Introduction
Phyto-oestrogens are dietary compounds of plant origin that mainly include flavonoids and lignans. Since their chemical structure is similar to those of oestrogens, they have been studied for their involvement in hormone-related disorders, such as reproductive failure and breast cancer (Setchell & Adlercreutz, Reference Setchell, Adlercreutz and Rowland1988). Meanwhile, it has become clear that it is crucial to study the bioavailability of phyto-oestrogens to evaluate the relevance of their health effects. The trend to consume increasing amounts of phyto-oestrogen-containing foods in Western countries shows the importance of studying the fate of phyto-oestrogens in the human body. Because abundant data are already available on flavonoids, the present review focuses on lignans.
A prerequisite for investigating lignan bioavailability is to accurately determine their occurrence in foods and to estimate their intake in human populations. Although flaxseed is the main source of lignans (approximately 4 mg/g dried mass), a variety of cereals, fruits, vegetables, legumes and beverages also contain lignans in substantial concentrations (10 ng to 400 μg/g) (Milder et al. Reference Milder, Arts, van de Putte, Venema and Hollman2005a). Thus, lignans are found in a wide range of foods consumed daily in Western countries. Secoisolariciresinol diglucoside (SDG), its aglycone secoisolariciresinol (SECO), and matairesinol (MAT) are the most frequently studied dietary lignans. They have been studied for their possible role in the prevention of breast and prostate cancer (McCann et al. Reference McCann, Gill, McGlynn and Rowland2005; Thompson et al. Reference Thompson, Chen, Li, Strasser-Weippl and Goss2005) and atherosclerosis (Prasad, Reference Prasad2005). They have also been used as model substrates to assess the bacterial production of the enterolignans enterodiol (ED) and enterolactone (EL) (Borriello et al. Reference Borriello, Setchell, Axelson and Lawson1985), the biological properties of which are proposed to be more potent than those of plant lignans (Brooks & Thompson, Reference Brooks and Thompson2005; Jacobs et al. Reference Jacobs, Nolan and Hood2005). Numerous data presented in the present review deal primarily with SDG. However, it must be emphasised that enterolignans are produced from plant lignans other than SDG and MAT (Axelson et al. Reference Axelson, Sjovall, Gustafsson and Setchell1982), such as arctigenin, arctiin, 7-hydroxymatairesinol, isolariciresinol, lariciresinol (LARI), pinoresinol (PINO), sesamin and syringaresinol (Thompson et al. Reference Thompson, Robb, Serraino and Cheung1991; Liggins et al. Reference Liggins, Grimwood and Bingham2000; Heinonen et al. Reference Heinonen, Nurmi, Liukkonen, Poutanen, Wahala, Deyama, Nishibe and Adlercreutz2001; Xie et al. Reference Xie, Ahn, Akao, Abdel-Hafez, Nakamura and Hattori2003a,Reference Xie, Akao, Hamasaki, Deyama and Hattorib; Penalvo et al. Reference Penalvo, Heinonen, Aura and Adlercreutz2005). Enterolignans are also produced from lignins in rats (Begum et al. Reference Begum, Nicolle, Mila, Lapierre, Nagano, Fukushima, Heinonen, Adlercreutz, Remesy and Scalbert2004). Recent studies agree on the need to expand databases on the lignan content of foods in order to more accurately determine dietary intakes of enterolignan precursors. Reference Milder, Feskens, Arts, Bueno de Mesquita, Hollman and KromhoutMilder et al. (2005b) estimated that the total mean intake of LARI, MAT, PINO and SECO is approximately 1 mg/d in Dutch adults. Although the authors did not systematically take into account the influence of food processing on lignan concentrations and that lignan intake may vary between individuals and study populations, an appealing goal remains to know what proportion of this daily ingested mg of plant lignans may be metabolised in the digestive tract, be absorbed and eventually reach target tissues.
The aim of the present article is to provide a comprehensive and critical overview of the current knowledge on lignan bioavailability in human subjects, including data on the production, origin and physiological concentrations of lignan metabolites, with emphasis on the influence of intestinal bacteria on lignan bioavailability.
Lignan metabolism in the upper part of the gastrointestinal tract
Host mechanisms, such as saliva action and chewing in the mouth, chemical hydrolysis in the stomach and mucosal enzymic activities, probably influence lignan bioavailability in the upper part of the gastrointestinal tract. However, the relevance of these processes is essentially unknown.
Results obtained in our laboratory showed that SDG was stable after 3 h at 37°C in artificial stomach juice (Clavel et al. Reference Clavel, Borrmann, Braune, Doré and Blaut2006a). Similar results were reported for other glycosylated lignans in rat gastric juice (Nose et al. Reference Nose, Fujimoto, Takeda, Nishibe and Ogihara1992). This agrees with data obtained by Mazur (Reference Mazur2000), who found that glycosidic bonds of SDG are difficult to hydrolyse. For lignan extraction from foods, the authors used harsh conditions (2 m-HCl, 100°C, 2·5 h) to obtain SECO. SDG was also stable after 3 h at 37°C in artificial intestinal juice (Clavel et al. Reference Clavel, Borrmann, Braune, Doré and Blaut2006a). Thus, it is possible that SDG is not hydrolysed during its passage through the stomach and small intestine.
While deglycosylation of flavonoids by host enzymes occurs in the mouth and small intestine (Walle et al. Reference Walle, Browning, Steed, Reed and Walle2005), studies have not yet been conducted with lignans. Germ-free animal or in situ rat intestinal perfusion (Arts et al. Reference Arts, Sesink, Faassen-Peters and Hollman2004) models could be used to gain basic knowledge on the role of host mechanisms in lignan conversion, such as epithelial deglycosylation and absorption of plant lignans. Penalvo et al. (Reference Penalvo, Nurmi, Haajanen, Al-Maharik, Botting and Adlercreutz2004) recovered approximately 2 % of the ingested dose of PINO and LARI in the plasma of four individuals 1 h after intake of 50 g sesame seeds. This suggests that a certain amount of ingested plant lignans may be quickly absorbed. This is in agreement with the detection of plant lignans in urine (Bannwart et al. Reference Bannwart, Adlercreutz, Wahala, Brunow and Hase1989; Lampe et al. Reference Lampe, Gustafson, Hutchins, Martini, Li, Wahala, Grandits, Potter and Slavin1999; Hutchins et al. Reference Hutchins, Martini, Olson, Thomas and Slavin2000; Nurmi et al. Reference Nurmi, Voutilainen, Nyyssonen, Adlercreutz and Salonen2003). However, mechanisms responsible for uptake of plant lignans in the upper part of the gastrointestinal tract are unknown.
Due to rapid swallowing, it is unlikely that oral bacteria contribute to the conversion of plant lignans. The same applies to the oesophagus and stomach, where physical and chemical conditions do not favour bacterial colonisation. In contrast, at cell densities of approximately 107 viable cells/g dried content in the ileum and 1012 cells/g in the colon, intestinal bacteria are considered to be a major factor that influences lignan bioavailability.
Production of enterolignans by intestinal bacteria
In vitro conversion of plant lignans by faecal slurries (Borriello et al. Reference Borriello, Setchell, Axelson and Lawson1985; Thompson et al. Reference Thompson, Robb, Serraino and Cheung1991) and studies with germ-free rats (Axelson & Setchell, Reference Axelson and Setchell1981) have shown that intestinal bacteria are crucial for enterolignan production. To convert SDG to EL, bacteria catalyse four sequential reactions: O-deglycosylation, O-demethylation, dehydrogenation and dehydroxylation (Fig. 1) (Wang et al. Reference Wang, Meselhy, Li, Qin and Hattori2000). Enterolignan production from LARI and PINO includes one and two additional reduction steps, respectively (Xie et al. Reference Xie, Akao, Hamasaki, Deyama and Hattori2003b).
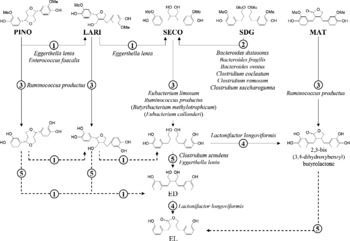
Fig. 1 Conversion of the plant lignans pinoresinol (PINO), lariciresinol (LARI), secoisolariciresinol (SECO), secoisolariciresinol diglucoside (SDG) and matairesinol (MAT) by human intestinal bacteria. Bacterial names are identified next to the reactions catalysed by the given organisms. Reactions are: (1) reduction; (2) O-deglycosylation; (3) O-demethylation; (4) dehydrogenation; (5) dehydroxylation. The SECO-demethylating species Butyribacterium methylotrophicum and Eubacterium callanderi are not yet known as members of the human intestinal microbiota. (---), Reactions for which no bacteria have been identified so far; ED, enterodiol; EL, enterolactone.
Diversity of lignan-converting bacteria and prevalence of enterolignan production
The production of enterolignans from SDG requires the interaction of phylogenetically and functionally distantly related anaerobic bacteria (Fig. 1). The ability to O-deglycosylate SDG has been demonstrated for closely related species of the genera Bacteroides and Clostridium (Clavel et al. Reference Clavel, Henderson, Engst, Doré and Blaut2006b,c). The O-demethylation and dehydroxylation steps underlying SECO conversion are catalysed by several strains of Ruminococcus productus and Eggerthella lenta, respectively (Clavel et al. Reference Clavel, Henderson, Engst, Doré and Blaut2006b). Thus, the presence of different SDG-converting bacteria in the intestine may explain the high prevalence of enterolignan production in human subjects (Clavel et al. Reference Clavel, Henderson, Alpert, Philippe, Rigottier-Gois, Dore and Blaut2005). In our laboratory, enterolignans (ED + EL) were produced by all thirty-one human faecal samples tested. One sample from a male adult did not produce EL. Although these data were obtained with SECO only, bacteria involved in enterolignan production from SDG also convert other plant lignans. R. productus not only catalyses the O-demethylation of SECO, but also the O-demethylation of LARI, MAT, PINO and of a variety of other methylated aromatic compounds (Clavel et al. Reference Clavel, Borrmann, Braune, Doré and Blaut2006a). E. lenta catalyses both the dehydroxylation of di-demethylated SECO and the reduction of PINO to LARI and of LARI to SECO. These data indicate that enterolignan production from various plant lignans results from a network of reactions (Fig. 1). It should be kept in mind that intestinal bacteria other than the ones identified so far may contribute to the conversion of plant lignans.
Occurrence of lignan-converting bacteria in relation to host factors
Most species mentioned in Fig. 1 are common members of dominant bacterial groups in the human intestine (Finegold et al. Reference Finegold, Sutter, Mathisen and Henteges1983; Suau et al. Reference Suau, Bonnet, Sutren, Godon, Gibson, Collins and Dore1999). Culture-based enumerations of SECO-converting bacteria showed that organisms involved in the production of ED occurred at a mean cell density of 6 × 108 cells/g faeces (Clavel et al. Reference Clavel, Henderson, Alpert, Philippe, Rigottier-Gois, Dore and Blaut2005). In contrast, organisms involved in the production of EL from SECO were detected at a mean cell density of 3 × 105 cells/g. Since the occurrence of the latter organisms was related to the amount of EL produced, it is conceivable that variations in the cell density of lignan-converting bacteria explain the marked inter-individual differences observed for enterolignan production (Mazur et al. Reference Mazur, Uehara, Wahala and Adlercreutz2000; Clavel et al. Reference Clavel, Henderson, Alpert, Philippe, Rigottier-Gois, Dore and Blaut2005; Kuijsten et al. Reference Kuijsten, Arts, Vree and Hollman2005b). However, this needs to be confirmed by future human intervention studies, in which proportions of lignan-converting bacteria could be compared with concentrations of ED and EL in biological matrices.
Interestingly, women tend to harbour more enterolignan-producing bacteria than men (Clavel et al. Reference Clavel, Henderson, Alpert, Philippe, Rigottier-Gois, Dore and Blaut2005). However, this was not associated with variations in proportions of dominant bacterial groups, possibly due to a limited number of samples. Nevertheless, this finding implies that host factors influence the bacterial production of enterolignans. In this context, it is worthwhile to discuss the role of the SECO-dehydroxylating species Clostridium scindens and E. lenta in the dehydroxylation of endogenous compounds that undergo enterohepatic circulation. E. lenta has been studied for its involvement in 21-dehydroxylation of biliary steroids (Feighner & Hylemon, Reference Feighner and Hylemon1980), C. scindens for its ability to 7α-dehydroxylate primary bile acids (Doerner et al. Reference Doerner, Takamine, LaVoie, Mallonee and Hylemon1997) and to synthesise desmolase and 20α-hydroxysteroid dehydrogenase (Krafft et al. Reference Krafft, Winter, Bokkenheuser and Hylemon1987), both of which are involved in the metabolism of steroid hormones. It is conceivable that sex differences in intestinal levels of steroid hormones, for example, oestrogens, progesterone and testosterone, influence metabolic activities of bacteria capable of converting structurally related dietary compounds. This applies to the ability of C. scindens and E. lenta to dehydroxylate SECO. The role of progesterone might be of particular interest. Bacterial dehydroxylases are involved in progesterone metabolism (Feighner & Hylemon, Reference Feighner and Hylemon1980). Also, progesterone relaxes smooth muscle tone resulting in a longer intestinal transit time (Bielefeldt et al. Reference Bielefeldt, Waite, Abboud and Conklin1996). Shoda et al. (Reference Shoda, He, Tanaka, Matsuzaki, Osuga, Yamamori, Miyazaki and Sjovall1995) and Kilkkinen et al. (Reference Kilkkinen, Stumpf, Pietinen, Valsta, Tapanainen and Adlercreutz2001) reported a positive correlation between intestinal transit and bacterial production of secondary bile acids and between serum EL concentration and constipation, respectively. Some bacteria, including C. scindens and strains of Eubacterium species, have several bile acid-inducible (bai) genes, which encode enzymes of the bile acid 7α-dehydroxylation pathway (Doerner et al. Reference Doerner, Takamine, LaVoie, Mallonee and Hylemon1997). Thus, progesterone levels and transit time may influence the conversion of dietary lignans by increasing their availability to bacteria or by inducing bacterial activities, directly or indirectly. However, to draw firm conclusions, it is imperative to characterise enzymes involved in lignan conversion. For example, enzyme expression could be assessed by proteomic analysis in response to the presence or absence of substrate. Another approach would be to screen metagenomic libraries of human intestinal microbiota for bacterial clones catalysing reactions underlying lignan conversion.
Influence of bacterial dehydrogenation on metabolite production
Of the five reactions underlying bacterial production of EL, the dehydrogenation step is of particular interest. First of all, lignan-dehydrogenating bacteria are subdominant members of intestinal microbiota (mean cell density of about 105 cells/g). Only one strain capable of dehydrogenating lignans was identified, namely Lactonifactor longoviformis DSM 17 459T (Clavel et al. 2006c). More work is needed to quantify this organism in faeces but, considering that EL production is detected in most individuals (Clavel et al. Reference Clavel, Henderson, Alpert, Philippe, Rigottier-Gois, Dore and Blaut2005; Kuijsten et al. Reference Kuijsten, Arts, Vree and Hollman2005b), either the prevalence of L. longoviformis is high in human subjects or other not yet identified organisms catalyse the dehydrogenation step underlying EL production.
L. longoviformis seems to catalyse only the enantio-specific conversion of (+)-ED to (+)-EL (Clavel et al. 2006c). Xie et al. (Reference Xie, Ahn, Akao, Abdel-Hafez, Nakamura and Hattori2003a) reported the ability of faecal bacteria to produce (+)-EL and ( − )-EL from different precursors and proposed that the absolute configuration of lignans is preserved throughout bacterial conversion. This agrees with data obtained in rats by Saarinen et al. (Reference Saarinen, Smeds, Makela, Ammala, Hakala, Pihlava, Ryhanen, Sjoholm and Santti2002). We hypothesise that so-far unidentified bacteria dehydrogenate the ( − )-enantiomer of lignans. Since the absolute configuration may influence production rates and biological properties of enterolignans, stereochemistry should be taken into consideration in future studies on bacterial production and biological properties of lignan metabolites. Concerning plant lignans, the (+)-enantiomer of SDG was detected at proportions of more than 90 % total SDG in two flaxseed species (Sicilia et al. Reference Sicilia, Niemeyer, Honig and Metzler2003). However, this ratio may vary in other flaxseed species and in dietary sources other than flaxseed. In a recent study (Knust et al. Reference Knust, Hull, Spiegelhalder, Bartsch, Strowitzki and Owen2006), ( − )-SDG was detected as the major enterolignan precursor in flaxseed, but the authors did not specify the flaxseed species tested. Xia et al. (Reference Xia, Costa, Pelissier, Davin and Lewis2001) purified an enantio-specific enzyme from plants of Forsythia intermedia and Podophyllum peltatum that dehydrogenates ( − )-SECO to ( − )-MAT. Concerning LARI and MAT, one enantiomer of each was found in large excess in flaxseed (Sicilia et al. Reference Sicilia, Niemeyer, Honig and Metzler2003), but stereochemistry was not determined. We conclude that the stereochemistry of plant lignans needs to be further investigated in order to accurately estimate physiological levels of active lignan metabolites.
The study of lignan conversion by L. longoviformis led to the identification of 2,3-bis(3,4-dihydroxybenzyl)butyro-lactone, a new intermediate in the formation of EL from SDG. This shows that enterolignan production from a single precursor may occur through different pathways (Fig. 1). However, it is difficult to make assumptions on the predominance of any of these pathways in vivo, and how this influences conversion rates and biological effects of lignans. Adlercreutz et al. (Reference Adlercreutz, Bannwart, Wahala, Makela, Brunow, Hase, Arosemena, Kellis and Vickery1993a) found that the ability of 2,3-bis(3,4-dihydroxybenzyl)butyro-lactone (referred to as 4,4′-dihydroxy-EL in the study) to inhibit aromatase activity was higher than that of EL. Which lignan metabolites are formed by bacteria in vivo and how they differ between individuals remains a key issue. These metabolites may not necessarily be ED and EL. The presence of certain bacterial groups in faeces might be a good indicator for certain patterns of lignan metabolites and their rate of formation. In the future, in vitro continuous-culture systems could be used to grow mixed cultures of lignan-converting strains under controlled conditions in order to assess how changes in community composition influence the production of lignan metabolites.
Limitations of bacteriological studies
Even if bacteriological data are crucial for the study of lignan bioavailability, two major limitations must be pointed out. First, to characterise the mechanisms underlying bacterial conversion of lignans, it is necessary to work not only with pure compounds, but also even with pure enantiomers. However, this makes it difficult to estimate rates of enterolignan production in vivo, since the fate of pure compounds does not necessarily reflect lignan availability from complex food matrices. For example, lignans are primarily found in the outermost layers of seeds and may not be easily accessible to bacteria (Mazur, Reference Mazur2000). Crushing and milling of flaxseed was shown to improve enterolignan bioavailability (Kuijsten et al. Reference Kuijsten, Arts, Van't Veer and Hollman2005a). Furthermore, ester-linked and 3-hydroxy-3-methyl-glutaric-acid-interconnected polymers of SDG have been described in flaxseed (Ford et al. Reference Ford, Huang, Wang, Davin and Lewis2001; Kamal-Eldin et al. Reference Kamal-Eldin, Peerlkamp, Johnsson, Andersson, Andersson, Lundgren and Aman2001). Although mucosal and bacterial esterase activities towards phenolic acids have been reported in human subjects (Andreasen et al. Reference Andreasen, Kroon, Williamson and Garcia-Conesa2001) and although enterolignans are produced from lignins in rats (Begum et al. Reference Begum, Nicolle, Mila, Lapierre, Nagano, Fukushima, Heinonen, Adlercreutz, Remesy and Scalbert2004), it is unclear to what extent lignan polymers are hydrolysed in the human intestine. Hence, to assess lignan conversion rates, it would be preferable to use complex food matrices, as done previously by Thompson et al. (Reference Thompson, Robb, Serraino and Cheung1991) and Aura et al. (Reference Aura, Oikarinen, Mutanen, Heinonen, Adlercreutz, Virtanen and Poutanen2006) with faecal slurries. Second, defined mixed cultures catalyse the conversion of SDG to EL in vitro (Clavel et al. Reference Clavel, Henderson, Engst, Doré and Blaut2006b). However, it is difficult to draw any conclusion on the role that each of the identified lignan-converting species plays in vivo. Experiments with gnotobiotic animals could bring to the test the relevance of these in vitro findings. Besides, batch cultures of faecal slurries do not accurately mimic the physico-chemical conditions in the intestine. Depending on the media and incubating conditions used, growth of specific bacterial groups may be favoured. This would lead either to overgrowth of lignan-converting bacteria with ensuing overestimation of corresponding cell densities, or to overgrowth of non-converting bacteria. This may also explain the 60 % recovery of enterolignans after incubating SECO with faecal slurries for 48 h (Clavel et al. Reference Clavel, Henderson, Alpert, Philippe, Rigottier-Gois, Dore and Blaut2005). Heinonen et al. (Reference Heinonen, Nurmi, Liukkonen, Poutanen, Wahala, Deyama, Nishibe and Adlercreutz2001) reported a comparable recovery (72 % of the SECO was converted to enterolignans within 24 h) and noted that the efficacy of conversion varied between plant lignans.
Enterolignan absorption and blood levels
Enterolignan absorption
Once produced by intestinal bacteria, enterolignans may be efficiently absorbed, conjugated and the resulting metabolites excreted by enterocytes, as proposed by in vitro experiments with human colonic cell cultures (Jansen et al. Reference Jansen, Arts, Nielen, Muller, Hollman and Keijer2005). EL-sulfate, -glucuronide and ED-glucuronide were detected after exposing cells to enterolignans, but molar proportions were not determined. Conjugation and excretion occurred within 8 h and EL was metabolised or excreted more rapidly than ED. However, these results must be regarded with caution concerning the kinetics of metabolite production, since cancer cell lines might have an increased conjugation and efflux activity. Besides, only two of the three cell lines tested (HT29 and CaCo-2 cells) were responsive to enterolignan exposure.
Baseline concentrations of lignans in blood
In most studies on blood levels of lignans, total lignans are measured following hydrolysis of conjugates. However, conjugation certainly influences the biological properties of lignans, even if conjugation patterns in blood do not necessarily reflect those in target tissues. Based on the analysis of blood samples from twenty-seven women, Adlercreutz et al. (Reference Adlercreutz, Fotsis, Lampe, Wahala, Makela, Brunow and Hase1993b) proposed that the biologically active fraction of enterolignans, including free, mono- and di-sulfated ED and EL, makes up 21–25 % of total (conjugated plus unconjugated) enterolignans. The major fraction (approximately 80 % total enterolignans) included biologically inactive mono- and di-glucuronides and sulfo-glucuronides. These ratios and blood concentrations of enterolignans probably depend on study cohorts. Nonetheless, it is proposed that average baseline concentrations of enterolignans (in the blood of subjects on their usual diet) are in the range of 10 to 25 nmol/l (Adlercreutz et al. Reference Adlercreutz, Wang, Lapcik, Hampl, Wahala, Makela, Lusa, Talme and Mikola1998; Hong et al. Reference Hong, Kim, Kwon, Lee and Chung2002; Horner et al. Reference Horner, Kristal, Prunty, Skor, Potter and Lampe2002; Grace et al. Reference Grace, Taylor, Botting, Fryatt, Oldfield, Al-Maharik and Bingham2003; Kilkkinen et al. Reference Kilkkinen, Valsta, Virtamo, Stumpf, Adlercreutz and Pietinen2003; Valentin-Blasini et al. Reference Valentin-Blasini, Blount, Caudill and Needham2003; Kuijsten et al. Reference Kuijsten, Arts, Van't Veer and Hollman2005a; Low et al. Reference Low, Taylor and Grace2005). Recently, the plant lignans LARI and MAT were detected in human serum at concentrations sometimes higher than those of ED and EL (Smeds et al. Reference Smeds, Hakala, Hurmerinta, Kortela, Saarinen and Makela2006). For instance, the highest concentration of LARI was 190 nmol/l in the serum of one female subject. On the other hand, SECO was not detected in any of the ten samples tested. Clearly, marked inter-individual differences are observed. In particular, dietary habits influence blood concentrations of lignans. Intake of vegetables, fibres and wholegrain products has been associated with higher EL concentrations (Kilkkinen et al. Reference Kilkkinen, Stumpf, Pietinen, Valsta, Tapanainen and Adlercreutz2001; Horner et al. Reference Horner, Kristal, Prunty, Skor, Potter and Lampe2002). The highest concentration of EL reported in the literature exceeded 1 μmol/l in the blood of one vegan postmenopausal woman (Adlercreutz et al. Reference Adlercreutz, Fotsis, Lampe, Wahala, Makela, Brunow and Hase1993b). Hence, it has been proposed that blood concentrations of EL are related to the intake of plant lignans (Kilkkinen et al. Reference Kilkkinen, Valsta, Virtamo, Stumpf, Adlercreutz and Pietinen2003).
Blood levels of lignans after dietary intervention
A growing number of human studies have shown that dietary intervention with lignan-containing foods leads to an increase in blood levels of enterolignans in nearly all individuals (Nesbitt et al. Reference Nesbitt, Lam and Thompson1999; Juntunen et al. Reference Juntunen, Mazur, Liukkonen, Uehara, Poutanen, Adlercreutz and Mykkanen2000; Mazur et al. Reference Mazur, Uehara, Wahala and Adlercreutz2000; Stumpf et al. Reference Stumpf, Pietinen, Puska and Adlercreutz2000; Jacobs et al. Reference Jacobs, Pereira, Stumpf, Pins and Adlercreutz2002; Tarpila et al. Reference Tarpila, Aro, Salminen, Tarpila, Kleemola, Akkila and Adlercreutz2002; Kuijsten et al. Reference Kuijsten, Arts, Van't Veer and Hollman2005a). Since most of these studies used amounts of whole grains, oilseeds or fruits that are relevant in terms of daily food consumption, moderate changes in dietary habits may significantly alter blood levels of enterolignans. For instance, Mazur et al. (Reference Mazur, Uehara, Wahala and Adlercreutz2000) and Kuijsten et al. (Reference Kuijsten, Arts, Van't Veer and Hollman2005a) showed that blood levels of EL significantly increased after a single meal of 500 g strawberries or after daily consumption of approximately 20 g flaxseed for 10 d. Because the type and duration of intervention vary greatly between studies, it is hard to tell whether enterolignan levels in biological matrices reach maximum values beyond a certain ingested dose of dietary precursors or after intake over long periods. During a 12-week intervention favouring intake of lignan-containing foods (Stumpf et al. Reference Stumpf, Pietinen, Puska and Adlercreutz2000), most increase in the blood concentration of EL occurred during the first 6 weeks (median values were 12·2, 17·2 and 19·5 nmol/l at baseline and after 6 and 12 weeks, respectively; n 85). Conversely, Tarpila et al. (Reference Tarpila, Aro, Salminen, Tarpila, Kleemola, Akkila and Adlercreutz2002) observed a continuous increase during 4 months of intervention with flaxseed (serum EL concentrations were 33, 52 and 70 nmol/l at baseline and after 2 and 4 months, respectively; n 80). However, the participants of the latter study also ingested inulin, which possibly altered microbial activities. Juntunen et al. (Reference Juntunen, Mazur, Liukkonen, Uehara, Poutanen, Adlercreutz and Mykkanen2000) proposed that a daily intake of more than 90 g rye bread (approximately 300 μg lignans) (Milder et al. Reference Milder, Arts, van de Putte, Venema and Hollman2005a) for 4 weeks does not trigger a further increase in blood levels of enterolignans. On the other hand, Nesbitt et al. (Reference Nesbitt, Lam and Thompson1999) observed a dose–response in daily urinary excretion of lignans after intake of 5, 15 or 25 g flaxseed/d for 7 d and did not report a plateau effect at 25 g/d (approximately 75 mg lignans) (Milder et al. Reference Milder, Arts, van de Putte, Venema and Hollman2005a). Thus, we hypothesise that a constant increase in the amount of enterolignans produced in the intestine occurs if dietary intervention is long enough, maybe due to adaptive responses in bacterial activities, which are not necessarily accompanied by changes in bacterial diversity or proportions. However, blood levels of enterolignans may not exceed an individual-specific threshold, due to adaptive responses in host metabolism and excretion mechanisms. The detection of LARI and MAT in blood implies that some plant lignans are rapidly absorbed as such and that the microbial capacities to produce enterolignans can be saturated beyond intake of a certain amount of precursors. However, it is to date not possible to estimate this amount. Future intervention studies in human subjects should assess changes in blood concentration of lignans in relation to changes in intestinal microbiota and should include measurements when dietary treatment is over to determine how durable changes are.
Blood levels in relation to bacterial conversion of lignans
Based on results obtained in our laboratory (Clavel et al. Reference Clavel, Henderson, Alpert, Philippe, Rigottier-Gois, Dore and Blaut2005) and on a recent pharmacokinetic study by Kuijsten et al. (Reference Kuijsten, Arts, Vree and Hollman2005b) who measured enterolignans in blood and urine after a single dose of 500 mg SDG, we can draw a number of conclusions which are relevant to both bacteriological and human studies:
(1) Inter-individual differences in cell densities of enterolignan-producing bacteria may explain inter-individual differences in blood concentrations of enterolignans.
(2) The detection of enterolignans in the blood of most individuals is most probably linked to the high prevalence of enterolignan-producing bacteria and implies that health effects associated with enterolignans are relevant to all individuals.
(3) The predominance of EL v. ED in blood may be partly due to the enterohepatic circulation of ED. Since the ED:EL ratio might be important with regard to health effects, it would be interesting to know whether individuals with low cell densities of lignan-dehydrogenating organisms are those in whom ED is the main metabolite detected after ingestion of SDG.
(4) Kuijsten et al. (Reference Kuijsten, Arts, Vree and Hollman2005b) found that enterolignans are first detectable in blood 8 to 10 h after dietary intake. Although pharmacokinetic parameters may vary between intake of a single dose of SDG in water and continuous intake of SDG-containing foods, this finding agrees with previous data (Nesbitt et al. Reference Nesbitt, Lam and Thompson1999; Mazur et al. Reference Mazur, Uehara, Wahala and Adlercreutz2000) and confirms that enterolignans are primarily produced and absorbed in the colon.
(5) The tendency of women to have higher cell densities of enterolignan-producing bacteria than men (Clavel et al. Reference Clavel, Henderson, Alpert, Philippe, Rigottier-Gois, Dore and Blaut2005) agrees with higher blood concentrations of enterolignans in women (Jacobs et al. Reference Jacobs, Pereira, Stumpf, Pins and Adlercreutz2002; Kilkkinen et al. Reference Kilkkinen, Valsta, Virtamo, Stumpf, Adlercreutz and Pietinen2003; Kuijsten et al. Reference Kuijsten, Arts, Vree and Hollman2005b). Another sex difference is the earlier appearance of enterolignans in the blood of women (Kuijsten et al. Reference Kuijsten, Arts, Vree and Hollman2005b). However, the cohort size was small in three of the cited studies (n ≤ 20) (Jacobs et al. Reference Jacobs, Pereira, Stumpf, Pins and Adlercreutz2002; Clavel et al. Reference Clavel, Henderson, Alpert, Philippe, Rigottier-Gois, Dore and Blaut2005; Kuijsten et al. Reference Kuijsten, Arts, Vree and Hollman2005b). Moreover, it is necessary to take into account sex differences in dietary intake and blood volume when comparing blood levels of enterolignans between women and men. In one study involving twenty-one women and eighteen men with slightly elevated serum cholesterol levels, Juntunen et al. (Reference Juntunen, Mazur, Liukkonen, Uehara, Poutanen, Adlercreutz and Mykkanen2000) refuted the assumption of significant sex differences in lignan metabolism after rye-bread intake. After a 4-week intervention, women had a non-significant higher mean concentration of EL in serum than men (39·3 v. 28·1 nmol/l) and lignan intake on a per kg body-weight basis did not differ. When assessing sex differences, detailed information on the menstrual cycle should be given too. Early studies showed that enterolignan excretion is associated with pregnancy and the menstrual cycle, although the number of samples analysed was limited (Setchell et al. 1979; Stitch et al. Reference Stitch, Toumba, Groen, Funke, Leemhuis, Vink and Woods1980). The possible role of progesterone detailed above would partly explain higher excretion of enterolignans during the mid-luteal phase and early pregnancy. However, a study by Lampe et al. (Reference Lampe, Martini, Kurzer, Adlercreutz and Slavin1994) does not support association between enterolignan excretion and the menstrual cycle. Although more work is required to achieve a consensus in results on this topic, a relationship between lignan and hormone bioavailability is supported by the observed alteration of both the menstrual cycle and levels of sex hormones following flaxseed consumption (Phipps et al. Reference Phipps, Martini, Lampe, Slavin and Kurzer1993; Brooks et al. Reference Brooks, Ward, Lewis, Hilditch, Nickell, Wong and Thompson2004).
Beyond the crucial role of bacteria in lignan conversion, the study by Kuijsten et al. (Reference Kuijsten, Arts, Vree and Hollman2005b) highlights the importance of host mechanisms in regulating lignan bioavailability. Hepatic metabolism, entero-hepatic circulation and excretion certainly regulate tissue exposure to the lignans produced in the intestinal tract.
Hepatic metabolism of lignans and tissue concentrations
Early work in rats suggested that lignans undergo enterohepatic circulation (Axelson & Setchell, Reference Axelson and Setchell1981). Since then, only two in vitro studies have investigated the production of lignan metabolites by the liver. Jacobs et al. (Reference Jacobs, Kulling and Metzler1999) obtained aliphatic and aromatic hydroxylated metabolites of ED and EL after incubating synthesised enterolignans with human hepatic microsomes. The authors also identified aromatic hydroxylated metabolites in the urine of two women and two men (Jacobs et al. Reference Jacobs, Kulling and Metzler1999). Using hepatic microsomes too, Niemeyer et al. (Reference Niemeyer, Honig, Kulling and Metzler2003) detected oxidative metabolites of MAT and SECO. The biological properties of these lignan metabolites remain to be determined and the hepatic conjugation of lignans in human subjects has to be proven.
In a study using a single dose of [3H]SDG in twenty-four female Sprague–Dawley rats (Rickard & Thompson, Reference Rickard and Thompson1998), the twelve tissues analysed for the presence of radioactivity contained in total about 5 % of the recovered dose of radioactivity. Most SDG metabolites were excreted within 48 h after administration. Approximately 30 % of the recovered dose was detected in urine and 50 % in faeces. The liver was one of the tissues with high concentrations of lignan metabolites. Other tissues with high concentrations were the intestine (mainly the caecum), kidney and uterus. In human subjects, lignan concentrations have been measured in semen, because in the early 1980s enterolignans were suspected to be of gonadal origin, in prostatic fluid and tissue and in breast cyst fluid because of their possible role in the prevention of prostate and breast cancer (McCann et al. Reference McCann, Gill, McGlynn and Rowland2005; Thompson et al. Reference Thompson, Chen, Li, Strasser-Weippl and Goss2005). In semen of six men, EL occurred in both conjugated and unconjugated forms at concentrations between 63 and 557 nmol/l (Dehennin et al. Reference Dehennin, Reiffsteck, Jondet and Thibier1982). In prostatic fluid, the mean concentration of EL ranged from 68 nmol/l in British men to 544 nmol/l in Portuguese men, in whom the highest concentrations exceeded 2 μmol/l (Morton et al. Reference Morton, Chan, Cheng, Blacklock, Matos-Ferreira, Abranches-Monteiro, Correia, Lloyd and Griffiths1997). In prostatic tissue, enterolignan concentrations were two- to three-fold higher than in plasma (17–93 v. 7·5–29 nmol/l) (Hong et al. Reference Hong, Kim, Kwon, Lee and Chung2002), but the authors did not assess differences in tissue concentrations between ED and EL. In 191 women, the intracystic median concentration of EL was 63 nmol/l whereas the concentration in the serum was 17 nmol/l (Boccardo et al. Reference Boccardo, Lunardi, Petti and Rubagotti2003). These data indicate that tissue accumulation of lignans may occur. This is of primary importance with regard to the health effects of lignans. For instance, tissue accumulation of the plant lignan arctigenin could lead to changes in immune responses through modulation of MAP kinase kinase activity, NF-κB activation and TNFα production (Cho et al. Reference Cho, Kim, Yoo, Baik and Park1999). Tissue accumulation of lignan metabolites could also lead to the inhibition of steroid-metabolising enzymes (Adlercreutz et al. Reference Adlercreutz, Bannwart, Wahala, Makela, Brunow, Hase, Arosemena, Kellis and Vickery1993a). If at all possible, future clinical studies focusing on the health effects of dietary lignans should include measurements of lignans in tissues. For example, lignan concentrations in breast tissues could be measured in studies with breast cancer patients scheduled for biopsy.
Lignan excretion
Urinary excretion
Urinary excretion has been the most widely studied aspect of lignan bioavailability. A few key points are summarised here. As in the case of blood levels, urinary excretion of enterolignans is characterised by higher baseline values of EL (100–5000 nmol/d) than of ED (10–1000 nmol/d) (Axelson & Setchell, Reference Axelson and Setchell1980; Adlercreutz et al. Reference Adlercreutz, van der Wildt, Kinzel, Attalla, Wahala, Makela, Hase and Fotsis1995b; Lampe et al. Reference Lampe, Gustafson, Hutchins, Martini, Li, Wahala, Grandits, Potter and Slavin1999; Hutchins et al. Reference Hutchins, Martini, Olson, Thomas and Slavin2000). A dose-dependent increase in ED and EL excretion is observed after dietary supplementation of lignan-containing foods (Lampe et al. Reference Lampe, Martini, Kurzer, Adlercreutz and Slavin1994; Hutchins et al. Reference Hutchins, Martini, Olson, Thomas and Slavin2000) and enterolignan concentrations in urine correlate well with those in blood (Valentin-Blasini et al. Reference Valentin-Blasini, Blount, Caudill and Needham2003; Kuijsten et al. Reference Kuijsten, Arts, Vree and Hollman2005b). However, while the mean proportion of free and sulfated enterolignans is approximately 20 % in blood (Adlercreutz et al. Reference Adlercreutz, Fotsis, Lampe, Wahala, Makela, Brunow and Hase1993b), their proportion in urine is below 10 % (Axelson & Setchell, Reference Axelson and Setchell1980; Adlercreutz et al. Reference Adlercreutz, van der Wildt, Kinzel, Attalla, Wahala, Makela, Hase and Fotsis1995b). Mono-glucuronidated and sulfo-glucuronidated enterolignans ranged from 73 to 94 % and from 1 to 17 % of total urinary enterolignans, respectively. Similar percentages have been observed for the plant lignan MAT and for endogenous oestrogens (Axelson & Setchell, Reference Axelson and Setchell1980; Adlercreutz et al. Reference Adlercreutz, van der Wildt, Kinzel, Attalla, Wahala, Makela, Hase and Fotsis1995b). Interestingly, enterolignans were detected in the urine of a 6-year-old child at approximately 168 nmol/d (Axelson & Setchell, Reference Axelson and Setchell1980), which shows the potential of a child's intestinal microbiota to produce enterolignans. In the study by Kuijsten et al.. (Reference Kuijsten, Arts, Vree and Hollman2005b), the total amount of ED and EL detected in urine was 40 % of the ingested dose of 500 mg SDG, the majority of which was excreted within 2 d. The residence time of enterolignans was lower in women than in men (17·3 v. 23·9 h). In earlier studies (Stitch et al. Reference Stitch, Toumba, Groen, Funke, Leemhuis, Vink and Woods1980; Setchell et al. Reference Setchell, Lawson, Conway, Taylor, Kirk, Cooley, Farrant, Wynn and Axelson1981), both enantiomers of EL were detected in urine and the authors proposed that urinary EL is racaemic.
Faecal excretion
Very few data on faecal excretion of lignans are available. In nine omnivorous Finnish women consuming their usual diet, the daily amount of MAT and ED excreted in faeces was approximately 22 and 148 nmol, respectively (Adlercreutz et al. Reference Adlercreutz, Fotsis, Kurzer, Wahala, Makela and Hase1995a). The excretion of EL was much higher (1–500 nmol/d). The authors found that faecal excretion of lignans was 2- to 4-fold higher in nine vegetarian women but statistical analyses were not performed. In an intervention study with thirteen women consuming 10 g ground flaxseed/d in addition to their usual diet for a period of three menstrual cycles, Kurzer et al. (Reference Kurzer, Lampe, Martini and Adlercreutz1995) observed baseline values of approximately 7, 80 and 640 nmol/d for MAT, ED and EL, respectively. After intervention, the excreted amounts of MAT, ED and EL significantly increased (12, 2-560 and 10 300 nmol/d, respectively). These data, and the fact that urinary excretion of MAT in thirty-one postmenopausal women did not increase after consumption of 10 g flaxseed/d for 7 weeks (Hutchins et al. Reference Hutchins, Martini, Olson, Thomas and Slavin2000), suggest that MAT is efficiently converted to enterolignans in the intestine. Alternatively, MAT may be absorbed as such and subsequently metabolised, for example, hydroxylated, by host enzymes.
Conclusion
Table 1 summarises important facts on lignan bioavailability in human subjects. The study of lignan bioavailability requires the investigation of lignan absorption, metabolism and excretion at different body sites. The goal is to integrate all data to gain information on physiological concentrations of biologically active metabolites. To reach this goal, more work in needed. For instance, novel host and bacterial metabolites of lignans could be found and their biological properties investigated. Quantitative approaches must be used to determine production rates and ratios of lignan metabolites. Moreover, lignan bioavailability is characterised by marked inter-individuals differences. Future human studies should include intestinal microbiota analysis to help characterising inter-individual differences in the ability to metabolise dietary lignans. Finally, almost all studies on lignans highlight possible beneficial health effects and no adverse effects of lignans have been reported so far (Kulling et al. Reference Kulling, Jacobs, Pfeiffer and Metzler1998). However, it must be kept in mind that lignans can interact with highly sensitive hormonal systems. Lignan-containing nutritional supplements should be used with great caution, especially in infants and children or during pregnancy and lactation (Ward et al. Reference Ward, Jiang and Thompson2000). No matter what health effects lignans have, it is essential to study their bioavailability.
Table 1 Important data on lignan bioavailability in human subjects*

EL, enterolactone; ED, enterodiol.
* The Table is meant to give a one-look overview of so-far acknowledged trends. The data list is not exhaustive. Values are given as references and vary between individuals and populations.
† Restricted to lariciresinol, matairesinol, pinoresinol and secoisolariciresinol.
‡ Proportion of food items (n 109) containing detectable amounts of plant lignans.