Ageing is associated with a progressive decline in both cognitive and physical function, which can lead to a number of age-related health conditions including frailty and dementia(Reference Cesari, Landi and Vellas1). Walking is a complex task with a significant cognitive aspect, and changes in several gait parameters including speed often coexist with or precede the onset of cognitive decline in older adults(Reference Savica, Wennberg and Hagen2). Gait speed is a clinically relevant marker in older adults, due to strong associations with physical functioning and disability(Reference Montero-Odasso, Schapira and Soriano3). Previous work in our laboratory suggests that a high-DHA multi-nutrient supplement providing the n-3 PUFA, DHA and EPA, and supporting nutrients phosphatidylserine, d-α tocopherol, folic acid, vitamin B12 and Ginkgo biloba improve cognition and mobility in older females(Reference Strike, Carlisle and Gibson4).
Providing n-3 PUFA in combination with other compounds indicated to support brain function may provide greater efficacy than if supplemented in isolation, although results are so far mixed. For example, the same multi-nutrient supplement as used in our previous and present studies did not improve cerebral haemodynamic or cognitive function in healthy older adults(Reference Jackson, Forster and Bell5); however, the authors acknowledge that the cognitive tests were selected on the basis that they are able to activate the prefrontal cortex and may not be sensitive to the components in the supplement. Similarly, an n-3 PUFA multi-nutrient supplement showed no significant effects on a battery of cognitive tests of participants with prodromal Alzheimer's disease, although improvements were seen in secondary outcomes of cognitive function and hippocampal atrophy(Reference Soininen, Solomon and Visser6).
Aerobic exercise has consistently been shown to improve both mobility and cognition in older adults(Reference Smith, Blumenthal and Hoffman7). n-3 PUFA and exercise share a range of overlapping biological effects, including enhancing neurogenesis, neural plasticity and reducing inflammation(Reference Dyall8,Reference Ryan and Nolan9) . Indeed, preliminary evidence suggests combining n-3 PUFA with exercise may provide additional benefit when compared with either approach alone. For example, n-3 PUFA supplementation combined with twice weekly stationary cycle training and cognitive stimulation led to an enhanced reduction of brain atrophy in grey matter regions compared with supplementation(Reference Köbe, Witte and Schnelle10). Similarly, combining n-3 PUFA supplementation with resistance training in older females provided an additional benefit to muscle strength compared with the exercise alone(Reference Da Boit, Sibson and Sivasubramaniam11). In addition, an n-3 PUFA multi-nutrient supplement combined with exercise was recently shown to improve verbal recall and executive function in older men, more than supplementation alone(Reference Bell, Fang and Snijders12). The mechanisms underpinning these interactions are currently unclear, as the studies were not been designed to elucidate these effects. However, decreasing homocysteine (Hcy) levels may be a potential factor, as n-3 PUFA regulate the expression of genes encoding enzymes involved in Hcy metabolism(Reference Huang, Hu and Khan13) and exercise decreases Hcy levels(Reference Vincent, Braith and Bottiglieri14).
The aim of this study is to further our initial observations and examine for the first time the effects of the multi-nutrient supplement and aerobic exercise on mobility and cognition in older women. The study was restricted to female participants to enable comparison with our previous work(Reference Strike, Carlisle and Gibson4), and it has been suggested that the additive effects of combining exercise and n-3 PUFA supplementation may be limited to women only(Reference Da Boit, Sibson and Sivasubramaniam11). Women have also been shown to have a greater compliance to exercise interventions(Reference Kelley and Kelley15). Habitual gait speed was the primary outcome, with secondary outcomes related to mobility, cognition and quality of life(Reference Lara, Godfrey and Evans16).
Methods
The study is a randomised semi-blinded, placebo-controlled trial in women aged 60 years and above with a factorial design. Detailed information of the study procedure has been previously published(Reference Fairbairn, Tsofliou and Johnson17), in line with guidelines of the Template for Intervention Description and Replication(Reference Hoffmann, Glasziou and Boutron18). This study was conducted according to the guidelines laid down in the Declaration of Helsinki, and all procedures involving human subjects/patients were approved by the Bournemouth University Science Technology and Health research ethics panel (Ethics ID 10788). Prior to data collection, participants provided written informed consent. The trial was listed on www.clinicaltrials.gov (NCT03228550) and follows the Consolidated Standards of Reporting Trials statement on randomised trials(Reference Schulz, Altman and Moher19). All data were collected and analysed at Bournemouth University, UK.
Participants
Females aged 60 years and above were recruited according to the following inclusion criteria: (1) able to walk at least 50 m unaided, (2) classified as non-frail or pre-frail(Reference Fried, Tangen and Walston20) and (3) community dwelling. Exclusion criteria were: (1) vestibular impairments, (2) diagnosed neurological disorder, (3) cognitive impairment (Mini Mental Status Examination score <24), (4) lower limb surgery, (5) seafood allergy, (6) regular consumption of multivitamin or fish oil supplements within 6 months prior to baseline measurements and (6) previously received advice from a health care professional not to undertake strenuous exercise. A stratified block randomisation design was followed based on frailty classification of non-frail or pre-frail, followed by permuted block randomisation. Participant demographics can be found in Table 1. For further information, please refer to the published protocol(Reference Fairbairn, Tsofliou and Johnson17).
Table 1. Participant characteristics at baseline (Mean values and standard deviations; median values and interquartile ranges (IQR); numbers)

P, placebo supplement only; P + EX, placebo supplement and exercise; MS, multi-nutrient supplement only; MS + EX, multi-nutrient supplement and exercise; NART, National Adult Reading Test; MMSE, Mini Mental State Exam; PASE, Physical Activity Scale in the Elderly.
Interventions
Participants were allocated to one of the four groups: multi-nutrient supplement and exercise (MS + EX), placebo supplement and exercise (P + EX), multi-nutrient supplement only (MS) or placebo supplement only (P).
Dietary supplement
Participants received four capsules per d of their respective dietary supplement for 24 weeks. The daily dose from the active capsules contained 1000 mg DHA, 160 mg EPA, 20 µg vitamin B12, 1 mg folic acid, 124 mg phosphatidylserine, 240 mg G. biloba standardised leaf extract and 20 mg vitamin E. The placebo capsules contained an isoenergetic oil blend typical of the UK diet including a small amount of fish oil. A small amount of fish oil was added to the placebo capsules to aid with participant blinding, which provided a daily dose of 21·6 mg EPA and 14·4 mg DHA, an amount unlikely to produce any therapeutic benefit (online Supplementary Table S1). Participants were told to take their capsules with the largest meal of the day. The capsules were coded by the Principal Investigator, who had no involvement in the data collection, and all subsequent operational oversight and interpretation of the data were undertaken with the Principal Investigator blinded to specific participant codes. Compliance to the dietary supplement was measured by changes in whole-blood DHA levels compared with baseline, counting returned pills at 12 and 24 weeks, and exit questionnaire. Adverse events were monitored by subject self-reporting and exit questionnaire.
Exercise
The exercise intervention consisted of two group sessions per week on a Spinner Fit stationary bike. Classes lasted 30 min for the first 6 weeks and increased to 45 min for the second 6 weeks, with 5 min warm up and cool down at 7–8 on the Borg scale of rate of perceived exertion. During the main body of the sessions, participants maintained an intensity of 12–14 on the Borg scale. Compliance to the exercise intervention was monitored by recording attendances by each participant and calculated as the percentage of classes attended.
Outcome measures
The outcome measures were based on changes in mobility, cognitive function and health-related quality of life, with the primary outcome measure change in habitual walking speed. Information on the age, BMI, verbal intelligence and medication use was collected from each participant. Information on medications was self-reported, with both type and number of medications recorded. The National Adult Reading Test was used to assess verbal intelligence. All measurements were performed at baseline and end of the study.
Mobility
Participants were assessed under three gait conditions: habitual walking, fast walking and dual task (DT) walking. During the DT, participants were required to count backwards in integers of three from a randomly generated three digit number and were not instructed to prioritise either walking or counting backwards during the task. Gait speed was measured using Opal inertial sensors and analysed using Mobility Lab™ software version 3.1 (APDM Inc, http://apdm.com). Sensors were placed over the shoes. Each tested condition was repeated five times, and the mean value for each trial was used for habitual and DT gait with the maximum gait speed value being used for fast walking. The five times sit to stand test was used to assess dynamic balance and functional mobility.
Cognition
A Stroop test was used to assess interference control using Open Sesame version 3.1.1. software(Reference Mathot, Schreij and Theeuwes21). Participants were presented names of one of the four colours: blue, red, green and white, in four different font colours varying between these colours. Participants were required to identify the colour of the text. Interference control was defined as the difference between the mean time taken to respond to the congruent and non-congruent trials. Spatial memory was also assessed using Open Sesame software, based upon Nagamatsu and colleagues(Reference Nagamatsu, Chan and Davis22). Each trial comprised presentation of three dots at randomly allocated locations for 500 ms, followed by a fixation cross for 3 s. The test comprised presentation of a single red dot on the screen, either in the same location (match) or a different (non-match) location, and participants had to identify if the test dot was match or non-match. The Rey's Auditory Verbal Learning Test was used to assess verbal memory, where participants were required to recall a list of fifteen pre-set words(Reference Rey23). The trail making task was used to assess executive function(Reference Salthouse, Toth and Daniels24), where participants drew lines between targets on a piece of paper, as rapidly as possible, under four different conditions: (1) numbers condition (1–49), (2) letters condition, (A–Z), (3) alternated numbers (1–25) and letters (A–X) and (4) alternated letters (A–Y) and numbers (1–24). Scores were recorded as the total number of correct connections.
Health-related quality of life
The short-form thirty-six questionnaire was used to assess health-related quality of life(Reference Brazier, Harper and Jones25).
Biochemical measures
Biochemical measures were conducted to assess compliance to the supplementation and to better understand how changes may contribute towards any therapeutic effects on the measured outcomes. Finger pin-prick blood samples were collected, and total fatty acid content was analysed, as described previously(Reference De Rooy, Hamdallah and Dyall26). Individual fatty acids were quantified using a GC with flame ionisation detector (Agilent Technologies) and expressed as percentage of total fatty acids. A non-fasted venous blood sample was drawn to assess serum Hcy. Each sample was allowed to clot and immediately centrifuged at 2000 g for 10 min at 4°C and serum extracted. Samples were stored at –80°C and analysed within 3 months. Hcy levels were measured by competitive ELISA (Cell Biolabs Inc.) according to the manufacturer's instructions.
Diet and lifestyle
Participants’ diet and lifestyle habits were monitored. Three-day food diaries were used, and results were analysed using Nutritics dietary analysis software (www.nutritics.com). A validated FFQ was used to quantify n-3 PUFA intake(Reference Sublette, Segal-Isaacson and Cooper27). The Community Health Activities Program for Seniors questionnaire was used to assess physical activity levels. These measures were taken so that baseline diet and physical activity habits could be included as covariates and to monitor whether there were any significant changes in diet and lifestyle during the course of the intervention period.
Statistical analysis
Statistical analyses were performed using IBM SPSS statistics version 21. Sample size was determined based on the primary outcome of habitual walking speed. Using an effect size based on previously published values, minimally significant changes in gait speed were 0·03 m/s and 0·05 m/s with substantial changes at 0·08 m/s(Reference Kwon, Perera and Pahor28). The sample size calculation was based on a difference of 0·08 m/s with the level of variability set at 0·1 based on our previous work(Reference Strike, Carlisle and Gibson4) with a minimum sample size of thirteen participants per group required to detect an effect size d of 0·5. In our original protocol, a large effect size was suggested; however, this was subsequently changed to a medium effect size based on our previous pilot study(Reference Strike, Carlisle and Gibson4,Reference Fairbairn, Tsofliou and Johnson17) . Data were tested for normal distribution using the Shapiro–Wilk test and Q-Q plots and Levene's test of equality of error variances to check for assumptions of homogeneity. Correlations between mobility, cognitive function, and health-related quality of life, exercise, circulating n-3 PUFA and Hcy were examined using partial correlations, controlling for age, BMI and dietary protein. For any variables that did not meet the assumptions of normality for partial correlations, a syntax was used to produce a non-parametric partial correlation. This method consisted of using the NONPAR CORR and /MATRIX OUT commands to produce a Spearman Rho matrix, which could then be read by the /MATRIX IN and PARTIAL CORR command whilst factoring in the appropriate covariates. For all correlation analysis, a sample size of forty-six was required to achieve a power of 0·80 for a correlation coefficient of 0·40 and an α of 0·05(Reference Algina and Olejnik29). Evidence suggests a non-linear relationship between Hcy and health of older adults(Reference Wong, Almeida and McCaul30); therefore, non-parametric correlation analysis was performed. National Adult Reading Test score was included as a covariate in preliminary analysis, but was excluded in final models.
Baseline and 24-week results from the diet and physical activity assessments were compared within groups, using paired t tests. A general linear model was used to compare the active intervention groups v. the placebo over time (from pre- to post-measurement) on changes on the dependent variables on an intention-to-treat basis. Effect size calculation (η 2 (eta squared)) was performed. Demographic and health information, such as age, and BMI were included as covariates in the analysis if they were significantly correlated with the dependent variable. The Benjamini–Hochberg procedure was used to decrease the false discovery rate and probability of type I errors, with a Q value of <0·25 accepted as significant, and all P values are expressed as raw values(Reference Benjamini and Hochberg31). Linear regression we used to identify whether a difference in whole-blood DHA was associated with a change in outcome score. All results are expressed as mean values and standard deviations. Pre-trial registration plans dictated that a 2 × 2 ANOVA would be conducted to examine treatment effects; however, due to the potential interaction between the supplementation and exercise, independence of these variables could not be guaranteed; thus, examining treatment effects based on the assigned groups was determined to be a more robust method. Correlation analysis was used to identify whether a difference in whole-blood DHA was associated with a change in outcome score. All results are expressed as mean values and standard deviations.
Results
Participant flow is shown in Fig. 1. The study had three separate intakes of participants, starting in March 2017, May 2017 and February 2018, with data collection taking place between March 2017 and January 2019. In total, sixty participants were allocated to intervention groups and fifty-one completed the study. Analyses were conducted on all who completed the study with no exclusions irrespective of compliance or protocol violations. Participants who withdrew during the study were invited to attend the follow-up assessment; however, the invitations were declined. Role limitations due to physical health problems, role limitations due to emotional health problems and social functioning from the short-form thirty-six questionnaire showed clear signs of ceiling effects; therefore, these were omitted from analysis. Hcy data were only available for forty-eight baseline values. Upon inspection, four results of the baseline whole-blood DHA levels were identified as unreliable and were subsequently excluded from the analysis.
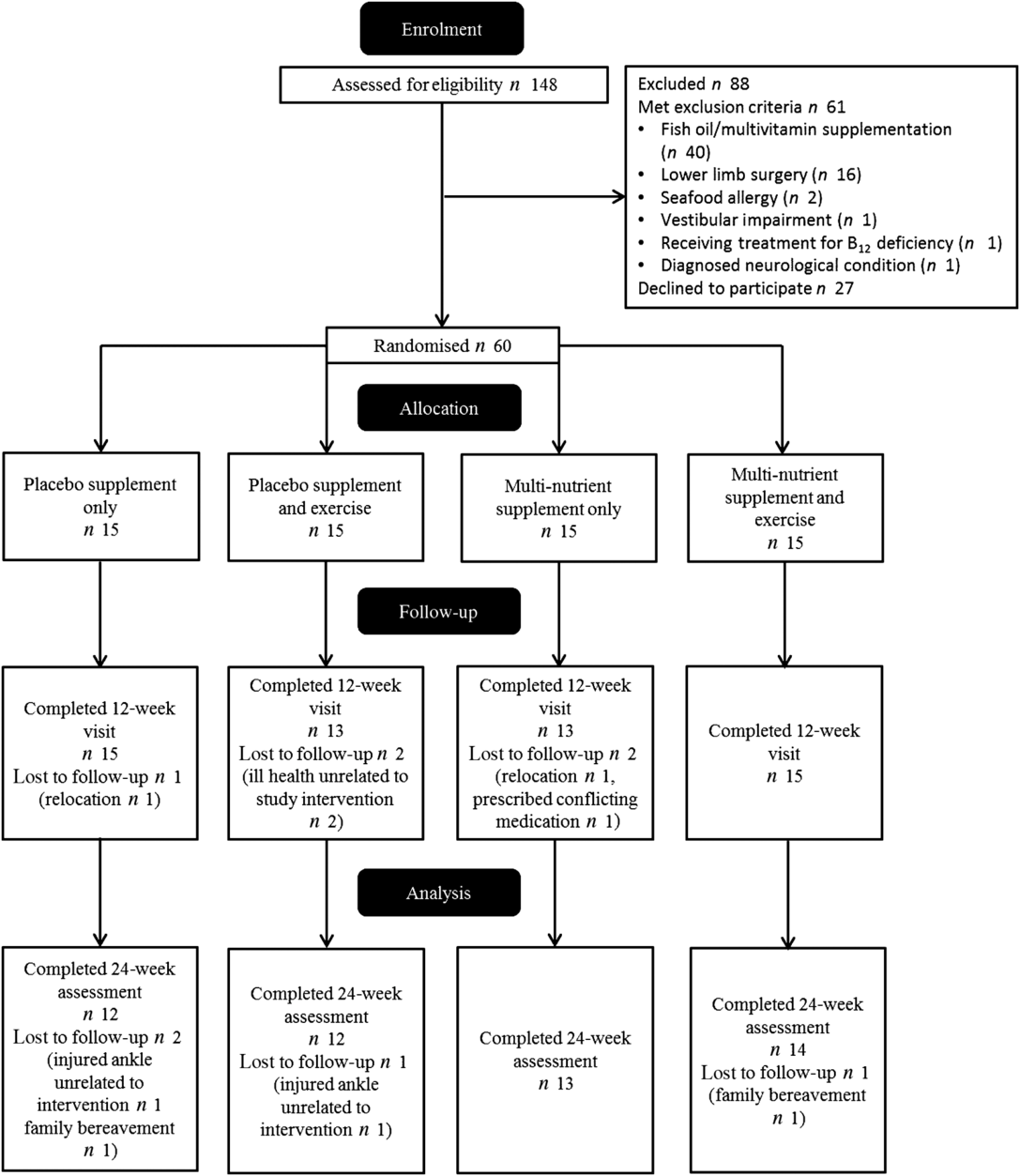
Fig. 1. Participant flow through study.
The group baseline characteristics are shown in Table 1. There were no reported adverse effects in any group over the study. The multi-nutrient supplementation led to significant increases in DHA and EPA compared with baseline levels in the MS (28 and 190 %, respectively) and MS + EX (43 and 140 %, respectively) groups and significant decreases in the n-6 PUFA, arachidonic acid levels of –28 and –26 %, respectively, all P < 0·05, Table 2. Compliance to the supplementation measured via changes in DHA and was 91 % for MS + EX, and 80 % for MS, 100 % for P + EX and 100 % for P, which was corroborated by capsule counting. Compliance to the exercise intervention was 55 % for MS + EX and 56 % for P + EX, which is consistent with other exercise intervention trials in a similar demographic(Reference Nagamatsu, Chan and Davis22). There were no significant changes in reported energy, macronutrient or n-3 PUFA intake between baseline and follow-up, Table 2. Participants maintained their current use of medications, and there were no reported changes in health status throughout the duration of the study with the exception of two participants in the MS + EX who discontinued their use of analgesic and anti-inflammatory medications.
Table 2. Participant compliance (Mean values and standard deviations)
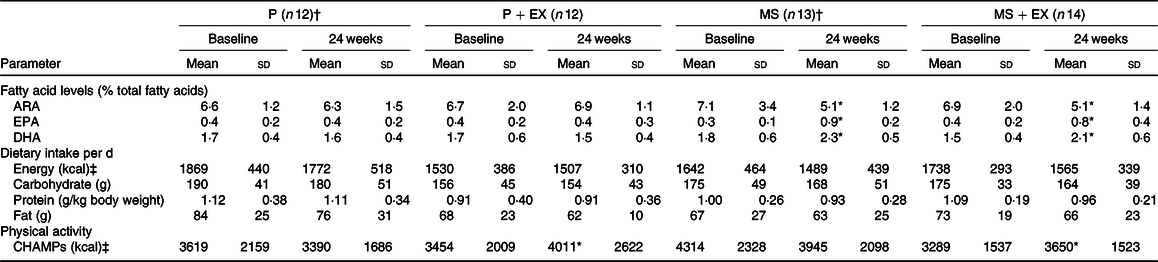
P, placebo supplement only; P + EX, placebo supplement and exercise; MS, multi-nutrient supplement only; MS + EX, multi-nutrient supplement and exercise; ARA, arachidonic acid; CHAMPS, Community Health Activities Program for Seniors.
* Significant change v. baseline, assessed using paired t test or Wilcoxon signed-rank test where appropriate.
† n 10 for whole-blood fatty acid value.
‡ To convert kcal to kJ, multiply the nutritional energy value by 4·184.
Primary outcome measure
There was a non-significant decrease of 0·8 % in the mean habitual walking speed by the P group over the study, whereas the other groups increased their mean walking speed by 0·05 m/s (0·07 m/s) (4·0 %) for the MS group, 0·03 m/s (0·09 m/s) (2·5 %) for the P + EX group and 0·01 m/s (0·12 m/s) (0·8 %) for the MS + EX group. However, these changes were not statistically significant for supplementation (P = 0·25), exercise (P = 0·50) or for the combined intervention (P = 0·79), Fig. 2.

Fig. 2. Effects of multi-nutrient supplement and exercise on the primary outcome of habitual gait speed. Values are means, with standard deviations represented by vertical bars. There were no significant effects for any intervention on changes in gait speed. , Placebo supplement only;
, placebo supplement and exercise;
, multi-nutrient supplement only;
, multi-nutrient supplement and exercise.
Secondary outcome measures
The results for the effects of the interventions on mobility, cognition and health-related quality of life are summarised in Table 3. There was a significant effect on verbal memory compared with the P group, by the MS (F 1,46 = 7·59, P = 0·008, partial η 2 0·144), P + EX (F 1,46 = 7·70, P = 0·008, partial η 2 0·144) and MS + EX interventions (F 1,46 = 15·82, P < 0·001, partial η 2 0·256), all with large effect sizes (Fig. 3). Significant effects v. placebo were also observed for executive function for the MS (F 1,47 = 8·02, P = 0·007, partial η 2 0·146), P + EX (F 1,47 = 8·37, P = 0·006, partial η 2 0·151) and MS + EX groups (F 1,47 = 8·60, P = 0·005, partial η 2 0·155) all with large effect sizes (Fig. 4). The MS + EX group also reported significant improvements in emotional well-being, compared with the P group (F 1,47 = 8·07, P = 0·03, partial η 2 0·146), with a large effect size. No significant treatment effects were observed for the other outcomes.
Table 3. Secondary outcome measures(Mean values and standard deviations)

P, placebo supplement only; P + EX, placebo supplement and exercise; MS, multi-nutrient supplement only; MS + EX, multi-nutrient supplement and exercise; DT, dual task.

Fig. 3. Effects of multi-nutrient supplement and exercise by general linear model on Rey's auditory verbal learning test. Values are means, with standard deviations represented by vertical bars. Significant effects for multi-nutrient supplement (MS) (P = 0·008), placebo supplement and exercise (P + EX) (P = 0·008) and combination of multi-nutrient and exercise (MS + EX) (P < 0·001). See text for further detail. * Significant effect of the intervention on changes in verbal memory v. placebo. , Placebo;
, P + EX;
, MS;
, MS + EX.

Fig. 4. Effects of multi-nutrient supplement and exercise by general linear model on executive function. Values are means, with standard deviations represented by vertical bars. Significant effects for multi-nutrient supplement (MS) (P = 0·007), placebo supplement and exercise (P + EX) (P = 0·006) and combination of multi-nutrient and exercise (MS + EX) (P < 0·005). See text for further detail. * Significant effect of the intervention on changes in executive function v. placebo. , Placebo;
, P + EX;
, MS;
, MS + EX.
Correlations of outcomes with DHA status and serum homocysteine
Significant baseline relationships between DHA and DT gait speed r(53) 0·32, P = 0·018, bodily pain r(51) 0·35, P = 0·013 and emotional well-being r(51) 0·30, P = 0·032 from the short-form thirty-six questionnaire were identified. These relationships were moderate to weak in strength. There were no significant associations between DHA and any cognitive outcomes. Following the interventions, there was a direct relationship between changes in verbal memory and DHA levels, r(18) 0·66, P = 0·001, Fig. 5; however, no relationship was detected for changes in executive function. At baseline, there were no significant associations between serum Hcy and any of the outcome measures (online Supplementary Table S2).

Fig. 5. Scatter plot for changes in DHA and change in verbal memory for multi-nutrient supplement (MS) and placebo (P) groups, r(18) 0·66, P = 0·001. , MS;
, P.
Discussion
The MOBILE study explores for the first time the effects of a high-DHA multi-nutrient supplement and aerobic exercise on mobility and cognition in older women. Although the supplementation led to significant increases in DHA levels, there were no significant improvements in the primary outcome measure of habitual walking speed. However, significant improvements in verbal memory and executive function were seen following the interventions, with the improvements in verbal memory in direct relation to increases in whole-blood DHA levels. Furthermore, lower circulating DHA was associated with poorer DT performance and measures of pain and emotional well-being at baseline.
Significant improvements in habitual walking speed were previously shown by us following treatment with the same multi-nutrient supplement, reflecting clinically relevant improvements within a similar sample size(Reference Strike, Carlisle and Gibson4). These improvements were driven by both improvements in the treatment group and decreases in the placebo group. However, in the present study, although there were increases following the multi-nutrient supplementation of a similar magnitude to our previous study, the decline in the placebo group was much less than predicted, with a mean decline of 0·8 %, as opposed to 2 % in our previous study(Reference Alcock, Vanicek and O’Brien32), which may reflect a high-functioning nature of the participants. Indeed, the lack of improvement following the exercise intervention supports this hypothesis, as aerobic exercise has consistently been shown to improve walking speed(Reference Lee and Cho33,Reference Henderson, Leng and Chmelo34) . Interestingly, the effect size for the combined supplementation and exercise group was modest in comparison with each intervention on their own; however, this may be partly explained by the aforementioned participants who discontinued daily use of analgesic and anti-inflammatory for joint pain who both observed small declines in gait speed of 0·01 m/s.
The individual and combined interventions led to improvements in verbal memory, with the total recall across the trials representing eight to twelve more words remembered than the placebo group. These findings corroborate previous observations showing positive effects on verbal memory following treatment with the same multi-nutrient supplement(Reference Strike, Carlisle and Gibson4). Similarly, the improvements following exercise are consistent with previous research(Reference Smith, Blumenthal and Hoffman7). The individual and combined interventions also resulted in significantly improved executive function. The observation of exercise improving this cognitive domain is consistent with the previous experimental work(Reference Smith, Blumenthal and Hoffman7). There is currently no clear consensus in the literature as to whether the factors in the multi-nutrient supplement improve executive function in older adults. For example, 900 mg DHA for 24 weeks had no effect on executive function(Reference Yurko-Mauro, McCarthy and Rom35), whereas 1320 mg EPA and 880 mg DHA for 26 weeks produced significant improvements(Reference Witte, Kerti and Hermannstädter36). Similarly, although B vitamins(Reference Clarke, Bennett and Parish37) and G. biloba (Reference Snitz, O'Meara and Carlson38) do not appear to improve executive function, preliminary evidence suggests phosphatidylserine has some beneficial effects(Reference Richter, Herzog and Lifshitz39). A recent study suggested an n-3 PUFA multi-nutrient supplement combined with exercise improves executive function in older men(Reference Bell, Fang and Snijders12); however, as there was no exercise-only control group, the contribution of n-3 PUFA multi-nutrient supplement to these effects cannot be identified. Indeed our analysis found no significant relationship between changes in blood level of DHA and the executive function suggesting that other factors may have contributed towards the change in this outcome.
Due to the small sample size, analysis to detect superiority of the active interventions over one another was not possible. It may be hypothesised that the effects of the multi-nutrient supplement and exercise operate via overlapping biological mechanisms. This includes decreasing inflammation, for example, IL-6 levels are linked to declines in verbal memory(Reference Beydoun, Weiss and Obhi40), and both n-3 PUFA and aerobic exercise have been shown to decrease IL-6 levels(Reference Zheng, Qiu and Xia41). Alternatively, exercise and factors in the multi-nutrient supplement, such as DHA and G. biloba, increase brain-derived neurotrophic factor levels, which may contribute to enhancing synaptic plasticity and cognitive function(Reference Janssen and Kiliaan42–Reference Chieffi, Messina and Villano44). However, further work is needed to explore these potential mechanisms, if they mediate any of the observed effects and if indeed the combination of the two interventions can provide an additional benefit to the older adult.
Due to the range of compounds in the multi-nutrient supplementation, it is not possible to ascribe the effects to any single factor and the present results further support the use of a combination of dietary factors in ageing(Reference Strike, Carlisle and Gibson4,Reference Dunn-Lewis, Kraemer and Kupchak45) . Unfortunately, due to methodological issues, we were unable to monitor changes in serum Hcy levels over the study and so cannot identify the potential role of folic acid and vitamin B12 in the treatment effects. However, a major unifying factor in the multi-nutrient intervention is DHA, and the supplementation increased circulating DHA and EPA levels. To identify the potential contribution of DHA to the treatment effects, correlations between changes in DHA and changes in outcome measures were performed. The increases in DHA levels over the study were in direct relation with improvements in verbal memory, suggesting increasing DHA was an important contributor to the treatment effects on this outcome. At baseline, significant relationships were seen with DHA and DT gait speed, although no other measures of mobility or cognition. Previous observations suggest circulating DHA levels correlate with gait and mobility outcomes(Reference Strike, Carlisle and Gibson4,Reference Hutchins-Wiese, Kleppinger and Annis46) , although erythrocyte DHA and EPA were not shown to be associated with a slower decline in gait speed over 3 years in a fully adjusted model(Reference Fougère, Goisser and Cantet47). The results from the present study do provide some further support for DHA status as a potential blood biomarker for monitoring physical performance in ageing, although further work is needed to establish this link more clearly.
The strengths of this study are that it was a randomised, placebo-controlled semi-blinded design, with high retention rate, thereby increasing the reliability of the data. The study used a range of detailed and sensitive mobility and cognitive tests to assess treatment effects. It is important in this type of study to monitor participant's dietary intake and physical activity; these were assessed by diet diary and FFQ and Community Health Activities Program for Seniors questionnaire, respectively. This meant it could be confirmed that the participants’ diet and physical activity outside of the interventions remained consistent. The only notable, although not statistically significant, change in diet was a decrease in fat intake across all four groups; however, we have no reason to believe this decrease across the groups would have influenced the results of the analysis. Furthermore, the trial design was reviewed and made publicly available on a clinical trials registry and was subject to peer review during the protocol publication process. Additionally, blood fatty acid levels were taken at the start and end of the study, to monitor absorption and incorporation of the n-3 PUFA. Limitations were the low recruitment and high functional ability of the participants. A further limitation was that ApoE genotype was not assessed, as this may affect participant's response to the supplementation(Reference Hennebelle, Plourde and Chouinard-Watkins48) and is associated with gait speed decline in ageing(Reference Verghese, Holtzer and Wang49).
Conclusion
Overall, these results suggest that the multi-nutrient supplement produces similar improvements in verbal memory and executive function to aerobic exercise, offering the intriguing prospect that supplementation may be able to mitigate some of the effects of low physical activity on cognitive function in the elderly. These improvements are clinically relevant and were identified in able female adults. Treatment effects were not identified in the primary outcome; nonetheless, the improvements in verbal memory and executive function do provide some promising insight into the benefits of dietary supplementation and exercise for the promotion of healthy ageing. The study identified for the first time relationships between DHA and DT ability. Further work should seek to explore the effects of the supplement on participants who may be most likely to respond, that is, those with low DHA and high Hcy levels, and also explore supplementation for a longer period, or in a more frail population.
Acknowledgements
The authors wish to express their thanks to all the participants. The authors would also like to thank Tom Wainwright of the Orthopaedic Research Institute, Bournemouth University, for helpful conversations aiding in development of the exercise programme, and Isabell Nessel for overseeing some of the laboratory work.
This work was supported by Bournemouth University and grants from Efamol Ltd and Sylvia Waddilove Foundation Trust.
S. C. D. developed the research question. S. C. D., A. J. and F. T. developed the study design. P. F. developed the measurements of the study and S. C. D. and F. T. acted as methodological council. S. C. D., F. T. and A. J. edited and revised the study protocol. P. F. was responsible for the final content of the paper, and all authors have read and approved the final manuscript.
The authors declare that there are no conflicts of interest.
Ethical approval was granted by Bournemouth University Science Technology and Health research ethics panel (Ethics ID 10788) and the study conformed to the declaration of Helsinki and guidelines for Good Clinical Practice.
Supplementary material
For supplementary material referred to in this article, please visit https://doi.org/10.1017/S0007114520000719