Human breast milk from healthy, well-nourished mothers is considered as the ideal nourishment to fulfil nutritional and physiological needs of full-term infants in the first 6 months( Reference Kramer and Kakuma 1 ). Among all provided nutrients, human milk lipids contribute approximately 50 % of total energy and are also essential for the developing organs of infants to achieve an accelerated growth.
TAG, the major form of lipids in human milk (approximately 98 %), contain a special composition of SFA, MUFA and PUFA. These fatty acids are selectively esterified to the glycerol skeleton at three stereo-specifically numbered (sn) positions in human milk, and the proportion of sn-2 to sn-1,3 can significantly affect the uptake, metabolism and tissue distribution of fatty acids( Reference Innis 2 ).
The most common SFA, palmitic acid (PA, C16 : 0), is mainly esterified at the sn-2 position. The abundance of 2-mono-palmitins in human milk has been correlated with significant roles in infant health, including fatty acid and Ca absorption, bone health, gut microbes and infant comfort( Reference Innis 2 , Reference Bar-Yoseph, Lifshitz and Cohen 3 ). In human milk, high amount of unsaturated fatty acids such as oleic acid (OA, C18 : 1n-9), linoleic acid (LA, C18 : 2n-6) and α-linolenic acid (ALA, C18 : 3n-3) are preferentially distributed at the outer sn-1,3 positions. Long-chain PUFA (LC-PUFA) consist only a very small part of human milk lipids but have been largely evidenced to provide immune and brain development benefits, especially arachidonic acid (AA, C20 : 4n-6), DHA (C22 : 6n-3) and adrenic acid (C22 : 4n-6)( Reference Willatts, Forsyth and Agostoni 4 , Reference Jumbe, Comstock and Harris 5 ). Recent studies have also suggested that nervonic acid (NA, C24 : 1n-9) functions crucially in cognition development( Reference Strandvik, Ntoumani and Lundqvist-Persson 6 ). The positional distribution patterns of NA and LC-PUFA related to brain development were less investigated, and most available data( Reference Lopez-Lopez, Lopez-Sabater and Campoy-Folgoso 7 – Reference Straarup, Lauritzen and Faerk 12 ) reported the sn-2 fatty acid composition as a percentage of total sn-2 fatty acid without consideration of the total percentage of each fatty acid as recommended( Reference Lopez-Lopez, Lopez-Sabater and Campoy-Folgoso 7 , Reference Jiang, Liu and Li 9 ).
Phospholipids (PL) contribute 0·2–2·0 % of total human milk lipids. The dominant PL can be classified into five types, including phosphatidylethanolamine (PE), phosphatidylcholine (PC), sphingomyelin (SM), phosphatidylinositol and phosphatidylserine of which the former three consist 62–80 % of PL in human milk( Reference Cilla, Diego Quintaes and Barbera 13 , Reference Verardo, Gomez-Caravaca and Arraez-Roman 14 ). PL function in both the structural formation and the stability maintenance of milk fat globule membrane( Reference Cilla, Diego Quintaes and Barbera 13 , Reference Verardo, Gomez-Caravaca and Arraez-Roman 14 ). In addition, PL are considered essential for optimum development of infant brain as an important source of LC-PUFA and choline.
Lipid profile in human milk changes with lactation stages and varies among different geographical regions due to diverse dietary patterns and genetic backgrounds( Reference Sosa-Castillo, Rodriguez-Cruz and Molto-Puigmarti 15 – Reference Bravi, Wiens and Decarli 17 ). Previous Chinese breast milk studies demonstrated different fatty acid and PL composition from Western reports, but the intra-molecular distribution of fatty acids has not been detailed yet. Geographically representative breast milk from healthy, well-nourished mothers should be considered as the golden reference in the development of localised baby formulas( Reference Qi, Sun and Xia 10 ). Shanghai, the most developed city with diverse population and great infant development, is ideal for Chinese human milk study.
The aim of this study was to determine the lipid profile of human breast milk collected longitudinally at different lactation stages (colostrum milk (CM), transitional milk (TM) and mature milk (MM)) from healthy mothers living in Shanghai, China. The fatty acid composition was analysed with the emphasis on the sn-2 fatty acid distribution. The PL composition and change trends over lactation stages were also determined and discussed.
Methods
Subjects and milk samples collection
Lactating mothers were recruited from the Labour and Delivery Unit in Xinhua Hospital affiliated to Shanghai Jiao Tong University School of Medicine, Shanghai, China. Subjects were breast-feeding mothers aged between 18 and 35 years old and of full-term, singleton delivery with breast-feeding planned for more than 2 months. Exclusion criteria included diabetes, hypertension, communicable diseases and congenital abnormalities. Smoking and drinking were also excluded.
Mothers were asked to provide CM (1–5 d post-partum), TM (11–15 d post-partum) and MM (41–45 d post-partum). About 2 h after the first morning feeding, both sides of the mammary gland were evacuated completely with an electric breast pump, and the milk was carefully mixed. A proportion of whole breast milk was taken (5–10 ml for CM and 10–15 ml for TM and MM) and immediately transported to the freezer (–80°C), while the remaining milk was fed to the infants. Shanghai Xinhua Hospital Ethics Committee approved all aspects of the study, and informed consent was obtained from all the participants.
Fatty acid and sn-2 fatty acid analysis
Lipids were extracted from milk samples (2 ml) using the method published by Folch et al. ( Reference Folch, Lees and Sloane Stanley 18 ) with some modifications. Dichloromethane–methanol–petroleum ether (30–60°C) (2:1:2, by vol.; 20 ml) was used as the reagent to obtain lipid extract. The extraction solution was collected and evaporated to dryness with N2 flushing. The extract was re-dissolved in 2 ml of hexane, of which 600 µl was used to determine the fatty acid profile, and the remaining was used to analyse the sn-2 fatty acid composition.
For the analysis of fatty acid profile, 2 ml of hexane was added into the 600-µl lipid solution, and an accurate amount of tritridecanoin (4 mg for each sample) (C13 : 0; Nu-Chek-Prep) was added as internal standard. The mixture was then trans-esterified with 2 ml sodium methoxide methanol solution (0·5 m; Sigma-Aldrich), and the generated fatty acid methyl ethers (FAME) were then extracted with hexane. Saturated NaCl solution (2 ml) was added to facilitate the layer separation. The clear upper phase was purified through a nylon organic-phase needle filter (0·22 µm; Anpel) and subjected to fatty acid profile analysis.
Hydrolysis of TAG to 2-monoacylglycerol was carried out according to the method described by Luddy et al. ( Reference Luddy, Barford and Herb 19 ). In brief, the 1400 µl lipid extract was evaporated under N2 to dryness and re-dissolved in 200 µl of anhydrous diethyl ether, and TRIS buffer (pH 8·0, 6 ml), bile salts (0·05 %, 1·5 ml), calcium chloride (2·2 %, 0·6 ml) and pancreatic lipase (porcine pancreatic lipase type II, 20 mg) were then added successively. The mixture was mixed well and incubated in a shaking air bath (Innova 40; New Brunswick) under 40°C for 5 min at 240 rpm. Then HCl solution (6 m, 2 ml) was added to stop the reaction. Diethyl ether (4 ml) was used to transfer the sample solution to a 15-ml centrifuge tube. The mixture was then vortexed for 30 s and subjected to centrifugation at 6000 rpm for 3 min. The obtained clear upper phase was collected and evaporated under N2 to dryness. The residue containing TAG, diacylglycerols, 2-monoacylglycerols and NEFA was re-dissolved in 0·5 ml of methanol–chloroform (1:2, v/v) and followed by purification with a silica solid-phase extraction cartridge (1 g, 6 ml; Anpel). Hexane (30 ml) was used to equilibrate the cartridge, and hexane (8 ml), hexane–diethyl ether (0·95:0·05, v/v; 12 ml) and hexane–diethyl ether (0·90:0·10, v/v; 4 ml) were used successively to elute the TAG, NEFA and diacylglycerols, respectively. The 2-monoacylglycerols were eluted finally with methanol (12 ml), and the elute was collected and evaporated to dryness under N2. The residue was re-dissolved in 2 ml of hexane and subjected to the same trans-esterification procedure as the fatty acid profile analysis.
The FAME sample solutions obtained earlier was analysed by GC with flame ionisation detector( Reference López-López, Castellote-Bargalló and López-Sabater 20 ) (Agilent 7890B). The separation was performed on a capillary column (Supelco 2560, 100 m×0·25 mm×0·20 µm; Sigma-Aldrich). Both the injector and the detector temperatures were 250°C. Helium was used as the carrier gas with a flow rate of 0·8 ml/min and split ratio was 1:20. The column oven temperature program was as follows: 100°C for the first 13 min, followed by an increase of 10°C/min to 180°C and maintained for 6 min, and subsequently elevated to 200°C at the rate of 1°C/min and maintained for 20 min and finally increased by 4°C/min until reaching 230°C and maintained at this temperature for 13·5 min. The FAME were identified by comparing retention times of sample peaks with those of a mixed FAME standard (GLC-746; Nu-Chek-Prep) and calculated using the internal standard added to the samples at the very beginning of pre-treatment.
Phospholipids analysis
PL were extracted according to modified Folch extraction( Reference Folch, Lees and Sloane Stanley 18 ). Briefly, 0·75 g of maternal milk was weighed into a test tube and extracted with chloroform–methanol (2:1, v/v; 4·5 ml), and 0·5 ml saturated NaCl solution was then added to facilitate the layer separation. The lower organic phase was separated and collected after centrifugation at 6000 rpm for 5 min. The extraction was repeated one more time, and the organic phases were combined and evaporated to dryness under N2. The crude extract was dissolved in chloroform–methanol (2:1, v/v; 1 ml) and further purified by a silica cartridge (Sep-Pak 500 mg, 6 ml; Waters). After equilibration with hexane (40 ml), non-polar lipids were eluted first by hexane–diethyl ether (1:4, v/v; 3 ml) and hexane–diethyl ether (1:1, v/v; 3 ml). The PL were then eluted with methanol (4 ml) and chloroform–methanol–water (3:5:2, by vol.; 2 ml) and evaporated to dryness under N2. The PL were then re-dissolved in chloroform–methanol (1:2, v/v; 200 µl) and filtered through a polytetrafluoroethylene membrane filter (0·20 µm; Anpel).
The PL sample solutions obtained earlier were analysed by HPLC with variable wavelength detector (Agilent 1260 Infinity; Agilent). The separation was performed on a normal-phase column (ZORBAX RX-SIL, 4·6 mm×250 mm×5 µm; Agilent). The mobile phase was a mixture of methanol, phosphoric acid (85 %; Sigma-Aldrich) and acetonitrile (32:0·6:67·4, by vol.) at a flow rate of 1 ml/min. The column temperature was at 30°C, and the wavelength of the detector was set at 205 nm. PE, PC and SM were analysed and quantified using standard curves (standards all from Sigma-Aldrich).
Statistical analysis
The results were reported as means and standard deviations and analysed with SPSS 20.0 (SPSS). Data were first checked for normality with the Kolmogorov–Smirnov test. When the variables were normally distributed, one-way ANOVA was adopted to evaluate differences at the significance level of 0·05, and then a comparison between any two means of the lactation stage was performed by a Bonferroni test if the results of ANOVA were significant. Otherwise, a non-parametric Kruskal–Wallis test was used.
Results
Basic characteristics
A total of 100 mothers provided at least one sample while sixty-eight provided three samples. A total of 248 milk samples including seventy CM, ninety-six TM and eighty-two MM samples were collected. The main characteristics of the sampled mothers and respective newborns are shown in Table 1. All mothers were Han Chinese. For newborns, fifty-five were delivered by caesarean section, and the others were born vaginally.
Table 1 Characteristics of the sampled 100 mothers and respective newborns (Mean values, standard deviations and ranges)

Fatty acid and sn-2 fatty acid composition over lactation
Fatty acid composition (FA%, w/w) described individual fatty acid in total fatty acids, and sn-2 fatty acid composition (sn-2 %, w/w) described a specific fatty acid at sn-2 position compared with the total amount of this fatty acid (relative percentage of each fatty acid in sn-2 position). An overall fatty acid composition is shown in Table 2, and the detailed fatty acid profiles (FA% and sn-2 %) are presented in Table 3 (SFA, twelve types), Table 4 (MUFA, five types) and Table 5 (PUFA, eleven types).
Table 2 Fatty acid composition of human milk (%, w/w) (Mean values and standard deviations)

LA, linoleic acid; ALA, α-linolenic acid; AA, arachidonic acid.
a,b Mean values within a row with unlike superscript letters were significantly different (P<0·05).
Table 3 Detailed fatty acid profile of SFA (Mean values and standard deviations)
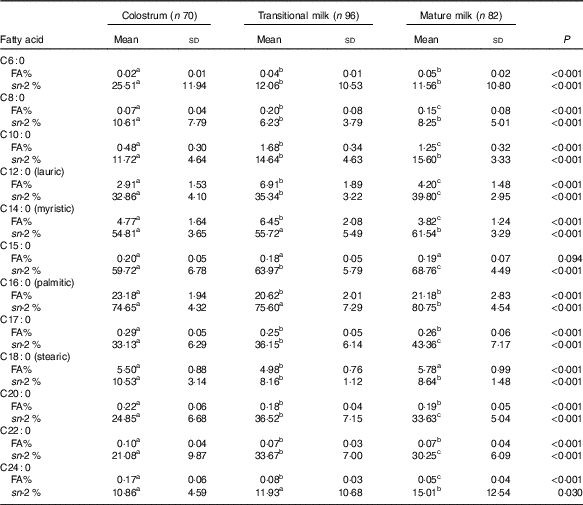
FA%, percentage of total fatty acids; sn-2 %, relative percentage of a specific fatty acid at sn-2 position.
a,b,c Mean values within a row with unlike superscript letters were significantly different (P<0·05).
Table 4 Detailed fatty acid profile of MUFA (Mean values and standard deviations)

FA%, percentage of total fatty acids; sn-2 %, relative percentage of a specific fatty acid at sn-2 position.
a,b,c Mean values within a row with unlike superscript letters were significantly different (P<0·05).
Table 5 Detailed fatty acid profile of PUFA (Mean values and standard deviations)

FA%, percentage of total fatty acids; sn-2 %, relative percentage of a specific fatty acid at sn-2 position.
a,b,c Mean values within a row with unlike superscript letters were significantly different (P<0·05).
The amount of total fatty acids showed a significant increment for three stages (P<0·001) as CM (15·20 (sd 7·21) mg/g human milk), TM (29·55 (sd 8·98) mg/g human milk) and MM (32·55 (sd 12·79) mg/g human milk), while the change in percentage of total SFA, total MUFA, total PUFA and individual fatty acid appeared differently (Table 2). SFA were the most abundant fatty acids in human milk that the percentage of SFA was slightly higher than MUFA and about 10 % higher than PUFA (SFA:MUFA:PUFA=approximately 1·4:1·3:1 in CM and MM; approximately 1·6:1·2:1 in TM). A higher percentage of SFA was reported in TM together with a lower percentage of MUFA. Percentages of total PUFA, n-6 PUFA and n-3 PUFA, were all constant over lactation stages, and stable levels were also seen in the ratios of n-6:n-3 (approximately 11), LA:ALA (approximately 19) and AA:DHA (approximately 1·3).
Fatty acid and sn-2 fatty acid composition of SFA
PA was the dominant SFA in content (approximately 20 % of total fatty acids), and lauric acid (C12 : 0), myristic acid (C14 : 0) and stearic acid (C18 : 0) were 2–6 % of total fatty acids, while C6 : 0, C8 : 0 and C24 : 0 only consisted a very small part (<1·0 %) of total milk fat. TAG in TM and MM contained higher level of short-chain (C6 : 0) and medium-chain SFA (C8–C14) but lower level of long-chain SFA (C15–C24). Interestingly, the relative proportion of SFA at the sn-2 position increased considerably from C10 : 0 to C16 : 0. Around 55 % of myristic acid, 65 % of C15 : 0 and 75 % of PA were distributed at the sn-2 position, while the other types of SFA were selectively located at the sn-1,3 positions.
Fatty acid and sn-2 fatty acid composition of MUFA
As shown in Table 4, OA was the absolute dominant MUFA (approximately 30 % of total fatty acids) with the content declined significantly from CM (32·00 (sd 2·64) %) to TM (29·80 (sd 3·66) %) and restored again in MM (32·70 (sd 3·76) %). For other MUFA, C16 : 1n-7 was approximately 2 % and showed a rising tendency among lactation, while C20 : 1n-9, C22 : 1n-9 and NA were lower than 1 % and enriched in CM. NA, the only MUFA mainly located at the sn-2 position (54·91–62·87 %), declined by 50 % from CM (0·32 %) to TM (0·11 %) and from TM to MM (0·06 %).
Fatty acid and sn-2 fatty acid composition of PUFA
As shown in Table 5, the content of LA (approximately 20 % of total fatty acids) predominated among n-6 series, and ALA (approximately 1·2 % of total fatty acids) predominated among n-3 series. C20 : 2n-6 was 1·38 % in CM but fell to approximately 0·6 % in TM and MM, and the other PUFA were lower than 1 % during the three stages. PUFA with a carbon chain length of 18 were more abundant in TM and MM and were preferentially located at the sn-1,3 positions (approximately 20 % at the sn-2 position). Over lactation stages, LA and ALA showed a significant but small increment, while C18 : 3n-6 increased by approximately 50 % over the level in CM.
LC-PUFA were enriched in CM, and a relatively low proportion was also found for C20 PUFA at the sn-2 position (approximately 20 %) with a higher proportion for AA (30·84–38·92 %). C22 PUFA including adrenic acid (C22 : 4n-6), C22 : 5n-6, DHA and docosapentaenoic acid (DPA, C22 : 6n-3), were mainly esterified at the sn-2 positions in TAG (approximately 60 %).
Phospholipid composition over lactation
PL composition of human milk is shown as absolute amount in Table 6 (mg/100 g human milk) and proportion of total PL in Table 7 (%, w/w). As shown in Table 6, the total amount of PE, PC and SM in human milk were significantly lower in MM (28·10 (sd 7·86) mg/100 g) than in CM (35·07 (sd 10·84) mg/100 g) and TM (35·09 (sd 8·63) mg/100 g). PC appeared at the highest level followed by SM and PE, with the ratio of 4:2:1. As shown in Table 7, the percentage of PE remained constant, and different change direction was report as decreasing in PC and increasing in SM.
Table 6 Phospholipid (PL) composition of human milk (mg/100 g human milk) (Mean values and standard deviations)

PE, phosphatidylethanolamine; PC, phosphatidylcholine; SM, sphingomyelin.
a,b Mean values within a row with unlike superscript letters were significantly different (P<0·05).
Table 7 Phospholipid (PL) composition of human milk (%, w/w) (Mean values and standard deviations)

PE, phosphatidylethanolamine; PC, phosphatidylcholine; SM, sphingomyelin.
a,b Mean values within a row with unlike superscript letters were significantly different (P<0·05).
Discussion
Fatty acid profile of total and sn-2 position
The fatty acid composition in Chinese human milk has been well-documented, showing regional disparities interiorly and great differences from Western profile. Studies from representative areas such as Shanghai are needed to conclude the optimal composition of Chinese human milk TAG. Also, the stereo-chemical distribution was less investigated, and most available data only detailed the sn-2 fatty acid composition as the percentage of total sn-2 fatty acids, without considering the total percentage of each fatty acid, which varied in studies. sn-2 profiles were recommended to be expressed as the relative fatty acid in sn-2 position to elaborate the positional selectivity of fatty acids( Reference Lopez-Lopez, Lopez-Sabater and Campoy-Folgoso 7 ). Researches also indicated that relative percentages might be better to compare the effect of milk fat on infant development( Reference Jiang, Liu and Li 9 ). In our present study, fatty acid profile of total and sn-2 position was detailed together for a comprehensive understanding of Chinese milk TAG.
SFA
SFA (37·18–41·63 %) dominated in fatty acid profile over the three lactation stages. The SFA content in mature stage was reported as slightly higher than other studies conducted in China( Reference Qi, Sun and Xia 10 , Reference Urwin, Zhang and Gao 21 – Reference Jiang, Wu and Yu 23 ) and slightly lower than conducted in Japan( Reference Yuhas, Pramuk and Lien 24 ), South Korean( Reference Kim, Kang and Jung 25 ) and some European countries( Reference Yuhas, Pramuk and Lien 24 ) (online Supplementary Table S1). Dietary pattern was commonly considered the major contributor. In general, Western dietary pattern with lower carbohydrate and higher fat than traditional Chinese diet may lead to higher SFA in human milk.
From this study, it has been well-recognised that PA was the dominant SFA in human milk (20·62–23·18 %), and lauric acid (2·91–6·91 %), myristic acid (3·82–6·45 %) and stearic acid (4·98–5·78 %) were also main SFA but with much lower levels that were comparable to previous Chinese data( Reference Qi, Sun and Xia 10 , Reference Urwin, Zhang and Gao 21 – Reference Jiang, Wu and Yu 23 ). In our study, PA existed primarily at the sn-2 position with a rising tendency over lactation stages (74·65 % in CM, 75·60 % in TM, 80·75 % in MM). A typical sn-2 positional preference for PA was also observed in previous studies (online Supplementary Table S2). The Wuxi study reported a similar percentage of 81·29 % from ten MM( Reference Sun, Wei and Su 11 ). And the Chinese multi-centre study from Beijing, Luoyang and Changsha reported a decreasing trend as 77·59 % in CM, 75·56 % in TM and 73·7 % in MM in 1 month and 69·39 % in MM 2 months( Reference Jiang, Liu and Li 9 ). Similar rising trend with different proportions were reported in foreign studies. A higher level was observed in Spain( Reference Lopez-Lopez, Lopez-Sabater and Campoy-Folgoso 7 ) (80·30 % in CM, 86·25 % in TM, 87·86 % in MM; n 12) and a lower level was reported in Italy( Reference Haddad, Mozzon and Frega 8 ) (62·00 % in CM, 59·11 % in TM, 62·86 % in MM; n 8). sn-2 PA has been proven to be positively related to improved lipids and Ca absorption, more faecal SCFA production, better intestinal microbiome development and less gut inflammation( Reference Bar-Yoseph, Lifshitz and Cohen 3 , Reference Jiang, Liu and Li 9 , Reference Miles and Calder 26 ).
Similar to other studies, the relative proportion of SFA at the sn-2 position increased considerably from C10 : 0 to C16 : 0, and the sn-2 positional preference was found for C14 : 0, C15 : 0 and C16 : 0, while other SFA were primarily distributed at the sn-1,3 positions( Reference Lopez-Lopez, Lopez-Sabater and Campoy-Folgoso 7 , Reference Haddad, Mozzon and Frega 8 , Reference Sun, Wei and Su 11 ). The carbon chain length may be a discriminating factor in distribution of SFA.
MUFA
The MUFA in our study was comparable to Asian data( Reference Qi, Sun and Xia 10 , Reference Urwin, Zhang and Gao 21 – Reference Kim, Kang and Jung 25 ) but was much lower than Mediterranean studies( Reference Lopez-Lopez, Lopez-Sabater and Campoy-Folgoso 7 , Reference Grote, Verduci and Scaglioni 27 ), mainly due to the lower level of OA (online Supplementary Table S1). The abundance of OA in Spanish and Italian human milk may be attributed to the high intake of olive oil in classic Mediterranean diet. OA, the main MUFA, plays an important role in the liquidity of human milk required for the formation, transport and metabolism of milk fat globules by reducing the lipid melting point( Reference Jensen 28 ).
The MUFA were mainly distributed at the sn-1,3 positions with the only exception of NA (54·91–62·87 % at the sn-2 position). NA is a low-content MUFA with limited report in previous human milk studies. Increasing numbers of studies have suggested that NA supercharges muscle and brain by forming myelin. The sn-2 positional selectivity of NA was also found in several human milk studies( Reference Qi, Sun and Xia 10 , Reference Sun, Wei and Su 11 ), but only one study reported the accurate percentage as 63·77 % from 10 Wuxi MM( Reference Sun, Wei and Su 11 ). The level of NA found in our study significantly decreased by approximately 50 % from CM (0·32 %) to TM (0·11 %) and from TM to MM (0·06 %). Previous longitudinal Chinese studies indicated a same decrease from CM (0·22–0·4 %) to TM (0·08–0·13 %), but the NA level was then maintained in MM (0·1–0·20 %)( Reference Qi, Sun and Xia 10 , Reference Urwin, Zhang and Gao 21 – Reference Jiang, Wu and Yu 23 ). However, MM studies in several Chinese cities including Shanghai also found a low level as 0·05 %( Reference Li, Fan and Zhang 29 ).
PUFA
The percentage of PUFA in our study, in agreement with previous Chinese studies( Reference Qi, Sun and Xia 10 , Reference Urwin, Zhang and Gao 21 – Reference Jiang, Wu and Yu 23 ), indicated a much higher level than foreign studies mainly due to the high level of LA (online Supplementary Table S1). Asian (0·92–1·33 %, Chinese data included)( Reference Qi, Sun and Xia 10 , Reference Urwin, Zhang and Gao 21 – Reference Kim, Kang and Jung 25 ) and American studies (1·05–1·22 %)( Reference Yuhas, Pramuk and Lien 24 ) also found a similar level of ALA in mature stage as our study (1·45 %), which is nearly twice the level of ALA in European studies (0·62–0·75 %)( Reference Lopez-Lopez, Lopez-Sabater and Campoy-Folgoso 7 , Reference Grote, Verduci and Scaglioni 27 ). The level of DHA in our study was on top of the previous Chinese data (0·22–0·55 %) but much lower than Japan (0·99 %)( Reference Yuhas, Pramuk and Lien 24 ) and South Korean (0·67 %)( Reference Kim, Kang and Jung 25 ) and higher than American reports (0·17 %)( Reference Yuhas, Pramuk and Lien 24 ). AA was of similar level among different continents. The differences mainly resulted from varied diet intake, and it was well-evidenced that increasing intake of animal and/or marine foods resulted in increment of ALA, and LA was influenced by intake of vegetable oils, while aquatic food intake was correlated with DHA levels in human milk, and AA was found as no clear correlation with dietary interferences( Reference Innis 16 , Reference Chung 30 ).
With the typical abundance of LA in our study, the n-6:n-3 PUFA was found as high as 11 and remained constant over lactation, which was extremely similar to the latest longitudinal human milk study of the neighbouring Chinese city Wuxi( Reference Qi, Sun and Xia 10 ). The increasing ratios of n-6:n-3 were also observed in Chinese as well as Western human milk but not in Japan and South Korean. The dominance of n-6 over n-3 fatty acids in early life has been ascribed as a contributing factor to childhood obesity and allergies, as n-6 fatty acids can promote the differentiation of pre-adipocytes and give rise to pro-inflammatory compounds( Reference Allhaud, Guesnet and Cunnane 31 , Reference Nwaru, Erkkola and Lumia 32 ). Additional reports also showed that the intake of a high ratio of n-6:n-3 fatty acids was correlated with declined neuro-development in offspring( Reference Jumbe, Comstock and Harris 5 , Reference Andrade-da-Costa, Isaac and Silva 33 ).
In agreement with previous studies, TAG in CM contained lower level of LA and ALA but higher level of LC-PUFA than other two milk stages. Both LA and ALA were mainly distributed at the sn-1,3 positions. With the abundance of LA and its sn-1,3 positional selectivity, Chinese human milk contains more OA–PA–LA than OA–PA–OA( Reference Tu, Ma and Bai 34 ), while OA–PA–OA is the most abundant TAG in Western human milk( Reference Haddad, Mozzon and Strabbioli 35 ). Commercial formulas based on foreign studies may be not suitable for Chinese babies.
The three most abundant PUFA in human brain are DHA, AA and adrenic acid, serving as essential bio-active substances for motor and cognitive development along with immune functions in infants. DPA has not been extensively studied because of the limited availability of the pure compound, but the available data suggest that it has beneficial effects in inflammation and immune response modulation and spatial learning( Reference Dyall 36 ). The study from Spain( Reference Lopez-Lopez, Lopez-Sabater and Campoy-Folgoso 7 ) showed a relatively lower proportion of DHA at the sn-2 position (52·63–61·39 %) and a higher AA (43·27–47·85 %) than our data (60·25–61·89 % for DHA, 30·84–38·92 % for AA). Current reported result from Wuxi, China( Reference Sun, Wei and Su 11 ), showed that the relative sn-2 proportion of DHA (63·23 %) and AA (39·65 %) in MM was similar to ours. Possible mechanisms for the distribution include better absorption( Reference Christensen, Hoy and Becker 37 , Reference Cruz-Hernandez, Thakkar and Moulin 38 ), improved oxidative stability( Reference Wijesundera 39 ) and their enrichment in brain tissues( Reference Christensen and Hoy 40 , Reference Christensen, Lund and Simonsen 41 ).
The levels of adrenic acid and DPA were less investigated, and our data in each lactation stage were similar to a multi-centre Chinese human milk study (n 477) in 2016( Reference Giuffrida, Cruz-Hernandez and Bertschy 22 ). However, available data showed conflicting positional distribution patterns for the two fatty acids. Adrenic acid (32·42 % at the sn-2 position) and DPA (28·24 % at the sn-2 position) were observed with preferable distribution at sn-1,3 positions in the Wuxi study( Reference Sun, Wei and Su 11 ), while our study, in agreement with the Spanish study( Reference Lopez-Lopez, Lopez-Sabater and Campoy-Folgoso 7 ), showed an sn-2 positional selectivity (higher than 60 %) for both the fatty acids (online Supplementary Table S2). Another Chinese study with 309 human milk samples determined also indicated that DPA and adrenic acid were both preferential esterified at the sn-2 positions (no accurate relative percentage reported)( Reference Qi, Sun and Xia 10 ). Thus, additional studies should be performed on the LC-PUFA distribution.
Phospholipids
Human milk PL studies were relatively limited and varied in results all over the world. Total PL content in human milk ranged between 9·8 and 47·4 mg/100 g, with SM (28·9–43·3 %), PC (19·0–38·4 %) and PE (5·9–36·1 %) found in most studies as the three most abundant PL( Reference Cilla, Diego Quintaes and Barbera 13 , Reference Verardo, Gomez-Caravaca and Arraez-Roman 14 , Reference Giuffrida, Cruz-Hernandez and Bertschy 22 ). Therefore, only the three main PL were measured in this study.
The total amount of PL in our study decreased significantly from 35·07 mg/100 g in CM to 28·10 mg/100 g in MM, indicating reduced bio-synthesis of PL in the later stage of lactation. Recent Western studies found a much lower level of PL (13·5–16·8 mg/100 g in CM and 9·8–19·2 mg/100 g in MM)( Reference Cilla, Diego Quintaes and Barbera 13 , Reference Verardo, Gomez-Caravaca and Arraez-Roman 14 ), but the latest Chinese study from Beijing, Guangzhou and Suzhou( Reference Giuffrida, Cruz-Hernandez and Bertschy 22 ) reported a comparable level of PL as 33·0–38·9 mg/100 ml in CM and 22·3–26·0 mg/100 ml in MM. The decline of PL in human milk was found concomitant with the maturation of digestion system in infants and responsible for the decrease in the thickness of the membrane that surrounds the fat globule in human milk( Reference Bitman, Wood and Mehta 42 ).
The content and relative content order of the three PL differed in previous studies, and most Western studies reported the content order as SM>PC>PE( Reference Cilla, Diego Quintaes and Barbera 13 , Reference Verardo, Gomez-Caravaca and Arraez-Roman 14 ). The order of PC>SM>PE was shown in this study, similar to the Chinese multi-centre study mentioned earlier( Reference Giuffrida, Cruz-Hernandez and Bertschy 22 ). However, compared to the total population data from the Chinese study (PC 8·2–12·0 mg/100 ml, SM 7·2–9·0 mg/100 ml, PE 6·4–8·5 mg/100 ml), the amount of PC (15·41–20·32 mg/100 g) was much higher, and SM (9·07–10·14 mg/100 g) showed a similar content while the amount of PE (3·63–4·61 mg/100 g) was much lower in our study.
Each of the three PL showed a decline in the absolute content with the prolonged lactation, which was consistent with previous studies. When reported as the relative proportion of total PL, PE remained stable while PC and SM showed decreasing and increasing trend, respectively, from CM to MM stage in our study. The Chinese multi-centre study( Reference Giuffrida, Cruz-Hernandez and Bertschy 22 ) did not detail the percentage data, and in Western studies, PC showed an increment over lactation, while varied trend was observed in different studies for PE (stable/increasing) and SM (stable/increasing/decreasing). More research should be performed on the PL composition along with possible mechanisms and consequent influences.
Conclusions
The fatty acid profile of total and sn-2 position and the composition of main PL were determined in 248 human milk samples collected longitudinally from Shanghai, a representative city for human milk studies. We observed significant changes in the composition of fatty acids, sn-2 fatty acids and PL over lactation, while the positional distribution pattern (sn-2 or sn-1,3) remained relatively consistent. Compared to Western studies, LA was more common in our study, which contributed to the high ratio of n-6:n-3 PUFA and the relative abundance of OA–PA–LA with its sn-1,3 selectivity. While the majority of unsaturated fatty acids were esterified at the sn-1,3 positions, long-chain unsaturated fatty acids closely related to brain and immune development were enriched in CM and mainly distributed at the sn-2 position. For the three dominant PL, the content order of PC>SM>PE was shown, with the relative percentage of PC, SM and PE decreasing, increasing and remaining constant across the three lactation stages, respectively. Results in this study provided comprehensive information for future infant formula design with optimal lipid profile close to Chinese human milk. Further studies are still needed to focus on the theory behind these specific profiles and the corresponding functions.
Acknowledgements
This study was supported by Abbott Nutrition Research & Development Center, Shanghai, China.
Y. G., M. C., Y. M. and F. T. conceived and designed the study protocols. B. W., L. Z. and L. S. conducted the sample collection. R. G. and K. W. conducted the determinations and analysed the data. K. W. wrote the manuscript. M. C. and Y. G. were primarily responsible for the final contents. All the authors read and approved the final manuscript.
None of the authors has any conflicts of interest to declare.
Supplementary material
For supplementary material/s referred to in this article, please visit https://doi.org/10.1017/S0007114518002994