Introduction
Unmanned aircraft systems (UASs), comprising an unmanned aerial vehicle (UAV) communicating with a ground-based controller, are gaining popularity in ecological research due to technological advances increasing their capabilities and affordability (Berger-Tal and Lahoz-Monfort Reference Berger-Tal and Lahoz-Monfort2018, Santangeli et al. Reference Santangeli, Chen, Kluen, Chirumamilla, Tiainen and Loehr2020, Lahoz-Monfort and Magrath Reference Lahoz-Monfort and Magrath2021). There is growing evidence that this technology, together with machine learning systems, have significant potential in collecting reliable information to better inform and improve the conservation and management of endangered species (Corcoran et al. Reference Corcoran, Denman and Hamilton2021). UASs have become much more accessible to both amateur and professional naturalists, creating new opportunities for research, but also present health and safety, and animal welfare challenges (Weston et al. Reference Weston, O’Brien, Kostoglou and Symonds2020).
Most UAVs belong to two main types: fixed-wing (unmanned planes, propelled by an engine) and multirotor (unmanned multicopters, with several pairs of engine-powered rotor blades) (Anderson and Gaston Reference Anderson and Gaston2013; Lahoz-Monfort and Magrath Reference Lahoz-Monfort and Magrath2021). UAS users could be categorised as follows: (1) “non-expert” leisure users; (2) professional photographers and film crews; (3) experienced biologists, using this tool for research purposes. Even though the people from the first category would generally use smaller, less expensive drones, probably generating less disturbance per se, the pilots may have no experience in animal behaviour, and unable to detect disturbance behaviour. The users from the second category may utilise much larger and noisier UAVs to support high quality professional TV standards, potentially generating much more disturbance and spending more time on site. The current review is particularly aimed at using UASs for scientific research (third category), aiming at professionals, and providing guidelines in an attempt to limit the potential disturbance (based on the best knowledge at present) and collect additional information and evidence on the impact.
We acknowledge the potential of UASs to increase research efficiency and reduce research effort, time, and financial and operational costs. For example, drones offer clear benefits such as access to remote, dangerous, or difficult locations, efficient large-area coverage, better or new vantage points, and are safer than manned flights (Anderson and Gaston Reference Anderson and Gaston2013). However, the discussion regarding the use of UASs in ornithology naturally focuses on disturbance and health hazard issues, but also on the possibility that exposure to UAVs will harm the reproductive success of the birds being studied. Recent behavioural studies have shown that apparent tolerance of UAVs by wild animals does not necessarily prove that the animals are not stressed (Ditmer et al. Reference Ditmer, Vincent, Werden, Tanner, Laske, Iaizzo, Garshelis and Fieberg2015, Barnas et al. Reference Barnas, Newman, Felege, Corcoran, Hervey, Stechmann, Rockwell and Ellis-Felege2018, Weimerskirch et al. Reference Weimerskirch, Prudor and Schull2018). Stress can cause deterioration of body condition and significantly impact overall breeding and population parameters (Thiel Reference Thiel2007, Price Reference Price, Lunney, Munn and Meikle2008, Glądalski et al. Reference Glądalski, Bańbura, Kaliński, Markowski, Skwarska, Wawrzyniak, Zieliński, Cyżewska, Mańkowska and Bańbura2016).
The evidence suggests that species which are both aerial and terrestrial are more likely to show behavioural responses to UAVs (Rebolo-Ifrán et al. Reference Rebolo-Ifrán, Grilli and Lambertucci2019), and that alterations to a bird’s behaviour can not only directly affect an individual, but also affect its breeding success through disturbance, increased energy expenditure, and reduced feeding times (Schnidrig-Petrig Reference Schnidrig-Petrig1998, Enggist-Düblin and Ingold Reference Enggist-Düblin and Ingold2003), and prompt abandonment of feeding sites or nests, ultimately leading to increased exposure to predation of eggs or chicks (Margalida et al. Reference Margalida, García, Bertran, Heredia, Garcia, Bertran, Heredia, García, Bertran and Heredia2003, Reference Margalida2012, González et al. Reference González, Arroyo, Margalida, Sánchez and Oria2006, Gill Reference Gill2007, Glądalski et al. Reference Glądalski, Bańbura, Kaliński, Markowski, Skwarska, Wawrzyniak, Zieliński, Cyżewska, Mańkowska and Bańbura2016).
Reducing disturbance through the use of modern technology to replace human work outdoors is among the main arguments in favour of UAS use and can often also be justified by lower costs and the avoidance of health risks to personnel (Borrelle and Fletcher Reference Borrelle and Fletcher2017).
Despite these controversies, the potential negative impacts of UAV deployment are still poorly understood and there have been many calls for further research into the issues raised and for the development of standardised methodologies (Weimerskirch et al. Reference Weimerskirch, Prudor and Schull2018, Rebolo-Ifrán et al. Reference Rebolo-Ifrán, Grilli and Lambertucci2019, Weston et al. Reference Weston, O’Brien, Kostoglou and Symonds2020, Krause et al. Reference Krause, Hinke, Goebel and Perryman2021, Tobajas et al. Reference Tobajas, Guil and Margalida2021).
So far, we know that species respond to UAVs in different ways and that the impact on their behaviour and physiology depends on a number of factors, including species ecology, phenology, time of day, or distance from the nest (Mulero-Pázmány et al. Reference Mulero-Pázmány, Jenni-Eiermann, Strebel, Sattler, Negro and Tablado2017, Weston et al. Reference Weston, O’Brien, Kostoglou and Symonds2020, Krause et al. Reference Krause, Hinke, Goebel and Perryman2021, Tobajas et al. Reference Tobajas, Guil and Margalida2021). Further research on this issue should therefore focus on the specific negative impacts of particular types of UAS use on particular species, and in specific locations and situations (Marion et al. Reference Marion, Davies, Demšar, Irvine, Stephens and Long2020).
Vultures are one of the most threatened guilds on the planet (Safford et al. Reference Safford, Andevski, Botha, Bowden, Crockford, Garbett, Margalida, Ramírez, Shobrak, Tavares and Williams2019, IUCN 2020). They perform unique functional roles in ecosystems as the main consumers of carrion and provide ecosystem services such as disease and pest control, provision of economic benefits, intellectual, spiritual, and aesthetic inspiration, and recreational services and ecotourism (Moleón et al. Reference Moleón, Sánchez-Zapata, Margalida, Carrete, Owen-Smith and Donázar2014, DeVault et al. Reference DeVault, Beasley, Olson, Moleón, Carrete, Margalida and Sánchez-Zapata2016). Vultures have therefore become the subject of increasing research interest (Alarcón and Lambertucci Reference Alarcón and Lambertucci2018) with calls for standardisation in their study, monitoring approaches, and methods, particularly when assessing demographic parameters such as breeding success (Perrig et al. Reference Perrig, Lambertucci, Donadio, Padró and Pauli2019). As vultures often nest in remote and inaccessible locations, expensive aerial surveys using piloted aircraft are sometimes required to accurately assess their breeding activity (Krüger and Amar Reference Krüger and Amar2017, Murn et al. Reference Murn, Botha and Wilson2017). UASs may offer a financially attractive alternative to piloted aerial surveys to provide the information necessary to improve our knowledge of vulture breeding ecology and to plan conservation action. However, the effects of UAVs on vultures have not been fully assessed, leaving a risk that such surveys may negatively affect their behaviour, physiology, or breeding success. This study considers the use of UASs in vulture research, focusing on the potential impact of UAVs, and in particular the most commonly used UAV – the multirotor drone. We review existing information on the reported responses of vultures and other comparable species to UAVs, summarising the relevant characteristics of four European vulture species, their inter- and intra-specific interactions, and their known susceptibility to UAV disturbance. Our goals were to assess the potential effects of UAVs and provide practical recommendations for their use in vulture conservation and research.
Materials and methods
The literature on the use of UASs to monitor and study vultures and their associated interactions and effects was reviewed up to 31 December 2021. A general search was performed using combinations of the following keywords: “drone”, “UAV”, “UAS”, “unmanned aircraft system”, “unmanned aerial vehicle”, “unmanned aircraft vehicle”, “vulture”, “disturbance”, “flight interactions”, and “response” using the Google Scholar search engine, specialised scientific search engines (e.g. Web of Science, Scopus), and ResearchGate. Initial searches showed that very little has been published on the interactions between vultures and UAVs, so the literature review was broadened to include studies on ecologically comparable species, such as large raptors, as well as relevant studies on the breeding success and behaviour of other species monitored using UASs, including waterfowl, penguins, and even marine and terrestrial mammals. Relevant citations in the published literature, grey literature (such as conference presentations), popular scientific papers, newspaper articles, and personal communications between conservation experts using UASs were also selected. Particular guidelines and suggested methodologies included in the literature were extracted for more detailed review and synthesis to produce recommended best practices for vulture monitoring and research.
The collated literature sources were screened and prioritised according to relevance, and then collated into a final unified bibliographical list of relevant literature using the Elsevier open-source bibliographical management software, Mendeley (https://www.mendeley.com). The final dataset was reviewed, synthesised, and critically appraised according to the quality of the evidence, the different methods used, geographical scope, target species, type of UAV, type of response, and observed interactions.
The findings were then reviewed in the light of the ecological characteristics, known interaction patterns, and behaviour of the four vulture species occurring in Europe, i.e. Bearded Vulture (Gypaetus barbatus), Egyptian Vulture (Neophron percnopterus), Cinereous Vulture (Aegypius monachus), and Griffon Vulture (Gyps fulvus), to provide practical recommendations and considerations for the use of UAVs in their monitoring and research.
Results and discussion
Vultures and UAVs – results from the literature review
The literature search yielded 86 relevant sources (in English, French, German, and Russian) describing interactions between wildlife and UAVs. As shown in Figure 1, the number of publications on this subject is increasing each year, providing evidence for the growing interest and importance of this issue. At the same time barely four articles provided some connection between vultures and UAS/aircrafts.
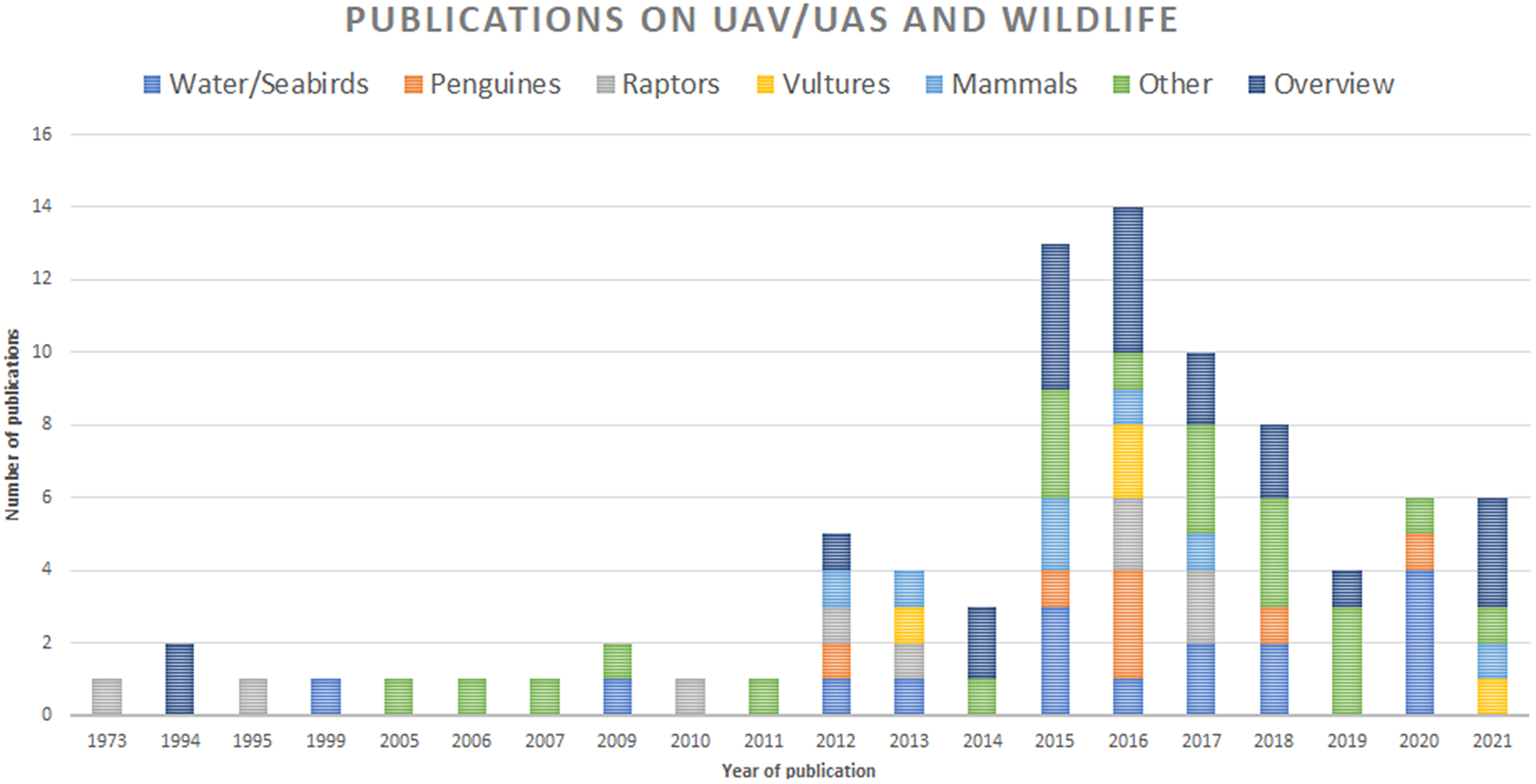
Figure 1. Total number of publications on UAV/UAS obtained from 1973 to 2021 (see text for details).
The literature review revealed two main uses of UASs in ornithological research: monitoring of breeding colonies, especially of gulls and terns (Sardà-Palomera et al. Reference Sardà-Palomera, Bota, Viñolo, Pallarés, Sazatornil, Brotons, Gomáriz and Sarda2012, Chabot et al. Reference Chabot, Craik and Bird2015, Scher Reference Scher2015), and penguins and waterbirds (Descamps et al. Reference Descamps, Béchet, Descombes, Arnaud and Zerubia2011, Chabot and Bird Reference Chabot and Bird2012, Duvala Reference Duvala2016, Hodgson et al. Reference Hodgson, Baylis, Mott, Herrod and Clarke2016, McClelland et al. Reference McClelland, Bond, Sardana and Glass2016, Edney and Wood Reference Edney and Wood2021); checking and monitoring individual nests of corvids (Weissensteiner et al. Reference Weissensteiner, Poelstra and Wolf2015) and tree-nesting raptors (Potapov et al. Reference Potapov, Utekhina, McGrady and Rimlinger2013, Junda et al. Reference Junda, Greene, Zazelenchuk and Bird2016, LPO AUVERGNE 2017).
Half of the articles discovered were overviews, discussing various practical and ethical aspects and describing the use of UASs in monitoring and conservation of whole groups of birds, or in providing practical technical solutions for individual counts and discovery surveys (50%). However, only 15% of the articles found referred to raptors and birds of prey and only four of these mentioned vultures in particular (T. Enkhtsetseg unpublished presentation, Using drones to survey raptor nests monitoring: case study of Cinerious Vulture Aegyphus monachus/nests in Kherlen Toono Nature Reserve; Soto-Largo et al. Reference Soto-Largo, Prada, Arroyo, García, Blanco, Durán and García2013, Hausheer Reference Hausheer2016, Tobajas et al. Reference Tobajas, Guil and Margalida2021).
The extent to which proximity to a UAV itself causes disturbance and triggers stress reactions was taken into account in most experiments, but so far has only rarely been accurately recorded and quantified. In the next section we explore the reported behavioural responses to UAVs in an attempt to predict the potential reactions of vultures.
Behavioural responses of birds to UAVs
Several overview studies provided extensive data on the advantages and disadvantages of using UAVs for studying colonial-, aquatic-, and seabirds, and also described their responses (Borrelle and Fletcher Reference Borrelle and Fletcher2017, Edney and Wood Reference Edney and Wood2021, Weston et al. Reference Weston, O’Brien, Kostoglou and Symonds2020). However, there were very few peer-reviewed publications on the responses of raptors to UAVs.
The reactions of the species studied varied from “no behavioural reaction” to either horizontal and vertical flights (Vas et al. Reference Vas, Lescroël, Duriez, Boguszewski and Grémillet2015), to “significant disturbance”, and no habituation to drone presence (Rümmler et al. Reference Rümmler, Mustafa, Maercker, Peter and Esefeld2016). Some of the studies suggested that drones may reduce investigator impact (Borrelle and Fletcher Reference Borrelle and Fletcher2017, Bushaw et al. Reference Bushaw, Ringelman, Johnson, Rohrer and Rohwer2020, Krause et al. Reference Krause, Hinke, Goebel and Perryman2021), while others suggested that in most cases responses triggered by UAVs at any altitude appeared to last longer than the response resulting from counts made on foot (Weston et al. Reference Weston, O’Brien, Kostoglou and Symonds2020). The limited studies on birds of prey provided evidence for critical periods, during which rotor-winged aircraft surveys could cause nest abandonment, egg breakage, or egg dumping (White and Sherrod Reference White and Sherrod1973), or reported a behavioural response similar to that observed during routine on-foot nest inspections (Potapov et al. Reference Potapov, Utekhina, McGrady and Rimlinger2013).
Aggression of birds towards UAVs
It is important to assess the possibility that UAVs may trigger an aggressive response because vultures are generally large, heavy birds, with the potential to damage a UAV and possibly suffer serious injury themselves. The limited amount of footage available online cannot exclude aggression towards UAVs by vultures; there may simply be a paucity of filmed evidence, as other evidence exists of aerial attacks by large raptors on paragliders, paramotors, and hang-gliders flown for leisure, as well as on UAVs (Georgii et al. Reference Georgii, Zeitler and Hofer1994, Zeitler and Linderhof Reference Zeitler and Linderhof1994, Jenny Reference Jenny2010, Junda et al. Reference Junda, Greene, Zazelenchuk and Bird2016), even though in some cases the response was comparable to that resulting from ground surveys (Junda et al. Reference Junda, Greene, Zazelenchuk and Bird2016). At the same time there is evidence of tolerance or interest and joint flights, but there is no quantification or assessment of the circumstances around these interactions (i.e. season, proximity to the nest, time of day), especially for territorial vulture species which tend to show aggression towards conspecific and heterospecific species during the breeding season, i.e. Bearded Vultures (Margalida and Bertran Reference Margalida and Bertran2005).
In any case, species-specific and individual behaviours should be well-known to researchers and considered when using UAVs in order to avoid aggression-related accidents and damage to both birds and the UAV, especially when working with vultures. It should be noted that attacks on UAVs filmed and available on YouTube and other data-sharing platforms do not occur exclusively during the breeding season and/or in territorially defended areas, and such possibility should never be excluded when designing a study with UASs.
Physiological responses of birds to UAVs
Direct behavioural observations alone only partially reflect the physiological state of wild animals and cannot adequately assess stress or other internal responses. Clear discrepancies between a lack of behavioural response and marked changes in cardiac rhythm as the result of an approaching UAV were observed in American Black Bears (Ursus americanus) (Ditmer et al. Reference Ditmer, Vincent, Werden, Tanner, Laske, Iaizzo, Garshelis and Fieberg2015). Although there are no direct observations on raptors, we found studies suggesting links between behavioural and physiological responses in colonial ground-nesting waterbirds, with potential impacts on reproductive output and overall mortality (Borrelle and Fletcher Reference Borrelle and Fletcher2017, Weimerskirch et al. Reference Weimerskirch, Prudor and Schull2018).
These studies illustrated the high interference potential of UAV use for wildlife monitoring purposes and show the necessity for further research on this topic, particularly in vulture monitoring and research where assessment is currently lacking.
Vultures and disturbance
The sections below describe the four vulture species that occur in Europe, highlighting relevant ecological and behavioural aspects and responses to human disturbance which could be relevant to the practical use and potential impact of UAVs in vulture conservation.
Bearded Vulture
Bearded Vultures (BVs) usually build their nests on steep cliffs, often at very high altitude, and occupy large home ranges while defending a relatively small territory (Margalida and Bertran Reference Margalida and Bertran2000a,b, Reference Margalida and Bertran2005, Ferguson-Lees and Christie Reference Ferguson-Lees and Christie2001, García-Jiménez et al. Reference García-Jiménez, Pérez-García and Margalida2018). Access to their nests is difficult, making them particularly interesting for UAV studies, for example to determine their breeding phenology (start of incubation, hatching period, etc.), or clutch size. This information can be valuable in determining the sensitive periods for breeding failure or adaptive management actions such as the rescue of the second chick in double clutches (Margalida et al. Reference Margalida, Benítez, Sánchez-Zapata, Ávila, Arenas and Donázar2012, Colomer et al. Reference Colomer, Oliva‐Vidal, Jiménez, Martínez and Margalida2019).
A number of studies in Spain and France prove that human and other disturbance negatively impact the breeding success of BVs, often leading to nest abandonment during incubation (Donazar et al. Reference Donazar, Hiraldo and Bustamante1993, Margalida et al. Reference Margalida, García, Bertran, Heredia, Garcia, Bertran, Heredia, García, Bertran and Heredia2003, Arroyo and Razin Reference Arroyo and Razin2006). Studies showed significant individual differences in response to disturbance (Arroyo and Razin Reference Arroyo and Razin2006), with particular sensitivity during the pre-fledging period (Arroyo and Razin Reference Arroyo and Razin2006, Arroyo et al. Reference Arroyo, Lafitte, Sourp, Rousseau, Albert, Heuacker, Terrasse and Razin2021). It has also been suggested that nest abandonment caused by disturbance might result in more general or delayed impacts on breeding success, such as pair instability, increased intra-specific competition, and loss of optimal breeding territories (Arroyo and Razin Reference Arroyo and Razin2006, Margalida et al. Reference Margalida, Benítez, Sánchez-Zapata, Ávila, Arenas and Donázar2012).
The evidence that BV breeding is negatively impacted by disturbance in breeding territories suggests that this species is likely to be sensitive to passing UAV flights, particularly during the breeding season (i.e. incubation and the first weeks after hatching) (see Table 1, Figure 2).
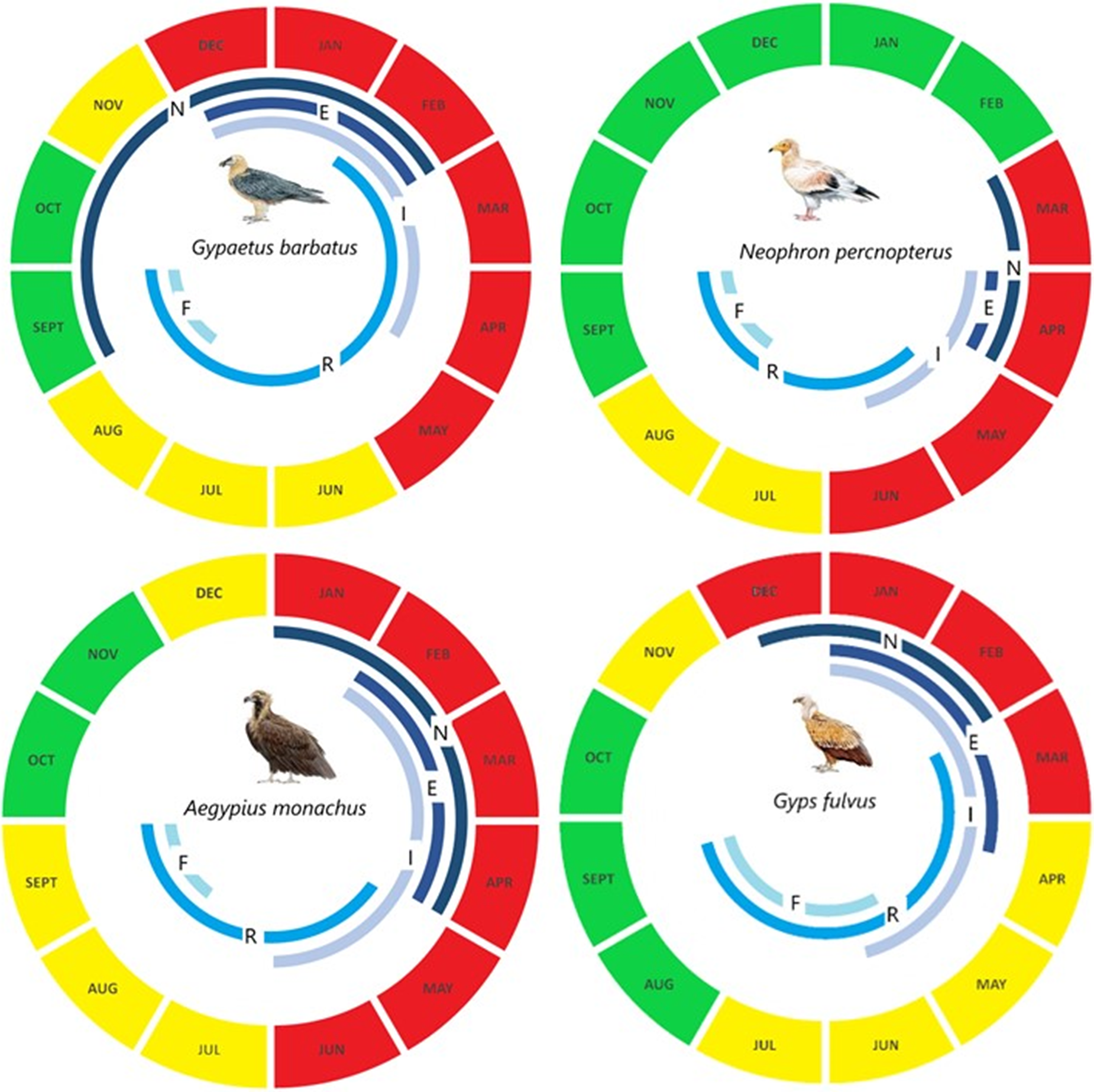
Figure 2. Phenological cycle of Egyptian Vulture (Neophron percnopetrus), Cinereous Vulture (Aegypius monachus), Griffon Vulture (Gyps fulvus), Bearded Vulture (Gypaetus barbatus) and critical periods for UAV operations, based on breeding data for the Iberian and Balkan Peninsula, Central Europe, the island of Crete, and Turkey, as presented in Table 1 (red = critical period; yellow = moderate risk; green = low risk).
Table 1. Breeding phenology of vultures, based on data in Europe (including the island of Crete).

Egyptian Vulture
Egyptian Vultures (EVs) prefer low, south-facing cliffs along river valleys in hilly or mountainous areas (Ferguson-Lees and Christie, Reference Ferguson-Lees and Christie2001). Most of their nests are in caves, more or less covered by an overhang for weather protection (Olea and Mateo-Tomás Reference Olea and Mateo-Tomás2009). The use of UAVs could potentially improve the available information on their breeding phenology, or the number of chicks present at the nest (EVs are the only European vulture species that can rear two chicks in a single nest).
EVs are moderately gregarious and the nests of neighbouring pairs can be only a few 100 m apart in densely populated areas (Messabhia et al. Reference Messabhia, Ababsa and Saheb2014), but a breeding pair usually defends a territory of over 10 km2, especially against conspecifics (Margalida et al. Reference Margalida, García and Cortés-Avizanda2007). EVs prefer to nest some distance from areas densely occupied by humans, suggesting disturbance is a significant factor in nest selection (Liberatori and Penteriani Reference Liberatori and Penteriani2001, Sarà and di Vittorio, Reference Sarà and Di Vittorio2003, Carrete et al., Reference Carrete, Grande, Tella, Sánchez-Zapata, Donázar, Díaz-Delgado and Romo2007, Mateo-Tomás and Olea Reference Mateo-Tomás and Olea2015, Şen et al. Reference Şen, Tavares and Biligin2017).
Human activities and direct persecution have had direct impacts on the breeding success of EVs (Liberatori and Penteriani Reference Liberatori and Penteriani2001, Zuberogoitia et al. Reference Zuberogoitia, Zabala, Martínez, Martínez and Azkona2008), and have been suggested as the main factors affecting breeding output (Zuberogoitia et al. Reference Zuberogoitia, Zabala, Martínez, González-Oreja and López-López2014).
Nest monitoring by UAV at eight EV nests in Bulgaria in the period 2017–2020 reported that adults showed signs of disturbance, but no aggression, while chicks retreated to the inside of the nesting cave when approached. Adults behaved as if approached by another bird and would return to the nest as soon as the UAV had left. The nests were closely monitored throughout the breeding season, and no long-term negative impacts on chick survival and fledging were recorded.
Griffon Vulture
Griffon Vulture (GV) is a cliff-nesting species that breeds in large colonies, sometimes comprising more than 100 pairs (Cramp and Simmons Reference Cramp and Simmons1980, Freund et al. Reference Freund, Bahat and Motro2017), and should receive special consideration when discussing UAV disturbance. Birds often breed at high elevation, usually in the uppermost parts of rock walls, at the edges of karst plateaus, and in deep gorges, and enjoy higher breeding success in sheltered sites with rock overhangs (del Hoyo et al. Reference del Hoyo, Elliott and Sargatal1994).
Various studies suggested that GVs are generally susceptible to human disturbance (Donázar Sancho et al. Reference Donázar Sancho, Ceballos Ruiz, Fernández Leon, Meyburg and Chancellor1989), yet there are no particular studies on the direct impacts. Various authors reported nestling mortality due to disturbance caused by tourists approaching in boats below nesting cliffs in Croatia (van Beest et al. Reference Beest, Bremer, De Boer, Heitkönig and Monteiro2008, Susic and Radek Reference Susic, Radek and Andevski2013, Aresu et al. Reference Aresu, Rotta, Fozzi, Campus, Muzzeddu, Secci, Fozzi, De Rosa and Berlinguer2021). GV researchers and conservationists in France have observed that when approached by UAVs, foraging GVs all responded with evasive flights (R. Néouze and O. Duriez, pers. comm). However, when UAVs approached from above, this provoked a massive disturbance with additional take-off of all individuals. In contrast, in the same area, the presence of a large and noisy TV hexacopter UAV only induced the curiosity of a GV, as it approached in flight and observed the UAV, and circled with the UAV without any sign of disturbance. Thus, the behaviour of the GVs at roost and on the ground cannot be used as a proxy to a UAV approaching to monitor nest content, based on the sole experiment made on feeding vultures on the ground (O. Duriez, pers. comm).
Cinereous Vulture
Cinereous Vultures (CVs) usually form loose colonies, in some cases with the active nests only a few 100 m apart. They are considered semi-territorial, and adults actively defend their current nest during the breeding season, at least when invaders are close by (especially other vultures) (Cramp and Simmons Reference Cramp and Simmons1980). In southern Europe, CVs prefer nesting on mature oak or pine trees on steep slopes, surrounded by openings or with short foliage (Fargallo et al. Reference Fargallo, Blanco and Soto-Largo1998, Donázar et al. Reference Donázar, Blanco, Hiraldo, Soto-Largo, Soto-Largo and Oria2002, Poirazidis et al. Reference Poirazidis, Goutner, Skartsi and Stamou2004, Moreno-Opo et al. Reference Moreno-Opo, Fernández-Olalla, Margalida, Arredondo and Guil2013).
Some studies suggested that large tree-nesting raptors nest further from roads than large cliff-nesting raptors (Martínez-Abraín et al. Reference Martínez-Abraín, Oro, Jiménez, Stewart and Pullin2010). Various studies indicated that nest site selection and/or breeding success of CVs are impacted by disturbance (Donázar et al. Reference Donázar, Blanco, Hiraldo, Soto-Largo, Soto-Largo and Oria2002, Morán-López et al. Reference Morán-López, Sánchez Guzmán, Costillo Borrego and Villegas Sánchez2006, Moreno-Opo et al. Reference Moreno-Opo, Fernández-Olalla, Margalida, Arredondo and Guil2013). Margalida et al. (Reference Margalida, Moreno-Opo, Arroyo and Arredondo2011) found that pairs exposed to intrusive anthropogenic activity showed lower breeding success and that reaction to disturbance was determined by distance and noise level. Other studies suggested a correlation between nest failure and recreational and economic activities, such as mining and forestry (Kirazl and Yamaç Reference Kirazli and Yamaç2013), and speculated that the sensitivity of CVs increases with increasing accessibility/visibility of nest sites (Kirazli Reference Kirazli2016). Furthermore, behavioural changes, such as changes in flight activity, altitude, and area covered were reported in CVs in the presence of paragliders (Soto-Largo et al. Reference Soto-Largo, Prada, Arroyo, García, Blanco, Durán and García2013).
Particularly interesting is a study on the nesting and breeding success of CVs in Kherlen Toono Uul Nature Reserve, Mongolia, using UASs to document breeding success during both the egg-laying and chick-rearing phases (Hausheer Reference Hausheer2016). The study was carried out at the end of July 2015, a few weeks before chicks fledged (Hausheer Reference Hausheer2016, T. Enkhtsetseg pers. comm.), and reported no particular response from parents or other raptors, although one out of seven chicks displayed aggression towards the drone, which was interpreted as a sign of stress (T. Enkhtsetseg pers. comm.)
Interspecific and intraspecific interactions
Some field researchers have suggested that birds respond to UAVs as they would to a flying predator or other bird (Weissensteiner et al. Reference Weissensteiner, Poelstra and Wolf2015). However, there was also evidence that wild birds do not react to UAVs as strongly as they would to typical aerial predators, even though UAVs represent novel aerial objects (McEvoy et al. Reference McEvoy, Hall and McDonald2016). Some authors suggested that many individuals displayed a level of confusion and were reluctant to approach a UAV with the same aggressive behaviour that may be shown towards an intruder or predator, perhaps because an approaching machine does not elicit a well-developed evolutionary response (Junda et al. Reference Junda, Greene and Bird2015).
When considering the possible reactions of vultures to UAVs, it should be noted that intraspecific and interspecific aerial and close-to-nest interactions are common in this group. Although such interactions rarely end in aggressive attacks or injuries, they may sometimes result in a reduction of breeding success.
BVs, the least gregarious species, are known to often lose nests to other species. There are records of competitors flushing the occupant or impeding nest-changeover during incubation, e.g. aggressive, intimidating flights by Golden Eagles (Aquila chrysaetos) around a BV’s nest (Margalida and García Reference Margalida and García1999). Another study on the interactions between GVs and BVs reported that 80.9% of observed BV attacks on GVs occurred less than 300 m from the BV nest (Bertran and Margalida Reference Bertran and Margalida2002). Almost all observed attacks involved an aerial chase of the flying target and only 5% ended in physical aggression, all of which were within 100 m of the nest. There is evidence that males are significantly more aggressive pre-laying and that aggression levels of both sexes increase significantly as the nesting cycle progresses (Margalida and Bertran Reference Margalida and Bertran2000a,Reference Margalida and Bertranb, Reference Margalida and Bertran2005).
There are very little data on EVs, but frequent attacks on immature and adult conspecifics, and other cliff-nesters, have been documented near the nest (Mateo and Olea Reference Mateo and Olea2007). When considering the potential reaction to UAVs, it should be noted that there is strong evidence that disturbance by cliff-nesting Common Ravens (Corvus corax) can be correlated with a reduction in breeding success of EVs on the Balkan Peninsula (Oppel et al. Reference Oppel, Dobrev, Arkumarev, Saravia, Bounas, Manolopoulos, Kret, Velevski, Popgeorgiev and Nikolov2017).
Colonially nesting GVs only defend the immediate proximity of their nests (2–4 m) (Cramp and Simmons Reference Cramp and Simmons1980). One study reported a number of aggressive interactions, namely flights and attacks, which only targeted conspecifics (Xirouchakis and Mylonas Reference Xirouchakis and Mylonas2007). The level of observed aggression was related to stage of the breeding cycle, the age of the intruder, and the nest density. Another study reported intrusions at GV nests, even when one or both adults were present, yet very few of the agonistic behaviours ended in aggression (Bertran et al. Reference Bertran, Macià and Margalida2016).
Imperial Eagle (Aquila heliaca) and White-tailed Eagle (Haliaeetus albicilla) attacks on adult CVs over the nesting tree have been reported, even leading to their landing on the nest while vultures were incubating, although the impact on CV breeding success was not reported (Vasilakis et al. Reference Vasilakis, Poirazidis and Elorriaga2008).
Based on such evidence, aggression and aerial chases towards UAVs can be expected, especially when they are very close to the nests of territorial species. Additionally, individuals might be flushed from their nests, presenting potential dangers to the eggs or chicks in poor weather or when potential predators (e.g. Common Ravens) are present in the area.
Recommendations on the use of UASs for monitoring, research, and conservation of vultures in Europe
The potential of UASs to increase research efficiency is obvious and ethical protocols in scientific projects can justify their use in sensitive periods and sites (i.e. breeding season and nests).
Considering the evidence, we collected for the negative impacts of human disturbance and noise on vulture breeding activity and the lack of sufficient assessments of the specific effects of using UAVs to monitor vultures, and following the precautionary principle, we provide some species-specific recommendations which should be followed whenever UASs are considered for vulture research. These should be updated as new evidence becomes available.
-
• No use of UAVs at, or close to, active nests during the pre-laying or breeding periods (see Figure 2), unless there is strong evidence of an emergency need, for example, an adult or chick is suspected to have suffered injury or illness.
-
• UAVs should be considered, within a detailed emergency plan, to rescue chicks in the worst-case scenarios of abandonment by parents or when they have fallen from the nest.
-
• UAVs should be operated only for the minimum in-flight time, at the maximum possible distance from the nest, and at the maximum flight altitude required, given the need to acquire sufficient resolution of the recorded images. UAV operations should cease immediately if signs of aggression or extreme disturbance are seen.
-
• A minimum UAV take-off distance of 100 m from birds and nests should be adhered to. Consideration should be given to the size of the UAV, with larger platforms operating at greater distances, since these produce greater responses over longer distances than small ones (Vas et al. Reference Vas, Lescroël, Duriez, Boguszewski and Grémillet2015, Weston et al. Reference Weston, O’Brien, Kostoglou and Symonds2020).
-
• Target-oriented flights should be avoided, with no flights aimed directly at the target bird/nest and no rapid changes of speed/direction of flight near the monitored objects, in order to reduce changes in noise intensity; vertical flights should be avoided in favour of horizontal and regularly spaced surveillance patterns; UAVs should not fly directly over birds (Vas et al. Reference Vas, Lescroël, Duriez, Boguszewski and Grémillet2015, Mulero-Pázmány et al. Reference Mulero-Pázmány, Jenni-Eiermann, Strebel, Sattler, Negro and Tablado2017, Weston et al. Reference Weston, O’Brien, Kostoglou and Symonds2020).
-
• The risks of flushing birds and nest abandonment provoking breeding failure should be considered; take-off should only be performed when there are no potential predators detected in the area and low temperatures or adverse weather conditions favouring breeding failure occur.
-
• There should be rigorous documentation of any flight surveys and their impact, using a standardised protocol so that results can be published, analysed, and compared. Furthermore, protocols should distinguish cliff-nesting and tree-nesting species, as well as nesting, roosting, and foraging sites.
-
• When considering the critical phenological periods, shown in Figure 2, it should be kept in mind that they are based on a wide geographical area (i.e. Iberian Peninsula, Central Europe, Balkan Peninsula, and the island of Crete, and Turkey) and that regional variations may exist. The data on the nest defence distances in Table 2 and the data presented in Table 3 should also be taken into consideration when working with each specific vulture species.
Table 2. Defence territorial distances documented for European vultures.

Table 3. Critical periods to avoid flight operations and guidelines based on disturbance studies reported in the scientific literature.
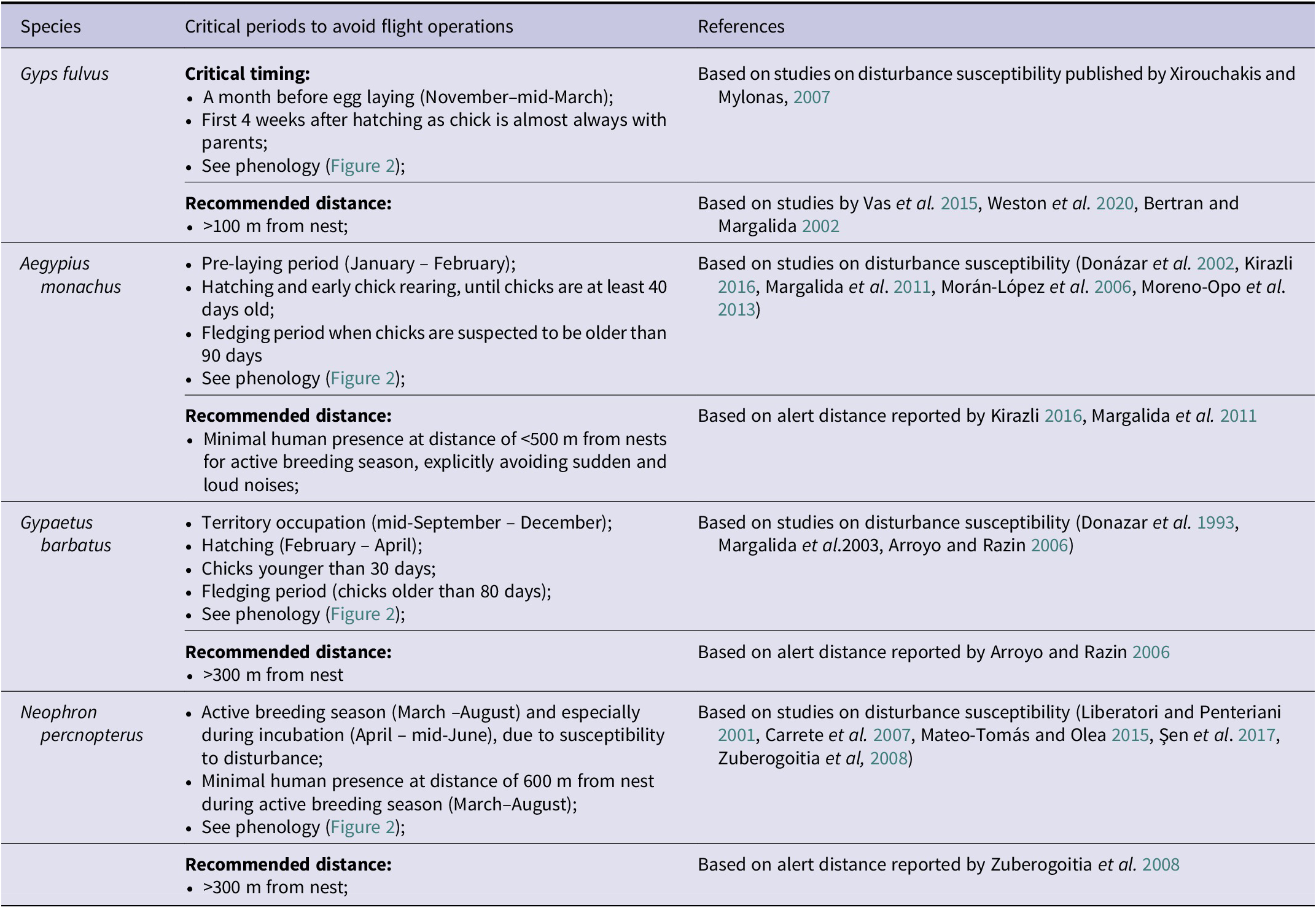
Bearded Vulture
-
• Well-planned flights and photographs of abandoned and unoccupied nests, or outside the actual breeding season, can provide valuable information regarding nest exposure, condition, or contents to improve our knowledge regarding breeding failure.
Egyptian Vulture
-
• As EVs do not strongly defend themselves and their broods, they are generally exposed to quite high pressure from predators and competing species (Eagle Owl Bubo bubo and corvids) (Morant Extebarria et al. Reference Morant Etxebarria, López-López and Zuberogoitia Arroyo2019). Due to the generally precarious state of EV conservation, nest-to-nest UAV operations should only be carried out in areas where the population is stable. Furthermore, given the strong evidence that EVs are sensitive to disturbance (Zuberogoitia et al. Reference Zuberogoitia, Zabala, Martínez, Martínez and Azkona2008), monitoring with UAVs during the breeding season should only be done with extreme caution, or as a last resort (van Beest et al. Reference Beest, Bremer, De Boer, Heitkönig and Monteiro2008), when there is strong evidence of an emergency situation, i.e. an adult or chick is in distress.
-
• Flight missions to already deserted nests may be carried out outside the breeding season (October–February) to inspect food remains, nest substrate, etc.
-
• Test flights may be made to roosting sites or to the vicinity of supervised supplementary feeding sites to document bird behaviour and collect valuable information on roosting and feeding. Results should however not be used as a proxy to suggest the behaviour of the species near nest and while breeding.
Cinereous Vulture
-
• As a tree breeder otherwise difficult to access, CVs seem to be comparatively well-suited to UAV nest checks. However, we consider CVs to be the most sensitive of all the four European vultures and susceptible to disturbance from UAVs. A discrete approach is virtually impossible due to the nature of the species’ nesting terrain and the reaction of CVs to an approaching UAV is impossible to predict. Additionally, where nest sites are adjacent, a UAV could simultaneously disturb several pairs. Comparatively isolated breeding sites in use by experienced CV pairs are therefore preferable for any testing of UAS operations.
-
• The risk of aggression towards UAVs should be especially considered when studying CVs. The pilot should therefore make all approaches with particular care and have prepared strategies for evasive manoeuvres, retreat, and emergency landings.
Griffon Vulture
-
• Due to their abundance and comparatively good conservation status, GVs are well suited for testing the use of UAS. The behaviour of a group or colony when approached by a UAV should be documented, carefully considering the potential for causing the failure of nearby breeders. It may be reasonable to also target foraging or roosting individuals outside the breeding season to test their response. It should however be noted that the behaviour of GVs in these situations may differ significantly from that at breeding sites, or close to the nest, so researchers should be cautious when making general conclusions based on the level of disturbance and possible tolerance of UAVs outside the breeding season. At the same time the response of GV should not be used as a proxy for other vultures, since results may not be directly transposable to other species, given that behaviour and sensitivity to disturbance may not be correlated to phylogenetic proximity. Test flights should be carried out on individual nestlings, as well as non-breeding birds, before attempting widespread nest checks.
Other potential uses of UASs in vulture conservation
It should be noted that the use of UASs in vulture conservation should not only be considered in studies of their breeding ecology (e.g. breeding parameters, identification of prey items delivered to the nest, or to discover the causes of breeding failure). The technology offers other possible applications of great benefit.
-
• Identification and isolation of dangerous powerlines. Drones have already been successfully used in the French Vanoise National Park to install anti-collision bird markers to indicate powerlines posing a threat to BVs (VCF 2018). UAVs can also be used to survey power lines for electrocution casualties and to map hotspots and particularly dangerous pylons in rough terrain (Mulero-Pázmány et al. Reference Mulero-Pázmány, Negro and Ferrer2014).
-
• Identification of individual birds and counts of roosting or foraging birds. Successful methods have been developed for waterbirds (Duvala et al. 2016), and the potential of automated drone-based detection has been recognised for mammals to overcome biases due to behavioural and environmental factors (Corcoran et al. Reference Corcoran, Denman and Hamilton2021). Furthermore, there are many marked vultures in Europe (e.g. with clearly visible tags, PVC rings, and transmitters) and high-resolution cameras could be used for individual identification at roosting, watering, or foraging sites, provided that minimum disturbance is guaranteed.
-
• Location of individual birds. UASs have great potential in locating individual birds fitted with tracking devices in areas otherwise difficult to access, especially when incidents are suspected. In addition, drone-based triangulation of radio-tracked animals has indeed great potential to become increasingly available (Saunders et al. Reference Saunders, Nguyen, Cowen, Magrath, Marsh, Bell and Bobruk2022). Cliff et al. (Reference Cliff, Fitch, Sukkarieh, Saunders and Heinsohn2015) used a small aerial robot to autonomously locate radio-tagged wildlife. Drones may therefore be useful for locating dead tagged birds by visiting their last known coordinates or exploring the area around about to provide a quick response to poisoning, poaching, and collision incidents.
-
• Assistance for chick rearing. In July 2020, a military drone was used in Israel to provide additional food for a nearly fledged GV, whose mother was confirmed to have been electrocuted. The experiment was successful, and the chick fledged in good health (ITV News 2020: https://www.youtube.com/watch?v=S1RZKeO01s8).
-
• Surveying large-scale wildlife poisoning scenes in hardly accessible habitats to assess the extent and severity of poisoning events and remotely search for carcasses of poisoned animals for recovery and removal from the landscape following proper processing. This method has been successfully used at poaching scenes in Africa (Bergenas et al. Reference Bergenas, Georgieff and Stohl2013; Duporge Reference Duporge2016)
-
• Habitat surveys and modelling of occupancy and abundance. There are studies showing that the capabilities of contemporary UASs can offer a less invasive, non-hazardous, repetitive, and reliable monitoring technique to collect data on species abundance and distribution (Jiménez López and Mulero-Pázmány Reference Jiménez López and Mulero-Pázmány2019), which can also be used to model habitat suitability and map occurrence.
-
• Locating potential vulture food sources. Abundance and distribution surveys have been successfully conducted for Red Deer (Cervus elaphus) (USGS 2014) and Fallow Deer (Dama dama) (Israel Reference Israel2012). The necessary precautions to guarantee that the subjects are not stressed must be considered and undertaken.
-
• UASs in conjunction with GPS logging. Rodríguez et al. (Reference Rodríguez, Negro, Mulero, Rodríguez, Hernández-Pliego and Bustamante2012) used GPS loggers mounted on adult Lesser Kestrels (Falco naumanni) to record basic spatiotemporal data during foraging for habitat and behavioural analyses. The recorded routes were then “flown” by a programmed UAV to better understand the movement patterns, visual stimuli, and ecology of the species. In the future, this tool could be used for specific behavioural studies on species with reduced foraging areas such as breeding BVs (around 50 km2) (Margalida et al. Reference Margalida, Pérez-García, Afonso and Moreno-Opo2016) or EVs. For example, focusing on the identification of preferred selected areas (patches) to obtain food resources, or to improve our knowledge on less documented aspects, such as bone-breaking behaviour at ossuaries or the use of cosmetics at colouring pits, or the impact of rural abandonment and the passive rewilding effects on scavenging efficiency (Oliva-Vidal et al. Reference Oliva-Vidal, Hernández-Matías, García, Colomer, Real and Margalida2022), are some possibilities for improving our understanding of the complex social life of vultures, critical to harmonising their conservation with anthropogenic activities (van Overveld et al. Reference van Overveld, Blanco, Moleón, Margalida, Sánchez-Zapata, de la Riva and Donázar2020).
Conclusions
The use of UASs can often be justified by reduced research effort, time, financial cost, and the prevention of health risks to personnel. Reducing disturbance of wild animals is the main argument used for the deployment of modern techniques for monitoring and photography. However, the disturbance potential of UAV use is still poorly understood and requires further investigation. A significant unknown is the physiological responses of animals to UAV exposure and the potential for long-lasting effects on health and reproductive success. To fill this gap, carefully documented UAV test missions in captivity or in the wild are urgently needed. It is crucial to develop universal standardised monitoring protocols to unify the methodologies for reporting disturbance and bird responses to allow comparison and common processing of the data collected. Furthermore, considering the difference of the aerial approach to cliffs and trees and the different disturbance patterns and vulnerability displayed by the studied species, we recommend distinguishing between protocols for control of cliff-nesting and tree-nesting species, as well nesting, roosting, and foraging sites. These protocols may not be restricted to only the European vulture species but utilised worldwide after being adapted to the specific phenological periods of different species and their behavioural characteristics.
Many studies and nest-checks on European vultures could be facilitated by the use of UAVs in future, once additional data are available to prove that there are no long-term negative impacts on their productivity. Due to their favourable conservation status and population trend, the large and stable populations of GVs offer the best opportunity to attempt test nest-checks using UAVs. Initial trials could be conducted outside the breeding season on unoccupied nests or individuals aggregating away from their breeding sites. Roosting sites and managed supplementary feeding sites offer excellent opportunities to document the responses of different vulture species to their first experience of an approaching UAV, and to consider the presence of other potentially sensitive or protected species in the proposed survey areas. It should however be noted that responses at the nest or during reproduction might differ significantly, both at the species and individual level.
However, the high sensitivity and territorial behaviour of most vulture species pose significant challenges to the use of UAVs. Outside the reproductive period, and in inactive vulture breeding grounds, UAV missions offer the possibility to document nests and their contents, but the breeding ecology of the species concerned should be thoroughly considered beforehand.
Therefore, considering the lack of sufficient data on long-term disturbance effects, and applying the precautionary principle, we generally discourage the regular use of UAVs for nest-checks on active vulture nests during the most sensitive breeding phases, as well as in poor weather or when potential predators are present nearby. Exceptions are situations where the advantages outweigh the risks and there is a clear contingency plan in place in case of an emergency. Such attempts should be well documented and published in order to collect the evidence required to inform the future use of UAVs in vulture conservation.
We are not calling for an ultimate ban on the use of UAVs for vulture research, but rather are advocating for careful consideration of the circumstances and meticulous documentation of the impact, suggesting some practical recommendations on how to reduce disturbance. Our aim is to sensitise and focus the attention of the researchers to the potential negative impact of drones on vultures, since no systematic experiments have ever been performed and there is no peer-reviewed evidence on the impact (or lack of thereof).
Common guidelines, as well as applicable national, regional, or conservation regulations and requirements for UAS operations, must always be observed and missions should always be justified and approved by ethical committees, with sound scientific and conservation purposes. All flights should be properly registered with the relevant authorities and conservationists using UASs should always lead by example in their operations.
Acknowledgements
Special thanks to H. Frey, L. Phipps, J. Lahoz-Monfort, O. Duriez, and an anonymous reviewer for their constructive comments. The current study was developed within the position paper Overview and guidelines for the use of Unmanned Aircraft Systems (UAS) in ornithology of the Vulture Conservation Foundation. AM was supported by the Ministry of Science, Innovation and Universities (Project RTI2018-099609-B-C22). Authors’ contributions: Richard Zink and Stefan Agnezy conceived the ideas and designed the methodology and collected and analysed the data together with Elena Kmetova-Biro and Ivailo Klisurov. Richard Zink and Elena Kmetova-Biro led the writing of the manuscript. Antoni Margalida assessed and edited the text, tables and figures. All authors contributed critically to the drafts and gave final approval for publication.