Colorectal cancer (CRC) is one of the most commonly diagnosed gastrointestinal malignancies in Western industrialised countries in both men and women aged > 50 years, but a dramatic increase among those younger people (20–49) has been reported(Reference Siegel, Miller and Goding Sauer1,Reference Sung, Ferlay and Siegel2) . Globally, CRC is the third most commonly diagnosed neoplasia and the second leading cause of cancer-related deaths(Reference Siegel, Miller and Goding Sauer1,Reference Sung, Ferlay and Siegel2) . About 55–70 % of cases are classified as sporadic, and more than 50 % of these cases are related to modifiable risk factors. In fact, epidemiological and animal evidence indicate that a sedentary lifestyle and ‘westernised’ dietary habits, including a high intake of red and processed meat, saturated fats and refined starches, associated with a low consumption of fresh fruit/vegetables and fibre, are risk factors for CRC development(Reference Siegel, Miller and Goding Sauer1–Reference Keum and Giovannucci6). In order to identify potential dietary agents against sporadic CRC development, carcinogen-induced preclinical models in rodents have been widely used for the screening of new preventive strategies(Reference Corpet and Pierre7–Reference Fleet9). In these translational rodent models, 1,2-dimethylhydrazine (DMH) hydrochloride and its main metabolite azoxymethane (AOM) are potent genotoxic agents to induce colonic preneoplastic (i.e. aberrant crypt foci (ACF)) and neoplastic (i.e. adenomas and adenocarcinomas) lesions that resemble human CRC in most morphological and molecular aspects(Reference Rosenberg, Giardina and Tanaka10–Reference Ward and Treuting12).
In this context, the photosynthetic cyanobacteria Arthrospira spp., an edible blue-green microalga, has been widely studied and commercialised worldwide. These cyanobacteria contain high protein levels that can reach between 60 and 70 % of its dry-weight biomass(Reference Lupatini, Colla and Canan13–Reference Barros de Medeiros, da Costa and da Silva15). Other bioactive compounds have been described including antioxidants (β-carotene and C-Phycocyanin (C-PC)), minerals (P, K, Na, Ca, Mg, Fe, Zn), vitamins (E, B1, B2, B3, B9), essential amino acids, PUFA (especially γ-linolenic acid (18:3 n-6)) and some phenolic compounds(Reference Lupatini, Colla and Canan13–Reference Papalia, Sidari and Panuccio16). In special, C-PC is a natural light-blue pigment used in food, cosmetic and pharmaceutical products or formulations(Reference Pez Jaeschke, Teixeira and Marczak17). Nowadays, Arthrospira spp. powder is used as a nutraceutical and functional food supplement and is clinically recommended for patients with higher risk factors for CVD or the metabolic syndrome, including arterial hypertension, insulin resistance, hypercholesterolaemia and obesity(Reference Martínez-Sámano, Torres-Montes de Oca and Luqueño-Bocardo18–Reference Ramos-Romero, Torrella and Pagès20).
Some in vivo studies have demonstrated that Arthrospira spp. has preventive properties against oral, liver, skin and breast carcinogenesis, exerting anti-inflammatory, antioxidant and pro-apoptotic properties(Reference Grawish, Zaher and Gaafar21–Reference Mahmoud, Shehata and Fares24). Álvarez-González et al. (Reference Álvarez-González, Islas-Islas and Chamorro-Cevallos25) demonstrated a potential preventive effect of oral administration of high concentrations of Arthrospira maxima (100, 400 and 800 mg/kg) on the initial stages of AOM-induced colon carcinogenesis. Nonetheless, the mechanistic landscape of this preventive effect is not fully unveiled. Thus, in the light of the potential beneficial properties of Arthrospira (Spirulina) platensis (AP)(Reference Wu, Liu and Miron26), we evaluated the protective effects of AP feeding, before and during DMH administrations, against carcinogen-induced colonic mucosal damage and preneoplastic lesion development in rodents.
Materials and methods
Chemicals, Arthrospira (Spirulina) platensis composition and chow preparation
1,2-dimethylhydrazine hydrochloride (PubChem CID: 1322) was obtained from Sigma–Aldrich, and AP powder was originated and generously donated by Fazenda Tamanduá with the following composition per gram: proteins (66·7 %), total carbohydrates (20·0 %), total lipids (9·33 %) and fibre (6·0 %) expressed on dry weight. According to Pereira et al. findings, C-PC levels in AP powder are 0·492 (sd 0·086) mg/g (in triplicate). AP powder was incorporated into a standard rodent chow (Nuvilab-CR1, Nuvital), achieving the final concentration of 1 or 2 % (10 or 20 g of AP powder/kg chow). Thus, C-PC concentrations in chows were estimated: 4·92 (AP 1 %) and 9·84 (AP 2 %) mg/kg. The balanced diet was standardised according to the National Research Council’s recommendation affording the rodent nutritional necessities(27). The chow was completely homogenised and humidified into an industrial mixer (model M60, CAF), then pelleted (7·5 CV model, Chavantes), dried trough ventilation, stored into identified plastic bags and finally kept under refrigeration (−4°C). Representative photos of each chow are displayed in Fig. 1. The nutritional composition of the experimental chow can be found in Table 1.

Fig. 1. Experimental design (for details see Material and Methods section). DMH, 1,2-dimethylhydrazine hydrochloride; EDTA, ethylenediamine tetraacetic acid; ACF, aberrant crypt foci; RT-qPCR, real-time quantitative PCR; IHC, immunohistochemistry; E, euthanasia.
Table 1. Nutritional composition of experimental chows (in g/g)

AP, Arthrospira platensis; F-PC, C-Phycocyanin.
Study design and sampling
For the sample size calculation, the total number of animals (n 68) was estimated using the G × Power 3.1118 software, considering a significance level (α) of 5 %, power (1-β) close to 95 % and effect (f) corresponding to 0·55 (large effect), as previously recommended(Reference Charan and Kantharia28). The calculation can be found in online Supplementary Data 1. Following a 2-week acclimation period, seventy-six four-week-old male Sprague Dawley rats – obtained from Multidisciplinary Center for Biological Research (CEMIB) at the State University of Campinas (UNICAMP, Campinas, SP, Brazil) – were randomly distributed into five groups (G1–G3, n = 16 rats group; G4–G5, n = 10 rats/group) (Fig. 1). Chows were offered to the groups as it follows: standard chow (G1 and G5), or standard chow containing AP at 1 (G2) or 2 % (G3 and G4) for 4 weeks. AP powder concentrations in chow were based on European Food and Safety Authority Scientific Committee’s recommendations, indicating that feeding interventions should not exceed 5 % in order to avoid nutritional imbalances in rodents(29). Indeed, AP was safely consumed – considering body weight evolution and histopathological endpoints – up to 5 % supplemental level in both male and female mice(Reference Yang, Park and Cassada30). Injections of DMH (G1–G3; 40 mg/kg body weight) or DMH vehicle (G4 and G5; Na2EDTA, 37 mg/l, 5 ml/kg body weight) were subcutaneously applied twice a week at weeks 3 and 4 (Fig. 1), as previously established by our research group for male rats(Reference Caetano, Tablas and Romualdo31). At the week 4, 24 h after the last DMH administration, eight (G1–G3) or five (G4 and G5) animals of each group were euthanised. The remaining rats had their AP-supplemented chows (G2–G4) replaced for standard chow (as G1 and G5) for more 8 weeks, until they were euthanised at week 12 (Fig. 1). In both procedures, rats were euthanised by exsanguination under ketamine/xylazine anaesthesia (160 and 10 mg/kg body weight, respectively).
At necropsy (weeks 4 and 12), colon samples were opened longitudinally, cleaned with 0·9 % NaCl, pinned flat, fixed in 10 % phosphate-buffered formalin during 24 h and then kept in 70 % ethanol for histological analysis. Distal portions of the colon mucosa (week 4) were also sampled, snap frozen in nitrogen and stored at −80°C for molecular analysis. Body weight, water and chow consumption were weekly registered during the experiment. Animals were accommodated in polypropylene cages (2 rats per cage) under accepted conditions (22 (sd 2°C) temperature, 55 (sd 10) % humidity, 12 h light/12 h dark cycle) with free access to drinking water and chow. The study was developed according to the principles of the institution’s Ethics Committee Board under the protocol CEUA-1128/2019.
Immunohistochemistry for Ki-67, caspase-3 and γ-H2AX at week 4
Ki-67 (i.e. proliferation), active caspase-3 (i.e. apoptosis) and γ-H2AX (i.e. DNA damage) markers were detected in colon sections. Briefly, deparaffinated 5-μm colon histological sections were placed onto silanised slides and sequentially treated with 0·01 M citrate buffer (pH 6·0) at 120°C for 5 min in a Pascal Pressure Chamber (Dako Cytomation Denmark A/S), 10 % H2O2 in PBS for 10 min, skimmed milk for 60 min, anti-Ki-67 (1:200 dilution, MA5-14520, Thermo Fisher Scientific), anti-active caspase-3 (1:100 dilution, ab179517, 1:200, Abcam) and anti-γ-H2AX (1:100 dilution, MA5-27753, Thermo Fisher Scientific) antibodies overnight at 4°C, a one-step horseradish peroxidase polymer (EasyPath – Erviegas) for 20 min at room temperature. Chromogen colour was accomplished with 3,3-diaminobenzidine tetrahydrochloride (Sigma–Aldrich). The slides were counterstained with Harris haematoxylin for 1 min. For each group (n 5 each group), twenty randomly entire colonic crypts were scored per animal. Ki-67, caspase-3 and γ-H2AX labelling indexes (LI%) were scored by the number of positive-stained cells/number of cells per crypt ratio.
Low density gene array, functional and network analysis at week 4
Total RNA was isolated from distal colon samples (n 5 each group) with the RNeasy Mini kit (Qiagen) following the manufacturer’s instructions. The quantification of RNA was performed by NanoVue Plus (GE HealthCare) and the quality was evaluated using the Bioanalyzer 2100 platform (Agilent Technologies). The cDNA synthesis was performed using the SuperScript VILO cDNA Synthesis Kit and Master Mix (Thermo Scientific) following the manufacturer’s instructions. TaqMan Low Density Array cards (TLDA, Life Technologies) were used for real-time quantitative PCR following the manufacturer’s instructions.
TLDA cards comprised ninety-four genes involved in DNA repair, anti/pro-oxidant metabolism, cell proliferation, death and differentiation (online Supplementary Data 2). Briefly, 100 µl of cDNA template (422 ng mRNA) was added to 100 μL of TaqMan Fast Advanced Master Mix (Life Technologies) and dispensed into loading wells on the TLDA card. The cycling protocol included heat activation at 50°C for 1 min and denaturation at 95°C for 10 min, followed by 40 cycles of 95°C for 15 s and 60°C for 1 min. Fluorescence detection was performed on a QuantStudio 12 K Flex Real-Time PCR System (Life Technologies). Relative quantitation was calculated based on the 2–(ΔΔCt) method(Reference Livak and Schmittgen32), using Expression Suite Software v1.1 (Life Technologies). Actb, Gapdh, Gusb and Hprt housekeeping genes were used for normalisation.
The output list of differentially expressed mRNA (≥ 1·5 fold change) was analysed on Gene Ontology and Kyoto Encyclopedia of Genes and Genome database via the DAVID tools website (https://david.ncifcrf.gov/) in order to identify significantly enriched biological pathways (P < 0·05; enrichment score = –log (P value))(Reference Huang, Sherman and Lempicki33). Network confidence analysis was carried out using the STRING database (https://string-db.org/).
Screening of colonic preneoplastic lesions at week 12
All the segments (distal, medial and proximal) from each animal were stained with 0·2 % methylene blue solution. ACF are clusters of abnormal crypts (AC) displaying oval-like lumens and a thicker epithelial cell lining(Reference Bird and Good34). The total number of ACF, AC and the number of ACF with ≤ 4 AC or > 4 AC were counted according to Bird’s criteria, under light microscopy at 20× magnification(Reference Bird and Good34).
Statistical analysis
Data from body weight gain, water and chow consumption, immunohistochemistry and ACF analysis were compared among the groups using one-way ANOVA or Kruskal Wallis followed by post hoc Tukey’s test. The analyses were performed using the GraphPad Prism software (V4.03, GraphPad). The pairwise comparisons of gene expression data were performed and analysed by using the Student t test. Correlations between gene expression (week 4) and ACF data (week 12) were performed using Pearson’s coefficient (r), considering data from animals that shared the same housing environment (cage) from weeks 1 to 4. The differences among groups were considered significant when P < 0·05.
Results
Both weight, water and chow consumption at weeks 4 and 12
During the 4-week AP feeding period, no significant alteration in body weight, body weight gain or chow and water consumption was observed with exception to absolute liver weight that was lower in DMH-initiated groups (G1–G3) when compared with the non-initiated groups (G5 and G6) (Table 2). The average consumption of AP powder during the 4 weeks was 236·4 (sd 25·5) (G2), 489·4 (sd 52·1) (G3) and 470·1 (sd 42·8) (G4) mg/rat per d (data are mean values and standard deviation). Based on average AP intake, the estimated C-PC consumption was 0·11 (sd 0·01) (G2), 0·24 (sd 0·02) (G3) and 0·23 (sd 0·02) (G4) mg/rat per d (data are mean values and standard deviation). In addition, the mean body weight and liver absolute and relative weights did not differ among groups from weeks 5 to 12 (online Supplementary Data 3). At week 12, survival rates were 100 % in both DMH and vehicle groups.
Table 2. Effects of Arthrospira (Spirulina) platensis feeding on body weight (g), food (g/rat per d) and water (ml/rat per d) consumption and liver weights (absolute and relative) in the different experimental groups at week 4 (Mean values and standard deviations)

* Different from groups G4 and G5, P < 0·001.
† AP = Arthrospira (Spirulina) platensis at 1 % or 2 % in the chow and DMH = 1,2-dimethylhydrazine dihydrochloride (4 × 40 mg/kg body weight, subcutaneous.); n = number of rats/group. Data were analysed using one-way ANOVA followed by Tukey’s test.
Colonic cell proliferation, apoptosis and DNA damage at week 4
Twenty-four hours after the last DMH administration, the γ-H2Ax and caspase-3-associated with DNA insult LI%, but not Ki-67 LI%, were significantly increased in the colonic mucosa from DMH-initiated groups (G1–G3, n 8 each) in comparison with non-initiated groups (G4 and G5, n 5 each) (Fig. 2–4). Noteworthy, a significant (P < 0·001, for both) reductions in γ-H2AX and caspase-3 LI% were observed in the colonic mucosa from DMH-initiated fed with AP at 1 and 2 % groups (G2 and G3) when compared with only control DMH-initiated group (G1) (Fig. 2 and 3). However, colonic cell proliferation LI% did not significantly differ among groups (Fig. 3).

Fig. 2. Effects of Arthrospira (Spirulina) platensis feeding on γ-H2AX labelling index (LI%) in the different experimental groups at week 4. Representative photomicrographs of phospho-H2A.X-immunostained sections of colonic crypts are presented (scale bar: 50 µm). Data are mean values and standard deviation (n 5–8 rats each group). Different letters correspond to statistical difference by ANOVA followed by Tukey’s test (P < 0·05). AP, Arthrospira (Spirulina) platensis powder at 1 % or 2 % in the chow (w/w); DMH, 1,2 dimethylhydrazine dihydrochloride (4 × 40 mg/kg body weight by subcutaneous injections).

Fig. 3. Effects of Arthrospira (Spirulina) platensis feeding on caspase-3 labelling index (LI%) in the different experimental groups at week 4. Representative photomicrographs of γ-H2AX-immunostained sections of colonic crypts are presented (scale bar: 25 µm). Data are mean values and standard deviation (n 5 rats each group). Different letters correspond to statistical difference by ANOVA followed by Tukey’s test (P < 0·05). AP, Arthrospira (Spirulina) platensis powder at 1 % or 2 % in the chow (w/w); DMH, 1,2 dimethylhydrazine dihydrochloride (4 × 40 mg/kg body weight by subcutaneous injections).
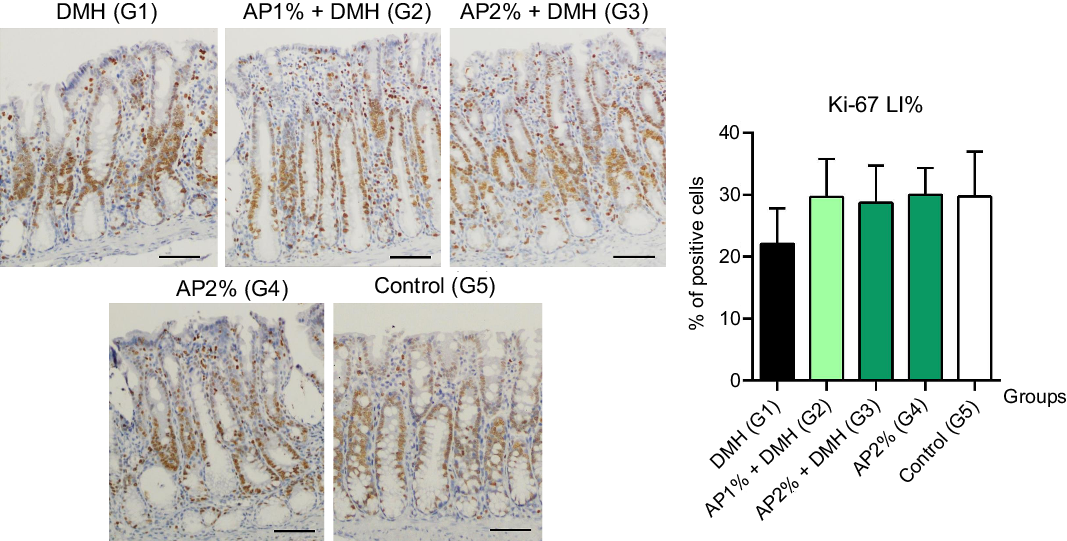
Fig. 4. Effects of Arthrospira (Spirulina) platensis feeding on Ki-67 labelling index (LI%) in the different experimental groups at week 4. Representative photomicrographs of Ki-67-immunostained sections of colonic crypts are presented (scale bar: 50 µm). Data are mean values and standard deviation (n 5 rats each group). Different letters correspond to statistical difference by ANOVA followed by Tukey’s test (P < 0·05). AP, Arthrospira (Spirulina) platensis powder at 1 % or 2 % in the chow (w/w); DMH, 1,2 dimethylhydrazine dihydrochloride (4 × 40 mg/kg body weight by subcutaneous injections).
Gene expression analysis at week 4
Twenty-four hours after the last DMH administration, the levels of mRNA encoding target proteins involved in the cell cycle, DNA repair and apoptosis pathways were measured in colonic mucosa samples at week 4(Reference Caetano, Tablas and Romualdo31). Gene expression analysis revealed 16 down-regulated genes and one up-regulated gene in the colonic mucosa of DMH-initiated and AP 2 %-fed rats (G3) compared with the DMH counterpart (G1) (Table 3). Genes involved in the Notch pathway were decreased – as Notch1, Notch2 and Jag1 – as well as cell cycle/DNA repair-related ones – including Atm, Wee1, Chek2, Mgmt, Ogg1 and Xrcc6. Of note, Cyp2e1 gene encoding DMH metabolising cytochrome P450 CYP2E1 enzyme was down-regulated in AP 2 %-fed rats. Raw data of the gene expression profile from and DMH-initiated fed 2 % AP group (G3) are included in online Supplementary Data 2.
Table 3. Differentially expressed genes in the colonic mucosa from the animals fed Arthrospira (Spirulina platensis) at 2 % and DMH-initiated (group G3) compared with only DMH-initiated group (G1) (n 5 rats/group.)*

* AP = Arthrospira (Spirulina) platensis) at 2 % in the chow and DMH = 1,2-dimethylhydrazine dihydrochloride (4 × 40 mg/kg b.wt., s.c.). The groups were compared using Student’s t test. Fold change boundary of 1·5 and a P < 0·05 were used.
As expected, functional enrichment analyses of the differentially expressed genes in AP 2 %-fed mice (G3) indicated a significant correlation between the down-regulation of these genes and ‘Notch signalling pathway’, ‘Cell cycle’ and ‘Base excision repair’ functional annotations (Fig. 5). STRING network confidence analysis was in keeping with these functional correlations, as Notch1, Notch2 and Jag1 network nodes are strongly functionally correlated, a featured also observed among Xrcc6, Atm, Chek2 and Wee1.

Fig. 5. (a) Effects of AP2 % treatment on the enrichment of pathways ranked by –log10 (P value). The mRNA expressed differentially are associated with apoptosis (P = 0·008) and Notch signalling pathways (P = 0·0038). (b) STRING confidence network analysis. Nodes of the correlated proteins are presented (with 3D structure inside). Edges correspond to the confidence of functional correlation (caption).
Colonic preneoplastic lesion analysis at week 12
Classical ACF were evaluated in methylene blue-stained whole-mount colon samples(Reference Bird and Good34) at the end of week 12 (online Supplementary Data 4). No ACF were observed in non-initiated groups (G4 and G5). Data from the number and multiplicity (AC/ACF) of stereoscopically analysed ACF in the different DMH-initiated groups are summarised in Table 4. The mean number of ACF with ≤ 4 crypts, and > 4 AC, and the total number of ACF and AC were significantly (0·008 < P < 0·01) lower in the group fed AP at 1·0 or 2·0 % (G2 and G3) when compared with the DMH-initiated group (G1).
Table 4. Effects of Arthrospira (Spirulina) platensis feeding on the development of colonic aberrant crypt foci (ACF) in the different groups at the end of week 12† (Mean values and standard deviations)

† Values are mean values and standard deviations.
‡ AP = Arthrospira (Spirulina) platensis powder at 1 % or 2 % in the chow (w/w) and DMH = 1,2-dimethylhydrazine dihydrochloride (4 × 40 mg/kg b.wt., s.c.).
§ AC = aberrant crypts. Data were analysed using one-way ANOVA followed by Tukey’s test.
*,**,***Different from group G1, P < 0·05, P = 0·008 and P < 0·01, respectively.
Correlations
We evaluated whether the 2 % AP-mediated alterations in DNA damage and Notch pathway at week 4 are correlated with AC/ACF development at week 12. The reduction of γ-H2AX was positively correlated with the reductions in AC (P = 0·047, r 0·63) and ACF (P = 0·007, r = 0·77) (Fig. 6). Only the down-regulation of Notch1 was positively correlated with the reductions in AC (P = 0·089, r 0·56) and ACF (P = 0·04, r 0·64) (Fig. 6), not Notch2.

Fig. 6. Correlation between DNA damage marker or Notch1 and Notch2 genes at week 4 and preneoplastic ACF development at week 12. Correlations were performed using Pearson’s coefficient (r) and were considered significant when P < 0·05.
Discussion
In this feeding study, we investigated the beneficial effects of dietary AP interventions, before and during carcinogen administration, on the colonic mucosal acute damage as well as on the late development of putative preneoplastic lesions using a medium-term bioassay for rat colon carcinogenesis. Twenty-four hours after the last DMH administration, the results showed that both dietary AP interventions significantly reduced carcinogen-induced DNA damage and apoptosis in epithelial cells of the colonic crypts. In addition, AP at 2 % feeding significantly reduced expression of Notch1, Notch2 and Jag1 genes (Notch pathway), Atm, Wee1, Chek2, Mgmt, Ogg1 and Xrcc6 genes (DNA repair pathway) and Cyp2e1 (Metabolism) in the colonic mucosa in comparison with the only DMH-initiated group. As AP feeding reduced the noxious effect of DMH on the colonic mucosa, a significant reduction in mean number of AC and ACF development was also detected for both AP interventions groups at the end of week 12. The estimated AP intake was 236 (G2) and ∼470–490 (G3 and G4) mg/rat per d, corresponding to a daily dose of 0·81 (G2) and 1·6 (G3 and G4) g/kg/d (online Supplementary Data 5). Using the Human Equivalent Dose allometric dose translation formula(Reference Reagan-Shaw, Nihal and Ahmad35), the estimated animal dose used herein corresponds to a dose of 130 (AP at 1 %) and 250 (AP at 2 %) mg/kg per d in humans (online Supplementary Data 5). Although there are no clinical studies on the chemopreventive effects of AP in humans, our preclinical intervention converted by Human Equivalent Dose approach is higher – while safe – than previous human studies showing antihypertensive (∼28 mg/kg per d) or anticoagulant (∼32 mg/kg per d) activities in high dose interventions(Reference Jensen, Drapeau and Lenninger36,Reference Ghaem Far, Babajafari and Kojuri37) .
Several animal studies have demonstrated potential beneficial effects of AP intervention in different chemically induced carcinogenesis models. Dietary 1 % SP powder resulted in a lower incidence of tumours, tumour Ki-67 and oestrogen receptor-positive labelling indexes in a classical 7,12-dimethylbenz(a)anthracene-induced mammary cancer model in female Sprague-Dawley rat strain(Reference Ouhtit, Ismail and Othman23), when Arthrospira spp. was administered after a single carcinogenic exposure. In a 7,12-dimethylbenz(a)anthracene-induced hamster buccal pouch carcinogenesis, an AP extract (10 mg/d) fed during the 7,12-dimethylbenz(a)anthracene painting regimen suppressed the development of epithelial dysplasia and squamous cell carcinoma accomplished by a reduction in cell proliferation indexes(Reference Grawish, Zaher and Gaafar21).
Álvarez-González et al. (Reference Álvarez-González, Islas-Islas and Chamorro-Cevallos25) showed that a Spirulina maxima powder suspension, administered before, during and after AOM administrations, inhibited ACF development by 66·4, 46·2 and 42·3 % for 200, 400 and 800 mg/kg doses in relation to the AOM counterpart. These 4-week oral treatments also decreased lipid and DNA oxidation levels as evaluated by malondialdehyde and 8-hydroxy-2’-deoxyguanosine (8-oxoG) adducts markers. In an hepatocarcinogenesis mouse model induced by a specific diethylnitrosamine and carbon tetrachloride regimen, a SP powder suspension (250 and 500 mg/kg body weight), administered by gavage during weeks 25–28, reduced the number and size of macroscopic liver nodules and serum tumour biomarkers such as α fetoprotein, MDA and total antioxidant capacity while it increased serum total protein and animal survival(Reference Mahmoud, Shehata and Fares24). Yogianti et al. (Reference Yogianti, Kunisada and Nakano22), using an ultraviolet radiation B (UVB)-induced skin mouse tumour development model(Reference Yogianti, Kunisada and Nakano22), demonstrated that dietary 10 % SP powder attenuated tumour induction and development with a reduction in 8-oxoG adducts in the skin after UVB exposure in both Ogg1 knockout- and wild-type male mice. In addition, dietary SP suppressed the phosphorylation of biomarkers p38 mitogen-activated protein kinase, stress-activated protein kinase/c-Jun N-terminal kinase and extracellular signal-regulated kinase proteins in the skin after UV radiation exposure, especially in Ogg1-knockout male mice. In general, these findings are in keeping with our investigation, reinforcing the protective effect of AP treatment on different preclinical models of carcinogenesis.
Regarding the chemically induced model used, DMH and its main metabolite AOM are procarcinogens that undergo metabolic activation by a hepatic CYP2E1 (encoded by Cyp2e1), and later also by gut bacterial enzymes to produce reactive metabolites that induces DNA insults in colon epithelial cells(Reference Rosenberg, Giardina and Tanaka38–Reference Venkatachalam, Vinayagam and Arokia Vijaya Anand40). These reactive ions alkylate specific genomic DNA bases, resulting in specific DNA adducts, such as O6-methylguanine (O6-mG) and N7-methylguanine (N7-mG) in both liver and colon targets. If not removed, these DNA adducts can lead to genomic instability and after a replication cycle and significantly contribute to the initiation of rodent colon carcinogenesis(Reference Rosenberg, Giardina and Tanaka38–Reference Venkatachalam, Vinayagam and Arokia Vijaya Anand40).
In this rat study, we demonstrated that AP at 2·0 %-treated rats showed decreased expression of Cyp2e1 gene, an important key target also involved in drug metabolism, including DMH bioactivation on colonic mucosa(Reference Zanger and Schwab41,Reference Reed, Arlt and Phillips42) . An increase or decrease in CYP2E1 activity may affect the DMH/AOM metabolism, which in turn could influence tumour initiation induced by these two classical colon carcinogens(Reference Megaraj, Ding and Fang39). Sohn et al. (Reference Sohn, Fiala and Requeijo43) demonstrated that metabolic activation of AOM is affected differently in Cyp2e1-knockout mice in comparison with the Cyp2e1- wild-type mice. The metabolic activation of AOM in Cyp2e1-knockout mice leads to a significant reduction in DNA guanine alkylation on epithelial colonic cells when compared with the counterpart mice. In concordance, our results revealed a significant reduction in γ-H2Ax (i.e. a DNA damage biomarker) and in acute apoptotic response (i.e. active caspase 3) in epithelial cells of the colonic crypts in both dietary AP interventions groups in comparison with the counterpart group. Therefore, our finding suggests that AP feeding could inhibit CYP2E1 activity in the colon and, potentially in the liver, reducing the genotoxic and apoptotic impact of DMH on the colonic mucosa. In accordance with our findings, Savranoglu and Tumer(Reference Savranoglu and Tumer44) have demonstrated that SP oral treatment resulted in inhibition of the specific cytochrome P450 hepatic isozymes in male Wistar rats. In this animal study, the authors observed a significant reduction in hepatic expression levels and inhibition in enzymatic activities of CYP1A2 and CYP2E1.
In addition, our findings showed that the AP at 2 % feeding results in a reduced expression in Mgmt, Ogg1 and Xrcc6 on the colonic mucosa in comparison with the counterpart group. MGMT encodes a specific repair enzyme that removes the O6-mG adducts through covalent transfer of the alkyl group to the conserved active site, cysteine, restoring the normal structure of guanine. The OGG1 (8-oxoguanine DNA glycosylase) gene encodes a DNA glycosylase/AP lyase that removes 8-OH-G lesions from genomic DNA while the Xrcc6 (X-ray repair cross complementing 6) gene is associated with DNA recombination and repair events(Reference Jia, Ren and Yan45–Reference Yu, Zhang and Wei47). According to this finding, we suggest that these repair genes are down-regulated due to lower DNA damage induced by DMH, probably due to the failure to activate this carcinogen and consequently the lower O6-mG and free radical formation, since DMH, as a prototype alkylating agent, also inducing extensive DNA oxidative damage in both liver and colon(Reference Rosenberg, Giardina and Tanaka38,Reference Perše and Cerar48) . Herein, authors understand that decreased DMH-induced impact may be associated with the direct radical scavenging of AP compounds, as C-PC and β-carotene. Its photosynthetic pigment C-PC scavenges free radicals, suppresses iNOS expression and nitrite production and inhibits lipid peroxidation while β-carotene reduces singlet oxygen-mediated lipid peroxidation, intracellular accumulation of reactive oxygen species (ROS) and expression of several inflammatory genes(Reference Fiedor and Burda49).
In our feeding study, it was demonstrated that dietary 2 % AP inhibited the expression of genes in the Notch pathway. The Notch pathway was more significantly enriched, according to the DAVID functional analysis, with enriched Gene Ontology terms in the colonic mucosa from 2 % AP feeding animals. Notch signalling is commonly deregulated in CRC and increased Notch1 expression has been associated with tumour progression, tumour grade and metastasis(Reference Vinson, George and Fender50). It is known that Notch signalling is a potential therapeutic target against tumour initiation and progression, as it plays a major role in the colonic crypt homoeostasis via the fine regulation of stem cell self-renew, behaviour and controlled differentiation(Reference Miyamoto and Rosenberg51). As such, bioactive compounds contained in AP modulating Notch signalling could be used as a strategy to prevent CRC initiation since this pathway is mediated by a highly conserved ligand-receptor apparatus that plays key roles in the regulation of cellular homoeostasis, including proliferation, survival, apoptosis, differentiation and other(Reference Platonova, Lesma and Basile52). There are no in vitro or in vivo findings regarding C-PC modulation of Notch pathway, but β-carotene, another common AP bioactive compound, reduced the protein levels of many proteins involved in Notch pathway during BALB/c mouse smoking model of gastric epithelial-mesenchymal transition(Reference Lu, Chen and Li53). Therefore, both down-regulation of Notch pathway and reduced DMH-induced impact (apoptosis, DNA damage and repair) may contribute to the decreased ACF burden at week 12. Indeed, we observed a statistical positive correlation between the decrease in DNA damage (γ-H2AX) or the down-regulation of Notch1 and AC/ACF development. As these lesions are considered putative biomarkers of the early stages of colon carcinogenesis(Reference Bird and Good34), our results elicit a chemopreventive effect of AP – in special the higher intervention – on this widely applied chemically induced models.
The present investigation lacked a dose–response effect in AP 1 % and 2 % treatments. The authors theorise that this point may be associated with the DMH protocol chosen(Reference Caetano, Tablas and Romualdo31). In fact, the administration of high DMH doses (40 mg) may cause increased deleterious impact in the colonic mucosa (higher DNA damage and apoptotic response) compared with lower doses this colon carcinogen(Reference Karthikkumar, Sivagami and Viswanathan54,Reference Ganaie, Al Saeedan and Madhkali55) , thus demanding a more pronounced protective response by AP, and implying in the absence of a dose–response effect. Further experiments using higher AP concentrations or other DMH regimens are warranted.
Conclusion
The findings of this animal study allow us to conclude that AP feeding, before and during DMH administrations, significantly reduces carcinogen-induced colonic crypt insult, contributing for a lower ACF development. This protective effect could be due to a reduction in DMH-induced mucosa damage (gene expression/DNA damage and apoptosis biomarkers) so suggesting that AP feeding may positively influence DNA damage, mutation and tumour initiation induced by a specific colon carcinogen used in a classic rat colon carcinogenesis model. Since AP has been widely studied as a food additive (in similar concentrations applied herein)(Reference Niccolai, Venturi and Galli56), our findings could inspire future clinical investigations on the role of this photosynthetic cyanobacteria.
Acknowledgements
The authors would like to thank the Coordenação de Aperfeiçoamento de Pessoal de Nível Superior (CAPES)-PROAP system for their financial support.
L. M. S.-M. and A. R. B. received fellowships from the Coordenação de Aperfeiçoamento de Pessoal de Nível Superior (CAPES)-Finance code 001 and Fundação de Amparo à Pesquisa do Estado de São Paulo (FAPESP)-# 2017/26217-7, respectively.
L. F. B., G. R. R. and N. A. de M.: conceptualisation, methodology, analysis and interpretation, writing – reviewing and editing writing; S. O. A., L. M. S-M. and A. R. B.: methodology, analysis and interpretation; M. A. G. C.: analyses and writing – reviewing and editing.
The authors report no conflict of interest.
Supplementary material
For supplementary materials referred to in this article, please visit https://doi.org/10.1017/S0007114522001350