Regular physical activity associated with a balanced diet can be an important factor in promoting health. However, high-intensity or exhaustive physical exercises can increase the susceptibility to liver and kidney injuries(Reference Wu, Chen and Huang1–Reference Lin, Jiang and Luo3), promoting chronic fatigue and overtraining, partly due to the high synthesis of reactive oxygen species(Reference Cruzat, Rogero and Borges4).
These compounds can have effects considered beneficial to the immune system and exert metabolic functions essential for cellular homoeostasis depending on the quantity produced(Reference Cruzat, Rogero and Borges4,Reference Strobel, Peake and Matsumoto5) . However, high concentrations may be involved with the development of several pathophysiological processes, such as DNA, proteins and cellular lipids damage(Reference Goldfarb, Garten and Cho6,Reference Caputo, Vegliante and Ghibelli7) .
Exercise is able to increase the use of oxygen 200 times above resting levels in active muscles, which can further induce oxidative stress(Reference Powers, Nelson and Hudson8). A way to avoid such damage is by increasing the concentration of antioxidants in the diet(Reference Peternelj and Coombes9), aiming at improving health and optimising the benefits of training(Reference Sgarbieri10).
In recent years, much attention has been paid to the possible role of bioactive compounds in preventing the deleterious effects of oxidative damage induced by exercise(Reference Wawrzyniak, Górnicka and Hamułka11). In this context, turmeric (Curcuma longa L.) stands out, and its main compound, curcumin (CCM), started to be studied in sports due to its antioxidant and anti-inflammatory potential(Reference Shanmugam, Rane and Kanchi12). Its rhizome has been widely used in the food industry, as a colourant, a flavour and culinary ingredient, making it easy to be incorporated into food and drinks(Reference Silva, Bolini and Antunes13).
Whey protein (WP) also deserves attention, because besides being present in a variety of sports supplements, several benefits have been reported regarding the combination of WP and physical exercise. WP have high biological value and are related to increased strength, muscle hypertrophy, stimulation of insulin secretion, in addition to preventing the oxidation of muscle proteins and keeping the levels of peroxidated lipids low(Reference Pescuma, Hébert and Mozzi14–Reference Teixeira, Silva and Lima16).
Considering that consumers have been concerned with their food and health, giving preference to foods that may bring some additional benefit in relation to traditionally traded products, a large number of foods with functional characteristics have been developed by the industry(Reference Ziegler and Sgarbieri17–Reference Siqueira, Machado and Stamford20). To date, no study has assessed the impact of the association of CCM with a protein supplement of high biological value, such as WP. Therefore, our objective was to evaluate the impact of this association on metabolic control, inflammation and oxidative stress in Wistar rats submitted to an exhaustive exercise protocol. Once the effectiveness of the product has been verified, it can be considered as an alternative drink to be made available to the market.
Facing such a scenario, the WP admixtured of CCM seems to meet the demands of consumers, since this spice would increase the nutritional characteristics of WP due to its antioxidant and nutritional potential(Reference Drobnic, Riera and Appendino21,Reference Sciberras, Galloway and Fenech22) . Furthermore, the potential health benefits of turmeric, such as its good safety profile, ease of acquisition, in addition to not being toxic even at high doses, led to the interest in incorporating it into functional food products as a nutraceutical ingredient(Reference Alamdari, O’neal and Hasselgren23,Reference Zou, Liu and Liu24) .
Materials and methods
Test product
Curcumin
The animals received CCM (Theracurmin®; Theravalues) highly absorbable and dispersed in colloidal nanoparticles. Theracurmin® consists of 30 w/w% CCM and shows twenty-seven times more bioavailability than conventional CCM.
Development of a whey protein concentrate by spray drying
Firstly, milk was submitted to microfiltration to obtain whey. Samples of 4 days of cheese production were collected. The whey was stored in plastic containers with a lid, identified and stored in a freezer (–80° C) until the moment of use.
The whey drying procedure was carried out in a single level spray dryer, single model (model MSD 1.0), equipped with a 1 mm diameter spray nozzle (Labmaq). The air and product flow rates, as well as the air temperature, were defined through initial tests(Reference Perrone, Simeao and Rodrigues Junior25), in which the inlet air and the outflow of the product were 170; SD: 2°C and 1 l/h.
The characterisation of the whey protein concentrate (WPC) was carried out according to the Association of Official Analytical Chemists(26), in triplicate. The moisture content was determined by the gravimetric method at 105°C until constant weight. Protein was determined by method 991.20 (n × 6·38)(26), lactose by method ISO 22662/IDF198(Reference Parente27), lipids by method 989.05(26) and ash by method 942.05(26). The results were expressed on a dry basis. The total energy value was calculated using the conversion factor of 16.736 kJ/g (4.0 kcal/g) for carbohydrates and proteins, and 37.656 kJ/g (9.0 kcal/g) for lipids(Reference Merrill and Watt28).
Whey protein concentrate admixtured of curcumin
CCM was added to WPC so that a product with a concentration of 0·8 g of CCM for each 100 g of WPC was obtained. For this calculation, an intake of 3·0 mg/kg per d of CCM (maximum value of acceptable daily intake for humans)(29) was considered for an individual of 70 kg who performs a protein substitution through WPC of 0·3 g/kg per d(Reference Schoenfeld and Aragon30). Thus, the replacement of protein in animals, using WPC, corresponded to 30 % of the requirements stipulated for rats in the maintenance phase(Reference Reeves, Nielsen and Fahey Junior31), in accordance with that substitution used for humans.
Determination of total phenolic compounds and antioxidant activity of whey protein concentrate, whey protein concentrate admixtured of curcumin and curcumin
Obtaining extracts
In order to prepare the extracts, 2 g of each sample and 20 ml of extraction solution composed of acetone: water (70:30 v/v) were used. The mixtures were subjected to agitation in a metabolic bath (DUBNOFF, model MA-093) (180 rpm) at room temperature for 15 min. Afterwards, the samples were centrifuged (Multi-purpose centrifuge, NF 1200/1200R, 14 000 rpm) for 15 min and the supernatant fractions were filtered through paper filter, and their volumes made up to 20 ml with extracting solution. The extracts were stored in an amber bottle at −18°C. Extract aliquots were used to estimate total phenolics and antioxidant testing.
Estimated concentration of total phenolics
The concentration of total phenolics in the extracts was estimated using the Folin–Ciocalteu reagent(Reference Singleton, Orthofer and Lamuela-Raventós32). 500 μl of the extract, 500 μl of 20 % Folin–Ciocalteu reagent diluted in water (Sigma-Aldrich) and 500 μl of 7·5 % sodium carbonate solution (diluted in water) were added to test tubes. Vortexing was performed (Bio Vortex V1 BIOSAN®) and then the samples were left to react for 30 min. The absorbance was read on a spectrophotometer (Thermo Scientific, Evolution 60S), at a wavelength of 760 nm. An analytical curve was constructed from the absorbance reading of solutions with different concentrations (0·006–0·09 mg/ml) of gallic acid (Sigma-Aldrich). The results were expressed in milligrams of gallic acid equivalents per gram of sample (mg AGE/g sample).
Determination of antioxidant activity
The radical removal activity of the samples was determined from the extracts prepared for the analysis of total phenolics, using the 2,2-diphenyl-2-picryl-hydrazila method(Reference Bloor33). A 100 μl aliquot of the extracts was added to 1500 μl of 2,2-diphenyl-2-picryl-hydrazila methanolic solution (Sigma-Aldrich). Vortexing was performed and the extracts were then left to rest for 30 min, protected from light. The absorbance was read on a spectrophotometer (Thermo Scientific, Evolution 60S) at 517 nm. The results were expressed in mM of trolox equivalent per gram of sample (mM trolox/g of sample). The analyses were performed at the Vitamin Analysis Laboratory (DNS/UFV).
Experimental design
Animals
Forty-eight male Wistar rats (young adults), 12 weeks old (Rattus norvegicus, variety albinus, Rodentia), were used. The animals were kept in individual stainless-steel cages, with a 12-h light/dark cycle and an average temperature of 22 ± 2°C, throughout the experimental period.
The experiment was developed at the Experimental Nutrition Laboratory of the Department of Nutrition and Health of the Federal University of Viçosa, Minas Gerais, Brazil, from January to April 2019 (acclimatisation period + 4 weeks of experiment).
Experimental diets
All animals received a standard commercial diet until they reached 12 weeks of age, when the experiment started with the allocation of animals to their respective treatment groups. Experimental diets were formulated according to the AIN-93M recommendations, proposed by the American Institute of Nutrition(Reference Reeves, Nielsen and Fahey Junior31) for the maintenance of adult animals, which are: AIN-93M diet (control), in which albumin was the source of protein; WPC + CCM diet, in which 30 % (36 g) of the protein requirement came from the WPC, considering its purity, and the remainder came from albumin, admixtured of CCM; and CCM diet, which consisted of AIN-93M diet admixtured of CCM, also considering its purity (Table 1).
Table 1. Composition of experimental diets

WPC, whey protein concentrate; CCM, curcumin.
* Albumin was considered to have 80 % protein.
All ingredients were weighed on a semi-analytical balance (Gehaka®, model BG2000), mixed manually and sieved through plastic sieves. The diets were made in powder and were stored protected from light, at temperatures from 0 to 4°C, until the moment of use. The animals received distilled water and ad libitum diet.
Experimental design
The animals were randomly divided into six experimental groups (n 8) (Fig. 1).

Fig. 1. Experimental design. G, group; AIN-93M, standard diet; WPC + CCM, concentrate whey protein admixtured of curcumin; CCM, curcumin; ET, animals submitted to the exhaustion test.
Exhaustive exercise protocol
The animals from groups G2, G4 and G6 were submitted to an acute exhaustive swimming test, after 4 weeks of consumption of the experimental diets. The animals were forced to swim until exhaustion, carrying a load correspondent to 5 % of their body weight attached to their tail. Exhaustion was defined when the rat remained submerged for 10 s or when it was no longer able to maintain its body movements(Reference Dawson and Horvarth34).
The test was carried out in a tiled masonry tank (width: 65 cm, length: 75 cm, height: 85 cm), with water height of 45 cm and temperature around 28°C ± 1°C.
Euthanasia and dissection of organs
The animals were euthanised 24 h after performing the exhaustive exercise by a trained team in a quiet and adequate environment, far from the experimentation room. After being subjected to a 12-h fast, the animals were anaesthetised for 3–5 min with 3 % isoflurane (Isoforine, Cristália®).
Blood was collected by cardiac puncture and subsequently centrifuged at 1006× g for 10 min (FANEM®) to obtain the serum, which was being stored at −80°C for further analysis. The liver was also weighed and frozen for analysis, with a small portion being separated for histological analysis.
Biometric and food consumption measures
The animals’ body weight was assessed weekly (Mettler Toledo®), and the final weight of each animal was subtracted from the initial weight to determine weight gain. Food consumption was also assessed weekly based on the weight difference (in g) between the amount of diet offered and the remaining amount not consumed in the feeders plus the leftover bottom of the cage. The BMI was obtained by the relationship between weight and the square of the naso-anal length(Reference Novelli, Diniz and Galhardi35). Abdominal circumference at the beginning and at the end of the experiment was obtained at the midpoint between the skull and the pelvic limbs of the animal(Reference Pereira, Teixeira and Aguilar36). The relationship between weight gain (g) and food intake was used to calculate the food efficiency coefficient [(final weight gain/total food consumption) × 100](Reference Silva, Dias and Moreira37).
Biochemical analyses
Plasma concentrations of total, direct and indirect bilirubin; uric acid, alkaline phosphatase, glucose, total proteins, albumin, globulin, albumin/globulin ratio, alanine aminotransferase and aspartate aminotransferase were evaluated. The methodological procedure was performed according to the recommendation of the kit manufacturer (Bioclin®, Inc.) and the readings performed on the BS-200 (Mindray®, Inc.).
Quantitative PCR
The quantitative PCR was performed using the GoTaq® 1-Step RT-qPCR System kit (Promega). For each reaction, 10 μl of Master Mix (2X), 0·4 μl of GoScript ™ RT Mix for 1-Step RT-qPCR (50X) and 0·4 μl of each primer (forward and reverse) were used. The concentration of 600 ng/μl of RNA was standardised and water was added to complete the final volume of 20 μl. The sequences of the primers used are described in Chart 1.
Amplification and detection were performed with the Eco™ Real-Time PCR system (Illumina) and the 2-ΔCt method was used to analyse the expression.
Oxidative stress analysis
Fragments of liver tissue were immediately frozen in liquid N2 (–196°C) and stored in a freezer at −80°C. The samples were homogenised in phosphate buffer and centrifuged at 4°C. The supernatant was stored for the analysis of malondialdehyde (MDA)(Reference Wallin, Rosengren and Shertzer38), nitric oxide (NO)(Reference Tsikas39), catalase (CAT)(Reference Aebi40), superoxide dismutase (SOD)(Reference Dieterich, Bieligk and Beulich41) and glutathione S-transferase (GST)(Reference Habig and Jakoby42). The formed pellet was used to quantify protein carbonyl (PC)(Reference Levine, Garland and Oliver43). Biochemical data were normalised according to the total protein levels in the supernatant(Reference Bradford44).
Histological analyses
Liver fragments from all animals were removed and fixed in formaldehyde at room temperature. After fixation, the tissues were dehydrated in an increasing gradient of ethanol (70 % to absolute) and included in resin containing hardener (Leica, Historesin®). 3-μm thick semi-serial histological sections were obtained in a Leica® automatic microtome using a glass razor and stained using the haematoxylin/eosin technique. The slides (Precision®) were mounted with Entellan (Merck®) and analysed under a light microscope (LEICA DM750).
The images of the histological sections were captured with 20× objective lens using a Leica MC170 HD digital camera. From ten photos per animal, the areas containing lipid vesicles and inflammatory infiltrate were quantified using the ImagePro-Plus® application version 4.5 (Media Cybernetics) through manual counting of points on the tissue. Histomorphometric analysis considered the insertion of 1230 points on the image.
The degree of steatosis was assessed according to a scale of five gradations: grade 0, if the percentage of fat was absent or less than 5 %; grade 1, if ≥ 5 % and < 25 %; grade 2, if ≥ 25 % and < 50 %; grade 3, if ≥ 50 % and < 75 %; and grade 4, if ≥ 75 %(Reference Turlin, Mendler and Moirand45).
The analyses were carried out at the Experimental Pathology (DBA/UFV) and Structural Biology (DBG/UFV) laboratories.
Statistical analysis
For the sample calculation, each animal was considered as a repetition, so that the difference in the average weight between the groups did not exceed 10 g. The probability of the type 1 error (α) used was 0·05 and the type 2 error (β) was 0·1.
The normality of the data was assessed using the Shapiro–Wilk test. Comparisons between two groups (group that did not perform the test × group that had undergone the swimming test) were performed using the t test or Mann Whitney depending on normality. The comparisons between all groups were performed by one-way ANOVA, followed by Tukey’s post hoc test for data with parametric distribution, whereas for non-parametric data, the Kruskal–Wallis test was applied, complemented by Dunn’s multiple comparisons test.
The processing and statistical analysis of the data were performed using the software SPSS version 20 for Windows (SPSS Inc.) and STATA version 13, with a statistical significance level of α 5 %. The results were expressed as mean values and standard deviation for results with parametric distribution and median and minimum and maximum for non-parametric results.
GraphPad Prism software, version 6 for Windows (GraphPad Prism Inc.) was used to construct the graphs.
Ethical aspects
This project was approved by the Animal Use Ethics Committee of the Federal University of Viçosa (CEUA/UFV), under protocol number 72/2018. The experiment was carried out in accordance with the National Animal Experimentation Control Council (Normative Resolution nº 37, 2018).
Results
Centesimal composition of whey protein concentrate
The WP developed and used in the present study was obtained through spray drying and showed a protein content of almost 88 % (Table 2).
Table 2. Centesimal composition of whey protein concentrate

TEV, total energy value; ED, energy density.
Concentration of total phenolic compounds and antioxidant activity of whey protein concentrate, curcumin and whey protein concentrate admixtured of curcumin
CCM showed higher concentrations of total phenolics and antioxidant capacity compared to WPC (P < 0·05) (Fig. 2). No significant difference was found in the concentrations of phenolics and in the antioxidant capacity of WPC + CCM in relation to CCM and WPC.

Fig. 2. Total phenolics (A) and antioxidant capacity (B) of concentrate whey protein (WPC), curcumin (CCM) and concentrate whey protein admixtured of curcumin (WPC + CCM). Data expressed as median (minimum–maximum). Medians followed by the same letter do not differ by the Kruskal–Wallis test complemented by the Dunn’s multiple comparison test.
Biometric and food consumption measures
The consumption of WPC + CCM, as well as the isolated CCM, did not change the animals’ biometric and food consumption measures (P > 0·05) (Table 3).
Table 3. Biometric and food consumption measurements of rats that were or were not subjected to an exhaustive swimming test
(Mean values and standard deviations)*

AIN-93M, standard diet group; AIN-93M ET, standard diet group submitted to exhaustion test; WPC + CCM, whey protein concentrate admixtured of curcumin group; WPC + CCM ET, WPC + CCM submitted to exhaustion test group; CCM, curcumin group; CCM ET, CCM submitted to exhaustion test group. WC, waist circumference; TFC, total food consumption; FEC, feed efficiency coefficient (n 8).
* Means or medians without letter mean that there were no significant differences among the experimental groups (P > 0·05) by the t test or Mann–Whitney test and one-way ANOVA, followed by the Tukey’s post hoc test or the Kruskal–Wallis test complemented by the Dunn’s multiple comparison test.
Biochemical variables
It has been observed that WPC + CCM was able to reduce the glycaemia of the animals when compared to the groups AIN-93M ET and CCM ET (P < 0·05). It has also been noted that in AIN-93 M group (control), the animals that were submitted to the exhaustion test (AIN-93M ET) showed higher aspartate aminotransferase values when compared to the ones that did not perform the exercise (AIN-93M) (P < 0·05) (Table 4).
Table 4. Biochemical variables of rats that were or were not subjected to an exhaustive swimming test*
(Median values and minimum–maximum)

AIN-93M, standard diet group; AIN-93M ET, standard diet group submitted to exhaustion test; WPC + CCM, whey protein concentrate admixtured of curcumin group; WPC + CCM ET, WPC + CCM submitted to exhaustion test group; CCM, curcumin group; CCM ET, CCM submitted to exhaustion test group. A/G ratio, albumin/globulin ratio; AST, aspartate aminotransferase; ALT, alanine aminotransferase. RV, reference value (n 8).
* Mean or median followed by the same letter on the same line do not differ. The lower case letters indicate comparisons among all groups by one-way ANOVA, followed by Tukey’s post hoc test or Kruskal–Wallis test complemented by Dunn’s multiple comparison test. Capital letters indicate comparisons among the same group (animals that were not subjected to the test × animals that were subjected to the swimming test) by t test or Mann–Whitney test. Means or medians without a letter mean that there were no significant differences among the experimental groups (P > 0·05).
Gene expression of TNF-α, IL-6 and IL-10
The gene expression of TNF-α and IL-6 was higher in the control group submitted to the exhaustion test (AIN-93M ET) when compared to the others (P < 0·05). IL-10 expression was higher in the WPC + CCM group compared to the other groups (P < 0·05), except for the WPC + CCM ET group, which did not show significant differences (Fig. 3).

Fig. 3. Levels of gene expression of TNF-α (A), IL-6 (B) and IL-10 (C), in the liver of sedentary rats that underwent or not to an exhaustive swimming test. Data expressed as mean values and standard deviations. Means with the same letters do not differ by one-way ANOVA, followed by Tukey’s post hoc test. AIN-93M, standard diet group; AIN-93M ET, standard diet group subjected to exhaustion test; WPC + CCM, whey protein concentrate admixtured of curcumin group; WPC + CCM ET, WPC + CCM submitted to exhaustion test group; CCM, curcumin group; CCM ET, CCM submitted to exhaustion test group (n 5). The results are expressed in relation to the AIN-93M group.
Oxidative stress
We observed that the animals in CCM and CCM ET groups showed lower concentrations of MDA, in relation to the groups AIN-93M and AIN-93M ET, respectively (P < 0·05). The AIN-93M group also showed higher MDA values, compared to the AIN-93M ET group (P < 0·05) (Fig. 4).

Fig. 4. Values of malondialdehyde (MDA) (A), protein carbonyl (PC) (B), nitric oxide (NO) (C), catalase (CAT) (D), superoxide dismutase (SOD) (E) and glutathione S-transferase (GST) (F) in the liver of rats that have or have not undergone an exhaustive swimming test. Data expressed as mean values and standard deviations or median (minimum–maximum). The lower case letters indicate comparisons among all groups by one-way ANOVA, followed by Tukey’s post hoc test or Kruskal–Wallis test complemented by Dunn’s multiple comparison test. Capital letters indicate comparisons between the same group (sedentary × swimming) using the t test or Mann–Whitney. AIN-93M, standard diet group; AIN-93M ET, standard diet group subjected to exhaustion test; WPC + CCM, concentrate whey protein admixtured of curcumin group; WPC + CCM ET, WPC + CCM submitted to exhaustion test group; CCM, curcumin group; CCM ET, CCM submitted to exhaustion test group (n 8).
The animals in the WPC + CCM and CCM groups also showed lower concentrations of PC, when compared to animals in the AIN-93M groups (P < 0·05). Among the animals that were submitted to the exhaustion test, the AIN-93M ET group showed the highest PC values, followed by the WPC + CCM ET and CCM ET groups (P < 0·05). The WPC + CCM ET group also showed higher concentrations of PC compared to the WPC + CCM group (P < 0·05) (Fig. 4).
Among the animals that performed the exhaustive swimming test, it was noted that the WPC + CCM ET was able to reduce the NO concentration, when compared to the groups AIN-93M ET and CCM ET (P < 0·05). Among those who did not swim, the WPC + CCM was able to increase the NO concentration, while the CCM group had lower values when compared to the WPC + CCM ET and CCM ET groups, respectively (P < 0·05) (Fig. 4).
As for CAT levels, it was observed that those who consumed the AIN-93M diet had higher enzyme concentrations, when compared to those who consumed CCM (P < 0·05). However, among those who swam, CCM group was the one with the highest concentrations of CAT in relation to the other groups (P < 0·05). The AIN-93M group had higher CAT values, while the CCM group had lower values, when compared to the AIN93-M TE and CCM ET groups, respectively (P < 0·05) (Fig. 4).
In relation to SOD, the group AIN-93M ET showed lower values in relation to its control group that did not perform the swimming test (AIN-93M) (P < 0·05). In addition, the animals in the AIN-93M ET group showed lower SOD values compared to the other groups (P < 0·05) (Fig. 4).
However, the same was not observed for GST, since among the animals that swam, the group AIN-93M ET showed higher concentrations in relation to the group that received WPC + CCM ET (P < 0·05) (Fig. 4).
Histology
We observed that the animals in the CCM ET group showed higher amounts of fat droplets when compared to the other groups (P < 0·05), except in relation to the CCM group (P > 0·05). As for the inflammatory infiltrate, the animals in the WPC + CMM ET group showed smaller areas of inflammation when compared to the AIN-93M ET group (P < 0·05), corroborating the results found through the analysis of inflammatory cytokine gene expression (Table 5) (Fig. 5). This finding demonstrates that WPC + CCM was able to reduce inflammation caused by exhaustive exercise.
Table 5. Histomorphometry of the liver of rats that were or were not subjected to an exhaustive swimming test*
(Mean values and standard deviations)

AIN-93M, standard diet group; AIN-93M ET, standard diet group submitted to exhaustion test; WPC + CCM, whey protein concentrate admixtured of curcumin group; WPC + CCM ET, WPC + CCM submitted to exhaustion test group; CCM, curcumin group; CCM ET, CCM submitted to exhaustion test group (n 6).
* Means followed by the same letter in the same column do not differ. The lower case letters indicate comparisons among all groups by one-way ANOVA, followed by Tukey’s post hoc test. Capital letters indicate comparisons among the same group (sedentary × swimming) using the t test.
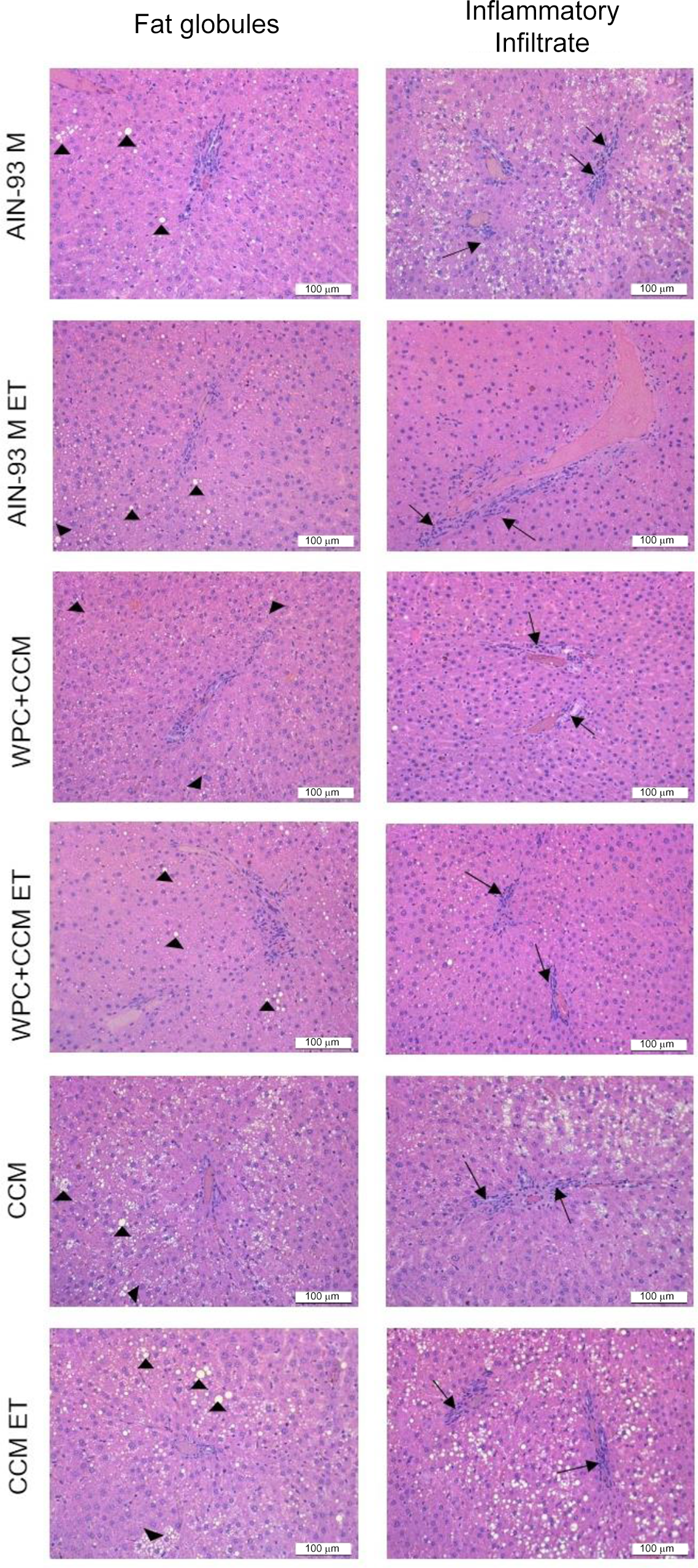
Fig. 5. Photomicrographs of the liver of sedentary rats that were or were not subjected to an exhaustive swimming test. AIN-93M, standard diet group; AIN-93M ET, standard diet group submitted to exhaustion test; WPC + CCM, whey protein concentrate admixture of curcumin group; WPC + CCM ET, WPC + CCM submitted to exhaustion test group; CCM, curcumin group; CCM ET, CCM submitted to exhaustion test group. Fat globules (►); inflammatory infiltrate (→); scale = 100 μm; haematoxylin–eosin staining; (n 6).

Chart 1. Primer sequences for qPCR analysis.
We also observed that all groups showed grade I steatosis, that is, they showed fat percentage ≥ 5 % and < 25 % (Table 5).
Discussion
Stress is a condition induced by several stimuli that unbalance the body’s homoeostasis(Reference Şahin and Gümüşlü47). In the present study, stress was induced in animals using an exhaustive exercise protocol, and we evaluated the impact of the association of CCM with WPC on the metabolic control, inflammation and oxidative stress of Wistar rats submitted to this test.
The CCM was added to the WPC so that a concentration of 0·8 g of CCM was obtained for each 100 g of WPC, which was attained through spray drying, one of the most used techniques in the encapsulation of products, since it shows low cost and it is the most viable for industry(Reference Talón, Lampi and Vargas48). The centesimal composition found is within expectations(Reference Silva and Souza49).
Given that the incorporation of phenolic compounds in foods is considered an effective approach to improve the antioxidant capacity and bioactivity of foods(Reference Cao and Xiong50), the concentration of total phenolics and the antioxidant activity of CCM, WPC and WPC + CCM was evaluated. CCM showed higher concentrations of total phenolics and antioxidant capacity when compared to WPC, which was expected, since CCM is a biologically active phenolic compound isolated from turmeric that shows a potent antioxidant effect(Reference Yousefian, Shakour and Hosseinzadeh51).
In order to fight the potential risks of oxidative damage (in this case, caused by exhaustive physical exercise), food consumption of phenolics can be considered as a line of defence against highly reactive toxins(Reference Akter, Hossain and Takara52). It is also scientifically established that WPC shows antioxidant activity (although lower than CCM), since it is related to the chelation of metals by lactoferrin, the elimination of free radicals by sulphur-containing amino acids and an increase on the level of glutathione peroxidase. Additionally, lactoferrin and casein can inhibit lipid peroxidation, the generation of peroxide radicals, the formation of reactive substances to thiobarbituric acid and the uptake of oxygen and iron oxide free radicals(Reference Khan, Nadeem and Imran53).
As for WPC + CCM, it was observed that the addition of CCM in WPC equated the concentrations found in CCM. Improved functional properties of proteins through interaction with phenolic compounds have been widely reported. WP modified with phenolics exhibit improved emulsifying capacity(Reference Wang, Zhang and Lei54), as they are capable of producing stable foams, as well as maintaining the anti-inflammatory activity of the polyphenols trapped in these particles(Reference Schneider, Esposito and Lila55), in addition to protecting other sensitive bioactive compounds(Reference Yi, Zhang and Liang56).
Regarding the animals biometric and food consumption measures, the consumption of WPC + CCM, as well as isolated CCM, was not able to change these parameters, which represents a positive result, since changes in these variables could influence the others, such as the biochemical and histological data.
WP consumption is associated with decreased hunger and food intake compared to casein, soya or albumin(Reference Veldhorst, Nieuwenhuizen and Hochstenbach-Waelen57). In addition, a protein-rich diet can play an important role in regulating energy expenditure or central appetite(Reference Halton and Hu58). However, it is noteworthy that, in the present study, we replaced part of the dietary protein (albumin) by WPC, and we did not perform a supplementation protocol, corroborating the results found.
Plasma concentrations of hepatic metabolism products, as well as liver enzymes, were also determined to check for possible toxicity, as the liver is intensely involved in the metabolism and synthesis of all macronutrients and shows highly relevant endocrine and exocrine functions(Reference Pulido-Moran, Moreno-Fernandez and Ramirez-Tortosa59). The animals that were submitted to the exhaustion test showed higher concentrations of aspartate aminotransferase when compared to the animals that did not perform the exercise, as expected, since the liver activity of aspartate aminotransferase is cytosolic and its highest increase is observed in relation to alanine aminotransferase in liver lesions(Reference Tietz, Burtis and Ashwood60).
Also, WPC + CCM was able to reduce the blood glucose of the animals when compared to the groups AIN-93M and CCM that were submitted to the exhaustion test. It is well established that, during prolonged exercises of moderate to high intensity, carbohydrate is used mainly to support energy metabolism due to its rapid efficiency and availability(Reference Alghannam, Tsintzas and Thompson61).
The higher glucose levels observed in groups in which animals were submitted to the exhaustion test may have been caused due to the release of catabolic hormones, such as cortisol, since physical exercise is a specific stressor known to drastically modify the circulating concentration of cortisol in the human body(Reference Hill, Zack and Battaglini62). On the other hand, the lower glucose levels observed in animals that consumed WPC + CCM are due to the ability of WP to decrease post-prandial glycaemia through several interconnected mechanisms, such as increased secretion of incretin and insulin, delayed gastric emptying, reduced appetite and energy consumption(Reference Mignone, Wu and Horowitz63), increased glycogen synthesis and AMP-activated protein kinase (AMPK) activity(Reference Ichinoseki-Sekine, Kakigi and Miura64), as well as activation from mammalian target of rapamycin (mTOR)(Reference Mitchell, McGregor and D’Souza65). In relation to CCM, it has also received considerable attention for demonstrating its effectiveness in reducing blood glucose since it can also raise the level of insulin in the plasma(Reference Seo, Choi and Jung66).
Exhaustive exercise is also related to the occurrence of oxidative stress, tissue damage, as well as changes in the systemic balance of cytokines, which are cellular signals related to oxidative stress(Reference Kerasioti, Stagos and Jamurtas67). Our results demonstrate that WPC + CCM was able to reduce the expression of inflammatory cytokines, such as TNF-α and IL-6, and to increase the expression of IL-10, considered an important anti-inflammatory cytokine.
The magnitude of the inflammatory response depends on the intensity, duration and chronicity of the exercise(Reference Kerasioti, Stagos and Jamurtas67,Reference Liu, Wu and Zhang68) . To date, only few studies have reported the effects of acute exercise on inflammatory pathways in the liver(Reference Huang, Wu and Yang2,Reference Heinrich, Behrmann and Haan69–Reference Barcelos, Bresciani and Rodriguez-Miguelez71) . However, it is known that there is a great communication between the liver and the skeletal muscle during exercise, modulating the inflammatory response. IL-6 derived from muscle contraction triggers the production of several cytokines and anti-inflammatory signalling pathways and is considered a key mediator of the acute effects of exercise. However, during chronic inflammation, IL-6 activates monocytes and macrophages through TNF-α stimulation, in addition to remaining elevated during and after exercise, reaching various tissues, including the liver(Reference Pillon Barcelos, Freire Royes and Gonzalez-Gallego72).
The consumption of isolated CCM is effective to reduce the gene expression of pro-inflammatory cytokines, since it can negatively regulate the activity of the enzymes inducible NO synthase, cyclooxygenase-2, lipoxygenase and xanthine oxidase, suppressing the activation of NF-κB(Reference Kocaadam and Şanlier73), which is a transcription factor that controls the gene expression of many inflammatory proteins, cell growth and apoptosis, in addition to being activated in a sensitive way to oxidative stress during muscle contraction due to increased production of free radicals(Reference Sahin, Pala and Tuzcu74).
Naturally, NF-κB is maintained in the cytosol. Exposure to inflammatory stimulus results in the release of NF-κB dimers (p50 and p65), which can now be translocated to the nucleus, where transcription of the target gene is induced(Reference Davis, Murphy and Carmichael75). CCM, therefore, can act by blocking the activation of NF-κB(Reference Pan, Lin-Shiau and Lin76).
Also, it has been reported that CCM suppresses cyclooxygenase-2, a key enzyme in the formation of PG, a family of compounds derived from arachidonic acid. Although the mechanisms have not yet been fully elucidated, it is believed to be mediated by their effects on NF-κB and AP-1(Reference Davis, Murphy and Carmichael75), due to the hydroxyl and methoxy groups in their structure(Reference Boarescu, Boarescu and Bocșan77).
As for the anti-inflammatory role of WPC, this is probably due to its content of bioactive peptides(Reference Ma, Liu and Shi78). Studies also demonstrate the anti-inflammatory capacity of lactoferrin in animal models by regulating cytokines (TNF-α, IL-6, IL-10 and pro-Th1)(Reference Kimber, Cumberbatch and Dearman79,Reference Shimizu, Dairiki and Ogawa80) . As for glutamine, the most abundant amino acid in WPC, its protective effects have also been demonstrated through multiple mechanisms, including direct protection of cells and tissues against injuries, attenuation of inflammation and preservation of metabolic function(Reference Weitzel and Wischmeyer81).
Also, since the liver plays an important role in redox status(Reference Pillon Barcelos, Freire Royes and Gonzalez-Gallego72), we evaluated the exercise-induced oxidative stress on oxidation products and antioxidant enzymes. We observed that, among the animals that did or did not perform the exhaustive test, the consumption of CCM was able to reduce the concentrations of MDA, as well as the consumption of WPC + CCM and isolated CCM were also able to reduce the concentrations of PC in relation to the control group. Among the animals that swam, WPC + CCM was able to reduce the NO concentration, when compared to the control and CCM groups.
MDA is one of the final stages of lipid peroxidation products and is an indicator of oxidative stress in cells and tissues, indicating an increase in membrane damage(Reference Ren, Yi and Zhang82). NO, in turn, despite having an unpaired electron and is related to the production of many reactive intermediates, is a short-lived lipophilic molecule generated from l-arginine by NO synthase and is involved with vasodilation, neurotransmission, inhibition of platelet aggregation, immune defense and intracellular signalling(Reference Menon and Sudheer83).
The administration of CCM can significantly decrease MDA due to its ability to reduce lipid peroxidation induced by hydrogen peroxide (H2O2) and inhibit NO synthase activity by reducing NO(Reference Boarescu, Boarescu and Bocșan77).
Exhaustive exercise is related to the increased production of these oxidising compounds, as found in our results, due to the high oxygen consumption during exercise, which can increase up to 200 times(Reference Mazani, Nemati and Baghi84), increasing mitochondrial activity and the body’s inability to balance its energy demand, causing imbalance and consequent metabolic damage. The fact that these compounds have unpaired electrons makes them very reactive to any other type of molecule such as lipids, proteins and nucleic acids(Reference Vale, Ferreira and Benetti85).
Regarding antioxidant enzymes, among the animals that did not perform the test, those that consumed the control diet showed higher concentrations of CAT when compared to those that consumed CCM.
Exhaustive exercise was able to decrease the concentration of CAT and SOD in the animals of the control group, while the opposite was observed with the group that consumed CCM, in relation to the CAT levels.
Also, among the animals that swam, CCM was able to increase the CAT pool more quickly after exhaustive exercise in relation to the other groups, while in relation to the SOD concentrations, both WPC + CCM and isolated CCM were able to increase the concentration of this antioxidant enzyme. CCM is able to indirectly induce the expression of antioxidant proteins, such as SOD, CAT, glutathione peroxidase, glutathione reductase, GST and g-glutamyl cysteine ligase(Reference Samarghandian, Azimi-Nezhad and Farkhondeh86,Reference Hu, Carey and Lindor87) .
The same was not observed for GST, since the control group was the one with the highest levels of this enzyme in relation to the other groups. In addition to the enzymatic mechanism, cells developed several non-enzymatic defenses composed of exogenous antioxidants, components of the diet, to alleviate the deleterious effects caused by oxidative stress(Reference Ren, Yi and Zhang82). Vitamins C and E, carotenoids and phenolics are currently considered the main exogenous antioxidants(Reference Fallahi, Rajaei and Malekzadeh88). Therefore, the failure to observe an increase in GST and CAT in the WPC + CCM and CCM groups, and CCM, respectively, indicates that, due to the ingestion of CCM, the non-enzymatic defense system was sufficient to fight oxidative stress, without the need for the system enzyme being required.
Finally, in order to analyse whether liver tissue functions remained preserved with the ingestion of WPC + CCM or CCM alone, after exhaustive swimming, the areas referring to the fat deposit and infiltrate inflammatory were evaluated by histological analysis. Surprisingly, we observed that the groups that received the AIN93M diet have developed fatty liver. The high carbohydrates content presented in this diet could be responsible for the increased TAG from glucose metabolisation(Reference Ferramosca, Conte and Damiano89). This diet contains in its formulation 70 % of carbohydrates and it is known that an increased fats and carbohydrates’ intake in the diet may cause non-alcoholic fatty liver disease. Additionally, it is already known that other mechanisms are involved in lipidic metabolism, such as the production of fatty acids from acetyl-CoA, reduction in lipoprotein formation, inflammatory processes and free radicals responsible for lipid chain peroxidation(Reference Baptista, Sarandy and Gonçalves90). Our results showed increased lipids inside the hepatocytes in the CCM group; however, all the oxidative stress markers (MDA, PCN) remained low. Also, inflammatory markers involved in the lipid deposit (TNF and IL-6) remained low in the CCM groups. These issues showed that although there was an increased lipid deposit in the CCM group, it was not related to an oxidative imbalance or the development of an inflammatory process, since CCM inhibited the release and production of important markers related to these processes. The hepatoprotective effect of CCM was not verified in our study, most likely due to the low dose tested (3 mg/kg per d) and the short experiment duration (4 weeks). Therefore, future studies are necessary for a better understanding of the molecular, cellular and immunological mechanisms activated during CCM exposure.
As for the inflammatory infiltrate, among the animals that swam, those that consumed WPC + CMM showed smaller areas of inflammation when compared to the control group, corroborating the results found through the analysis of inflammatory cytokine gene expression, demonstrating that WPC + CCM is able to reduce the inflammation caused by exhaustive exercise.
Finally, it is important to emphasise that inflammation can be considered a type of hormetic stress, that is, it exerts positive results at low levels (physiological inflammation), while the opposite effect is observed in individuals in the post-reproductive period, especially in those who are unable to maintain an ideal balance between pro and anti-inflammatory mediators. This systemic inflammatory state sustained through exposure to chronic stressors, with a consequent increase in reactive oxygen species, represents a particular ageing phenotype or ‘inflammaging’(Reference Calabrese, Santoro and Monti91,Reference Brunetti, Di Rosa and Scuto92) . Increased oxidative stress also leads brain cells to develop response networks that detect and control various forms of stress, such as the Vitagenes network, which actively act in promoting cell survival in pathophysiological conditions(Reference Miquel, Champ and Day93,Reference Siracusa, Scuto and Fusco94) .
Recently, inflammaging has been recognised as one of the seven pillars of ageing, in addition to being related to the incidence of many age-related diseases, including neurodegenerative diseases. Thus, approaches aimed at reducing inflammation, such as polyphenol intake, can be effective in delaying the onset of a range of age-related illnesses. These approaches can be used concurrently with a hormetic strategy, which in this case involves repeated exposure to low levels of specific stressors through physical exercise (pre-conditioning and post-conditioning), in order to induce the activity of the physiological mechanisms of maintenance and repair, neutralising the effects of neuroinflammatory stimuli and contributing to adaptive resistance to stress and neuroinflammation, in addition to being able to increase neuroplasticity, preventing neurodegenerative changes in the brain that are typical of ageing(Reference Calabrese, Santoro and Monti91,Reference Brunetti, Di Rosa and Scuto92,Reference Calabrese, Cornelius and Dinkova-Kostova95) .
Conclusion
CCM showed high concentrations of total phenolics and antioxidant capacity, improving WPC nutritional characteristics due to its antioxidant and nutritional potential.
The consumption of WPC + CCM, as well as isolated CCM, did not alter the biometric measurements, the animals’ food consumption and the hepatic and kidney function, as well as the protein balance of the animals, which makes it a safe, viable and efficient product.
Also, WPC + CCM reduced blood glucose in relation to AIN-93M and CCM groups that were submitted to the exhaustion test and the gene expression of inflammatory cytokines such as TNF-α and IL-6, in addition to increasing the expression of IL-10 compared to the other groups. It also reduced the concentration of NO when compared to the control and CCM groups, and PC in relation to the control group. The product also increased the concentrations of SOD in relation to the control group.
Despite the fact that all the groups showed grade I hepatic steatosis, possibly due to the consumed diet, the animals of the WPC + CCM ET group showed smaller areas of hepatic inflammatory infiltrate in relation to the group AIN-93M ET, as demonstrated in the histomorphometric analysis.
Therefore, WPC admixtured of CCM can be considered as an alternative product to WP consumers, as it represents a strategy capable of decreasing blood glucose and liver oxidative and inflammatory damage caused by exhaustive physical exercise.
Further studies are needed to assess the beneficial effects of the association of CCM with WPC, through analyses in other organs, such as skeletal muscle, as well as to develop studies in humans, in order to try to elucidate the molecular pathways and mechanisms responsible for the results found.
Acknowledgements
The authors are grateful to Theracurmin®; Theravalues, Tokyo, Japan for the donation of curcumin, to Conselho Nacional de Desenvolvimento Científico e Tecnológico (CNPq) and to Coordenação de Aperfeiçoamento de Pessoal de Nível Superior – Brasil (CAPES) – Finance Code 001, for the financial support.
This work was supported by the MCTIC/CNPq nº 28/2018 process: 428701/2018-3 and by the Coordenação de Aperfeiçoamento de Pessoal de Nível Superior – Brasil (CAPES) – Finance Code 001.
A. R. C. and K. A. D.: conception, conceptualisation, resources, investigation, writing, data curation, formal analysis. S. M. S. P., L. C. S. and L. A. O.: investigation, laboratory analysis. E. C. G. S., R. V. G., S. L. P. M., A. J. N., H. S. D. M.: critical review of intellectual content, revising, supervision, resources, validation. C. M. D. L.: conception, resources, critical review, review and editing, writing, supervision, project administration, validation. All authors approved the final version of the manuscript.
The authors have no conflict of interest to declare.