as justification for erecting the taxon.
Discovered during excavations at the Flat Rocks site in the Wonthaggi Formation (Figs. 1.2, 2), Qantassaurus intrepidus is known from the three isolated dentaries (Rich and Vickers-Rich, Reference Rich and Vickers-Rich1999)—that of the holotype (NMV P199075) and two additional specimens (NMV P198962, P199087). Originally referred to Hypsilophodontidae, the Q. intrepidus dentary was diagnosed by a combination of three features: possession of 10 cheek teeth; foreshortened morphology; and anteriorly convergent dorsal and ventral margins (sensu Rich and Vickers-Rich, Reference Rich and Vickers-Rich1999). Agnolin et al. (Reference Agnolin, Ezcurra, Pais and Salisbury2010) reassessed Q. intrepidus as a nondryomorphan ornithopod, agreeing that the foreshortened dentary with anteriorly convergent alveolar and ventral margins presented a combination of features that distinguished Q. intrepidus from other ornithopods.
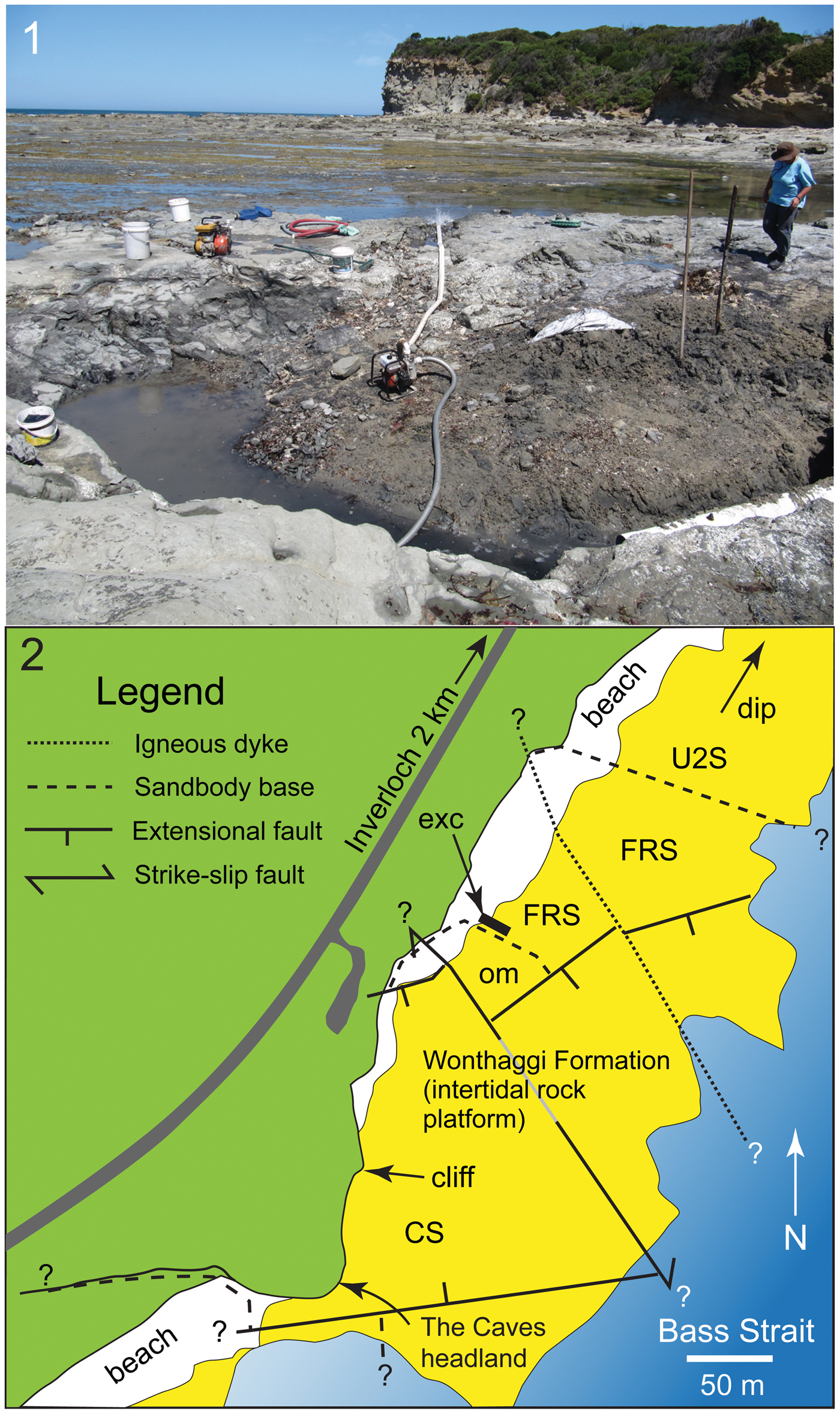
Figure 2. Flat Rocks locality in the Bunurong Marine National Park of the Strzelecki region, Victoria, southeastern Australia: (1) view looking southwest showing the Flat Rocks excavation (foreground), wave eroded rock platform (midground), and The Caves headland (background), which is ~ 230 m southwest of the Flat Rocks excavation; (2) map showing site positions, topographic and geostructural features, and estimated boundaries of sandstone units. ? = unknown extension of feature; CS = The Caves Sandstone; exc = excavations; FRS = Flat Rocks Sandstone; om = overbank mudstone; U2S = Unit 2 Sandstone.
A plethora of isolated body fossils have been collected from the Flat Rocks site, which among the dinosaur materials, some have been identified as ankylosaurian, avian, and nonavian theropodan bones and teeth (Close et al., Reference Close, Vickers-Rich, Trusler, Chiappe, O'Connor, Rich, Kool and Komarower2009; Barrett et al., Reference Barrett, Rich, Vickers-Rich, Tumanova, Inglis, Pickering, Kool and Kear2010; Benson et al., Reference Benson, Rich, Vickers-Rich and Hall2012; see also Poropat et al., Reference Poropat, Martin, Tosolini, Wagstaff, Bean, Kear, Vickers-Rich and Rich2018). However, Qantassaurus intrepidus has been the only ornithopod named from the Wonthaggi Formation. The partial maxilla of an ornithopod (NMV P186440) was further reported by Rich and Vickers-Rich (Reference Rich and Vickers-Rich1999) from a cliffed coastal headland site called The Caves ~200 m from the Flat Rocks excavations (Figs. 1.2, 2). However, Rich and Vickers-Rich (Reference Rich and Vickers-Rich1999) were uncertain whether NMV P186440 was assignable to Atlascopcosaurus loadsi or Q. intrepidus. In this investigation, we describe new craniodental materials of ornithopods from the Flat Rocks locality (=Flat Rocks and The Caves sites), revise Q. intrepidus, reassess the diversity and phylogenetic relationships of the Victorian ornithopods, and update the distribution of Australian ornithopods.
Geological setting
Most of the specimens of interest to this investigation were collected from the Flat Rocks site (=Dinosaur Dreaming Field Site; Seegets-Villiers, Reference Seegets-Villiers2012), which consists of a series of ~1 m deep excavations on the coastal, wave-eroded rock platform within the Bunurong Marine National Park, 2.2 km southwest of the town of Inverloch in Victoria, southeastern Australia (38.660792°S, 145.681009°E, GDA94 [Intergovernmental Committee on Surveying and Mapping, 2014]; Seegets-Villiers, Reference Seegets-Villiers2012), ~112 km southeast of the city of Melbourne (Figs. 1–2). One additional specimen (NMV P186440; reported by Rich and Vickers-Rich, Reference Rich and Vickers-Rich1999) discovered in a large boulder that had fallen from the sea cliff at The Caves (38.662792°S, 145.680108°E, Map Grid of Australia, 1994; see also Kool, Reference Kool2010, p. 60), ~230 m southwest of the Flat Rocks site (Figs. 1.2, 2) is also of interest to this study. The Flat Rocks site is located within the undifferentiated upper section of the Strzelecki Group in the Gippsland Basin (see Tosolini et al., Reference Tosolini, Mcloughlin and Drinnan1999), which has been informally termed the Wonthaggi Formation (Constantine and Holdgate, Reference Constantine and Holdgate1993; see also Chiupka, Reference Chiupka1996), the name used herein (Fig. 1.2).
The predominantly volcanogenic sediments of the Wonthaggi and Eumeralla formations (Fig. 1.2) in the Gippsland and Otway basins, respectively, were deposited during the Early Cretaceous as thick (to 3,000 m) depocenters within the extensional rift valley that formed between Australia and Antarctica, coinciding with the fragmentation of Gondwana (Willcox and Stagg, Reference Willcox and Stagg1990; Bryan et al., Reference Bryan, Constantine, Stephens, Ewart, Schon and Parianos1997; Tosolini et al., Reference Tosolini, Mcloughlin and Drinnan1999; Hall and Keetley, Reference Hall and Keetley2009). The Gippsland and Otway basins, however, are most likely a single basin system (VandenBerg et al., Reference VandenBerg, Cayley, Willman, Morand, Seymon, Osborne, Taylor, Haydon, McLean, Quinn, Jackson and Sandford2006). The sediments were sourced from the Whitsunday Silicic Large Igneous Province (WSLIP) that was situated along the eastern margin of the Australian Plate (Bryan et al., Reference Bryan, Constantine, Stephens, Ewart, Schon and Parianos1997; Bryan, Reference Bryan2007; Fig. 1.3). In addition, quartzose grit and gravel admixtures were sourced from older basement rocks that formed the rift margins (based on Felton, Reference Felton1997). The Wonthaggi Formation has been described as comprising multistorey sheet-flood to braided river-like fluvial channel complexes to 200 m thick, interspersed with overbank sequences up to 100 m thick (Bryan et al., Reference Bryan, Constantine, Stephens, Ewart, Schon and Parianos1997). However, new research (unpublished data, Tait, Hall, and Herne, 2018) further suggests that the Wonthaggi and Eumeralla formations could be the product of a large-scale meandering river system with associated vegetated flood plains.
Sediments at the Flat Rocks site (Fig. 2) comprise interbedded volcaniclastic sandstones and mudstone conglomerates within the basal meter of a fluvial sandbody ~24 m thick (see also Seegets-Villiers, Reference Seegets-Villiers2012). This sandbody is hereafter termed the ‘Flat Rocks Sandstone.’ The erosive base of the Flat Rocks Sandstone has a relief of ~0.5 m at the dig site, cut into thinly interbedded mudstones and very fine-grained sandstones, thin coals, and paleosols with in situ tree stumps deposited on a fluvial floodplain (see also Seegets-Villiers, Reference Seegets-Villiers2012). The fossil-bearing sandstones and conglomerates also contain copious fossilized plant fragments up to small log-sized, which are now coalified and flattened, as well as contemporaneous charcoal (see also Seegets-Villiers, Reference Seegets-Villiers2012). The mudstone clasts range to cobble-size and include pale gray types, lithologically identical to the paleosol mudstones, and pale brown types thought to represent riverbank or abandoned channel deposits. The brown mudstone contains freshwater bivalve shell fossils, including two unionoidean species (Thompson and Stilwell, Reference Thompson and Stilwell2010). The bedding within the sandbody becomes thinner upward and the grain size becomes finer starting from medium to coarse sand at the unit base. Thus, the Flat Rocks Sandstone is potentially a single storey unit deposited by lateral accretion of a meandering river >24 m deep (considering postdepositional compaction). The fossil-bearing sediments were deposited as bedload by high-speed flow at the base of the river, with the alignment of elongate plant fragments and prograding bedforms indicating a local current direction toward ~240° (sensu Seegets-Villiers, Reference Seegets-Villiers2012, fig. 2.12). The boulders at the foot of the cliffed headland site (The Caves; Fig. 2) result from undercutting of the cliff by waves, or from weathering. The sandstone at The Caves could be a down-faulted section of the Flat Rocks Sandstone, although this assessment has yet to be verified. However, for the purposes of this investigation, we informally term the unit in this region ‘The Caves Sandstone’ (Fig. 2).
Detailed taphonomic investigation of the fossil vertebrate remains from the Flat Rocks site was conducted by Seegets-Villiers (Reference Seegets-Villiers2012). The specimens consist of isolated, reworked, multispecific whole bones and bone fragments that accumulated under conditions of in-channel hydraulic flow on low-angle prograding bedforms on the channel floor. Many bones from the locality had been subject to surficial weathering, possibly including heating and charring by fire (Seegets-Villiers, Reference Seegets-Villiers2012). Various degrees of in-channel abrasion suggest the bones differed in periods of transport, with some undergoing multiple stages of reworking (Seegets-Villiers, Reference Seegets-Villiers2012). Thus, the accumulation can be considered time-averaged (e.g., Behrensmeyer, Reference Behrensmeyer1982). Most of the vertebrate fossils in this deposit comprise disassociated bones and bone fragments. However, NMV P186440—collected at The Caves site and reported by Rich and Vickers-Rich (Reference Rich and Vickers-Rich1999, fig. 2) as a maxilla—constitutes an associated cranial fragment, rarely found in Victoria.
Palynological work previously suggested that the region of the Wonthaggi Formation encompassing the Flat Rocks locality was middle Valanginian–middle Barremian in age (following Wagstaff and McEwen Mason, Reference Wagstaff and McEwen Mason1989). However, renewed palynological investigations (personal communication, B. Wagstaff, 2018) suggest that the Flat Rocks locality falls within the upper part of the Foraminisporis wonthaggiensis (Cookson and Dettmann, Reference Cookson and Dettmann1958) spore-pollen zone of Helby et al. (Reference Helby, Morgan and Partridge1987), indicating a late Barremian age (~125–127.2 Ma, following Cohen et al., Reference Cohen, Finney, Gibbard and Fan2013). Using GPlates (v. 2.0.0; www.gplates.org), the position of the Flat Rocks locality at 125 Ma is estimated at ~72°S, 119°E (Fig. 1.3).
Materials and methods
Craniodental remains of ornithischians from the collections of Museums Victoria (NMV) and other comparative materials (Table S1) were examined first-hand in this investigation, from which new assignments were made and the diversity and phylogenetic relationships of the Victorian ornithopods were revised. The specimens were documented using digital photography, vernier callipers, and a microscope-mounted camera-lucida attachment. New NMV specimens were mechanically prepared (by L. Kool, Monash University, and D. Pickering, Museums Victoria). Additional anatomical data and imagery for the maxilla NMV P229196 utilized micro-Computed Tomography (μCT) scans (Zeiss Xradia XRM Versa520 X-Ray Microtomography: voxel size 45.61 µm; power 10 W; voltage 140 kV). The scans were digitally modelled and volume-rendered using Avizo software, v. 9 (FEI, Berlin). The production of figures utilized Adobe Illustrator and Photoshop software, CS4 (www.adobe.com). Nomenclature for the dentition used in the Systematic paleontology section and phylogenetic dataset is outlined in Figure 3 and Table 1. The phylogenetic relationships of the Australian taxa of interest were hypothesized from a cladistic analysis using TNT 1.5 (Goloboff and Catalano, Reference Goloboff and Catalano2016). The Systematic paleontology section follows the phylogenetic framework resulting from the cladistic analysis, and referrals in open nomenclature follow the criteria of Bengston (Reference Bengston1988). The phylogenetic definitions of clades predominantly follow Madzia et al. (Reference Madzia, Boyd and Mazuch2018; see Text S1) and the relative stratigraphic ages of taxa of interest are provided in Text S1.
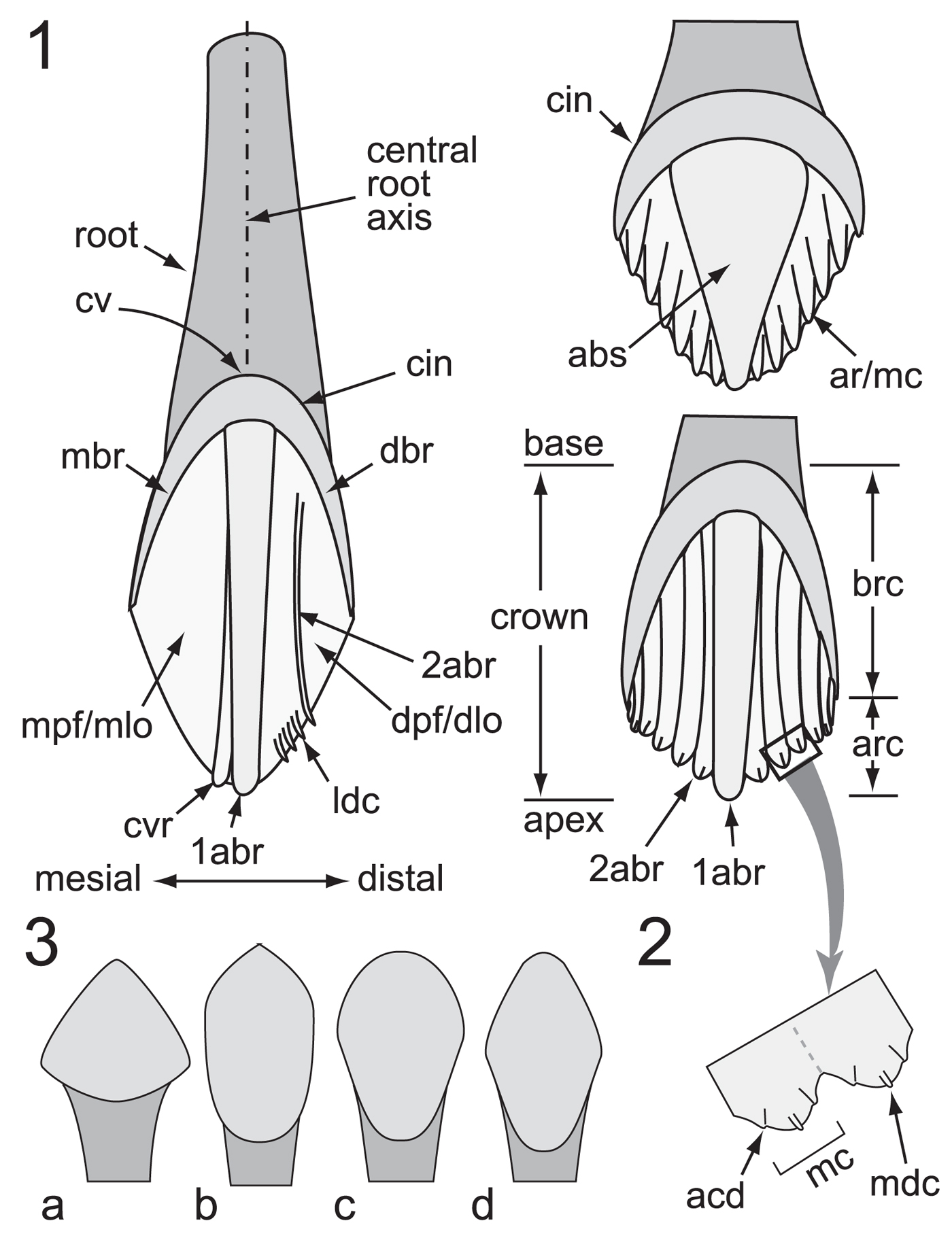
Figure 3. Ornithischian dental nomenclature: (1) crown ornamentation features; (2) portion of crown surface indicated by large arrow in (1), showing location of accessory denticles relative to the median denticle on the apical cusp (typically lingual maxillary and labial dentary faces); (3) variation in crown shape: subtriangular (a), urn-shaped (b), spatulate (c), and rhomboidal/lanceolate (d). 1abr = primary apicobasal ridge; 2abr = secondary apicobasal ridge; abs = apicobasal swelling; acd = accessory denticle; ar = apical ridge; arc = apical region of crown; brc = basal region of crown; cin = cingulum (= dbr + mbr); cv = cingular vertex; cvr = convergent (accessory) ridge; dbr = distal bounding ridge; dlo = distal lobe; dpf = distal paracingular fossa; ldc = lingulate denticle; mbr = mesial bounding ridge; mc = mamillated cusp; mdc = median denticle; mlo = mesial lobe; mpf = mesial paracingular fossa.
Table 1. Terminology used for ornithischian cheek tooth crown descriptions.

Repository and institutional abbreviations
CD = New Zealand Geological Survey Collection, Lower Hutt, New Zealand; CM = Carnegie Museum of Natural History, Pittsburgh, Pennsylvania, USA; MB.R. = Collection of Fossil Reptilia, Museum für Naturkunde, Berlin, Germany; MCF-PVPH = Museo Carmen Funes-Paleontología de Vertebrados, Plaza Huincul, Neuquén Province, Argentina; MUCPv = Museo de Geologia y Paleontologia de la Universidad Nacional del Comahue, Paleontologia de Vertebrados, Neuquén Province, Argentina; NMV = Museums Victoria, Melbourne, Victoria, Australia; NHMUK = The Natural History Museum, London, UK; QM = Queensland Museum, Brisbane, Queensland, Australia; RBINS = Royal Belgian Institute of Natural Sciences, Brussels, Belgium; SAM-PK = South African Museum (Karoo Palaeontology collection), Cape Town, South Africa; UBB = Catedra de Geologie, Facultatea de Biologie şi Geologie, Universitatea din Babes-Bolyai, Cluj-Napoca, Romania; YPM VP = Yale Peabody Museum (Vertebrate Paleontology), New Haven, Connecticut, USA.
Systematic paleontology
Dinosauria Owen, Reference Owen1842
Ornithischia Seeley, Reference Seeley1888
Neornithischia Cooper, Reference Cooper1985
Cerapoda Sereno, Reference Sereno1986
Ornithopoda Marsh, Reference Marsh1881
Genus Galleonosaurus new genus
Type species
Galleonosaurus dorisae n. gen. n. sp., by monotypy.
Diagnosis
As for the type species.
Etymology
From galleon (Latinization of the English for a type of large sailing ship) + saurus (New Latin from the Greek sauros for lizard), in reference to the appearance of the maxilla to the upturned hull of a galleon.
Occurrence
Flat Rocks locality in the Inverloch region of Victoria, southeastern Australia (Fig. 1); Flat Rocks Sandstone and The Caves Sandstone, upper Barremian of the Wonthaggi Formation in the Gippsland Basin.
Remarks
Prior to the recognition of Galleonosaurus n. gen., Atlascopcosaurus loadsi and Leaellynasaura amicagraphica were the only Victorian ornithopods identified from maxillary remains (Rich and Rich, Reference Rich and Rich1989). The maxillae of Atlascopcosaurus loadsi are highly incomplete and the only known maxilla of L. amicagraphica (that of the holotype, NMV P185991) is damaged, and due to its diminutive size, difficult to study. The maxillae of Galleonosaurus n. gen., as well as the complete palatine and fragment of the lacrimal, now provide new information from which the anatomy of the other Victorian ornithopods can be better understood. The holotype of Galleonosaurus dorisae n. gen. n. sp. (NMV P229196) represents the most complete maxilla of a dinosaur currently known from Victoria.
Galleonosaurus dorisae new species
Figures 4–8, 10–13, 15–16, 17.4; Table 2

Figure 4. Specimens of Galleonosaurus dorisae n. gen. n. sp. from the Flat Rocks Sandstone in the upper Barremian, Wonthaggi Formation, Gippsland Basin, southeastern Australia: (1–2) holotype (NMV P229196), left maxilla in lateral (1) and medial (2) views; (3) NMV P208178, left maxilla in lateral view; (4) NMV P212845, left maxilla in lateral view; (5) NMV P209977, left maxilla in lateral view; (6) NMV P186440, left maxilla in lateral view; (7) NMV 208113, right maxillary tooth in labial view. Scale bars = 10 mm (1–6); 1 mm (7).

Figure 5. Galleonosaurus dorisae n. gen. n. sp., digital 3D models of holotypic left maxilla (NMV P229196), derived from μCT scans in dorsal (1), lateral (2), medial (3), ventral (4), posterior (5), and anterior (6) views. Dashed arrow in (1) indicates line of neurovascular tract and posterodorsal foramen. alf = anterolateral fossa; alv = alveolus; aof = antorbital fossa; ap = anterior (premaxillary) process; asr = ascending ramus of maxilla; avp = anteroventral process; bur = buccal ridge; dmt = dorsal maxillary trough; eaof = region of external antorbital fossa; epf = ectopterygoid flange; for = foramen; iaof = region of internal antorbital fossa; js = jugal shelf; lpf = sutural flange for the lateral palatine ramus; m# = maxillary tooth position (from anterior end) and replacement number; mfo = anterior maxillary foramina; ml = medial lamina; mpf = medial palatine facet; mra = maxillary ramus; nuf = nutrient foramen; nvf = neurovascular foramen; plp = posterolateral process; pmp = posteromedial process; rid = ridge (crista); sal = supralveolar lamina; sgj, sutural grove for jugal; vgv = vomer groove. Scale bar increments = 5 mm.

Figure 6. Galleonosaurus dorisae n. gen. n. sp. (NMV P186440), left cranial fragment in dorsal (1), ventral (2), lateral (3), medial (4), medial (5, bottom lighting), and anterior (6) views, and schematics (7–11) of (1–4, 6), respectively. Specimen has been ammonium chloride coated. Dashed arrow in (7) indicates line of neurovascular tract and posterodorsal foramen. alv = alveolus; aof = antorbital fossa; bur = buccal ridge; cem = cementum; cho = choana; epf = ectopterygoid flange; lac = lacrimal; m#’ = maxillary tooth position (from posterior end) and replacement number; mfos = muscular fossa; mgv = medial groove; mra = maxillary ramus; nuf = nutrient foramen; nvf = neurovascular foramen; nvt = neurovascular tract; pfos = pneumatic fossa; plf = palatine lateral flange; plp = posterolateral process; plr = palatine lateral ramus; pmal = palatine medial ala; pmf = palatine maxillary flange; pmp = posteromedial process; sal = supralveolar lamina; sgj = sutural grove for jugal; sul = sulcus on lacrimal fragment. Scale bars = 10 mm.

Figure 7. Galleonosaurus dorisae n. gen. n. sp. (NMV P209977), left maxillae and schematics in ventral (1), dorsal (2), lateral (3), and medial (4) views. Dashed lines in (2) indicate approximate margins of the palatine facet. alf = anterolateral fossa; alv = alveolus; aof = antorbital fossa; ap = anterior (premaxillary) process; asr = ascending ramus of maxilla; avp = anteroventral process; bur = buccal ridge; dalv = developing alveolus; dmt = dorsal maxillary trough; epf = ectopterygoid flange; gv = groove; iaof = region of internal antorbital fossa; lpf = sutural flange for the lateral palatine ramus; lprf = facet for the lateral ramus of the palatine; m# = maxillary tooth position (from anterior end) and replacement number; ml = medial lamina; mpf = medial palatine facet; mra = maxillary ramus; nuf = nutrient foramen; nvf = neurovascular foramen; nvt = neurovascular tract; plp = posterolateral process; pmp = posteromedial process; pro = protuberance; sal = supralveolar lamina; sep = septum; sgj = sutural grove for jugal; sl = slot; sml = sutural margin for lacrimal; vgv = vomer groove. Scale bars = 10 mm.

Figure 8. Galleonosaurus dorisae n. gen. n. sp. (NMV P212845), left maxillae and schematics in ventral (1), dorsal (2, 3), dorsolateral (4), lateral (5), and medial (6) views. Specimen in (3) has been ammonium chloride coated; dashed line in schematic indicates presumed internal path of the neurovascular tract. alf = anterolateral fossa; alv = alveolus; aof = antorbital fossa; ap = anterior (premaxillary) process; asr = ascending ramus of maxilla; avp = anteroventral process; bur = buccal ridge; dmt = dorsal maxillary trough; epf = ectopterygoid flange; iaof = region of internal antorbital fossa; lpf = sutural flange for the lateral palatine ramus; lprf = facet for the lateral ramus of the palatine; m# = maxillary tooth position (from anterior end) and replacement number; ml = medial lamina; mra = maxillary ramus; nuf = nutrient foramen; nvf = neurovascular foramen; nvt = neurovascular tract; plp = posterolateral process; sal = supralveolar lamina; sep = septum; sgj = sutural grove for jugal; vgv = vomer groove. Scale bar = 10 mm.

Figure 9. Camptosaurus dispar Marsh, Reference Marsh1879, left maxilla and schematics (YPM VP 1886) in dorsal (1) and ventral (2) views. Dashed arrow in (1) indicates presumed dorsal path of the neurovascular tract; dashed lines indicate approximate margins of the palatine facet. alv = alveolus; aof = antorbital fossa; ap = anterior (premaxillary) process; asr = ascending ramus; avp = anteroventral process; bur = buccal ridge; epf = ectopterygoid flange; js = jugal shelf; lprf = sutural flange for the lateral palatine ramus; m# = maxillary tooth position (from anterior end) and replacement number; ml = medial lamina; mpf = medial palatine facet; mra = maxillary ramus; nuf = nutrient foramen; plp = posterolateral process; pmg = premaxillary groove; pmp = posteromedial process; pro = protuberance; rid = ridge; sal = supralveolar lamina; sut = sutural margin. Scale bars = 50 mm. Image (1) by A. Heimer, courtesy of YPM. Image (2) by S. Hochgraf, courtesy of YPM.

Figure 10. Comparisons of Galleonosaurus dorisae n. gen. n. sp.: (1–4) schematics of holotype of G. dorisae n. gen. n. sp. (NMV P229196) (1), G. dorisae n. gen. n. sp. (NMV P209977) (2), Atlascopcosaurus loadsi Rich and Rich, Reference Rich and Rich1989 (NMV P157390) (3), and original Atlascopcosaurus loadsi holotype (NMV P166409) (4), posterior maxillary regions in ventral view, showing extent of the posteromedial processes; (5) schematic of holotypic maxilla of Leaellynasaura amicagraphica Rich and Rich, Reference Rich and Rich1989 (NMV P185991) in ventral view showing shapes of the alveolar and posterior margins; (6, 7) illustration of G. dorisae n. gen. n. sp. (NMV P186440) (6) and cast of the original Atlascopcosaurus loadsi holotype (NMV P166409) (7) in dorsal view, with schematics showing difference in the shape of the lateral palatine rami/sutural facets. alv = alveolus; aof = antorbital fossa; bur = buccal ridge; cho = choana; epf = ectopterygoid flange of maxilla; lpf = lateral palatine flange on maxilla; lprf = facet for the lateral ramus of the palatine; m# = maxillary tooth position (from anterior end) and replacement number; m#’ = maxillary tooth position (from posterior end) and replacement number; mfos = muscular fossa on palatine; mpal = medial palatine ala; mpf = facet for medial palatine flange on maxilla; mra = maxillary ramus; nvt = neurovascular tract; pfos = pneumatic fossa on palatine; plp = posterolateral process; plf = palatine lateral flange; plr = palatine lateral ramus; pmp = posteromedial process; sal = supralveolar lamina; sgj = sutural grove for jugal. Scale bars = 10 mm.

Figure 11. Internal anatomy of the Galleonosaurus dorisae n. gen. n. sp. holotypic left maxilla (NMV P229196) from μCT scans: (1) antorbital region in dorsal view; (2) maxilla in lateral view with schematic overlay showing internal locations of the antorbital fossa (in green; ventral extent, cross-hatching) and neurovascular tract (in blue); (3) coronal sections through maxilla as indicated in (2). alv = alveolus; aof = antorbital fossa; asr = ascending ramus of maxilla; brk = breakage; bur = buccal ridge; cem = cementum; dmt = dorsal maxillary trough; for = foramen; iaof = region of internal antorbital fenestra; lpf = lateral palatine facet and flange on maxilla; m# = maxillary tooth position (from anterior end) and replacement number; mfo# = anterior maxillary foramen (1 = dorsal branch; 2 = ventral branch); ml = medial lamina; mra = maxillary ramus; nvt = neurovascular tract; nvt? = uncertain dorsal moity of neurovascular tract; pdf = posterodorsal foramen of the maxilla; sal = supralveolar lamina; sep = septum; sgj = sutural groove for jugal; sgl = sutural groove for lacrimal; slt = slot. Scale bars: 10 mm (1, 2); 5 mm (3).

Figure 12. Galleonosaurus dorisae n. gen. n. sp., holotypic left maxilla (NMV P229196) in dorsal view and schematic showing broken surface (dark shading) of the ascending ramus and separation of the supralveolar and medial laminae indicated by a seam of sediment infill. aof = antorbital fossa; asr = ascending ramus of maxilla; fos = fossa; ml = medial lamina of maxilla; mra = maxillary ramus; sed = host sediment infill; sal = supralveolar lamina. Scale bar = 10 mm.

Figure 13. Comparisons of Victorian ornithopod maxillae in lateral view and schematics, showing dorsalmost extent of the maxillary ramus (indicated by large arrows): (1) Galleonosaurus dorisae n. gen. n sp., holotypic left maxilla (NMV P229196); (2) Atlascopcosaurus loadsi Rich and Rich, Reference Rich and Rich1989, left maxilla (NMV P157390); (3) cast of original holotypic left maxilla of Atlascopcosaurus loadsi (NMV P166409). alv = alveolus; bur = buccal ridge; lpf = lateral palatine flange of maxilla; m#’ = maxillary tooth position (from posterior end) and replacement number; ml = medial lamina of maxilla; mra = maxillary ramus; nvt = neurovascular tract; plp = posterolateral process of maxilla; pmp = posteromedial process of maxilla; sal = supralveolar lamina of maxilla. Scale bars = 10 mm.

Figure 14. Hypsilophodon foxii Huxley, Reference Huxley1870, partial right cranium (NHMUK R2477) and schematics in oblique posterodorsal (1) and medioventral (2) views. Cross-hatching in (1) indicates missing supralveolar lamina bordering the antorbital fenestra; dashed arrow indicates entrance of the posterodorsal foramen of the neurovascular tract. aiaof = anterior (accessory) internal antorbital fenestra; aof = antorbital fossa; ap = anterior process of maxilla; asr = ascending ramus of maxilla; cem = cementum; dmt = dorsal maxillary trough; ept = ectopterygoid; iaof = internal antorbital fenestra; j = jugal; lac = lacrimal; mfos = muscular fossa of palatine; ml = medial maxillary lamina; mra = maxillary ramus; nld = nasolacrimal duct; nvf = neurovascular foramina; nvt = neurovascular tract; ped = pedestal; plr = palatine lateral ramus; pm = premaxilla; pmal = palatine medial ala; pmf = palatine maxillary flange; ppt = pterygoid process of the palatine; sal = supralveolar lamina. Scale bar = 10 mm (2; for scale in 1, refer to 2).

Figure 15. Maxillary dentition of Galleonosaurus dorisae n. gen. n. sp.: (1, 2) holotypic left dentition (NMV P229196) in labial (1) and lingual (2) views; (3) enlargement of maxillary tooth (m7.2) in (2); (4) left dentition of NMV P186440 in labial view. 1abr = primary apicobasal ridge; 2abr = secondary apicobasal ridge; 3abr = tertiary apicobasal ridge; abr = apicobasal ridge (see definition, Table 1); acd = accessory denticle; cv = cingular vertex; dbr = distal bounding ridge; dpf = distal paracingular fossa; gch = growth channel/fossa; m# = maxillary tooth position (from anterior end) and replacement number; m#’ = maxillary tooth position (from posterior end) and replacement number; mbr = mesial bounding ridge; mdc = median denticle; ocf = occlusal facet. Scale bars: 5 mm (1, 2, 4); 1 mm (3).

Figure 16. Maxillary dentition of Galleonosaurus dorisae n. gen. n. sp., digital 3D model of holotypic maxillary tooth m8 (NMV P229196) derived from μCT scans in labial (1), dorsal (2), apical (3), and lingual (4) views. Larege arrow in (4) indicates sinuous bend on the lingual margin of the root. 1abr = primary apicobasal ridge; alv = alveolus; cr = crown; cv = cingular vertex; dbr = distal bounding ridge; gch = growth channel/fossa; mra = maxillary ramus; ocf = occlusal facet; rt = root. Scale bar = 5 mm.

Figure 17. Comparisons of Australian ornithopod maxillary tooth crowns in left labial view: (1, 2) Atlascopcosaurus loadsi Rich and Rich, Reference Rich and Rich1989 (NMV P157390), m5’ (counted from posterior end) and schematic (2); (3) Victorian ornithopodan maxillary morphotype 4 (cf. Atlascopcosaurus loadsi; NMV P208133), schematic of right m3’ (reversed; counted from posterior end); (4) Galleonosaurus dorisae n. gen. n. sp., schematic of holotypic (NMV P229196) m8; (5, 6) Leaellynasaura amicagraphica Rich and Rich, Reference Rich and Rich1989, holotypic (NMV P185991) m5–m12 with schematic of m9 (6); (7) Muttaburrasaurus sp. (QM F14921), schematic of left ~m4’ (counted from posterior end). 1abr = primary apicobasal ridge; 2abr = secondary apicobasal ridge; cv = cingular vertex; cvr = convergent (secondary) ridge; dbr = distal bounding ridge; ldc = lingulate denticle; mbr = mesial bounding ridge; rt = root. Scale bars: 10 mm (7); 5 mm (5); 1 mm (1–4, 6).
Holotype
NMV P229196, a complete left maxilla with partial dentition.
Table 2. Measurements (in mm) of Victorian ornithopod maxillary specimens. APL1 = anteroposterior length of maxillary ramus (without anterior process); APL2 = anteroposterior length of the dentulous maxillary portion (extent of alveoli); APL3 = anteroposterior length of anterior (premaxillary) process; DVDR = greatest dorsoventral depth of maxillary ramus; TVW1 = greatest transverse width of maxillary ramus; TVW2 = transverse width of ramus at fifth alveolus; TVW3 = narrowest transverse width of ramus; + = measurement incomplete. In incomplete specimens (NMV P166409, P157390, P208133), the alveoli are counted from the posterior end of the ramus.

Diagnosis
Small-bodied, noniguanodontian ornithopod characterized by five potential autapomorphies: (1) ascending ramus of maxilla has two slot-like foramina on the anterior margin that communicate with the neurovascular tract; (2) neurovascular tract bifurcates internally to exit at two anteroventral maxillary foramina; (3) lingual margin of maxillary tooth roots in midregion of tooth row form an S-bend at their bases; (4) posterior third of maxilla on some, but not all, specimens deflects posterolaterally at an abrupt kink; and (5) lateral end of palatine lateral ramus forms a hatchet-shaped flange.
Occurrence
Flat Rocks locality in the Inverloch region of Victoria, southeastern Australia (Fig. 1); Flat Rocks Sandstone and The Caves Sandstone, upper Barremian of the Wonthaggi Formation in the Gippsland Basin.
Description
The taxon is known from five isolated left maxillae with dentition, an isolated right maxillary tooth, the palatine, and a partial lacrimal. The most complete of the maxillae, the holotype (NMV P229196), retains four fully erupted teeth (Figs. 4, 5). Marginally larger than the holotype but incomplete, NMV P186440 (Figs. 4, 6) preserves the posterior region of the maxilla with six tooth positions (five crowns fully erupted), the complete left palatine, and a ventrolateral fragment of the lacrimal, preserved in situ. NMV P208178 is ~73% of the holotype in size and retains five erupted crowns (Fig. 4). The two smallest maxillae (NMV P209977, P212845) lack erupted dentition (Figs. 4, 7, 8). Because the complete lacrimal is presently unknown, the anteroposterior and dorsoventral extent of the antorbital fossa and external antorbital fenestra are also unknown.
Maxilla
Two maxillary forms are apparent. On NMV P229196 (the holotype), P208178, and P212845, an abrupt kink causes the posterior third of these maxillae to angle posterolaterally outward (Figs. 5, 8). This kink coincides with a bulge on the medial surface at the anterior end of the facet for the palatine, and resembles the kink on the maxilla of Camptosaurus dispar Marsh, Reference Marsh1879 (YPM VP 1886; Fig. 9). However, in the latter taxon, deflection is relatively less. On one of the smallest maxillae (NMV P209977; Fig. 7.1, 7.2), the kink is absent and could also be absent on the largest maxilla (NMV P186440; Fig. 6.1, 6.2, 6.7, 6.8), although noting that the latter specimen is incomplete anteriorly (see further comments under Variation, below). The largest complete maxillae (NMV P229196, P208178) each contain 15 alveoli, and the smallest maxillae (NMV P209977, NMV P212845) contain 13 and 14 alveoli, respectively. Viewed ventrally, the maxillary teeth are arranged en echelon, with one replacement crown present per alveolus (Figs. 5, 6). Staggered tooth replacement occurs across groups of two tooth families. The tooth row is laterally concave with the alveoli in the middle of the tooth row obliquely angled relative to the anteroposterior tooth row axis. The anterior alveoli outturn laterally relative to the lateral margin of the maxilla (Fig. 5), as in Camptosaurus dispar (YPM VP 1886; Fig. 9) and Dysalotosaurus lettowvorbecki Pompeckj, Reference Pompeckj1920 (Janensch, Reference Janensch1955, table 11), and similar to these taxa, no substantial diastema is developed. As a result, the anterior alveolus locates in the anteroventral process that abuts the posterolateral end of the premaxilla (Figs. 5, 7, 8). In Leaellynasaura amicagraphica, the anterior alveoli are oriented parallel to the lateral margin of the maxilla (Fig. 10.5).
The ventral region of an anteroposteriorly extensive antorbital fossa is formed by the maxilla (Figs. 5–8, 11, 12). The supralveolar lamina forms the lateral wall of the maxilla and the buccal ridge formed by this lamina is shallowly rounded dorsoventrally (Figs. 5.6, 6.11, 7, 8, 11, 12). Buccal emargination, measured midway along the tooth row in ventral view, approximately equals the labiolingual width of one crown on the holotype (Fig. 5) and two crowns on the smallest maxillae (NMV P209977, P212845; Figs. 7, 8). Buccal emargination on the maxilla of Leaellynasaura amicagraphica is shallower (approximately two-thirds of the crown width at the deepest point along the tooth row; Fig. 10.5). Similarly to L. amicagraphica, the buccal ridge is protrusive in Atlascopcosaurus loadsi, however, buccal emargination is at least as deep as in Galleonosaurus dorisae n. gen. n. sp. or possibly deeper (Fig. 10).
Viewed laterally (Figs. 4–8), the alveolar margin is shallowly concave and the anterior margin of the ascending ramus is convex. The premaxillary process is spinose and slightly inset medially from the lateral surface of the maxilla by the anteroventral process at the base of the ascending ramus (Figs. 5, 7, 8). A groove on the medial margin of the premaxillary process could have accommodated the vomer. A shallow cleft-like fossa containing neurovascular foramina is present anteriorly on the ventrolateral margin of the maxilla, dorsal to the anteroventral process (Figs. 5, 7, 8, 11), as in Changchunsaurus parvus Zan et al., Reference Zan, Chen, Jin and Li2005 (Jin et al., Reference Jin, Chen, Zan, Butler and Godefroit2010), Jeholosaurus shangyuanensis Xu, Wang, and You, Reference Xu, Wang and You2000 (Barrett and Han, Reference Barrett and Han2009), Tenontosaurus tilletti Ostrom, Reference Ostrom1970 (Thomas, Reference Thomas2015, fig. 2) and Zalmoxes robustus Nopcsa, Reference Nopcsa1900 (NHMUK R3395; unpublished data, Herne, 2009). A fossa on the anterolateral margin of the maxilla has been considered an ornithopodan synapomorphy (Butler et al., Reference Butler, Upchurch and Norman2008). Along the anterior margin of the ascending ramus, the medial lamina forms a thin, buttress-like crista with a straight anterodorsal edge connecting the dorsal edge of the premaxillary process (Figs. 5, 7). A fortuitous break through the ascending ramus on the holotype (during its preparation) indicated that the ascending ramus is formed from both the thicker supralveolar and far thinner medial laminae (Fig. 12), as in Lesothosaurus diagnosticus Galton, Reference Galton1978 (Porro et al., Reference Porro, Witmer and Barrett2015). A thin seam of sediment infill indicates that these two laminae are unfused. In places, the medial lamina is <150 µm thick and its medial surface in the region of the ascending ramus is roughened, forming a shallow fossa (Figs. 5, 12). The external antorbital fenestra is bordered anteriorly by the ascending ramus (Figs. 5, 12). Although the full form of the external antorbital fenestra cannot be assessed, because the ventral margin formed by the supralveolar lamina is degraded on all of the specimens, its ventral margin is positioned dorsally, well above the buccal ridge.
Medially (Fig. 6), the maxillary ramus is differentiated from the alveolar parapet by a medial groove, along which elongate nutrient foramina (‘special foramina’ of Edmund, Reference Edmund1957) align with the alveoli. The developing crowns and roots are encased in cementum (suggested by grayer contrast in the μCT imagery; Fig. 11). The dorsal surface of the maxillary ramus is penetrated by the alveoli (Figs. 5–8, 10, 11), as in Zalmoxes robustus, which was previously considered unique in that taxon (Weishampel et al., Reference Weishampel, Jianu, Csiki and Norman2003).
Viewed dorsoventrally, the posterior margin of the ectopterygoid flange is straight to shallowly concave and oriented orthogonally to the axis of the tooth row (Figs. 5–7). A knob- to spike-like posteromedial process projects from the posteromedial corner of the flange. The posteromedial process is separate from the maxillary ramus, which might also occur in Camptosaurus dispar (YPM VP 1886; Fig. 9). We are presently uncertain whether a separate posteromedial process is commonly developed in other taxa. The posterolateral process is weakly developed, as in Leaellynasaura amicagraphica, and contrasts with that of Atlascopcosaurus loadsi, which is more pronounced (Fig. 10). The dorsal surface of the ectopterygoid flange is striated and horizontal in posterior view (Fig. 5). Viewed medially, the dorsal surface of the maxillary ramus is convex and dorsoventrally deepest roughly midway along the ramus (Figs. 5, 7, 8). A deeply striated sutural surface for the medial flange of the palatine is developed posterior to the medial bulge that coincides with the anterior end of the medial palatine facet (Figs. 5, 7). A slotted sutural facet for the jugal and lacrimal is developed dorsally on the posterolateral edge of the maxilla (Figs. 5–8, 10, 11). Viewed laterally, the jugolacrimal margin is sinuous (Figs. 5, 6). The overall line of these margins slopes posteroventrally at ~30° relative to the alveolar margin, as in Atlascopcosaurus loadsi (Fig. 13) and L. amicagraphica (see Herne, Reference Herne2014, fig. 5.3). This margin is more steeply angled in Hypsilophodon foxii Huxley, Reference Huxley1870 (Galton, Reference Galton1974, figs. 2–3) and the anterior end of the sutural margin for the jugal on the maxilla of Gasparinisaura cincosaltensis Coria and Salgado, Reference Coria and Salgado1996 is distinctly stepped (Coria and Salgado, Reference Coria and Salgado1996, fig. 2).
The dorsal and internal structures of the maxilla are complex. Micro-CT imagery (Fig. 11) reveals regions of the antorbital fossa hidden by the matrix and the internal passage of the neurovascular system. The neurovascular tract (= neurovascular canal of Witmer, Reference Witmer, Currie and Padian1997; maxillary canal of Thomas, Reference Thomas2015) extends the length of the maxillary ramus (Fig. 11.2) and would have conveyed the maxillary division of the trigeminal nerve (cn V2; see Witmer, Reference Witmer1995, Reference Witmer, Currie and Padian1997; Benoit et al., Reference Benoit, Manger and Rubidge2016; Barker et al., Reference Barker, Naish, Newham, Katsamenis and Dyke2017). Posteriorly, the neurovascular tract forms a shallow channel on the dorsal surface of the maxilla medial to the sutural groove for the jugal (Figs. 5–8, 11). The neurovascular tract enters the internal region of the maxilla at the posterodorsal foramen (Figs. 5.1, 6.7, 11.1), which is roofed dorsally by a rugose flange on the maxilla for the lateral ramus of the palatine (Figs. 5–8; 11.2, 11.3, coronal section A). A slot opening dorsally from the neurovascular tract surrounds the lateral and anterior margins of this flange (Fig. 11.1; 11.2, 11.3, coronal sections A, B).
The antorbital fossa is walled laterally and ventrally by the supralveolar lamina and medially by the medial lamina (Figs. 7, 8, 11). The posterior region of the antorbital fossa is located anterior to the flange for the lateral ramus of the palatine and lateral to the internal antorbital fenestra (Fig. 11.1, 11.2; 11.2, 11.3, coronal sections B, C). In this posterior region of the antorbital fossa, the neurovascular tract forms an internalized duct separated from the antorbital fossa by a thin septum (Fig. 11.3, coronal section C). This septum thickens in the midregion of the antorbital fossa and the neurovascular tract appears to divide into dorsal and ventral moieties (Fig. 11.3, coronal section D). In the anteriormost region of the antorbital fossa, the neurovascular tract and the antorbital fossa merge (Fig. 11.2, 11.3, coronal sections E, F). The neurovascular tract forms a dorsally opening channel on the ventral floor of the antorbital fossa. The anteriormost end of the antorbital fossa terminates at the ascending ramus (Fig. 11.1, 11.2). From this point, the neurovascular tract continues anteriorly and bifurcates to exit at two anterior maxillary foramina within a shallow anterolateral fossa, dorsal to the anteroventral process (Figs. 5, 7, 8; 11.2, 11.3, coronal sections G, H). The neurovascular tract is separated medially from the alveoli by a septum through which foramina pass (e.g., Fig. 11.3, coronal sections B, H).
Two slot-like foramina penetrate the anterolateral margin of the ascending ramus and extend posteroventrally to communicate with the merged region of the neurovascular tract and the anteriormost region of the antorbital fossa (Fig. 11.2, 11.3, between coronal sections F–H). These slot-like foramina are walled medially by the medial lamina, which in this region is exceedingly thin (<100 µm). A small foramen that exits laterally on the ascending ramus communicates with the uppermost of the two slot-like foramina (Fig. 11.2, 11.3, coronal section G). A further small foramen extends from the anterior end of the antorbital fossa dorsally to the neurovascular tract to exit laterally on the ascending ramus (Fig. 11.2, 11.3, between coronal sections F, G). Apart from the aforementioned foramina of the neurovascular tract, ~13 additional neurovascular foramina penetrate the supralveolar lamina to communicate with the neurovascular tract. The ventralmost of these foramina (Fig. 11.2, 11.3, between coronal sections B–F) pass ventrally to the antorbital fossa to communicate directly with the neurovascular tract.
The dorsal maxillary trough extends anteriorly on the dorsal surface of the maxilla, from the region of the internal antorbital fenestra and onto the dorsal surface of the premaxillary process (Figs. 5, 7, 8; 11.2, 11.3, coronal sections D–H). The antorbital fossa and dorsal maxillary trough are separated by the medial lamina. The central portion of the medial lamina extends dorsally as a thin sheet of bone (~1.2 mm thick). Sutural striae on the medial surface of the lamina suggest the region of contact with the medial lamina of the lacrimal, as in Leaellynasaura amicagraphica (see Herne, Reference Herne2014, fig. 5.8). Viewed dorsally (Fig. 5), the medial lamina on the holotype bows medially at its midpoint, partly encroaching on the dorsal maxillary trough. The degree of medial bowing is greater in the smallest maxillae (NMV P209977, P212845; Figs. 7, 8), which in this aspect, approaches the condition in Camptosaurus dispar (YPM VP 1886: Fig. 9). However, bowing of the medial lamina in the latter taxon is greater and more posteriorly positioned. A gap in the medial lamina between the anterior ascending ramus and its central region (Fig. 5) could indicate the presence of an anterior internal antorbital fenestra (‘promaxillary fenestra’ of Carpenter, Reference Carpenter, Mateer and Chen1992; Witmer, Reference Witmer, Currie and Padian1997) as in Heterodontosaurus tucki Crompton and Charig, Reference Crompton and Charig1962 (Norman et al., Reference Norman, Crompton, Butler, Porro and Charig2011) and Hypsilophodon foxii (see Galton, Reference Galton1974), or alternatively, could have resulted from breakage.
Palatine
Viewed dorsoventrally (Fig. 6), the ala of the palatine forms a reniform, posteroventrally sloping sheet of bone that projects medially from the medial maxillary flange, which tightly adjoins the maxilla. Viewed medially, the maxillary flange has a boot-shaped profile (Fig. 6.4, 6.10). The medial ala slopes posteroventrally and fails to rise dorsally above the level of the maxillary ramus. Viewed anteriorly, the medial ala is horizontally oriented (Fig. 6.6, 6.11), as in Leaellynasaura amicagraphica (see Herne, Reference Herne2014, figs 5.6, 5.7) and differs from the angled to subvertical orientation of the alae in Muttaburrasaurus langdoni Bartholomai and Molnar, Reference Bartholomai and Molnar1981 (unpublished data, Herne, 2018), Hypsilophodon foxii (Fig. 14), styracosternans, Tenontosaurus tilletti (see Thomas, Reference Thomas2015), and Thescelosaurus neglectus Gilmore, Reference Gilmore1913 (Boyd, Reference Boyd2014). Viewed dorsally, the choanal margin is anterolaterally concave and the posterolateral margin of the flange in contact with the maxilla is laterally concave (Fig. 6.1, 6.7). The choana coincides with the internal antorbital fenestra, as in other ornithopods (Witmer, Reference Witmer, Currie and Padian1997). A transverse ridge, posterior to the choana (‘postchoanal strut’ of Witmer, Reference Witmer, Currie and Padian1997), crosses the dorsal surface of the palatine body (Fig. 6.1, 6.7). A deep muscular fossa is developed posterior to the strut, as in H. foxii (Fig. 14), and a shallower pneumatic fossa is developed anterior to the strut (see Witmer, Reference Witmer, Currie and Padian1997), as in Lesothosaurus diagnosticus (see Porro et al., Reference Porro, Witmer and Barrett2015, fig. 8A, D). The pneumatic fossa is reportedly absent in Heterodontosaurus tucki (see Norman et al., Reference Norman, Crompton, Butler, Porro and Charig2011) and among ornithopods, also absent in at least H. foxii and Iguanodon bernissartensis Boulenger, Reference Boulenger1881 (Witmer, Reference Witmer, Currie and Padian1997), Tenontosaurus tilletti (see Thomas, Reference Thomas2015, fig. 14), and the hadrosaurid Edmontosaurus regalis Lambe, Reference Lambe1917 (Heaton, Reference Heaton1972, figs. 2, 5). A strap-like lateral ramus extends over the convex dorsal surface of the maxilla and a hatchet-shaped, dorsoventrally compressed flange is developed at the end of the lateral ramus. This flange is accommodated in the sutural flange on the maxilla (Figs. 5–8, 11.1; 11.3, coronal section A), coinciding with the medial face of the lacrimal (see below), and most likely the anteriormost end of the jugal.
Lacrimal
A small, plate-like fragment of bone adjoining the maxilla in NMV P186440 (Fig. 6) is interpreted as the posteroventral portion of the left lacrimal. The irregular ventral edge of the fragment locates in the sutural slot on the maxilla anterior to the margin for the jugal. The lacrimal slightly overlaps the sutural edge laterally on the maxilla. The anterolateral surface of the lacrimal is slightly scalloped.
Maxillary dentition
The crowns and roots have en echelon emplacement relative to the long axis of the tooth row and the erupted crowns are imbricated (Figs. 5, 6, 15), as in all ornithischians (e.g., Porro et al., Reference Porro, Butler, Barrett, Moore-Fay and Abel2011, Reference Porro, Witmer and Barrett2015). Viewed labially, the distal margins of the crowns mostly overlap the mesial margins of the adjacent crowns. This pattern of overlap is reversed on some crowns (Fig. 15). The roots taper toward their distal ends and are roughly ovoid in section (Fig. 16). The axis of the root is straight. However, the lingual margin of the root on the teeth in the middle of the tooth row forms a marked S-shaped bend extending dorsally from its base at the cingulum (Fig. 16.4). As a result, mesiolingual and distolingual fossae are formed that accommodate developing crowns of the abutting tooth families. Twisting of the root shaft also aligns the broad mesial and distal surfaces of the roots with the oblong, obliquely angled alveoli (Fig. 5.4). The resorption facets on the roots follow the profile of the apical margins of the successively developing crowns (Fig. 15). At full-length, the roots reach the dorsal surface of the maxillary ramus (Fig. 16). Tooth replacement is posterior to anterior with a Zahnreihe spacing (‘Z-spacing,’ sensu Edmund, Reference Edmund1960) of 1.65 suggested (calculated at M7–M8 on the holotype; based on methods of Osborn, Reference Osborn1975, fig. 1).
The largest maxillary crowns are in the posterior region of the tooth row. However, the two posteriormost teeth are reduced (Fig. 17), as in most ornithopods. At M8 on the holotype, the ratio of apicobasal depth/mesiodistal width is 1.8 (Fig. 17.4), noting that that the unworn depth of the crown would have been greater. The unworn crowns are spatulate and asymmetrical in labiolingual profile. Wear facets on the worn (working) crowns form a continuous occlusal surface, which slopes at ~ 45° to the root axis, in mesiodistal view (Figs. 5; 11.3, coronal section D; 15). Labially and lingually, the basal region forms a deep V-shaped cingular vertex, offset mesially relative to the central axis of the root (Figs. 15–17). Lingually, the cingulum forms a smooth base lacking bounding ridges. Labially, the cingulum forms the mesial and distal bounding ridges (Figs. 15–17). The mesial bounding ridge is straight and vertically oriented. The distal bounding ridge is longer than the mesial, obliquely sloping from the vertex and arcuate toward its apical end. The primary ridge, developed labially, is arcuate (mesially concave/distally convex), strongly offset to the distal third of crown surface, and merges with the distal bounding ridge, distal to the cingular vertex (Figs. 15–17). On many of the crowns, a shallow labial furrow is formed at the point of confluence with the primary ridge, as in Leaellynasaura amicagraphica (Fig. 17). On some crowns, the primary ridge is slightly undercut by the narrow distal paracingular fossa, as in Atlascopcosaurus loadsi and Muttaburrasaurus sp. (QM F14921). Labially, the secondary apicobasal ridges are closely abutting. Four stronger ridges are developed mesial to the primary ridge (Figs. 15–17). The ridge closest to the primary ridge is convergent with the latter. Two finer secondary ridges are developed in the paracingular fossa distal to the primary ridge (Figs. 15–17). These distal ridges merge with the inside margin of the mesial bounding ridge. Tertiary ridges are additionally developed on the surface of the primary and secondary ridges (Fig. 15.1), as in Mantellisaurus atherfieldensis (Hooley, Reference Hooley1925) (Norman, Reference Norman1986). Narrow apicobasal ridges, separated by channels, are developed on the lingual crown surfaces (Fig. 15). These ridges merge with the smooth crown base. The distal ends of all secondary ridges terminate apically in a transversely oriented, blade-like denticle. The stronger, mesial secondary ridges additionally form tridenticulate mamillated cusps (Fig. 15.3), as in Nanosaurus agilis Marsh, Reference Marsh1877 (Bakker et al., Reference Bakker, Galton, Siegwarth and Filla1990; Carpenter and Galton, Reference Carpenter and Galton2018).
Variation
Among the four maxillae with complete alveoli, the number of alveoli varies (Figs. 4, 5, 7, 8). The holotype (NMV P229196) and midsized maxilla (NMV P208178) each have 15 alveoli, and the smaller maxillae (NMV P209977, P212845) each have 13 and 14? (15?) alveoli, respectively. In NMV P209977, a protuberance at the anterior end of the alveolar margin, within the anteroventral process, is interpreted as a blind, developing alveolus (Fig. 7). In NMV P212845 (with 14 or 15 alveoli), the anteriormost alveolus is small and contains a germ tooth. This developing tooth is in the same position as the protuberance on NMV P209977 (Fig. 8). As a result of the abrupt kink on the maxillae of the holotype (NMV P229196), NMV P212845, and possibly in P208178, the posterior portion of the ramus is posterolaterally deflected (Figs. 5, 8). The kink, however, is lacking on NMV P209977 and P186440 (Figs. 6, 7). Therefore, although noting limitations in sample size, the kink is both present and absent on maxillae of larger and smaller sizes. We postulate that the presence and absence of the kink is due to dimorphic rather than ontogenic variation. The dorsal surface of the maxillary ramus on the small maxilla NMV P209977 differs from the other maxillae by forming a pyramid-shaped peak (compare Figs. 5–8). On the other maxillae, including the similarly sized NMV P212845, the surface is smoothly convex. This variation is also incongruent to maxillary size and could be dimorphic. The reasons for dimorphism are unknown. Subspecies variation seems possible, particularly given that the fossil assemblage of the Flat Rocks Sandstone is time-averaged. Sexual dimorphism is also possible, although extremely difficult to assess (see Mallon, Reference Mallon2017). Minor variation is apparent among the observable tooth crowns, although secondary ridge numbers appear uniform. The distal paracingular fossa excavates the distal bounding ridge on the crowns of NMV P186440 to a greater degree than on the holotype (Fig. 15).
Etymology
dorisae, in recognition of Doris Seegets-Villiers for her geological, palynological, and taphonomic work on the Flat Rocks fossil vertebrate locality.
Materials
Flat Rocks Sandstone: NMV P212845, partial left maxilla lacking erupted dentition; NMV P208178, partial left maxilla with erupted dentition; NMV P208113, worn right maxillary tooth; NMV P208523, worn left maxillary tooth; and NMV P209977, partial left maxilla, lacking erupted dentition. The Caves: NMV P186440, posterior portion of left maxilla, left palatine, and fragment of left lacrimal.
Remarks
The maxilla of Galleonosaurus dorisae n. gen. n. sp. possesses several traits typically shared with noniguanodontian neornithischians, including: (1) extensive excavation of the maxilla by the antorbital fossa; (2) articulation of the ectopterygoid restricted to the posterior margin of the maxilla; (3) a single replacement cheek tooth per tooth family; (4) shallow medial inset of the premaxillary process on the maxilla; (5) absence of channel on the anterior ascending ramus of the maxilla for the premaxilla; (6) an obtuse anterior margin on the maxilla; and (7) the lack of contact between the jugal and the external antorbital fenestra by insertion of the lacrimal (as indicated on NMV P186440; Fig. 6). These traits are apparent in neornithischians such as Heterodontosaurus tucki (see Norman et al., Reference Norman, Crompton, Butler, Porro and Charig2011), Hypsilophodon foxii (see Galton, Reference Galton1974), Lesothosaurus diagnosticus (see Porro et al., Reference Porro, Witmer and Barrett2015), and Changchunsaurus parvus (see Jin et al., Reference Jin, Chen, Zan, Butler and Godefroit2010). However, an extensive antorbital fossa is also present in the rhabdodontids (e.g., Zalmoxes robustus [NHMUK R3395; unpublished data, Herne, 2009; see also Nopcsa, Reference Nopcsa1904, table 2; Weishampel et al., Reference Weishampel, Jianu, Csiki and Norman2003]) and basal dryomorphans (e.g., Camptosaurus dispar [YPM VP 1886, Fig. 9] and Dysalotosaurus lettowvorbecki [see Janensch, Reference Janensch1955; Galton, Reference Galton1983; Hübner and Rauhut, Reference Hübner and Rauhut2010]). Like Galleonosaurus dorisae n. gen. n. sp., the premaxillary process on the maxilla of Muttaburrasaurus langdoni lacks strong medial inset (unpublished data, Herne and Nair, 2018). However, as in styracosternans, the premaxillary process in M. langdoni is dorsally elevated and a deep channel for the premaxilla is developed on the posterodorsally sloping anterior margin of the anterior ascending ramus (e.g., Norman Reference Norman, Weishampel, Dodson and Osmólksa2004; Gasulla et al., Reference Gasulla, Escaso, Ortega and Sanz2014).
The maxilla of Galleonosaurus dorisae n. gen. n. sp. differs from that of Atlascopcosaurus loadsi by having a less protrusive posterolateral process and a shallower posterior slope on the dorsal surface of the maxillary ramus leading from the dorsal summit of the maxillary ramus to the ectopterygoid shelf. The maxilla of Galleonosaurus dorisae n. gen. n. sp. differs from that of Leaellynasaura amicagraphica by having greater lateral concavity of the maxillary alveolar axis and deeper buccal emargination. The number of alveoli in the largest complete maxillae of Galleonosaurus dorisae n. gen. n. sp. (up to 15) is greater than that currently known in L. amicagraphica (12 in the holotype, NMV P185991). However differing numbers of alveoli between these two taxa could be due to differing stages of ontogeny. On three of the five maxillary specimens currently assigned to Galleonosaurus dorisae n. gen. n. sp., the posterior third of the maxilla deflects posterolaterally outward at an abrupt kink (see Variation, above). The kinked form is regarded herein as a potential autapomorphy, with its absence on some maxillae interpreted as dimorphic variation.
The neurovascular tract in Galleonosaurus dorisae n. gen. n. sp. is separated from the posterior region of the antorbital fossa by a septum (see coronal section F in Fig. 11.3), which is potentially autapomorphic; however, this region has not been described in adequate detail in most ornithischians to fully confirm its uniqueness. In contrast to Galleonosaurus dorisae n. gen. n. sp., the neurovascular tract of Hypsilophodon foxii merges with the antorbital fossa. In Galleonosaurus dorisae n. gen. n. sp., the ventrolateral neurovascular foramina bypass the antorbital fossa to directly connect the neurovascular tract (Fig. 11.3, coronal sections B–F). These foramina in H. foxii (NHMUK R1477; Fig. 14), and most likely Zalmoxes robustus (NHMUK R3395; unpublished data, Herne, 2009), penetrate the supralveolar wall to directly enter the antorbital fossa. A similar condition is described in Changchunsaurus parvus and Jeholosaurus shangyuanensis (see Barrett and Han, Reference Barrett and Han2009; Jin et al., Reference Jin, Chen, Zan, Butler and Godefroit2010). In Mantellisaurus atherfieldensis, the neurovascular tract is separated from its reduced antorbital fossa and restricted to the region between the internal and external antorbital fenestra (see Witmer, Reference Witmer, Currie and Padian1997, fig. 11B). Similar morphology is likely in Tenontosaurus tilletti (see Thomas, Reference Thomas2015). In this aspect, separation of the neurovascular tract and posterior region of the antorbital fossa in Galleonosaurus dorisae n. gen. n. sp. appears closer to that of the nonrhabdodontid iguanodontians than to the condition in H. foxii and basal neornithischians. Future investigation of this region in other ornithischians could prove phylogenetically informative.
In Hypsilophodon foxii (NHMUK R1477; Fig. 14) and Tenontosaurus tilletti (see Thomas, Reference Thomas2015), the size of the posterodorsal neurovascular foramen relative to the dorsal surface of the maxilla is substantially larger than in Galleonosaurus dorisae n. gen. n. sp. (Figs. 6–8, 10, 11). The relatively small foramen in Galleonosaurus dorisae n. gen. n. sp. more closely resembles the condition in Camptosaurus dispar (YPM VP 1886; Fig. 9) and Mantellisaurus atherfieldensis (see Witmer, Reference Witmer, Currie and Padian1997, fig. 11B). The two slot-like foramina on the anterolateral margin of the anterior ascending ramus in Galleonosaurus dorisae n. gen. n. sp. (Fig. 5) that communicate with the neurovascular tract have not been previously described in any other ornithischian. Bifurcation of the neurovascular tract in the maxilla forming two anterior exits is also unique. For example, in Tenontosaurus tilletti (see Thomas, Reference Thomas2015) and Zephyrosaurus schaffi Sues, Reference Sues1980 (Sues, Reference Sues1980), a single maxillary foramen is reported within the anteroventral fossa. However, there is the possibility that dual foramina in other ornithischians have been previously overlooked.
In Galleonosaurus dorisae n. gen. n. sp., the anterior region of the antorbital fossa is partitioned from the internal nasal cavity by the medial lamina of the maxilla (Figs. 8, 11, 12) as in basal neornithischians (e.g., Jeholosaurus shangyuanensis [see Barrett and Han, Reference Barrett and Han2009]), basal dryomorphans (e.g., Dryosaurus elderae Carpenter and Galton, Reference Carpenter and Galton2018 [see Galton, Reference Galton1983], Dysalotosaurus lettowvorbecki [see Janensch, Reference Janensch1955; Hübner and Rauhut, Reference Hübner and Rauhut2010, p. 4], and Camptosaurus dispar [YPM VP 1886]), and possibly the rhabdodontid Zalmoxes robustus (suggested on the incomplete left maxilla, NHMUK R3395). However, in Tenontosaurus tilletti (see Thomas, Reference Thomas2015, figs. 10, 19) and styracosternans (e.g., Iguanodon bernissartensis [see Witmer, Reference Witmer, Currie and Padian1997, fig. 11B]), the medial lamina fails to partition the antorbital fossa. Similarly, in Hypsilophodon foxii (NHMUK R2477), the medial lamina of the maxilla is absent along the posterior margin of the ascending ramus and as a result, the anterior region of the antorbital fossa opens into the nasal cavity through the anterior (accessory) internal antorbital fenestra (Fig. 14.2). Therefore, among ornithopods, the form of the medial lamina in Galleonosaurus dorisae n. gen. n. sp. appears closer to basal dryomorphans than to H. foxii and Tenontosaurus tilletti.
The presence of a well-developed dorsal maxillary trough, as in Galleonosaurus dorisae n. gen. n. sp., is uncertain in Atlascopcosaurus loadsi and Leaellynasaura amicagraphica. A dorsal maxillary trough has been reported in Zalmoxes robustus (NHMUK R4901; Weishampel et al., Reference Weishampel, Jianu, Csiki and Norman2003), although this region in NHMUK R4901 is obscured by matrix. However, on another maxilla of Z. robustus (NHMUK R3395; unpublished data, Herne, 2009), the dorsal maxillary trough is absent because the maxilla is strongly excavated by the antorbital fossa (see also Nopcsa, Reference Nopcsa1904, table 2.3). In comparison to Galleonosaurus dorisae n. gen. n. sp., the dorsal maxillary trough is shallow in Tenontosaurus tilletti (see Thomas, Reference Thomas2015, figs. 14, 17) and Hypsilophodon foxii (NHMUK R2477; Fig. 14) and absent in Camptosaurus dispar. In the last taxon, the medial lamina of the maxilla markedly bulges medially to accommodate a transversely broad antorbital fossa (Fig. 9). Bulging of the medial lamina is also developed on the maxillae of Galleonosaurus dorisae n. gen. n. sp., in particular, in the smaller maxillae (NMV P209977, P212845; Figs. 7.2, 8.2, 8.3), but not to the extent observed in Camptosaurus dispar.
The lateral ramus of the palatine in Galleonosaurus dorisae n. gen. n. sp. is horizontally oriented and strap-like and expands laterally to form a flange (Figs. 6, 10). The hatchet-shaped form of the lateral palatine flange is a potential autapomorphy of the taxon. The lateral flange on the palatine is received in an anteroposteriorly oriented sutural facet on the dorsal surface of the maxilla posterior to the antorbital fossa (Figs. 5–8, 11). This facet on the maxilla for the palatine forms the dorsal surface of the flange that overlies the neurovascular tract (Figs. 5, 7, 8, 11.1; 11.2, 11.3, coronal section A)—morphology shared with Atlascopcosaurus loadsi (Fig. 10.6, 10.7) and possibly Leaellynasaura amicagraphica—and might be unique to these taxa. A lateral palatine flange is also postulated for Atlascopcosaurus loadsi (Fig. 10), as indicated by the facet on the dorsal surface of the maxilla as mentioned. However, in contrast to the hatchet-shaped flange on the lateral ramus of the palatine in Galleonosaurus dorisae n. gen. n. sp., that of Atlascopcosaurus loadsi was likely to have been asymmetrically expanded in the posterior direction (Fig. 10). As in Galleonosaurus dorisae n. gen. n. sp., the lateral palatine ramus in Camptosaurus dispar (YPM VP 1886) would have been horizontally oriented, as suggested by a rugose facet on the dorsal surface of its maxilla (Fig. 9). However, unlike in Galleonosaurus dorisae n. gen. n. sp., a facet on the maxilla for a lateral flange of the palatine is not apparent. The lateral ramus of the palatine in Jeholosaurus shangyuanensis is reportedly horizontally oriented (Barrett and Han, Reference Barrett and Han2009), as in Galleonosaurus dorisae n. gen. n. sp., but differs in being hook-like. Unlike in Galleonosaurus dorisae n. gen. n. sp., the lateral rami on the palatines of Hypsilophodon foxii (NHMUK R2477; Fig. 14) and Tenontosaurus tilletti (see Thomas, Reference Thomas2015) form thickened, dorsolaterally directed struts, which adjoin dorsally raised pedestals on their maxillary rami. The distal ends of these rami lack an expanded flange. A lateral ramus on the palatine is absent in Thescelosaurus neglectus (see Brown et al., Reference Brown, Boyd and Russell2011; Boyd, Reference Boyd2014) and styracosternans (e.g., Horner, Reference Horner1992). This region currently lacks detailed description in dryosaurids and ornithischians in general, thus inhibiting more extensive comparisons.
The maxillae of Galleonosaurus dorisae n. gen. n. sp. suggest that new alveoli developed at the anterior end of the tooth row during ontogeny, which is consistent with the pattern of development interpreted in Dysalotosaurus lettowvorbecki (see Hübner and Rauhut, Reference Hübner and Rauhut2010), Jeholosaurus shangyuanensis (see Barrett and Han, Reference Barrett and Han2009), and Heterodontosaurus tucki (see Norman et al., Reference Norman, Crompton, Butler, Porro and Charig2011). The sinuous lingual margin on the maxillary tooth roots in Galleonosaurus dorisae n. gen. n. sp. is not presently reported in any other ornithischian (Fig. 16).
Galleonosaurus dorisae n. gen. n. sp. possesses a combination of seven maxillary crown features, which include: (G1) spatulate, asymmetrical crowns, resulting from having markedly V-shaped labial and lingual cingular vertices offset mesially relative to the central root axis and the primary ridge (developed labially) offset to distal third of the crown surface; (G2) the primary ridge on some crowns slightly undercut by the distal paracingular fossa; (G3) the distal bounding ridge developed labially being longer and more sloping than the mesial bounding ridge, which is relatively straight and vertical; (G4) secondary ridges, developed labially, closely abutting the ridges distal to the primary ridge more finely developed than those mesially; (G5) secondary ridges developed lingually, with mesiodistally narrow crests; (G6) the primary ridge and secondary ridges mesial to the primary ridge, terminating apically in mamillated, tridenticulate cusps; and (G7) apical crown margins lacking the development of multiple unsupported lingulate denticles.
Nearly all of the aforementioned maxillary crown features (G1–7) in Galleonosaurus dorisae n. gen. n. sp. are shared with Atlascopcosaurus loadsi, Leaellynasaura amicagraphica, and Muttaburrasaurus spp. (see comparisons, Fig. 17). However, specific differences are apparent among these taxa. Whereas the cingular vertex on the labial crown surfaces of Galleonosaurus dorisae n. gen. n. sp., L. amicagraphica, and Muttaburrasaurus spp. are V-shaped and mesially offset (in part, crown feature G1), the labial cingular vertex on the Atlascopcosaurus loadsi crowns is U-shaped and more centrally positioned. However, the lingual vertex on the Atlascopcosaurus loadsi crowns is V-shaped and mesially offset, as in Galleonosaurus dorisae n. gen. n. sp. The base of the primary ridge on the crowns of Galleonosaurus dorisae n. gen. n. sp., Atlascopcosaurus loadsi, and Muttaburrasaurus spp. merges more distally with the distal bounding ridge than on the crowns of L. amicagraphica (Fig. 17). On crowns of similar size, those of Galleonosaurus dorisae n. gen. n. sp. differ from those of Atlascopcosaurus loadsi by having fewer secondary ridges mesial to primary ridge (four ridges compared with six) and fewer, more finely developed, secondary ridges distal to primary ridge (two ridges compared with four). In secondary ridge number and development, the crowns of L. amicagraphica are closer to those of Galleonosaurus dorisae n. gen. n. sp. than to those of Atlascopcosaurus loadsi. The number of secondary ridges on the maxillary crowns of Muttaburrasaurus spp., mesial to the primary ridge, is substantially greater (~11 ridges) than on the crowns of the Victorian taxa. However, the number of secondary ridges in the distal paracingular fossa on the crowns of Muttaburrasaurus sp. (QM F14921; four ridges) is comparable to that in Atlascopcosaurus loadsi. Tridenticulate mamillated cusps on the crowns of Galleonosaurus dorisae n. gen. n. sp. (crown feature G6; Fig. 15) are shared with the holotype of Atlascopcosaurus loadsi (NMV P166409), and are unknown on the crowns of L. amicagraphica and Muttaburrasaurus spp. Tridenticulate marginal cusps have previously been reported only in the Late Jurassic Laurentian neornithischian Nanosaurus agilis (‘multi-cuspid cusps’ of Carpenter and Galton, Reference Carpenter and Galton2018).
Most of the maxillary crown features in Galleonosaurus dorisae n. gen. n. sp. are also shared with the Argentinian ornithopods Gasparinisaura cincosaltensis (MUCPv 208; unpublished data, Herne, 2008) and Talenkauen santacrucensis Novas, Cambiaso, and Ambrosio, Reference Novas, Cambiaso and Ambrosio2004 (based on Cambiaso, Reference Cambiaso2007, fig. 17). These include having: (1) spatulate crowns (Fig. 3.3c) with V-shaped cingular vertices and asymmetrical form, resulting from distal offset of the primary ridge (crown feature G1; noting that mesial offset of the cingular vertices is uncertain); (2) closely abutting secondary ridges developed labially, eliminating space for ridge-free paracingular fossae with finer secondary ridges developed distal to the primary ridge (crown feature G4); (3) the primary ridge slightly undercut by the distal paracingular fossa (crown feature G2); and (4) the absence of multiple unsupported lingulate denticles along the apical margins of the crowns (crown feature G7). Apicobasal ridges are also developed on the lingual crowns of Talenkauen santacrucensis (crown feature G5), but these are presently unknown in Gasparinisaura cincosaltensis.
Cambiaso (Reference Cambiaso2007) described the maxillary dentition of Anabisetia saldiviai Coria and Calvo, Reference Coria and Calvo2002, suggesting that the maxilla of the holotype (MCF-PVPH-74; see Coria and Calvo, Reference Coria and Calvo2002) possessed two crown morphologies, one larger and one smaller. In agreement with Cambiaso (Reference Cambiaso2007), the presence of two crown morphologies on the fragmentary holotypic maxilla (unpublished data, Herne, 2008) confirms that the maxillary dentition of Anabisetia saldiviai is unusually heterodont. Importantly, Anabisetia saldiviai shares several maxillary crown features with Galleonosaurus dorisae n. gen. n. sp. On the larger crown form (see Cambiaso, Reference Cambiaso2007): (1) the primary ridge is undercut by the distal paracingular fossa (crown feature G2); (2) the crown profile is spatulate (crown feature G1, in part, although not asymmetrical in form); (3) apicobasal ridges are possibly present on the lingual crown surface (crown feature G5); (4) mamillated marginal cusps are present (crown feature G6, in part, noting that fully-extending, lingual secondary ridges are absent, and the development of tridenticulate cusps unknown); and (5) multiple unsupported marginal lingulate denticles are absent (crown feature G7). However, the larger crown form of Anabisetia saldiviai also shares with dryomorphans (e.g., Dryosaurus spp. and Mantellisaurus atherfieldensis [see Galton, Reference Galton1983; Norman, Reference Norman1986]) relatively smooth mesial and distal paracingular fossae flanking a sharp-crested primary ridge; this is not present on the crowns of Galleonosaurus dorisae n. gen. n. sp. The smaller maxillary crown form in Anabisetia saldiviai shares with Galleonosaurus dorisae n. gen. n. sp.: (1) a spatulate asymmetrical form, with a mesially offset V-shaped cingular vertex and a distally positioned primary ridge (see also Cambiaso, Reference Cambiaso2007; crown feature G1, noting that the presence of a mesially offset vertex on the lingual crown surface is unknown; that is undercut by a narrow distal paracingular fossa [crown feature G2]); and (2) closely abutting, round-crested secondary ridges (Cambiaso, Reference Cambiaso2007) mesial to the primary ridge (crown feature G4, noting that secondary ridges distal to the primary ridge appear absent).
The maxillary crown features shared between Galleonosaurus dorisae n. gen. n. sp., other Victorian ornithopods, Muttaburrasaurus spp., and the Argentinian ornithopods, as mentioned, suggest that these taxa could be closely related. Moreover, the crown features differ from those of Tenontosaurus spp. and dryomorphans (e.g., Galton, Reference Galton1983; Norman Reference Norman1986; Thomas, Reference Thomas2015), in which the crowns are rhomboidal (diamond-shaped or lanceolate; Fig. 3.3d), the mesial and distal paracingular fossae are smooth (i.e., ridge-free) or have channels between the secondary ridges, and multiple unsupported lingulate denticles are present along the apical margins.
As a concept of tooth replacement efficiency in the mature stages of all reptiles, a Z-spacing of 1.75–2.5 indicates that at least three teeth are in a favorable functioning state within a sequence of eight tooth positions (following Osborn, Reference Osborn1975). The estimated Z-spacing in the holotype of Galleonosaurus dorisae n. gen. n. sp. of 1.65 is slightly less than the lowest replacement timing (i.e., 1.75) suggested by Osborn (Reference Osborn1975). However, because the value we calculated is based solely on alveolar positions M7–M8 on the holotype, the amount of Z-spacing in Galleonosaurus dorisae n. gen. n. sp. is inconclusive.
In summary, the overall morphology of the maxilla, dentition, and palatine suggest tht Galleonosaurus dorisae n. gen. n. sp., Atlascopcosaurus loadsi, and Leaellynasaura amicagraphica are closely related. Apart from these taxa, closer affinities between Galleonosaurus dorisae n. gen. n. sp., Anabisetia saldiviai, Gasparinisaura cincosaltensis, Muttaburrasaurus spp., and Talenkauen santacrucensis are suggested by shared features of the dentition. However, the larger maxillary crown form in Anabisetia saldiviai also suggests morphology shared with dryomorphans. The lack of an anterior groove on the anterior margin of the maxilla for the premaxilla and the shallow medial inset of the premaxillary process are features consistent with nondryomorphan neornithischians. However, the kinked shape of the maxilla in Galleonosaurus dorisae n. gen. n. sp. (in dorsoventral view), and the relatively small size of the posterodorsal neurovascular foramen relative to the maxillary ramus, resemble features in Camptosaurus dispar (Figs. 5, 8, 9, 11). These features suggest that Galleonosaurus dorisae n. gen. n. sp. might be closer to basal dryomorphans than to both Hypsilophodon foxii (NHMUK R2477; Fig. 14) and the basal iguanodontian Tenontosaurus tilletti (based on Thomas, Reference Thomas2015, fig. 12), in which the posterodorsal neurovascular foramen is comparatively large. Further investigations that compare the dorsal and internal structures of the maxilla in more neornithischians are likely to be phylogenetically informative.
Genus Atlascopcosaurus Rich and Rich, Reference Rich and Rich1989
Type species
Atlascopcosaurus loadsi Rich and Rich, Reference Rich and Rich1989; Point Lewis and Dinosaur Cove in the Otway region of Victoria, southeastern Australia; lower Albian of the Eumeralla Formation in the Otway Basin.
cf. Atlascopcosaurus loadsi (Rich and Rich, Reference Rich and Rich1989)
Figures 17.3, 18; Table 2
Occurrence
Flat Rocks locality in the Inverloch region of Victoria, southeastern Australia (Fig. 1); Flat Rocks Sandstone, upper Barremian of the Wonthaggi Formation in the Gippsland Basin.

Figure 18. Victorian ornithopodan maxillary morphotype 4 (cf. Atlascopcosaurus loadsi Rich and Rich, Reference Rich and Rich1989) from the Flat Rocks Sandstone in the Wonthaggi Formation, southeastern Australia: (1) right maxilla (NMV P208133) in lateral view; (2, 3) maxillary dentition in lingual (2) and labial (3) views, with schematic of crown surface in (2). aof = antorbital fossa; bur = buccal ridge; cv = cingular vertex; epf = ectopterygoid flange on maxilla; m#’ = maxillary tooth position (from posterior end) and replacement number; mra = maxillary ramus; nvt = neurovascular tract; ocf = occlusal facet; sal = supralveolar lamina; sgj = sutural groove for jugal. Scale bars = 10 mm.
Materials
NMV P208133, partial right maxilla with erupted dentition.
Remarks
The maxilla NMV P208133 (Fig. 18) is referred to cf. Atlascopcosaurus loadsi based on features of the maxillary tooth crowns shared with Atlascopcosaurus loadsi from the Eumeralla Formation. The maxilla is hereafter termed ‘Victorian ornithopod maxillary morphotype 4’ (VOM4). As in Atlascopcosaurus loadsi, the maxillary crowns of VOM4 have a U-shaped labial cingular vertex and strongly developed secondary apicobasal ridges labially in the distal paracingular fossa (Fig. 17). The crowns of Galleonosaurus dorisae n. gen. n. sp. differ from those of VOM4 by having: (1) a V-shaped cingular vertex on the labial crown surface; (2) greater mesial offset of the labial cingular vertex; (3) merger between the primary and distal bounding ridge more distally offset; and (4) secondary ridges, distal to the primary ridge, more weakly developed (see the listed combination of maxillary crown features in Remarks for Galleonosaurus dorisae n. gen. n. sp.). VOM4 possesses four strongly developed secondary ridges mesial to the primary ridge, as in Galleonosaurus dorisae n. gen. n. sp., and at least three secondary ridges distal to the primary ridge, as in Atlascopcosaurus loadsi (Fig. 17). Although incomplete, the dorsoventral depth of the maxillary ramus in NMV P208133 appears more like that of Galleonosaurus dorisae n. gen. n. sp. than of Atlascopcosaurus loadsi, particularly the referred maxilla NMV P157390, in which the dorsal peak of the ramus is comparatively higher (compare Figs. 13 and 18). Thus, from the morphology available, VOM4 (NMV P208133) appears intermediate to Atlascopcosaurus loadsi and Galleonosaurus dorisae n. gen. n. sp. Nonetheless, we consider features of the maxillary tooth crowns closer to those of Atlascopcosaurus loadsi.
Genus Qantassaurus Rich and Vickers-Rich, Reference Rich and Vickers-Rich1999
Type species
Qantassaurus intrepidus Rich and Vickers-Rich, Reference Rich and Vickers-Rich1999 from the Flat Rocks Sandstone, Victoria, southeastern Australia, by original designation.
Qantassaurus intrepidus Rich and Vickers-Rich, Reference Rich and Vickers-Rich1999
Figures 19, 20.1, 20.3–20.5; Tables 3, 4
- 1999
Qantassaurus intrepidus Rich and Vickers-Rich, p. 175, fig. 9.

Figure 19. Qantassaurus intrepidus Rich and Vickers-Rich, Reference Rich and Vickers-Rich1999, holotypic left dentary (NMV P199075) and schematics from the Flat Rocks Sandstone in the Wonthaggi Formation, southeastern Australia, in lateral (1), medial (2), and dorsal (3) views. alv = alveolus; bur = buccal ridge; cem = cementum; cop = coronoid process; cor = coronoid ridge; cos = coronoid shelf; d# = dentary tooth position (from anterior end) and replacement number; dfo = anterior dentary foramen; ldp = lateral dentary parapet; mdp = medial dentary parapet; mkc = Meckelian canal (fossa); mkg = Meckelian groove; nuf = nutrient foramen; nvf = neurovascular foramen; pdf = predentary facet; pdp = predentary process; spf = splenial facet; sym = symphyseal surface. Scale bar = 10 mm. Images (1, 2) courtesy of Museums Victoria.
Table 3. Measurements (in mm) of Victorian ornithopod dentary specimens. APL1 = total anteroposterior length of ramus; APL2 = anteroposterior length of alveolar margin; CPA = angle of coronoid process relative to alveolar margin; DVD1 = dorsoventral depth from lateral alveolar margin at midtooth row to ventralmost point; DVD2 = dorsoventral depth of dentary and dentition at midtooth row; TVWR = transverse width of ramus at midtooth row; + = measurement incomplete; e = estimation.

Table 4. Percentage of dentary depth against tooth row length for selected ornithopods. ALTR = total anteroposterior length of tooth row; DVD = greatest dorsoventral depth of dentary and dentition from ventral margin to occlusal margin (exclusive of coronoid process); DVD/ALTR = percentage of the dentary depth to tooth row length. All measurements in mm.

Holotype
NMV P199075, almost complete dentary from the Wonthaggi Formation, Victoria, Australia.
Diagnosis
Small-bodied ornithopod characterized by a combination of six features, including three potential autapomorphies (*): (1*) dentary markedly foreshortened (ratio of greatest dorsoventral height from ventral margin to alveolar margin/total tooth row length ≅ 0.6); (2*) dorsoventral depth of the lateral alveolar parapet higher than the portion of dentary ramus ventral to the buccal ridge (ratio of alveolar parapet height/height ventrally to buccal ridge ≅ 1.8); (3*) line of the buccal ridge deeply concave and offset ventrally relative to the line of the coronoid ridge; (4) level of the lateral alveolar parapet markedly elevated relative to the coronoid ridge and shelf; (5) occlusal margin on the dentary teeth markedly convex; and (6) ventral margin of the dentary ramus convex over its length as far as the symphyseal margin. (Amended from the original work of Rich and Vickers-Rich, Reference Rich and Vickers-Rich1999).
Occurrence
Flat Rocks locality in the Inverloch region of Victoria, southeastern Australia (Fig. 1); Flat Rocks Sandstone, upper Barremian of the Wonthaggi Formation in the Gippsland Basin.
Description
(Amended from that of Rich and Vickers-Rich, Reference Rich and Vickers-Rich1999 with some information by the original authors only repeated where new context is considered necessary.) The taxon is known only from a single dentary with almost complete dentition (Figs. 19, 20). Two previously referred dentaries (NMV P198962, P199087; Rich and Vickers-Rich, Reference Rich and Vickers-Rich1999) are removed and assigned herein to Qantassaurus ?intrepidus (Fig. 21). The predentary and coronoid processes are degraded and incomplete. The two posteriormost erupted teeth are missing. Ten alveoli are present (sensu Rich and Vickers-Rich, Reference Rich and Vickers-Rich1999), however, a small, anterior, triangular, matrix-filled pit suggests an eleventh alveolus could be present, but it is yet unconfirmed. Viewed dorsally (Fig. 19.3), the alveolar margin is shallowly concave laterally, and the buccal emargination depth (measured midway along the tooth row) roughly equals the labiolingual width of a single crown. Viewed lateromedially, the occlusal margin is markedly convex (Fig. 20.1), with the greatest depth of the dentition (taken from the anteroposterior cord along the tooth row) ~16% of the total tooth row length. The buccal ridge forms a deeply concave margin, the line of which is separated from and depressed ventrally to the line of the coronoid ridge (Fig. 19). The lateral dental parapet (= buccal shelf) forms a dorsoventrally deep fossa between the buccal ridge and the alveolar margin, as in Dysalotosaurus lettowvorbecki (see Janensch, Reference Janensch1955, table 11), Zalmoxes robustus (NHMUK R3407, although not R3392), and Owenodon hoggii Owen, Reference Owen1874 (NHMUK R2998). The lateral dental parapet is distinctly elevated dorsally relative to the coronoid shelf (Fig. 19), as in Dysalotosaurus lettowvorbecki (see Janensch, Reference Janensch1955, table 11). Five ovate neurovascular foramina penetrate the lateral dental parapet. The ventral margin of the dentary is convex up to the symmetrically triangular predentary process. The facet for the caudolateral process of the predentary is grooved and the anterior dentary foramen is approximately in the middle of the predentary process (Fig. 19). The dorsomedial surface of the predentary process is spout-shaped.

Figure 20. Dentition of Qantassaurus intrepidus Rich and Vickers-Rich, Reference Rich and Vickers-Rich1999 and comparisons: (1, 2) schematics of dentary occlusal margin profiles in medial view of Q. intrepidus (NMV P199075) (1) and Owenodon hoggii Owen, Reference Owen1874 (NHMUK R2998, holotype) (2); (3, 4) holotypic left dentition of Q. intrepidus (NMV P199075) in lingual (3) and labial (4) views (images courtesy of Museums Victoria); (5, 6) schematics showing lingual ornamentation of unworn left dentary tooth d5.1 in Q. intrepidus (NMV P199075) (5) and unworn isolated left dentary tooth (NMV P177934) from the Eumeralla Formation referred to Atlascopcosaurus loadsi Rich and Rich, Reference Rich and Rich1989 (6) (Rich and Vickers-Rich, Reference Rich and Rich1989, fig. 11B); (7, 8) unworn left dentary tooth (NMV P186426) from the Eumeralla Formation in lingual (7) and labial (8) views; (9–11) right worn dentary tooth of Kangnasaurus coetzeei Haughton, Reference Haughton1915 (SAM-PK-2732; reversed) showing schematic of lingual ornamentation (9) (from image courtesy of K. Poole) and labial (10) and distal (11) views of cast (NMV P197995). Lines in (1, 2) indicate tooth row length and depth. 1abr = primary apicobasal ridge; 2abr = secondary apicobasal ridge; abr = apicobasal ridge; cft = cleft; cv = cingular vertex; cvr = convergent (secondary) ridge; d# = dentary tooth position (from anterior end) and replacement number; dbr = distal bounding ridge; gch = growth channel; ldc = lingulate denticle; mbr = mesial bounding ridge; ocf = occlusal facet. Scale bars: 10 mm (1–2, 9–11); 5 mm (3–8).

Figure 21. Victorian ornithopodan dentary morphotype 2 (Qantassaurus ?intrepidus Rich and Vickers-Rich, Reference Rich and Vickers-Rich1999) from the Flat Rocks Sandstone in the Wonthaggi Formation, southeastern Australia: (1–3) left dentary (NMV P198962) and schematics in lateral (1), medial (2), and dorsal (3) views; (4, 5) right dentary (NMV P199087) and schematics in lingual (4) and dorsal (5) views; (6) schematic size comparison between NMV P198962 and P199087 (reversed) in dorsal view. 1abr = primary apicobasal ridge; alv = alveolus; bur = buccal ridge; cop = coronoid process; cor = coronoid ridge; cos = coronoid shelf; d# = dentary tooth position (from anterior end) and replacement number; dfo = anterior dentary foramen; ldp = lateral dentary parapet; mkc = Meckelian canal (fossa); mkg = Meckelian groove; nuf = nutrient foramen; nvf = neurovascular foramen; pdf = predentary facet; pdp = predentary process; sym = symphyseal surface. Scale bars = 10 mm.
Laterally (Fig. 19.1), the alveolar and ventral margins are convergent, as in Talenkauen santacrucensis, Hypsilophodon foxii, Dryosaurus spp. (in agreement with Agnolin et al., Reference Agnolin, Ezcurra, Pais and Salisbury2010), Dysalotosaurus lettowvorbecki (see Janensch, Reference Janensch1955, table 11), and Thescelosaurus neglectus (see Galton, Reference Galton1997, fig. 1). Medially (Fig. 19.2), the dorsal and ventral margins of the dentary ramus (taken as the region between the medial groove and the ventral margin) are also convergent, as in Dysalotosaurus lettowvorbecki (see Janensch Reference Janensch1955, fig. 12) and, provisionally, Talenkauen santacrucensis (see Cambiaso, Reference Cambiaso2007, fig. 15) and Owenodon hoggii (see Norman and Barrett, Reference Norman and Barrett2002, fig. 2). In contrast, these margins are divergent anteriorly in at least H. foxii (based on Galton, Reference Galton1974, fig. 10), Tenontosaurus tilletti (see Thomas, Reference Thomas2015, fig. 47), and possibly Thescelosaurus neglectus (see Galton, Reference Galton1997, fig. 1). The greatest dorsoventral height of the dentary and dentition is ~ 60% of the tooth row length. In this aspect, the dentary of Qantassaurus intrepidus is higher than in the other ornithopods compared herein (Table 4). Cementum forming the medial dental parapet encasing the erupted and developing crowns dorsally to the medial groove (Fig. 19.2) is distinguished by a slightly pitted texture. Elongate nutrient foramina distributed along the medial groove align with the tooth families. The triangular medial opening of the Meckelian canal forms an included angle of ~ 30°. The opening pinches out posterior to the middle of the dentary, and anterior to this point, a narrow Meckelian groove continues onto the dorsal surface of the predentary process (Fig. 19.2). The surface for the splenial/prearticular is slightly depressed with anteroposteriorly oriented striae. Dorsal to the splenial surface, another, smaller sutural surface with finer striae likely adjoined the coronoid.
Dentition
Only a single replacement crown is present per tooth family, as in basal ornithopods and basal iguanodontians (Norman, Reference Norman, Weishampel, Dodson and Osmólksa2004; Norman et al., Reference Norman, Sues, Witmer, Coria, Weishampel, Dodson and Osmólksa2004). The complete morphology of the root is uncertain. Viewed lingually, the crowns are spatulate and mesiodistally expanded relative to the roots (Fig. 20.3). The cingulum is more strongly developed lingually than labially. Labially, the cingular vertex is deeply V-shaped (Fig. 20.4), however, on most of the crowns (where observable), the cingular base merges with the root. Lingually, the mesial and distal bounding ridges converge to form a V-shaped cingular vertex (Fig. 20.3). The outer margins of the mesial and distal bounding ridges are convex and concave, respectively. The largest unworn crowns are midway along the tooth row. Measured lingually, the apicobasal depth of the largest observable crown (d5) is ~1.4 times its mesiodistal width. A continuous occlusal margin is formed. Lingually, the primary ridge is weakly developed, offset distally relative to the central axis of the crown, and has predominantly parallel margins that expand only slightly at the ridge base (Fig. 20.3, 20.5). The lingual margin of the primary ridge is apicobasally straight and the slightly expanded base of the ridge protrudes lingually from the cingulum. Clefts are formed between the primary ridge and the mesial and distal bounding ridges. Lingually, up to six lingulate denticles are developed in a cluster on the mesialmost margin, apically to the mesial bounding ridge, and a similar number of lingulate denticles are developed in the equivalent position on the distal margin. Six secondary ridges are developed on the mesial lobe, three of which are convergent with the primary ridge, and five ridges are developed on the distal lobe, one of which is convergent with the primary ridge. The pattern of ridge development appears consistent among the crowns. Apicobasal ridges, developed labially, have sharp crests and are separated by channels.
Remarks
A foreshortened dentary (sensu Rich and Vickers-Rich, Reference Rich and Vickers-Rich1999), dorsoventrally high lateral dental parapet, and a markedly convex occlusal margin are distinctive features of Qantassaurus intrepidus (Figs. 19, 20). The foreshortened dentary of Q. intrepidus, quantified by the dorsoventral dentary depth (at its deepest point along the tooth row) of 60% of the tooth row length, is greater than in the other ornithopods compared herein, with the depth in Zalmoxes shqiperorum Weishampel et al., Reference Weishampel, Jianu, Csiki and Norman2003 closest (Table 4). Among cerapods, dorsoventrally deep dentaries are also present in basal neoceratopsians, such as Bagaceratops rozhdestvenskyi Maryanska and Osmolska, Reference Maryanska and Osmolska1975 (Alifanov, Reference Alifanov2003, fig. 3a) and Archaeoceratops oshimai Dong and Azuma, Reference Dong, Azuma and Dong1997 (You and Dodson, Reference You and Dodson2003). The dorsoventrally high lateral dental parapet results from a deeply concave buccal ridge, a highly convex occlusal margin, and dorsally highset dentition (Figs. 19, 20). Marked ventral depression of the buccal ridge, relative to the coronoid ridge, differs from that in other ornithopods in which these ridges are less offset or roughly continuous. The highset dentition results from marked dorsal elevation of the dental parapet relative to the line of the coronoid shelf, as in Dysalotosaurus lettowvorbecki (see Janensch, Reference Janensch1955, table 11). Marked dorsal convexity of the occlusal margin closely resembles the margin in Owenodon hoggii (NHMUK R2998; Fig. 20.1, 20.2). The uniformly convex ventral margin on the dentary lacking recurvature anteriorly toward the symphyseal margin, also resembles the dentaries of Z. shqiperorum (Godefroit et al., Reference Godefroit, Codrea and Weishampel2009) and some basal neornithischians (e.g., B. rozhdestvenskyi; Alifanov, Reference Alifanov2003, fig. 3a) and differs from those in most other ornithopods, in which the ventral margin is concave or sinuous and procurved toward the symphyseal margin (in, e.g., Hypsilophodon foxii [see Galton, Reference Galton1974] and Thescelosaurus neglectus [see Boyd, Reference Boyd2014]).
The apicobasally tall, spatulate dentary crowns of Qantassaurus intrepidus, possessing closely abutting, basally convergent secondary ridges lingually, and apicobasal ridges labially with the primary ridge offset distally, are a combination of features resembling the crowns of Talenkauen santacrucensis (see Cambiaso, Reference Cambiaso2007, fig. 17) and the dryosaurid Kangnasaurus coetzeei Haughton, Reference Haughton1915 (SAM-PK-2732; Fig. 20.5, 20.9; see also Cooper, Reference Cooper1985). The Q. intrepidus crowns differ from the subtriangular crowns (Fig. 3.3a) of noncerapodan neornithischians, e.g., Changchunsaurus parvus, Jeholosaurus shangyuanensis, Orodromeus makelai Horner and Weishampel, Reference Horner and Weishampel1988, and Thescelosaurus neglectus—taxa that also have a prominent apicobasal swelling on the crowns, rather than a primary ridge, and fully extending apicobasal ridges on the crowns are lacking (following Jin et al., Reference Jin, Chen, Zan, Butler and Godefroit2010). The dentary crowns of the rhabdodontids, Zalmoxes robustus and Z. shqiperorum, differ from those of Q. intrepidus in being proportionally larger relative to the dentary, having higher, triangular apical peaks, and a greater number of apicobasal ridges (Weishampel et al., Reference Weishampel, Jianu, Csiki and Norman2003; Godefroit et al., Reference Godefroit, Codrea and Weishampel2009). The dentary crowns of Hypsilophodon foxii (NHMUK R2477) and Tenontosaurus tilletti (see Thomas, Reference Thomas2015) differ by having relatively vertical secondary ridges separated by channels, multiple unsupported lingulate marginal denticles, and a more strongly developed primary ridge. The crowns of dryomorphans (e.g., Bayannurosaurus perfectus Xu et al., Reference Xu, Tan, Gao, Bao, Yin, Guo, Wang, Tan, Zhang and Xing2018 [Xu et al., Reference Xu, Tan, Gao, Bao, Yin, Guo, Wang, Tan, Zhang and Xing2018, fig. 2], Camptosaurus dispar [YPM VP 1886], Dryosaurus altus Marsh, Reference Marsh1878 [YPM VP 1876], and other iguanodontians [Mantell, Reference Mantell1848; Fanti et al., Reference Fanti, Cau, Panzarin and Cantelli2016]), with the exception of K. coetzeei, differ from those of Q. intrepidus by having multiple lingulate marginal denticles on the apical crown margins, sparsely developed lingual apicobasal ridges on comparatively smooth mesial and distal paracingular fossae, and smooth labial crown surfaces. The crowns of Tenontosaurus tilletti (see Thomas, Reference Thomas2015, fig. 23), styracosternans (Godefroit et al., Reference Godefroit, Escuillié, Bolotsky, Lauters and Godefroit2012), and Z. robustus (NHMUK R3407), further differ from those of Q. intrepidus by having lingually facing lingulate denticles developed on an everted marginal lip. Mesial facing denticles also appear to be lacking on the crowns of Dryosaurus altus (YPM VP 1876).
On the labial surfaces of the dentary crowns in Qantassaurus intrepidus, narrow apicobasal ridges, separated by channels, resemble those in Hypsilophodon foxii (NHMUK R2477), Zalmoxes shqiperorum (see Godefroit et al., Reference Godefroit, Codrea and Weishampel2009, fig. 11B), and possibly Kangnasaurus coetzeei (SAM-PK-2732). Two isolated dentary teeth from the Eumeralla Formation (NMV P177934, previously referred to Atlascopcosaurus loadsi by Rich and Rich, Reference Rich and Rich1989, fig. 11B; and NMV P186426) also resemble the crowns of Q. intrepidus (Fig. 20.6–20.8), suggesting that Q. intrepidus or a Q. intrepidus-like ornithopod had also been present in the lower Albian of the Eumeralla Formation. One of the aforementioned isolated crowns (NMV P177934) demonstrates a similar degree of apicobasal convexity on the lingual surface of the crown as in Q. intrepidus, in distal view (Fig. 20.8). Furthermore, convexity of the crown surfaces in Q. intrepidus and NMV P177934 also resemble those of the partly worn K. coetzeei crown (Fig. 20.11), which is ~ 50% larger than the Victorian crowns.
Qantassaurus ?intrepidus Rich and Vickers-Rich, Reference Rich and Vickers-Rich1999
Figure 21; Table 3
Occurrence
Flat Rocks locality in the Inverloch region of Victoria, southeastern Australia (Fig. 1); Flat Rocks Sandstone, upper Barremian of the Wonthaggi Formation in the Gippsland Basin.
Materials
NMV P198962, incomplete left dentary lacking erupted dentition; NMV P199087, right dentary fragment lacking erupted dentition.
Remarks
The dentaries assigned herein to Qantassaurus ?intrepidus are hereafter termed ‘Victorian ornithopodan dentary morphotype 2’ (VOD2). In this context, Q. intrepidus is VOD1. The more complete of the two dentaries (NMV P198962) is similar in size to the Q. intrepidus holotype. As in Q. intrepidus, 10 alveoli are present (see NMV P198962; Fig. 21), and a small, triangular alveolus could be developed anteriorly, but its presence is uncertain. The dentary is strongly bowed in both mediolateral and dorsoventral views. The lateral margin is markedly concave and the medial margin is strongly convex. In contrast, curvature on the Q. intrepidus dentary is comparatively shallow (Fig. 19). The medial margin on VOD2 (NMV P198962) is smoothly convex, which differs from Q. intrepidus in which a distinct kink is evident. In mediolateral view, the coronoid process is angled at 70° relative to the anteroposterior axis of the alveolar margin, as in Q. intrepidus, and its distal end is slightly expanded and rounded (Fig. 21). The medioventral surface adjacent to the symphyseal margin is depressed by a shallow fossa that could have accommodated the ventrolateral process of the predentary. The symmetrically triangular predentary process resembles those of Dysalotosaurus lettowvorbecki, Hypsilophodon foxii, Tenontosaurus tilletti, and Zalmoxes robustus (Janensch, Reference Janensch1955; Galton, Reference Galton1974; Weishampel et al., Reference Weishampel, Jianu, Csiki and Norman2003; Thomas, Reference Thomas2015), and contrasts with the comparatively asymmetrical form in ankylopollexians, e.g., Camptosaurus dispar (YPM VP 1886) and Mantellisaurus atherfieldensis (see Norman, Reference Norman1986), in which the dorsal facet is longer than the relatively horizontal ventral facet. The shape of the VOD2 crowns and their ornamentation resemble those of Q. intrepidus.
As originally described by Rich and Vickers-Rich (Reference Rich and Vickers-Rich1999, p. 175), the left dentary (NMV P198962) was considered to differ from that of the Qantassaurus intrepidus holotype by having a “bloated” appearance, which according to these authors, potentially resulted from a pathological condition. However, the incomplete right dentary (NMV P199087), originally referred to Q. intrepidus (see Rich and Vickers-Rich, Reference Rich and Vickers-Rich1999), has morphology closer to NMV P198962 (Fig. 21.6) than to the Q. intrepidus holotype. The dentary (NMV P199087) appears to be slightly larger than in NMV P198962 and, therefore, is unlikely to pertain to the same individual. Thus, NMV P198962 and P199087 confirm the presence of two VOD2 individuals, based on comparable morphology differing from that of the Q. intrepidus holotype. VOD2 could represent dimorphism among Q. intrepidus or alternatively a separate taxon.
Ornithopoda indet.
Figures 22–24.6; Table 3

Figure 22. Victorian ornithopodan dentary morphotype 3 (Ornithopoda indet.) from the Flat Rocks Sandstone in the Wonthaggi Formation, southeastern Australia: (1–3) left dentary (NMV P228408) and schematics in lateral (1), dorsal (2), and medial (3) views; (4–6) right dentary (NMV P199135) and schematics in lateral (4), dorsal (5), and medial (6) views. alv = alveolus; cem = cementum; cop = coronoid process; cor = coronoid ridge; cos = coronoid shelf; d# = dentary tooth position (from anterior end) and replacement number; dfo = anterior dentary foramen; ldp = lateral dentary parapet; mcc = medial coronoid cusp; mdp = medial dentary parapet; mkc = Meckelian canal (fossa); mkg = Meckelian groove; nuf = nutrient foramen; nvf = neurovascular foramen; pdf = predentary facet; pdp = predentary process; sym = symphyseal surface. Scale bars = 10 mm.

Figure 23. Victorian ornithopodan dentary morphotype 3 (Ornithopoda indet.), right dentary (NMV P231182) and schematics in ventral (1), lateral (2), medial (3), and dorsal (4) views. Dashed lines in (2–4) indicates damaged ventral margin. alv = alveolus; bur = buccal ridge; cop = coronoid process; cor = coronoid ridge; cos = coronoid shelf; d# = dentary tooth position (from anterior end) and replacement number; dfo = anterior dentary foramen; ldp = lateral dentary parapet; mkc = Meckelian canal (fossa); mkg = Meckelian groove; nvf = neurovascular foramen; pdf = predentary facet; pdp = predentary process; sym = symphyseal surface. Scale bar = 10 mm.

Figure 24. Dentition of Victorian ornithopodan dentary morphotype 3 (Ornithopoda indet.), with comparisons: (1) left dentition of NMV P228408 (reversed) in lingual view; (2) right dentition of NMV P199135 in lingual view; (3) isolated left tooth NMV P208506 (reversed) in lingual view; (4, 5) isolated left tooth NMV P210049 (reversed) in lingual (4) and labial (5) views with schematic shading shown on occlusal facet in (5); (6) schematic right dentary tooth of Victorian ornithopodan dentary morphotype 3, based on composite of specimens at a midtooth row position; (7) isolated right dentary tooth, QM F52774, of an indeterminate neornithischian from the Winton Formation, central western Queensland. 1abr = primary apicobasal ridge; 2abr = secondary apicobasal ridge; abr = apicobasal ridge; cft = cleft; cv = cingular vertex; cvr = convergent (secondary) ridge; d# = dentary tooth position (from anterior end) and replacement number; dbr = distal bounding ridge; dcl = denticle; dep = depression; gch = growth channel; ldc = lingulate denticle; mbr = mesial bounding ridge; ocf = occlusal facet; rt = root; sul = sulcus. Scale bars = 5 mm.
Occurrence
Flat Rocks locality in the Inverloch region of Victoria, southeastern Australia (Fig. 1); Flat Rocks Sandstone, upper Barremian of the Wonthaggi Formation in the Gippsland Basin.
Materials
NMV P199135, complete but degraded right dentary with partial dentition; NMV P208506, worn left dentary tooth with root; NMV P210049, worn, incomplete left dentary tooth; NMV P228408, complete left dentary with partial dentition; NMV P231182, incomplete, degraded right dentary, lacking coronoid process and dentition; and NMV P208192, incomplete, degraded right dentary with partial dentition.
Remarks
The dentaries are hereafter referred to as ‘Victorian ornithopodan dentary morphotype 3’ (VOD3). They have up to 13 alveoli, contrasting with 10 in Qantassaurus intrepidus and VOD2. Viewed lateromedially, the ventral margin is shallowly convex in the posterior half and slightly procurved ventrally toward the symphyseal margin (Fig. 22). On the smaller dentaries (Fig. 22), the alveolar margin is slightly concave in the posterior half and the anterior portion procurved toward the predentary process. However, on the largest dentary (NMV P231182; Fig. 23), the margin is dorsally concave over its length. The predentary process is symmetrically triangular, as in Q. intrepidus and VOD2, with the dorsal and ventral facets for the predentary slightly concave and grooved. Viewed dorsally, the dentary ramus and alveolar margins are laterally concave/medially convex and the line of the anterior alveoli is convergent with the lateral margin of the dentary (Figs. 22–23). The posteriormost alveolus is anteromedial to the base of the coronoid process. Although the dorsalmost portions of the lateral alveolar parapets on the two dentaries are missing, the parapet was unlikely to have been elevated dorsally to the extent evident in Q. intrepidus. The coronoid process, best preserved on NMV P228408, is anteroposteriorly narrow and angled at ~ 45° relative to the alveolar margin. The coronoid processes on the dentaries of Q. intrepidus and VOD2 are more steeply angled (~ 70°; Figs. 19, 21). The dorsal end of the coronoid process is slightly expanded and rounded and is located dorsally above the apical level of the dentition (Fig. 22). The posteriorly directed medial coronoid cusp forms a small shelf at the base of the coronoid process (Fig. 22.3). The anteroposterior length of the coronoid process head is ~ 8% of the total alveolar length. In contrast, this ratio is almost double (~ 15%) in VOD2 (NMV P198962; Fig. 21.1, 21.2). Laterally, the short coronoid ridge merges with the lateral dentary parapet (Figs. 22, 23). The buccal ridge is smoothly rounded and lacking the ventral depression present in Q. intrepidus and VOD2 (Figs. 19, 21). The anterior dentary foramen is located centrally on the premaxillary process and a row of four neurovascular foramina penetrates the lateral alveolar parapet roughly corresponding to the line of the coronoid ridge (Figs. 22, 23). Two smaller foramina are posterior and slightly ventral to the anterior dentary foramen. Two further foramina form a row dorsally near the anterior end of the alveolar margin. Medially, the alveolar groove differentiates the medial dental parapet and the dentary ramus (Fig. 22.3). Nutrient foramina within the groove correspond to the alveoli. The Meckelian canal forms an elongate triangular opening in the posterior half of the dentary (Figs. 22, 23). The angle of the Meckelian canal opening of 15° is half that of Q. intrepidus and VOD2 (~ 30°). A narrow Meckelian groove continues anteriorly and extends onto the dorsomedial surface of the predentary process adjacent to the symphyseal margin. The dentary is more elongate than that of Q. intrepidus and VOD2 (Table 4).
Most of the erupted teeth are missing on the dentaries that retain dentition (NMV P199135, P208192 [not figured], P228408) and only the apical regions of some unerupted crowns are exposed (Figs. 22, 24). The isolated teeth provide additional information although none are without wear. The root is swollen with a roughly rectangular section, as in Zalmoxes (Weishampel et al., Reference Weishampel, Jianu, Csiki and Norman2003; Godefroit et al., Reference Godefroit, Codrea and Weishampel2009) and on some teeth forms a slight constriction near the crown base. The lingual surface of the root has a slight depression (Fig. 24). The roots of Qantassaurus intrepidus and VOD2 teeth cannot be presently compared with VOD3, because none are exposed and isolated teeth have not been categorically identified. Lingually, the cingulum is distinct from the root (Fig. 24). Although a complete unworn crown is presently unknown, the collection of specimens indicates that the crown is spatulate, with a mesiodistal width approximately equalling the apicobasal depth (Fig. 24.6). In contrast, the crowns of Q. intrepidus are relatively taller apicobasally, with a deeper basal region (Fig. 20.5). Lingually, the mesial and distal bounding ridges on the crowns of VOD3 form an asymmetrically U-shaped to shallowly W-shaped cingular vertex, with the cingulum mesiobasally sloping (Fig. 24). On some crowns, the base of the mesial bounding ridge is bulbous. The primary ridge, present lingually, expands toward its base and is offset distally from the central axis of the crown. The primary ridge on many of the crowns is obliquely oriented and roughly parallels the distal bounding ridge. On some crowns, clefts are formed between the primary ridge and the mesial and distal bounding ridges, as in Q. intrepidus (Figs. 20.5, 24.6). The lingual secondary ridges are closely abutting. Five are developed on the mesial lobe, two of which are convergent with the primary ridge, and at least three are developed on the distal lobe, with at least one convergent with the primary ridge. At least one lingulate denticle is further developed on the mesialmost apical margin, and one lingulate denticle also developed on the equivalent distal margin. Apicobasal ridges separated by channels are developed on the labial crown surfaces of the isolated crowns (NMV P208526, P210049; Fig. 24.5, 24.6), as in Q. intrepidus.
The crowns of VOD3 resemble those of an isolated ornithopod tooth (QM F52774; Fig. 24.7) described by Hocknull and Cook (Reference Hocknull and Cook2008) from the upper Albian of the Winton Formation in central-western Queensland. Although originally reported as a right maxillary tooth, the morphology of QM F52774 is consistent with a right dentary tooth.
Variation
The largest of the VOD3 dentaries (NMV P231182; Fig. 23) differs from the other dentaries (NMV P199135, P208192 [not figured], P228408; Fig. 22) by having more anteriorly divergent alveolar and ventral margins. In this aspect, the alveolar margin on NMV P231182 is more concave than on the other dentaries. The posteromedial end of the symphyseal margin on NMV P231182 forms a bulbous process, not present on the other dentaries. If of the same taxon, variation between NMV P231182 and the other VOD3 dentaries could result from age-related dimorphism, however, taxonomic variation is also possible.
Phylogenetic analysis
Dataset and search methods
The phylogenetic relationships of Australian ornithopods were assessed in TNT 1.5 (Goloboff and Catalano, Reference Goloboff and Catalano2016) using the dataset of Dieudonné et al. (Reference Dieudonné, Tortosa, Torcida Fernández-Baldor, Canudo and Díaz-Martínez2016) in a substantially modified form. Two principal search methodologies, ‘traditional’ equal weighting (EW) and implied weighting (IW), were employed. Implied weighting methodology seeks to reduce the effect of homoplastic information in the dataset and acts to produce a consensus with comparatively fewer polytomies (Goloboff, Reference Goloboff2014; Congreve and Lamsdell, Reference Congreve and Lamsdell2016; Goloboff et al., Reference Goloboff, Torres and Arias2018). The degree of down-weighting in an IW analysis is governed by a chosen concavity constant (k) (Goloboff, Reference Goloboff1993). The outcome of the search is dependent on the amount of homoplasy inherent in the data (Goloboff, Reference Goloboff2014) and size of the dataset (Goloboff et al., Reference Goloboff, Torres and Arias2018). A concavity constant of k = 7.00 was applied to the implied weighted search in this work (see Text S1 for further details). Because recent assessments of ornithischian phylogeny have produced highly unresolved strict consensus results based on generally similar underlying character data (e.g., Butler et al., Reference Butler, Upchurch and Norman2008, Reference Butler, Jin, Jun and Godefroit2011; Boyd, Reference Boyd2015; Madzia et al., Reference Madzia, Boyd and Mazuch2018), it was expected that new analyses with highly incomplete taxa would produce similarly poor results, if not worse resolution. To this end, the application of IW is a result-optimization procedure and in this respect, akin to supplementary analyses (e.g., maximum agreement subtrees, reduced consensus) utilized in other works, which typically follow retrieval of a strict consensus. However, unlike such methods, an IW search has the advantage of utilizing the full dataset without a posteriori pruning of taxa, and accordingly, its consensus tree is directly comparable to a ‘traditional’ EW strict consensus tree (i.e., allowing a one-on-one comparison).
Character matrix
A previous attempt to assess the phylogeny of Australian ornithopods within the dataset of Dieudonné et al. (Reference Dieudonné, Tortosa, Torcida Fernández-Baldor, Canudo and Díaz-Martínez2016; 288 characters) obtained poor resolution (Herne et al., Reference Herne, Tait, Weisbecker, Hall, Nair, Cleeland and Salisbury2018, supplemental data). To revivify this dataset, we revised character codings, amended several character scores, and augmented the dataset with new characters. From the original dataset, 34 characters were deleted and 48 novel or replacement characters were added (all modifications are detailed in the character list; see Text S2). Revision of the dataset was undertaken in Mesquite v. 3.40 (Maddison and Maddison, Reference Maddison and Maddison2009), with inapplicable states actively scored as ‘–’ rather than ‘?’ (Brazeau, Reference Brazeau2011). The resulting dataset comprised 302 active characters, all of which were unordered (including multistate characters that were ordered in previous works). Several of the compound characters in the original dataset were recoded into multiple contingent characters (via contingent coding methods, Forey and Kitching, Reference Forey, Kitching, Scotland and Pennington2000; Brazeau, Reference Brazeau2011). In highly incomplete fossil taxa, it is seldom known if a multistate morphology naturally transforms step-wise and linearly (Hauser and Presch, Reference Hauser and Presch1991). Thus, all remaining compound multistate characters were set as unordered, because ordering characters without in-depth a priori evolutionary understanding of that morphology (e.g., using genetic data) potentially imposes incorrect assumptions.
Operational taxonomic units (OTUs)
The dataset comprises 56 terminal taxa, with Herrerasaurus ischigualastensis Reig, Reference Reig1963 set as the outgroup. In addition to the original OTUs in the Dieudonné et al. (Reference Dieudonné, Tortosa, Torcida Fernández-Baldor, Canudo and Díaz-Martínez2016) dataset, new OTUs included Diluvicursor pickeringi (see Herne et al., Reference Herne, Tait, Weisbecker, Hall, Nair, Cleeland and Salisbury2018), Galleonosaurus dorisae n. gen. n. sp., Leaellynasaura amicagraphica (see Rich and Rich, Reference Rich and Rich1989), Owenodon hoggii (see Norman and Barrett, Reference Norman and Barrett2002; Galton, Reference Galton2009), Qantassaurus intrepidus (see Rich and Vickers-Rich, Reference Rich and Vickers-Rich1999), and five marginocephalians (Homalocephale calathocercos Maryanska and Osmolska, Reference Maryanska and Osmolska1974, Stegoceras validum Lambe, Reference Lambe1902, Goyocephale lattimorei Perle, Maryanska, and Osmolska, Reference Perle, Maryanska and Osmolska1982, Liaoceratops yanzigouensis Xu et al., Reference Xu, Makovicky, Wang, Norell and You2002, and Protoceratops andrewsi Granger and Gregory, Reference Granger and Gregory1923 [Gilmore, Reference Gilmore1924; Brown and Schlaikjer, Reference Brown and Schlaikjer1940; Maryanska and Osmolska, Reference Maryanska and Osmolska1974; Perle et al., Reference Perle, Maryanska and Osmolska1982; Galton and Sues, Reference Galton and Sues1983; Xu et al., Reference Xu, Makovicky, Wang, Norell and You2002; Tanoue et al., Reference Tanoue, You and Dodson2009; He et al., Reference He, Makovicky, Xu and You2018]). The addition of the marginocephalians arose out of the need to stabilize Cerapoda, identified as a problematically volatile node within a previous analysis (Herne et al., Reference Herne, Tait, Weisbecker, Hall, Nair, Cleeland and Salisbury2018). Othnielosaurus consors Marsh, Reference Marsh1894 was replaced by Nanosaurus agilis with augmented scores following recent systematic revision (Carpenter and Galton, Reference Carpenter and Galton2018), whereas ‘Dryosaurus altus’ was relabelled Dryosaurus spp., after establishment of the new species Dryosaurus elderae. Following re-evaluation of Pisanosaurus mertii Casamiquela, Reference Casamiquela1967 as a potential nondinosaurian (Agnolín and Rozadilla, Reference Agnolín and Rozadilla2018), this taxon was removed.
Heuristic searches
The analyses (EW, IW) were conducted with the maximum tree space pre-set to 200,000 and zero-length branches collapsed. These each comprised 1,000 tree bisection and reconnection replicates, with 10 trees held per replicate. The strict consensus from EW was augmented by standard bootstrap and Bremer values (see details in Text S1). Bootstrapping comprised 1,000 pseudoreplications, reported as ‘frequency distribution’ values (rather than ‘absolute frequencies’) to factor-in resampling that contradicts recovered clades in the consensus (Goloboff et al., Reference Goloboff, Farris, Källersjö, Oxelman, Ramirez and Szumik2003). Bremer support was calculated by running the TNT script ‘bremer.run,’ surveying trees up to five steps longer than the most parsimonious tree (MPT). Node support for the IW strict consensus utilized symmetric resampling (p = 33), which provides a more appropriate support measure for a weighted analysis than bootstrapping (Goloboff et al., Reference Goloboff, Farris, Källersjö, Oxelman, Ramirez and Szumik2003). Symmetric resampling comprised 1,000 pseudoreplications that were also reported as frequency distributions. Because of the way in which the IW MPT tree-fit measure is yielded, the resulting IW Bremer support values are reported as fractions rather than as integers (Text S1).
Consensus methods
Maximum agreement subtrees were obtained to present the more resolved ‘backbone’ topologies for each search following the removal of taxa considered unstable, via their conflicting positions (Kitching et al., Reference Kitching, Forey, Humphries and Williams1998). Further identification of wildcard taxa was made by applying an iterative reduced positional congruence index (PCR; Pol and Escapa, Reference Pol and Escapa2009) to the MPTs arising from each search. This procedure iteratively prunes taxa considered unstable, via their scored characters, while reporting successive improvements in the resolution of the consensus tree. Iterative PCR was assessed with the implementation of the original script (‘iterPCR.run’) available in TNT 1.5 (Goloboff and Szumik, Reference Goloboff and Szumik2015).
Heuristic search and reduced consensus results
Under EW, 814 MPTs were recovered, with tree lengths of 932 steps, with the resulting EW strict consensus tree being 1,103 steps in length (Fig. 25.1). Under IW, 26 MPTs were recovered, each with a best score (IW fit length) of 55.92 and tree length of 933 steps. The resulting IW strict consensus tree (Fig. 25.2) was 951 steps long (tree fit length 57.06). For both analyses, the consistency index is 0.37 and the retention index is 0.63. The EW strict consensus returned poor results in the relationships among almost all neornithischian OTUs (Fig. 25.1; see Text S1 for definitions). In the IW strict consensus tree, the predominantly Cretaceous Gondwanan neornithischians of interest to this work are recovered as nonclypeodontan (= ‘basal’) ornithopods, however, as part of a broad polytomy (Fig. 25.2).

Figure 25. Strict consensus cladograms, showing the relationships of Galleonosaurus dorisae n. gen. n. sp. and Qantassaurus intrepidus Rich and Vickers-Rich, Reference Rich and Vickers-Rich1999: (1) tree derived from EW analysis (814 MPTs); (2) tree derived from IW analysis (26 MPTs). For phylogenetic definitions of labelled nodes and stems, see Text S1. For Bremer and bootstrap support values accompanying each search, see Text S1. M = Mochlodon; Rh = Rhabdodon; T = Tenontosaurus; Z = Zalmoxes.
Efforts to retrieve a useful underlying topology with the raw EW trees using either method of determining a reduce consensus (i.e., agreement subtrees or iterative PCR) produced insufficient results. The maximum agreement subtree excluded a large number of OTUs (22), critically taxa of interest to this work, whereas iterative PCR produced more inferior results with most neornithischian OTUs unstable (Text S1). However, the maximum agreement subtree arising from the MPTs of the initial IW search pruned only two OTUs with unstable positions (Qantassaurus intrepidus and Diluvicursor pickeringi; Fig. 26.1). As a consequence of the excluded taxa, the basal ornithopod polytomy in the IW strict consensus became resolved as a clade of entirely Gondwanan OTUs—the Elasmaria (see Text S1 for definition)—that is sister to the remaining ornithopods (Clypeodonta). Iterative PCR of the IW MPTs also identified Q. intrepidus and Diluvicursor pickeringi as wildcard taxa (see Text S1 for details), and in effect corroborated the ‘backbone’ topology (IW maximum agreement subtree) of ornithopods.

Figure 26. Reduced consensus topologies, showing the relationships of Galleonosaurus dorisae n. gen. n. sp.: (1) maximum agreement subtree derived from 26 original IW MPTs (2 OTUs pruned: Qantassaurus and Diluvicursor); (2) strict consensus tree derived from IW analysis following a priori exclusion of wildcard taxa indicated by iterative PCR (i.e., Qantassaurus and Diluvicursor); (3) strict consensus tree derived from IW analysis following a priori exclusion of Qantassaurus only. For simplicity, clades peripheral of the region of interest, as labelled in Fig. 25.2, were collapsed (they did not vary in taxic composition between the analyses). For phylogenetic definitions, see Text S1.
Taking forward these results, an IW re-analysis of the dataset, with the iterative PCR wildcard taxa excluded a priori, produced seven MPTs, the strict consensus of which recovered an internally-unresolved Elasmaria within Ornithopoda as the sister clade to Clypeodonta (Fig. 26.2). When only Qantassaurus intrepidus was excluded, a similar overall topology was produced, differing only by its more internally resolved elasmarian node (Fig. 26.3). The differences between these topologies and the IW agreement subtree, and even to the original strict consensus, resulted from changes in the resolution of the elasmarian OTUs. Clearly, the stability of the elasmarian node, when recovered, was determined largely by the composition of the taxa retained. It is unsurprising that the majority of the pruned neornithischian OTUs, using either EW or IW method, are those associated with > 85% missing data (Text S1). Although obvious, it should not be understated how improved support for the lineages of interest will only be achieved when more complete materials become available.
Major neornithischian clades and interrelationships
The IW strict consensus tree (Figs. 25.2, 26.2) is the most resolved and provides our preferred hypothesis for the discussion of neornithischian interrelationships that follows. Lesothosaurus diagnosticus was recovered as the basalmost branching neornithischian, below Agilisaurus louderbacki Peng, Reference Peng1990, Hexinlusaurus multidens He and Cai, Reference He and Cai1983, and Yandusaurus hongheensis He, Reference He1979. A major split among more nested neornithischians occurs between Cerapoda and an unnamed clade that comprises taxa closer to Thescelosaurus neglectus. The Late Jurassic taxon Nanosaurus agilis diverges at the base of this latter clade and is the sister taxon to an entirely Cretaceous subgroup of taxa comprising the East Asian and largely North American clades Jeholosauridae and Thescelosauridae, respectively (Han et al., Reference Han, Barrett, Butler and Xu2012; Brown et al., Reference Brown, Evans, Ryan and Russell2013). Within Cerapoda, Marginocephalia and its internal branches are well supported (Text S1), however, the interrelationships of taxa at the base of Ornithopoda are weakly supported. Parksosaurus warreni Parks, Reference Parks1926 is recovered as the basalmost ornithopod, which contrasts to some previous analyses in which P. warreni was recovered allied to Thescelosaurus neglectus outside of Cerapoda (Brown et al., Reference Brown, Evans, Ryan and Russell2013; Boyd, Reference Boyd2015). The remaining ornithopods form an unnamed polytomy, which comprises all eight Cretaceous Victorian and South American OTUs and the clade Clypeodonta (Fig. 25.2). This last clade is split between Hypsilophodon foxii and the remaining taxa attributable to Iguanodontia. Rhabdodontidae forms the sister clade to all other iguanodontians, comprising the progressively more nested lineages of Muttaburrasaurus langdoni, Tenontosaurus spp., and Dryomorpha. Notably, M. langdoni is not allied with rhabdodontids (contra Dieudonné et al., Reference Dieudonné, Tortosa, Torcida Fernández-Baldor, Canudo and Díaz-Martínez2016), limiting the latter group to a European Cretaceous distribution.
The interrelationships of the Victorian and South American taxa included in the analysis are further revealed by the reduced consensus results (Fig. 26). Excluding Qantassaurus intrepidus as a wildcard, these taxa are recovered in the clade Elasmaria. Within this assemblage of taxa, Talenkauen santacrucensis regularly appeared to be the basalmost branch, and sister to the remaining taxa (Fig. 26.1, 26.3). Galleonosaurus dorisae n. gen. n. sp. clusters with Leaellynasaura amicagraphica, Anabisetia saldiviai, and Diluvicursor pickeringi (when included) as the sister taxon to Gasparinisaura cincosaltensis and Macrogryphosaurus gondwanicus Calvo, Porfiri, and Novas, Reference Calvo, Porfiri and Novas2007 (Fig. 26.3).
Discussion
Validity and distribution of the Victorian ornithopod taxa
Galleonosaurus dorisae n. gen. n. sp., from the upper Barremian Wonthaggi Formation, is the fourth Victorian ornithopod named from cranial remains and the third described from the maxilla (Figs. 4–9, 27; Table 5). The two other Victorian taxa known from their maxillae, Atlascopcosaurus loadsi (Figs. 10, 13) and Leaellynasaura amicagraphica, are from the younger, early Albian Eumeralla Formation to the west of the Wonthaggi Formation (Figs. 1.2, 27; Table 5). Ornithopods of the Eumeralla and Wonthaggi formations could be separated by ~ 12–17 Myr (based on dating of the Eumeralla Formation by Korasidis et al. [Reference Korasidis, Wagstaff, Gallagher, Duddy, Tosolini, Cantrill and Norvick2016] and revised dating of the Wonthaggi Formation by B. Wagstaff [personal communication, 2018]). The third-named ornithopod from Victoria, Qantassaurus intrepidus from the Wonthaggi Formation (Fig. 27; Table 5), is known only from its dentary. However, at least two isolated teeth from the Eumeralla Formation (NMV P177934, P186426) also resemble those of Q. intrepidus (Fig. 20.5–20.8). It is of note that one of these teeth (NMV P177934) was originally assigned to Atlascopcosaurus loadsi (Rich and Rich, Reference Rich and Rich1989, fig. 11B).
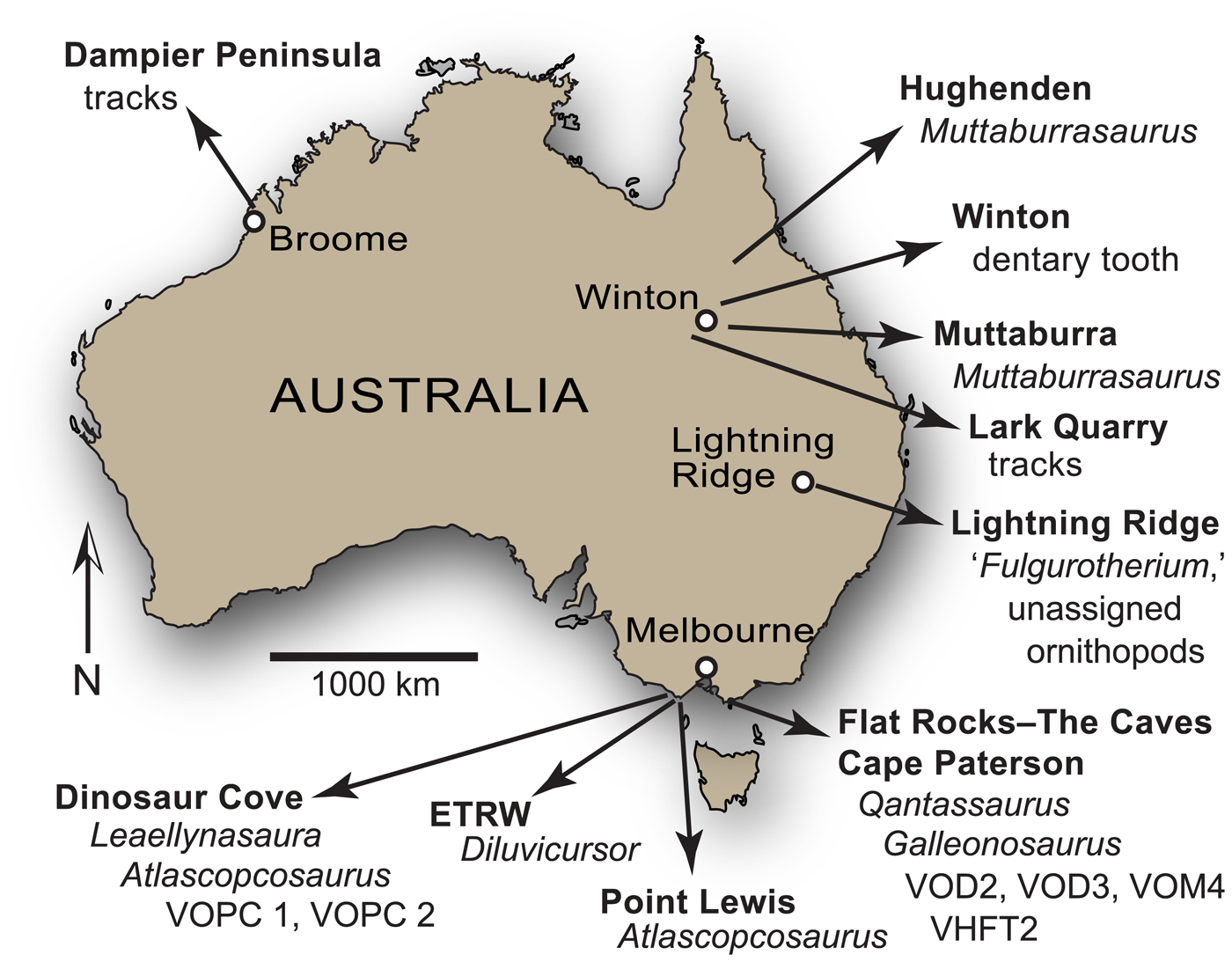
Figure 27. Australian ornithopod occurrences: ETRW = Eric the Red West; VHFT2 = Victorian Hypsilophodontid Femur Type 2; VOD2 = Victorian ornithopod dentary morphotype 2; VOD3 = Victorian ornithopod dentary morphotype 3; VOM4 = Victorian ornithopod maxilla morphotype 4; VOPC1 = Victorian ornithopod postcranium 1 (NMV P185992/P185993); VOPC2 = Victorian ornithopod postcranium 2 (NMV P186047). See Table 5 for associated information on ornithopod occurrences.
Table 5. Distribution of Australian ornithopods. VHFT2 = Victorian hypsilophodontid femur type 2; VOD2 = Victorian ornithopod dentary morphotype 2; VOD3 = Victorian ornithopod dentary morphotype 3; VOM4 = Victorian ornithopod maxilla morphotype 4; VOPC1 = Victorian ornithopod postcranium type 1; VOPC2 = Victorian ornithopod postcranium type 2.
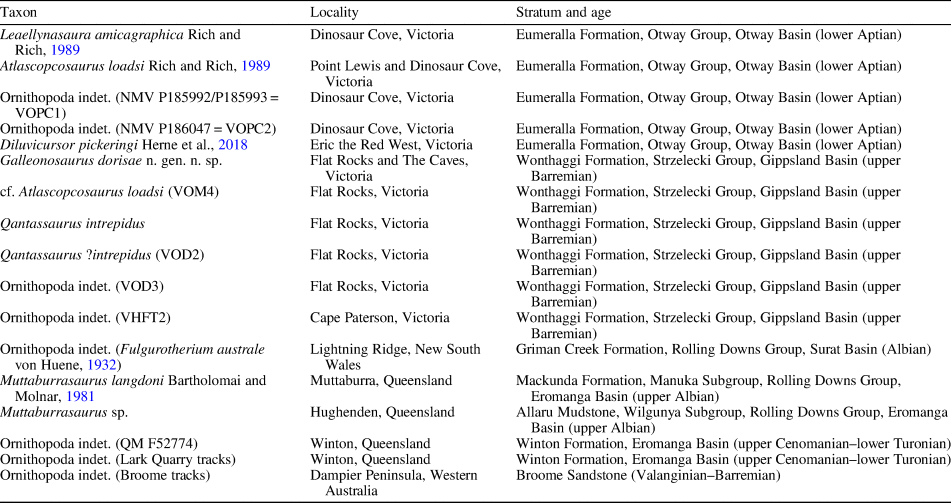
Galleonosaurus dorisae n. gen. n. sp. shares a combination of seven maxillary tooth crown features with Atlascopcosaurus loadsi and Leaellynasaura amicagraphica (Fig. 17) that suggest a close relationship between these three taxa (i.e., maxillary crowns features G1–7 in Remarks under Galleonosaurus dorisae n. gen. n. sp.). Most of the maxillary crown features of Galleonosaurus dorisae n. gen. n. sp. are also shared with the large-bodied Albian ornithopod from Queensland, Muttaburrasaurus spp. (Fig. 17). The phylogenetic relationships of Muttaburrasaurus spp. and the Victorian ornithopods will be discussed further below. It is notable that the tridenticulate mamillated cusps developed on the crowns of Galleonosaurus dorisae n. gen. n. sp. and Atlascopcosaurus loadsi have been previously reported in Nanosaurus agilis (Bakker et al., Reference Bakker, Galton, Siegwarth and Filla1990; Carpenter and Galton, Reference Carpenter and Galton2018). Tridenticulate cusps, however, are unknown on the maxillary crowns of L. amicagraphica and Muttaburrasaurus spp.
The maxilla of Galleonosaurus dorisae n. gen. n. sp. differs from that of Leaellynasaura amicagraphica by having: (1) deeper buccal emargination; (2) greater lateral concavity along the tooth row, with distinct outturning of the anterior alveoli; (3) a smoother buccal ridge, as opposed to sharply rounded; and (4) a stronger primary ridge, more distally offset on the labial crown surface (Figs. 5, 7, 8, 17). In addition, the largest specimens of Galleonosaurus dorisae n. gen. n. sp. also have a greater number of maxillary teeth than the L. amicagraphica holotype (15 compared to 12), although this difference could be attributable to ontogeny (e.g., Barrett and Han, Reference Barrett and Han2009) because the L. amicagraphica holotype is almost certainly a juvenile (see also Rich and Rich, Reference Rich and Rich1989). Galleonosaurus dorisae n. gen. n. sp. differs from Atlascopcosaurus loadsi by having: (1) a dorsoventrally lower maxillary ramus, (2) lesser protrusion of posterolateral process on the maxilla, (3) a more broadly rounded buccal ridge, (4) a symmetrically expanded flange on the lateral ramus of the palatine (rather than being asymmetrical and posteriorly expanded), (5) more finely developed secondary apicobasal ridges distal to the primary ridge, and (6) a markedly V-shaped (as opposed to U-shaped) cingular vertex on the maxillary crowns (Figs. 13, 17). These differences between Atlascopcosaurus loadsi, Galleonosaurus dorisae n. gen. n. sp., and L. amicagraphica support their taxonomic separation.
The two Wonthaggi Formation ornithopods—Galleonosaurus dorisae n. gen. n. sp., known from its maxilla, and Qantassaurus intrepidus, known from its dentary—are potentially synonymous. However, separation of these two Flat Rocks locality taxa is indirectly supported by the newly discovered ornithopod dentary morphotype VOD3 from the Flat Rocks site (Figs. 22–24, 27; Table 5). VOD3 is a more elongate dentary than that of Q. intrepidus and contains more alveoli in specimens of similar size (13 alveoli compared with 10 in Q. intrepidus). The presence of two distinctly differing ornithopod dentary morphotypes from the Flat Rocks deposit is therefore confirmed by VOD3, the other being Q. intrepidus. Importantly, we consider the number of dentary alveoli in VOD3 closer to the expected number in the presently unknown dentary of Galleonosaurus dorisae n. gen. n. sp., the maxilla of which has 15 alveoli. In comparable neornithischians, the number of dentary alveoli relative to the maxillary alveoli varies. The dentaries of some taxa contain more alveoli than the maxillae (e.g., 14 to 12 in Hypsilophodon foxii [see Galton, Reference Galton1974]), some have fewer (e.g., 13 to 15 in Jeholosaurus shangyuanensis [see Barrett and Han, Reference Barrett and Han2009] and Dryosaurus elderae [CM 3394], and 12 to 14 in Dysalotosaurus lettowvorbecki [see Janensch, Reference Janensch1955]) and some have an equivalent number (e.g., 20 in Thescelosaurus neglectus [see Boyd, Reference Boyd2014] and 10 in Zalmoxes robustus [see Weishampel et al., Reference Weishampel, Jianu, Csiki and Norman2003]). Therefore, typically in neornithischians, the dentaries contain either the same number of alveoli as the maxillae, or vary up or down by approximately two alveoli. With 13 alveoli, we consider VOD3 more congruent to the 15 maxillary alveoli in Galleonosaurus dorisae n. gen. n. sp. than the foreshortened dentary of Q. intrepidus, containing 10 alveoli.
No dentary from the Eumeralla Formation is presently assignable to Atlascopcosaurus loadsi or Leaellynasaura amicagraphica (noting that dentaries originally referred to Atlascopcosaurus loadsi by Rich and Rich [1989] were not found in association with any of the Atlascopcosaurus loadsi maxillae, and for this reason cannot be confidently assigned to that taxon). Therefore, future potential synonymy between Qantassaurus intrepidus and either Atlascopcosaurus loadsi or L. amicagraphica cannot be ruled out. However, out of Atlascopcosaurus loadsi and L. amicagraphica, the robust dentary form of Q. intrepidus seems a more likely candidate for the maxilla of Atlascopcosaurus loadsi, which appears to be relatively more robust than the maxilla of L. amicagraphica (see Fig. 10). The possibility that the range of Q. intrepidus extends to the Eumeralla Formation is strengthened by the presence of two isolated dentary teeth (NMV P177934, P186426) from Dinosaur Cove that closely resemble the teeth of Q. intrepidus (Fig. 20.3–20.8).
If temporal separation of the Wonthaggi and Eumeralla formations by ~ 12 Myr was considered a parameter supporting taxic separation of the ornithopods between these formations, the question of Q. intrepidus validity would diminish. This view finds support from the work of Dodson (Reference Dodson1990), who tentatively estimated that the mean duration of dinosaur genera might have been ~7.7 Myr. However, owing to the limited exposure of strata in the Eumeralla and Wonthaggi formations and the typically fragmentary and isolated nature of the fossils preserved, the nature of turnover and the duration of ornithopod genera across these formations is unlikely to be confidently ascertained in the immediate future.
We are presently uncertain whether the maxillary morphotype VOM4 (i.e., cf. Atlascopcosaurus loadsi) from the Wonthaggi Formation is categorically separable from the Eumeralla Formation genus Atlascopcosaurus (Figs. 17, 18). The U-shaped cingular vertex and ornamentation on the labial surfaces of the VOM4 maxillary tooth crowns are closer to those of Atlascopcosaurus loadsi than those of Galleonosaurus dorisae n. gen. n. sp. (Fig. 17). Given its older age, however, VOM4 is potentially precursory to Atlascopcosaurus loadsi. A further dentary form, identified in this work as VOD2 (= Qantassaurus ?intrepidus; Figs. 21, 27; Table 5), includes specimens (NMV P198962, P199087; Fig. 21) originally assigned to Qantassaurus intrepidus by Rich and Rich (Reference Rich and Vickers-Rich1999). According to these authors, the ‘bloated’ appearance of one of the specimens (NMV P198962) was potentially indicative of a pathological condition. However, the morphological similarity between the two VOD2 specimens alternatively suggests that this bloated form is not isolated to one individual and could represent dimorphism in Q. intrepidus, or alternatively, a feature of a separate but closely related taxon.
Originally referred to Leaellynasaura amicagraphica as a part of the holotype (Rich and Rich, Reference Rich and Rich1989), assignment of the partial postcranial skeleton NMV P185992/P185993 (=‘Victorian ornithopod postcranium type 1’ [VOPC1, herein]; Herne, Reference Herne2014) from Dinosaur Cove (Figs. 1.2, 27) is presently uncertain (Herne et al., Reference Herne, Tait and Salisbury2016). These remains, as well as the second partial postcranium from the same locality (NMV P186047 =‘VOPC2’ [based on Herne, Reference Herne2014]) could pertain to L. amicagraphica. However, taphonomic assessment of this locality also suggests that these two postcrania could alternatively pertain to Atlascopcosaurus loadsi, which has been identified at the same locality, or a separate taxon or taxa (Herne et al., Reference Herne, Tait and Salisbury2016). Diluvicursor pickeringi is potentially synonymous with any one of Atlascopcosaurus loadsi, L. amicagraphica, Galleonosaurus dorisae n. gen. n. sp., or Qantassaurus intrepidus. However, synonymy could only be determined from future discoveries of anatomically overlapping materials. ‘Victorian Hypsilophodontid Femur Type 2’ (Rich and Rich, Reference Rich and Rich1989) from Cape Paterson in the Wonthaggi Formation (Figs. 1.2, 27; Table 5) seems to represent a larger ornithopodan body form than inferred from the materials of the presently named Victorian ornithopods.
In summary, five ornithopod taxa have been named from the Early Cretaceous of Victoria (Figs. 1.2, 27; Table 5), including: Leaellynasaura amicagraphica and Atlascopcosaurus loadsi from the Eumeralla Formation, known from their maxillae; Qantassaurus intrepidus from the Wonthaggi Formation, known from its dentary; Diluvicursor pickeringi from the Eumeralla Formation, known from a partial postcranium (Herne et al., Reference Herne, Tait, Weisbecker, Hall, Nair, Cleeland and Salisbury2018); and Galleonosaurus dorisae n. gen. n. sp. from the Wonthaggi Formation, known from its maxilla. Synonymy between some of these taxa is possible but could only be ascertained from the discovery of more complete specimens in the future. Three jaw morphotypes are further identified (VOD2 [Q. ?intrepidus], VOD3 and VOM4 [cf. Atlascopcosaurus loadsi]). Associations between these morphotypes and the named Victorian ornithopods are presently uncertain. However, we consider that the dentary morphotype VOD3 a reasonable candidate for the presently unknown dentary of Galleonosaurus dorisae n. gen. n. sp. The large femoral morphotype (Victorian Hypsilophodontid Femur Type 2) potentially represents an additional taxon of Victorian ornithopod.
Phylogenetic affinities of the Victorian ornithopods
The strict consensus tree from the initial IW searches, with all taxa included, produced a polytomy near the base of Ornithopoda, above the branch of Parksosaurus warreni. Following a priori removal of Qantassaurus intrepidus, which was identified by iterative PCR as a wildcard taxon, a Gondwanan clade was subsequently recovered (Fig. 25.2), consistent with the revised definition of Elasmaria (Text S1; see also Calvo et al., Reference Calvo, Porfiri and Novas2007) as all taxa closer to Macrogryphosaurus gondwanicus and Talenkauen santacrucensis than to Hypsilophodon foxii or Iguanodon bernissartensis (see also Rozadilla et al., Reference Rozadilla, Agnolin, Novas, Aranciaga Rolando, Motta, Lirio and Isasi2016). Elasmaria was originally recovered within Iguanodontia (Calvo et al., Reference Calvo, Porfiri and Novas2007), but more recently as a polytomous clade of Argentinian and Antarctic nonhadrosaurian ornithopods, sister to Gasparinisaura cincosaltensis, which together, formed an unnamed clade nested within Ornithopoda (Rozadilla et al., Reference Rozadilla, Agnolin, Novas, Aranciaga Rolando, Motta, Lirio and Isasi2016). The analysis by Rozadilla et al. (Reference Rozadilla, Agnolin, Novas, Aranciaga Rolando, Motta, Lirio and Isasi2016) recovered an ornithopod topology substantially differing from ours, particularly in its inclusion of Thescelosaurus neglectus within Ornithopoda, Hypsilophodon foxii as the basalmost ornithopod, and P. warreni and all other ornithopods as sister to the aforementioned unnamed Gondwanan clade. However, it is notable that the Antarctic taxa Morrosaurus antarcticus Rozadilla et al., Reference Rozadilla, Agnolin, Novas, Aranciaga Rolando, Motta, Lirio and Isasi2016 and Trinisaura santamartaensis Coria et al., Reference Coria, Moly, Reguero, Santillana and Marenssi2013, included in the analysis by Rozadilla et al. (Reference Rozadilla, Agnolin, Novas, Aranciaga Rolando, Motta, Lirio and Isasi2016) and recovered as elasmarians, were excluded from our analysis.
The Early Cretaceous Victorian ornithopods, Diluvicursor pickeringi, Galleonosaurus dorisae n. gen. n. sp., and Leaellynasaura amicagraphica, along with Anabisetia saldiviai, Gasparinisaura cincosaltensis, Macrogryphosaurus gondwanicus, and Talenkauen santacrucensis from the Late Cretaceous of Argentina, were recovered herein within Elasmaria (Fig. 26). However, this grouping was too weakly supported at present to afford definitive systematic assignment of the Victorian taxa to Elasmaria. From the time-calibrated phylogeny, generated from the IW strict consensus (Fig. 28), Galleonosaurus dorisae n. gen. n. sp. represents the earliest elasmarian presently known. None of the elasmarian taxa, as recovered, possessed all of the synapomorphies identified over all nodes (see Text S1). However, the following three synapomorphies possessed by the two Victorian taxa Galleonosaurus dorisae n. gen. n. sp. and L. amicagraphica are of interest to this present investigation. These include having: (1) the apical end of the primary ridge labially on the maxillary crowns offset to the distal third of the crown surface (character 308), shared with Gasparinisaura cincosaltensis and Talenkauen santacrucensis; (2) the basal vertex on the maxillary crowns offset mesially relative to the central axis of the tooth root (character 309), not shared with any other elasmarian, although possibly present in Talenkauen santacrucensis; and (3) the apicobasal ridges labially on the maxillary crowns convergent in the direction of the primary ridge, toward the crown base (character 311), shared with Gasparinisaura cincosaltensis and Talenkauen santacrucensis. These three features, also present in Atlascopcosaurus loadsi, which was not included in the analysis owing to incompleteness, suggest that this taxon is likely to be an elasmarian.

Figure 28. Time-calibrated phylogeny of the ornithopods from the IW strict consensus cladogram (Fig. 25.2). Dashed lines indicate unknown times of Pangaean branch/lineage divergences prior to the middle Callovian. Time scale based on Cohen et al. (Reference Cohen, Finney, Gibbard and Fan2013). Thick lines indicate taxon (graduated shaded lines) and clade (solid lines) durations (for sources, see Text S1). Aal = Aalenian; AF = Africa; Alb = Albian; AN = Antarctica; Apt = Aptian; AU = Australia; Baj = Bajocian; Bar = Barremian; Bat = Bathonian; Ber = Berriasian; Cal = Callovian; Cam = Campanian; Cen = Cenomanian; Con = Coniacian; Hau = Hauterivian; Kim = Kimmeridgian; LA = Laurasia; Maa = Maastrichtian; NZ = New Zealand; Oxf = Oxfordian; SA = South America; San = Santonian; Tit = Tithonian; Tur = Turonian; Val = Valanginian.
In regard to the postcranially-based taxon Diluvicursor pickeringi, a T-shaped distal end profile on metatarsal I (character 334) is synapomorphically shared with Anabisetia saldiviai (see Herne et al., Reference Herne, Tait, Weisbecker, Hall, Nair, Cleeland and Salisbury2018). This feature is also present in the partial postcranium, VOPC1 (i.e., NMV P185992/P185993), from Dinosaur Cove. A further elasmarian synapomorphy, that of a proximally lunate, transversely compressed metatarsal II, at 50% or less than the width of metatarsal III (character 335), is synapomorphically shared among Anabisetia saldiviai, Diluvicursor pickeringi, and Gasparinisaura cincosaltensis (see Herne et al., Reference Herne, Tait, Weisbecker, Hall, Nair, Cleeland and Salisbury2018). This feature is also present in the aforementioned partial postcranium (VOPC1) and the other partial postcranium from Dinosaur Cove (VOPC2; i.e., NMV P186047).
The recovery of Muttaburrasaurus langdoni as a nondryomorphan iguanodontian signals a more complex history of ornithopod distributions extending to eastern Gondwana than that posed by the Gondwanan-centered Elasmaria (Fig. 28). This complexity is deepened further by the presence of an ilium (CD529) from the Campanian–Maastrichtian of New Zealand, tentatively regarded as a dryosaurid (Wiffen and Molnar, Reference Wiffen and Molnar1989; for age of specimen, see Text S1). Although dryosaurids are otherwise only known from Laurasia and Africa, this ilium strongly suggests that they also had an eastern Gondwanan distribution. Dryosaurids have not been positively identified from Australia yet, but their presence is possible. At least one isolated, although presently unassigned, ilium of an ornithischian from the Wonthaggi Formation (NMV P228444; figured by Rich, Reference Rich and Kool2010, p. 16; unpublished data, Herne, 2018), possessing features resembling those in Dryosaurus spp., Dysalotosaurus lettowvorbecki, and Valdosaurus canaliculatus Galton, Reference Galton1975, e.g., a low dorsoventral profile, a dorsoventrally ‘pinched’ postacetabular process, and a markedly triangular brevis shelf (see Janensch, Reference Janensch1955; Galton, Reference Galton1981; Galton and Taquet, Reference Galton and Taquet1982; Barrett et al., Reference Barrett, Butler, Twitchett and Hutt2011), support this possibility. However, it is important to note that these features are also present on the ilia of the elasmarians Anabisetia saldiviai, Gasparinisaura cincosaltensis, and Macrogryphosaurus gondwanicus.
Qantassaurus intrepidus was pruned from the secondary analyses, following its identification as wildcard. However, initial searches suggesting a basal position in Ornithopoda and comparisons of the dentary teeth indicating a close resemblance to those of Talenkauen santacrucensis, suggest that Q. intrepidus might be an elasmarian (Figs. 25.2, 28). However, the dentary teeth of Q. intrepidus, Talenkauen santacrucensis (see Cambiaso, Reference Cambiaso2007, fig. 17E, F), and the two isolated Q. intrepidus-like teeth from Dinosaur Cove (NMV P177934, P186426; Fig. 20) also resemble the singly known dentary tooth of Kangnasaurus coetzeei (Fig. 20) from the Late Cretaceous of South Africa (following de Wit et al., Reference de Wit, Ward and Spaggiari1992)—a taxon that has been considered a dryosaurid (Cooper, Reference Cooper1985; Barrett et al., Reference Barrett, Butler, Twitchett and Hutt2011). Although we did not include K. coetzeei in the dataset, primarily because of the problematic provenance of its materials (see also Haughton, Reference Haughton1915; Cooper, Reference Cooper1985), close resemblance among the dentary teeth of Q. intrepidus, Talenkauen santacrucensis, and the K. coetzeei-attributed tooth, suggests either the presence of an elasmarian in the Late Cretaceous of Africa (i.e., K. coetzeei), or that Q. intrepidus and Talenkauen santacrucensis might be closer to the dryosaurids than currently understood. This latter possibility finds support from the aforementioned ilium of a dryosaurid in New Zealand and the dryosaurid-like ilium from Victoria, as previously mentioned.
Some features of the dentition suggest that Muttaburrasaurus langdoni (and Muttaburrasaurus sp., QM F14921) could have closer affinities to the elasmarians than to the clypeodontans, as recovered. Firstly, multiple lingulate denticles along the apical margins of the cheek teeth (character 305) are lacking on the Muttaburrasaurus spp. crowns, but otherwise are present in all other clypeodontans, with the possible exception of Kangnasaurus coetzeei. This feature is also lacking in the elasmarians, Anabisetia saldiviai, Leaellynasaura amicagraphica, Galleonosaurus dorisae n. gen. n. sp., Gasparinisaura cincosaltensis, and Talenkauen santacrucensis, as well as on the dentary crowns of Qantassaurus intrepidus. Secondly, marked mesial offset of the cingular vertex on the maxillary tooth crowns was also recovered as autapomorphic in M. langdoni. However, even though the analysis failed to describe apomorphies in L. amicagraphica and Galleonosaurus dorisae n. gen. n. sp., this feature is also present in these two latter Victorian taxa. These two aforementioned features of Muttaburrasaurus spp. shared with elasmarians could be independently acquired, as the analysis suggests. However, we entertain the possibility that more complete data for the Victorian taxa could pull Muttaburrasaurus spp. and the elasmarians closer together than presently realized.
Several postcranial features variously possessed by elasmarian taxa are also possessed by the dryosaurids. Two of these features—a dorsoventrally low ilium (not included in the dataset) and a triangular brevis shelf (character 331), present in Anabisetia saldiviai (see Coria and Calvo, Reference Coria and Calvo2002), Gasparinisaura cincosaltensis (MCS-3; Salgado et al., Reference Salgado, Coria and Heredia1997; unpublished data, Herne, 2008), and Macrogryphosaurus gondwanicus (see Calvo et al., Reference Calvo, Porfiri and Novas2007)—were mentioned above. These features are also present in the four dryosaurids, Dryosaurus spp., Dysalotosaurus lettowvorbecki, Eousdryosaurus nanohallucis Escaso et al., Reference Escaso, Ortega, Dantas, Malafaia, Silva, Gasulla, Mocho, Narváez and Sanz2014, and Valdosaurus canaliculatus (Janensch, Reference Janensch1955; Galton, Reference Galton1981; Escaso et al., Reference Escaso, Ortega, Dantas, Malafaia, Silva, Gasulla, Mocho, Narváez and Sanz2014; Barrett, Reference Barrett2016), and also the iguanodontians Planicoxa venenica DiCroce and Carpenter, Reference DiCroce, Carpenter, Tanke and Carpenter2001 and Osmakasaurus depressus Gilmore, Reference Gilmore1909 (Carpenter and Wilson, Reference Carpenter and Wilson2008; McDonald, Reference McDonald2011) not included in this study. However, it is of note that the presence of a triangular brevis shelf (character 331) was not recovered as synapomorphic in any of the clades. In addition, two further features are of interest. A deep muscle scar for the m. caudofemoralis longus on the diaphysis of the femur, markedly inset from the base of the fourth trochanter (character 256), present in Anabisetia saldiviai (MCF-PVPH-75; unpublished data, Herne, 2008) and a proximally lunate, transversely compressed proximoplantal portion of metatarsal II (i.e., character 335), present in Anabisetia saldiviai, Diluvicursor pickeringi, and Gasparinisaura cincosaltensis, are features also present in the four aforementioned dryosaurids (see also Herne et al., Reference Herne, Tait, Weisbecker, Hall, Nair, Cleeland and Salisbury2018, regarding the form of metatarsal II). The inset femoral muscle scar on the femur for the m. caudofemoralis longus is also present in Callovosaurus leedsi Lydekker, Reference Lydekker1889 (Ruiz-Omeñaca et al., Reference Ruiz-Omeñaca, Pereda Suberbiola, Galton and Carpenter2006) and Elrhazosaurus nigeriensis Galton and Taquet, Reference Galton and Taquet1982 (Galton, Reference Galton2009)—taxa also considered to be dryosaurids. However, the three postcranial features mentioned above and included as characters in the dataset (i.e., characters 256, 331, and 335) failed to influence the recovery of taxa within Elasmaria closer to the dryosaurids, or even within Dyomorpha. Taken explicitly, the strict consensus results suggest that these postcranial features arose independently in the elasmarians and the dryosaurids.
The oldest record for the ornithopods is at least indicated by the dryosaurid Callovosaurus leedsi (see Ruiz-Omeñaca et al., Reference Ruiz-Omeñaca, Pereda Suberbiola, Galton and Carpenter2006) from the middle Callovian of the Oxford Clay Formation, England (~ 165 Ma; see Fig. 28). Based on this age, both Elasmaria and Muttaburrasaurus spp. would have diverged from other ornithopod lineages prior to the middle Callovian (>165 Ma). This timing of divergence indicates that the origins of Elasmaria and the stem of Muttaburrasaurus spp. trace to Pangaea, prior to the opening of seaways between Laurasia and Gondwana in the Callovian (e.g., Iturralde-Vinent, Reference Iturralde-Vinent2006; Pindell and Kennan, Reference Pindell and Kennan2009). The lengthy ghost lineages of Elasmaria (Fig. 28) and Muttaburrasaurus spp. likely reflect the poor body-fossil record of Gondwanan dinosaurs between the middle and earliest Cretaceous (e.g., Weishampel et al., Reference Weishampel, Barrett, Coria, Le Loeuff, Xu, Zhao, Sahni, Gomani, Noto, Weishampel, Dodson and Osmólska2004; Mateus, Reference Mateus2006, Dunhill et al., Reference Dunhill, Bestwick, Narey and Sciberras2016).
Distribution of Australian ornithopods
Based on newly described craniodental materials from the Flat Rocks locality, including Galleonosaurus dorisae n. gen. n. sp., assessment of Australian ornithopod diversity can be updated. The tooth morphology of the Victorian ornithopod dentary morphotype VOD3 resembles the isolated ornithopod tooth (QM F52774) described by Hocknull and Cook (Reference Hocknull and Cook2008, although reported as a maxillary tooth) from the Cenomanian–Turonian Winton Formation, Queensland (age based on Tucker et al., Reference Tucker, Roberts, Yu, Kemp and Salisbury2013; Figs. 24, 27). The similarity between these teeth suggests that the temporal and geographic range of closely related small-bodied ornithopods in eastern Australia extended from at least the upper Barremian of the Australian-Antarctic rift system to the middle Cretaceous of the Eromanga Basin, central-eastern Australia (Figs 1.3, 27). This distribution is augmented by the identification of small-bodied ornithopod body fossils from the Albian Griman Creek Formation, strata of which crop out at Lightning Ridge, northern New South Wales (Molnar and Galton, Reference Molnar and Galton1986; Bell et al., Reference Bell, Herne and Smith2017), and small-bodied (and possibly large-bodied) ornithopod trackways at Lark Quarry in the Winton Formation (Thulborn and Wade, Reference Thulborn and Wade1984; Romilio et al., Reference Romilio, Tucker and Salisbury2013; Fig. 27). Diverse small- to large-bodied ornithopod trackways have also been described from the Valanginian–Barremian Broome Sandstone, which crops out along the coast of the Dampier Peninsula in Western Australia (Salisbury et al., Reference Salisbury, Romilio, Herne, Tucker and Nair2017; Fig. 27). The only body fossils of a large-bodied ornithopod presently described from Australia are those of Muttaburrasaurus spp., all of which are from the late Albian Allaru Mudstone and overlying Mackunda Formation in central western Queensland (Bartholomai and Molnar, Reference Bartholomai and Molnar1981; Molnar, Reference Molnar1996; Fig. 27). Maxillary teeth further suggest the presence of a Muttaburrasaurus-like ornithopod in the Griman Creek Formation at Lightning Ridge. These teeth appear to be of a size between those of the Victorian taxa (e.g., Galleonosaurus dorisae n. gen. n. sp.) and Muttaburrasaurus sp. (QM F14921), suggesting that a midsized ornithopod could have also been present in this region. Large-bodied ornithopod remains are presently unknown from the Early Cretaceous of Victoria, with the largest taxon presently represented by the femur assigned to ‘Victorian Hypsilophodontid Femur Type 2’ (Rich and Rich, Reference Rich and Rich1989; Fig. 27). The occurrences of body fossils and tracks from the aforementioned Australian regions combined confirm that ornithopods formed a diverse fauna of dinosaurian herbivores across this continent during at least the Valanginian–Turonian (Fig. 27).
Conclusions
The identification of the new ornithopod, Galleonosaurus dorisae n. gen. n. sp., and three further jaw morphotypes (VOM4, VOD2, and VOD3) from the Flat Rocks locality in the upper Barremian Wonthaggi Formation complements the four previously named ornithopods from Victoria—Atlascopcosaurus loadsi, Diluvicursor pickeringi, Leaellynasaura amicagraphica, and Qantassaurus intrepidus. Although synonymy between some of these taxa is possible, Galleonosaurus dorisae n. gen. n. sp. and the newly identified craniodental morphotypes confirm that a highly diverse small-bodied ornithopod fauna flourished in the periodically disturbed, high-latitude, riverine floodplain environment of the Australian-Antarctic rift valley (see also Rich and Rich, Reference Rich and Rich1989; Rich and Vickers-Rich, Reference Rich and Vickers-Rich1999, Reference Rich and Vickers-Rich2000; Rich et al., Reference Rich, Vickers-Rich and Gangloff2002; Herne et al., Reference Herne, Tait and Salisbury2016, Reference Herne, Tait, Weisbecker, Hall, Nair, Cleeland and Salisbury2018).
The new dentary morphotype from the Flat Rocks Sandstone (VOD3) confirms the presence of an ornithopod with a more elongate dentary than that of Qantassaurus intrepidus, from the same locality, and with more alveoli in specimens of similar size (15 alveoli compared to 10). We speculate that VOD3 is a more likely candidate for the presently unknown dentary of Galleonosaurus dorisae n. gen. n. sp. than the dentary of Q. intrepidus, although this suggestion cannot be presently confirmed. The similarity between the dentary teeth of VOD3 and an isolated dentary tooth (QM F52774) discovered in the Winton Formation, central-western Queensland (Hocknull and Cook, Reference Hocknull and Cook2008) suggests that the spatiotemporal range of potentially closely related ornithopods in eastern Australia extended from at least the upper Barremian of the Australian-Antarctic rift system to the lower Turonian of the Eromanga Basin (Figs. 1.3, 27).
The phylogenetic analysis (Figs. 25.2, 26) recovered the Victorian ornithopods Diluvicursor pickeringi, Leaellynasaura amicagraphica, and Galleonosaurus dorisae n. gen. n. sp. within Elasmaria (Calvo et al., Reference Calvo, Porfiri and Novas2007). In addition to the Victorian taxa, Elasmaria also comprises the Argentinian taxa Anabisetia saldiviai, Gasparinisaura cincosaltensis, Macrogryphosaurus gondwanicus, and Talenkauen santacrucensis. Increased anatomical understanding of the ornithopods recovered within Elasmaria, and particularly the Victorian ornithopods, will undoubtedly impel renewed phylogenetic assessment. The large-bodied Australian genus Muttaburrasaurus, however, is a nonelasmarian and was recovered within Iguanodontia. The time-calibrated phylogeny derived from the IW strict consensus tree (Fig. 28) suggests that Elasmaria and the stem of Muttaburrasaurus langdoni had their origins in Pangaea prior to the opening of seaways between Gondwana and Laurasia in the middle Callovian.
Acknowledgments
The authors acknowledge the Eastern Maar and Bunurong peoples, the Traditional Owners of the fossil sites, and pay respect to their Elders past and present. For access to specimens in their care we thank P. Barrett, R. Coria, D. Pickering, L. Salgado, K. Seymour, K. Spring, and T. Zeigler. We additionally thank D. Brinkman (YPM), P. Currie, M. Hall, D. Henry, A. Heimer, D. Herne, G. Kool (discoverer of the holotype of Galleonosaurus dorisae n. gen. n. sp.), L. Kool, M. Lamanna, K. Poole, T. Rich, J. Rosine, S. Salisbury, D. Schwarz, D. Seegets-Villiers, P. Vickers-Rich, B. Wagstaff, W. White, and Monash University and Museums Victoria staff and volunteers of the Dinosaur Dreaming Project. We gratefully thank the late D. Pickering for preparation of the holotype of Galleonosaurus dorisae n. gen. n. sp. The authors thank the reviewers P. Galton and P. Godefroit, Academic Editor H.-D. Sues and JPA editors J. Kastigar and P. Mikkelsen for their reviews and comments that greatly improved the quality this paper, and L. Marra for journal production (Cambridge University Press). Travel expenses for MCH were covered in part by a UQ Graduate School Research Travel Grant.
Accessibility of supplemental data
Data available from the Dryad Digital Repository: http://doi.org/10.5061/dryad.rm8bk77.
Supplemental data include: Table S1, list of cranial specimens observed in this study; Text S1, additional remarks on phylogenetic data and analyses in this study; Text S2, phylogenetic character list; and Supplemental Data 1, character-taxon dataset; Supplemental Data 2, CT dataset.