In the last decades, researchers started to understand that the gut microbiota is a key player affecting human health, being an essential key in human health and disease(Reference Wang, Yao and Lv1). The focus shifted on understanding what constitutes a health- (eubiotic) or disease-promoting (dysbiotic) microbial community(Reference Wang, Yao and Lv1). Emerging evidence shows that alterations in the intestinal microbial balance could lead to dysbiosis and play a pathological role, contributing to the development of non-communicable diseases such as obesity, the metabolic syndrome (MetS), inflammatory bowel disease, irritable bowel syndrome, colorectal cancer, type 1 diabetes, rheumatoid arthritis, allergies, autism and major depressive disorders(Reference Wang, Yao and Lv1–Reference Hobbs, Williams and Hillerich6).
The human intestinal microbiota was shown to play a role as a vital regulator of basic human biological processes such as modulating the metabolic phenotype, extracting nutrients and energy from our diets, programming host immune system and regulating epithelial development(Reference Actis7). These core functions are linked to the production of essential and extremely diverse metabolites such as vitamins (vitamin B12, folic acid or vitamin K), bile acids, neurotransmitters (serotonin, dopamine, acetylcholine) and SCFA (acetic acid, propionic acid and butyric acid). These metabolites result from undigested fibre fermentation by the gut microbiota(Reference Fernandes, Su and Rahat-Rozenbloom8,Reference Ramakrishna9) .
More than 90 % of the entire population of the human gut microbiota are represented by two phyla, Firmicutes (which includes mainly Clostridium, Enterococcus, Lactobacillus and Faecalibacterium genera) and Bacteroidetes (which includes notably Bacteroides and Prevotella genera)(Reference Gomes, Bueno and de Souza5,Reference Senghor, Sokhna and Ruimy10) .
Preliminary studies showed that some microbial species from the gut ecosystem had been linked to specific dietary habits. To illustrate, strong research efforts initiated by projects such as the European Metagenomics of the Human Intestinal Tract (MetaHIT) (http://www.metahit.eu) project, the American Human Microbiome Project (HMP) (http://hmpdacc.org) or the Asian Microbiome Project (http://www.asiangut.com/) had emphasised a higher diversity of the gut microbes of rural and preagricultural or isolated populations compared with westernised or industrialised populations.
In this regard, observational studies on these populations highlighted that these communities are characterised by an abundance of Prevotella species within the gut, whereas westernised populations harbour higher levels of Bacteroides than Prevotella (Reference Conlon and Bird11–Reference De Filippo, Di Paola and Ramazzotti20).
To date, limited information is available linking microbiota or specific microbial species like the Prevotella genus to health markers; however, several studies have established a correlation between microbial taxa and disease(Reference Kobyliak, Virchenko and Falalyeyeva3,Reference Liu, Wu and Chen4,Reference Actis7) . Regarding Prevotella, studies focused on the associations between the diverse species, genome and habitats with dietary patterns, health and disease. Characterisation of the healthy human microbiota by using next-generation sequencing techniques has revealed a prevalence of Prevotella species at mucosal sites, within the respiratory system, oral and gut ecosystem. Evidence revealed beneficial effects of some Prevotella strains in the gut such as not only improving CVD risk factor profile and glucose metabolism(Reference Mancabelli, Milani and Lugli21,Reference Wang, Ames and Tun22) , but also pathobiontic properties of some strains which promoted diseases like the MetS, obesity, inflammatory bowel disease or other inflammatory diseases (rheumatoid arthritis, asthma, bacterial vaginitis, HIV infection)(Reference Larsen23).
The necessity of larger cohort studies in order to establish a disease-triggering role of Prevotella species is highlighted, since inflammatory diseases are multifactorial and Prevotella is also considered beneficial due to its abundance in healthy gut microbiota and association with plant-rich diets(Reference Geva-Zatorsky, Sefik and Kua24–Reference Zhu, Sunagawa and Mende26).
Therefore, the selection of the Prevotella genus from the gut as the main subject for this systematic review lies in its significance as commensal of the human gut and its characteristic as dietary fibre fermenter. We decided to further examine eligible studies that focus on unravelling the association between dietary habits, food products and the presence of Prevotella species and their metabolite signature as well as the relevance of this bacterial genus in health status.
Experimental methods
Literature search
We conducted our literature review in accordance with the Preferred Reporting Items for Systematic Reviews and Meta-Analyses (PRISMA) statement(Reference Moher, Liberati and Tetzlaff27). The literature search was conducted independently by both authors, with disagreements resolved by consensus.
We searched the scientific electronic databases PubMed, Web of Science Core Collection (WoS CC) and Google Scholar for potentially eligible articles. We developed a systematic search strategy, which included the following descriptors from PubMed: ‘diet’, ‘gut microbiota’, ‘Prevotella’ and ‘health’. In addition, we considered free-text terms like ‘dietary pattern’, ‘eating behaviour’, ‘plant-based diet’, ‘dietary fibre’, ‘gastrointestinal microbiome’, ‘health status’ and ‘disease’. The logical connectives ‘and’, ‘or’ and ‘and not’ were systematically used to combine descriptors and terms used to trace the publications. For the WoS CC database and Google Scholar, we adapted our final PubMed search strategy. In Table 1, we provide the exact search strategies of the literature databases. We also explored for additional articles by checking the references cited in the primary eligible studies included in this systematic review. The final search on 4 November 2018 resulted in 274 hits (Fig. 1) from the databases after excluding 9811 duplicates identified using Endnote (Thomson Reuters). An initial screening of the titles and abstracts of articles was then performed to exclude irrelevant studies. During the systematic evaluation, study data were reviewed, where possible, in relation to dietary patterns and levels of Prevotella, changes in the intestinal microbiota, the role of Prevotella in the gut system, the metabolite signature and any reported metabolic consequence of the Prevotella genus in rodents and human studies. Then, the full texts of potentially eligible studies were reviewed before definitive inclusion.
Table 1. Detailed search strategies for PubMed, Web of Science Core Collection (WoS CC) and Google Scholar
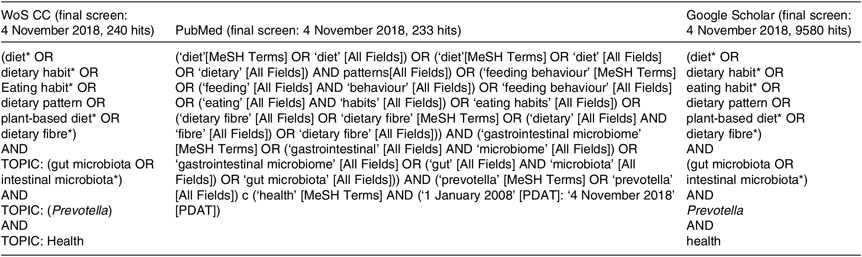
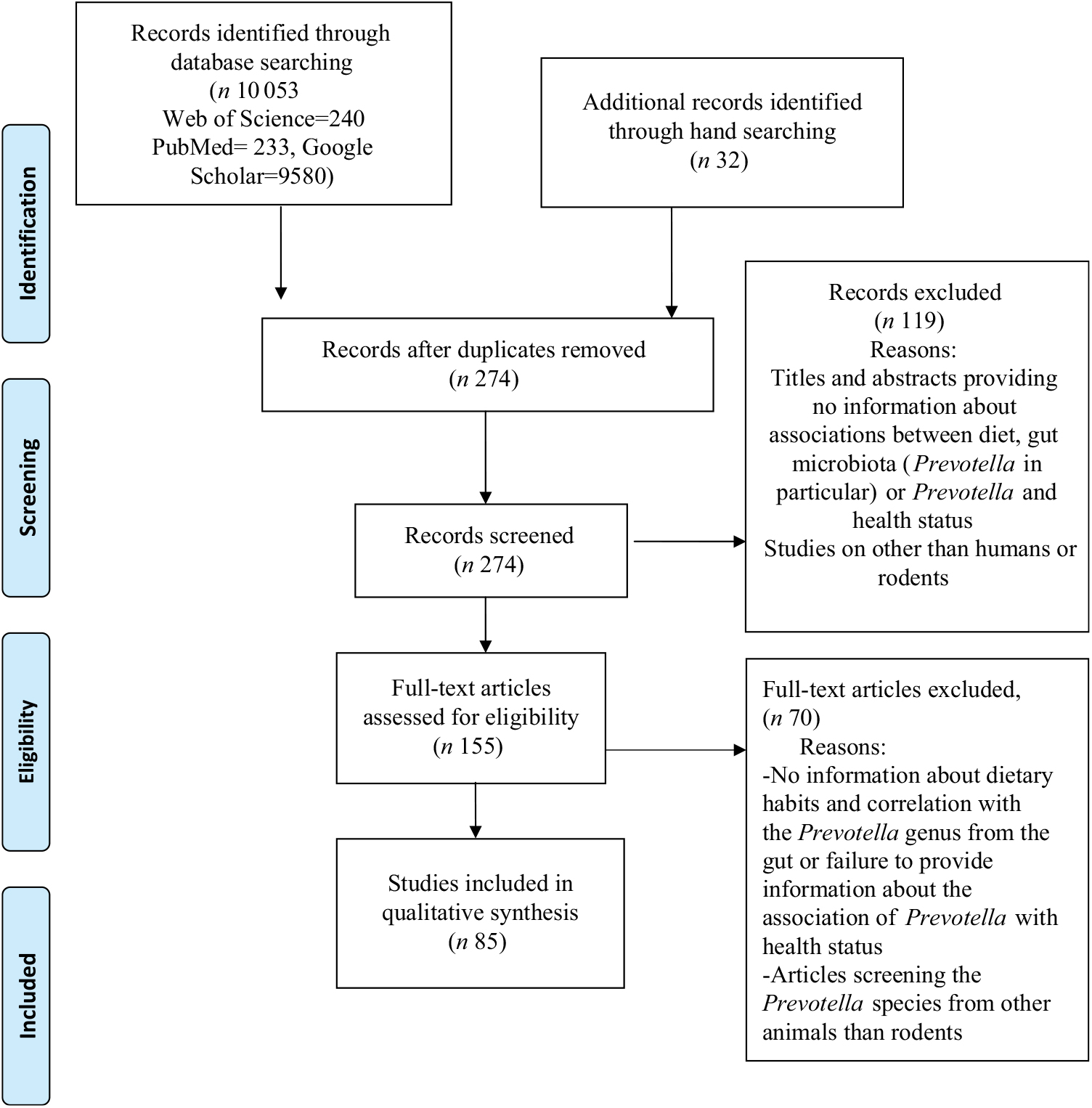
Fig. 1. Preferred Reporting Items for Systematic Reviews and Meta-Analyses (PRISMA) flow diagram of the study selection process.
Study selection
Table 2 provides an overview of the a priori defined inclusion and exclusion criteria that we applied to select eligible articles.
Table 2. Inclusion and exclusion criteria for the systematic review
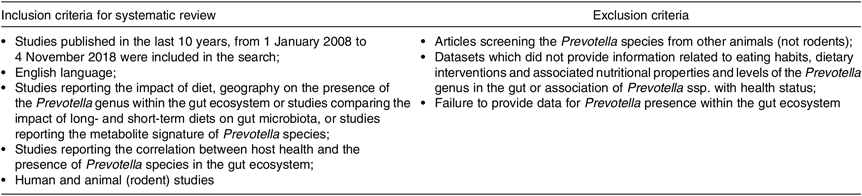
We (G. P. and D.-C. V.) independently screened all 274 abstracts. By applying inclusion/exclusion criteria to the information contained in the abstract, we reduced the pool of potentially eligible articles to 155 (Fig. 1). We evaluated the retrieved full-text articles applying the same inclusion and exclusion criteria that were used for the abstract selection. Any disagreements during the selection process were discussed among all the reviewers and unit consensus was reached. Finally, we included eighty-five full-text articles into our review, after we excluded seventy articles that did not meet the inclusion criteria.
Review statistics
A total of eighty-five studies extracted from 155 articles met the inclusion criteria and were analysed in the systematic review. The detailed steps of the systematic review article selection process are given as a flow chart in Fig. 1.
Risk of bias within studies
The risk of bias was unclear in all trials. Lack of information precluded a proper evaluation of the risk of bias for all studies.
Results
We identified sixty-eight articles and seventeen literature reviews published between 2008 and 2018 that have investigated the impact of different dietary habits on gut microbial communities, in particular on Prevotella levels or Prevotella/Bacteroides ratio. Moreover, the influence of urbanisation, industrialisation and geographical or ethnic factors on shaping the gut ecosystem as well as the role of gut microbiota in different diseases were evaluated (online Supplementary File S1)(Reference Fernandes, Su and Rahat-Rozenbloom8,Reference Martínez, Stegen and Maldonado-Gómez12–Reference Nakayama, Watanabe and Jiang16,Reference Gomez, Petrzelkova and Burns18,Reference De Filippo, Di Paola and Ramazzotti20,Reference Wang, Ames and Tun22,Reference Wu, Chen and Hoffmann29–Reference Shankar, Gouda and Moncivaiz34,Reference Ferrocino, Di Cagno and De Angelis38,Reference Gutiérrez-Díaz, Fernández-Navarro and Sánchez43–Reference Ruengsomwong, Korenori and Sakamoto45,Reference Matijašić, Obermajer and Lipoglavšek47,Reference Chen, Long and Zhang55,Reference Hu, Park and Jang62,Reference Pedersen, Gudmundsdottir and Nielsen63,Reference Haro, Garcia-Carpintero and Alcala-Diaz66–Reference Mejía-León, Petrosino and Ajami68,Reference Hjorth, Roager and Larsen70–Reference Kelly, Bazzano and Ajami74,Reference Prieto, Hidalgo and Segarra79,Reference Kang, Zhang and Zhu82–Reference De Filippo, Cavalieri and Di Paola114) .
The studies described significant differences in the gut microbial ecosystem, influenced by dietary habits, lifestyle, geography and environmental factors, by using molecular techniques such as 16S rDNA pyrosequencing and shotgun metagenomic data(Reference Senghor, Sokhna and Ruimy10–Reference Martínez, Stegen and Maldonado-Gómez12). Most of the gut bacteria (approximately 60 %) cannot be cultured due to their particular requirements for anaerobiosis and nutritional needs. Therefore, the development of these techniques provided new knowledge on the composition and functionality of the gut microbiome as an ecosystem and its variation across the world(Reference Senghor, Sokhna and Ruimy10).
Dietary patterns shape the gut microbiota composition
Specifically, studies on the human microbiome have suggested that long-term dietary habits, geographical and ethnic factors could shape the gut microbiota into the so-called ‘enterotypes’ that were not specific to certain continents or nations(Reference Martínez, Stegen and Maldonado-Gómez12,Reference Mancabelli, Milani and Lugli21) . The ‘enterotypes’ hypothesis was introduced in 2011 by Arumugam et al. as a useful method to stratify human gut microbiomes(Reference Arumugam, Raes and Pelletier28). Wu et al. used shotgun sequencing of the faecal samples from American individuals and used multiple clustering techniques, but found only two of the three original clusters: Bacteroides and Prevotella (Reference Wu, Chen and Hoffmann29). The third cluster was not distinct in their data, with Ruminococcus (Clostridiales order) equally abundant in both of the first two enterotypes. They compared their findings with a previously published study on Italian and rural African children, wherein Italian children had Bacteroides-dominated gut microbiomes and African children had Prevotella-dominated gut microbiomes(Reference Wu, Chen and Hoffmann29). Although consensus that these enterotypes are the result of long-term dietary behaviour is usually accepted, the concept itself has also been challenged, since other studies have proposed the existence of microbial community gradients rather than distinct enterotypes(Reference Costea, Hildebrand and Manimozhiyan30). Studies highlighted afterwards only two community types: one dominated by Prevotella and one dominated by Bacteroides or members of the Firmicutes Bifidobacterium (Reference Zhu, Sunagawa and Mende26).
De Filippo et al. (Reference De Filippo, Di Paola and Ramazzotti20) reported a considerable difference in gut microbiota at a phylum level, namely Firmicutes/Bacteroidetes ratio, between African children who followed a low-fat high-fibre diet and Italian children who consumed a high-fat and high-protein modern diet. The African children were living in a village of rural Burkina Faso, in an environment close to that of Neolithic subsistence farmers, while the Italian children were living in Florence, in an environment typical of industrialised Europe. The African children showed a significant enrichment in Bacteroidetes and depletion in Firmicutes (P < 0⋅001) with abundance of Prevotella and Xylanibacter bacteria and more SCFA (P < 0⋅001) compared with European children. The authors assumed that the polysaccharide-rich diets of African people shaped their gut microbiota in a way that helped to maximise energy intake from fibre while also protecting them from inflammation and non-infectious colonic diseases(Reference De Filippo, Di Paola and Ramazzotti20).
Several other observational and cohort studies underlined the abundance of Prevotella species in Indian(Reference Dehingia, Talukdar and Talukdar31), Chinese, Kazaks(Reference Li, Fu and Yang32), Turks, Moroccans(Reference Deschasaux, Bouter and Prodan33), Indonesian, Thai(Reference Martínez, Stegen and Maldonado-Gómez12), Filipinos(Reference Nakayama, Yamamoto and Palermo-Conde14), African (Malawian, Egyptian(Reference Shankar, Gouda and Moncivaiz34), Tanzanian and BaAka hunter–gatherers(Reference Schnorr, Candela and Rampelli15,Reference Gomez, Petrzelkova and Burns18) ) and Venezuelan rural or isolated populations(Reference Yatsunenko, Rey and Manary35), whereas Bacteroides were abundant in populations from western countries in Europe and America(Reference Conlon and Bird11–Reference Nakayama, Yamamoto and Palermo-Conde14,Reference Dubois, Girard and Lapointe19,Reference Wu, Chen and Hoffmann29,Reference Shankar, Gouda and Moncivaiz34) . The abundance of the Prevotella genus in these populations is determined by its capacity to digest complex carbohydrates and by the genetic and enzymatic potential to break down cellulose and xylan from foods(Reference Dubois, Girard and Lapointe19). A specific strain, Prevotella copri, has been shown to be an important biomarker for diet(Reference De Filippis, Pellegrini and Vannini13,Reference Gorvitovskaia, Holmes and Huse36) . Nakayama et al. have underlined in a study on Filipino children that the growth of P. copri and P. stercorea were stimulated by vitamin A and β-carotene from bananas and mangos(Reference Nakayama, Yamamoto and Palermo-Conde14). The authors also outlined that not only these two species of Prevotellaceae, but also species of Veillonellaceae (Dialister succinatiphilus) and Erysipelotrichaceae have a positive correlation with dietary carbohydrate. A high intake of fat positively correlated with Bacteroides, Ruminococcus, Blautia, Dorea, Megamonas and negatively correlated with Prevotella (Reference Nakayama, Yamamoto and Palermo-Conde14,Reference Dehingia, Talukdar and Talukdar31) . Moreover, Li et al. showed in their study on healthy Chinese herdsmen that Prevotella gradually decreased with the degree of urbanisation, while Bacteroides, Faecalibacterium, Blautia, Collinsella, Ruminococcus, Coprococcus and Dorea increased with the degree of urbanisation(Reference Li, Fu and Yang32). In addition, Mancabelli et al. highlighted in their meta-analysis of publicly available shotgun datasets of human faecal samples collected from urbanised and traditional preagricultural populations that industrialisation has shaped the gut microbiota through the acquisition and/or loss of specific gut microbes, thereby potentially impacting on the overall functionality of the gut microbiome(Reference Mancabelli, Milani and Lugli21). For example, Xylanibacter (Bacteroidetes) and Treponema (Spirochaetes) were only found in individuals from agricultural communities, which indicate that they could be symbionts lost in urban-industrialised societies(Reference Nakayama, Yamamoto and Palermo-Conde14,Reference Dubois, Girard and Lapointe19) . Enrichment in Succinivibrio and Treponema, which also possess a high-fibre degrading potential, is important for agrarian populations like the Africans, as the typical diet is high in fibre and complex carbohydrates(Reference Nakayama, Yamamoto and Palermo-Conde14).
Overall, people living in African countries have higher gut microbiota diversity dominated by Actinobacteria (Bifidobacterium); Bacteroidetes (Bacteroides–Prevotella); Firmicutes (C. histolyticum, Eubacterium, Oscillibacter, Butyricicoccus and Sporobacter); Proteobacteria (Succinivibrio, Shigella and Escherichia) and Spirochaetes (Treponema) and depleted in only Actinobacteria (Bifidobacterium catenulatum), Firmicutes (Clostridium difficile and Akkermansia muciniphila). Meanwhile, for people living in Western countries such as in Europe and America, gut microbiota is enriched in Firmicutes (Blautia, Dorea, Roseburia, Faecalibacterium, Ruminococcus, Oscillospira, C. perfringens, C. difficile and S. aureus); Bacteroidetes (Bacteroides), Actinobacteria (B. adolescentis and B. catenulatum) and Verrucomicrobia (A. muciniphila)(Reference De Filippo, Di Paola and Ramazzotti20,Reference Mancabelli, Milani and Lugli21) .
Association of dietary habits with microbial and metabolic profiles and the link with health or disease states
The distinct dietary habits of populations living in different geographical areas are reflected in their microbial diversity and metabolic profile. To exemplify, Western diet is characterised by high consumption of animal proteins and fat (meat, fish, dairy products), refined carbohydrates (processed foods, sweets) and low levels of fibre intake from plants(Reference Ramakrishna9,Reference Requena, Martínez-Cuesta and Peláez37) . In contrast, Eastern diet consists of staple foods such as rice or noodles, soup and several dishes with a lot of vegetables. Populations living in isolated areas (like preagricultural populations) have a diet based on foods gathered and/or hunted from their proximate environment rich in carbohydrates and fibre(Reference Nakayama, Yamamoto and Palermo-Conde14,Reference De Filippo, Di Paola and Ramazzotti20) .
Even though Prevotella has been linked with a vegetable-rich diet and Bacteroides with protein/fat rich diets, little is known about the composition of the gut microbiota in people with specific dietary patterns like vegetarian, vegan, Mediterranean or omnivorous(Reference De Filippis, Pellegrini and Vannini13).
In the last 10 years, few studies have reported the differences in faecal microbiota of individuals following a vegetarian, vegan or omnivorous diet, but studies on large cohorts are missing. Vegetarian, ovo-lacto vegetarian, lacto-vegetarian or vegan diets have increased in popularity lately, as studies reported beneficial effects on human health in terms of prevention of CVD, cancer and diabetes(Reference Ferrocino, Di Cagno and De Angelis38). The Mediterranean diet is characterised by a high intake of vegetables and fruits, legumes and whole grains combined with a moderately high consumption of fish, low intake of saturated fat, meat and dairy products and regular but moderate consumption of red wine and the use of olive oil as the principal source of dietary lipids(Reference Lopez-Legarrea, Fuller and Zulet39). This type of diet has also been linked to protective roles against several diseases like obesity, type 2 diabetes, inflammatory diseases and CVD(Reference Lopez-Legarrea, Fuller and Zulet39–Reference Santoro, Pini and Scurti41). On the contrary, the omnivore diet which is typical for Western diets from industrialised countries apparently led to a composition of the microbiota that is more associated with different types of diseases like obesity, insulin resistance, dyslipidemia and inflammatory disorders(Reference Albenberg and Wu42).
Most studies reported that vegetarians harbour higher numbers of Prevotella species as well as a high Prevotella/Bacteroides ratio compared with non-vegetarians (omnivores)(Reference Ferrocino, Di Cagno and De Angelis38,Reference Gutiérrez-Díaz, Fernández-Navarro and Sánchez43) . However, one study by Wu et al. (Reference Wu, Compher and Chen44) reported that levels of Prevotella were not significantly different between omnivores and vegans from the USA, as the residence in a westernised culture might determine a more ‘restrictive’ microbiota structure. Ferrocino et al. showed in a cross-sectional study that vegans and vegetarians have an abundance of Prevotellaceae and ovo-lacto vegetarians were characterised by the presence of P. micans, B. vulgatus and Faecalibacterium prausnitzii. P. copri was also found in a characteristic bacteria of the omnivore subjects, which is consistent with its presence in non-agrarian diets(Reference Ferrocino, Di Cagno and De Angelis38,Reference Ruengsomwong, Korenori and Sakamoto45) . B. vulgatus is associated with agrarian diets, being well known for its ability to encode the largest number of enzymes which target the degradation of pectin(Reference Hobbs, Williams and Hillerich6,Reference Egshatyan, Kashtanova and Popenko46) . F. prausnitzii was found to be a characteristic species in the faecal samples of the subjects who followed a vegetarian diet(Reference Matijašić, Obermajer and Lipoglavšek47), and it has recently been shown that it has the probiotic ability to produce vitamin B12 and to hydrolyse lactulose and galacto-oligosaccharides(Reference Cecchini, Laville and Laguerre48). F. prausnitzii has also been recognised as being one of the most abundant butyrate producers in human faeces(Reference Cecchini, Laville and Laguerre48).
Moreover, it has frequently been reported that SCFA (acetate, propionate and butyrate) are produced by intestinal microbiota during the fermentation of undigested polysaccharides. In contrast, non-digested proteins are broken down into smaller peptides or amino acids, in the end resulting in branched-chain fatty acids (valerate, caproate), phenolic compounds, amines and ammonia(Reference Portune, Beaumont and Davila49). It has been demonstrated that these microbial metabolites help controlling the colon pH, regulating energy control and prevent pathogen bacteria growth as well as modulating bacterial gene expression leading to the production of enzymes involved in amino acid metabolism(Reference Actis7,Reference Neis, Dejong and Rensen50) .
Evidence shows that SCFA levels were higher in native Africans on agrarian diet compared with African Americans or European children (Italian)(Reference Nakayama, Yamamoto and Palermo-Conde14,Reference Dubois, Girard and Lapointe19) . Propionate was positively correlated with the presence of Prevotella in African children and negatively correlated with Bifidobacterium, Blautia and Lachnospiraceae(Reference Nakayama, Yamamoto and Palermo-Conde14). Specifically, propionate is formed mostly through the succinate pathway that is characteristic of Bacteroidetes(Reference Reichardt, Duncan and Young51) and has been shown to be metabolised in the liver. Its implications in reducing serum cholesterol and decreasing hepatic lipogenesis, thus preventing weight gain in overweight adult humans, was demonstrated(Reference Chambers, Viardot and Psichas52).
Butyrate was enriched in European children and positively correlated with Bifidobacterium, Bacteroides, Blautia, Dorea and Faecalibacterium (Reference De Filippis, Pellegrini and Vannini13). Butyrate is frequently produced via butyryl-CoA:acetate CoA-transferase with consumption of acetate and is mainly characteristic of the Firmicutes phylum. Butyrate promotes barrier function and reduces inflammation when present in sufficient quantity(Reference Den Besten, van Eunen and Groen53). It becomes the major fuel source for colonic epithelial cells, reducing the need for energy allocation to these cells from the host(Reference Den Besten, van Eunen and Groen53,Reference Tremaroli and Backhed54) . In addition, Chen et al. (Reference Chen, Long and Zhang55) analysed the fibre utilisation capacities of Prevotella or Bacteroides by testing fibres (arabinoxylans from sorghum bran, arabinoxylans from maize bran and fructo-oligosaccharides) in an in vitro fermentation system. The authors highlighted that through the fermentation of the mentioned substrates, Prevotella produced two to three times more propionate than the Bacteroides-dominated microbiota. Conversely, lower levels of butyrate were obtained(Reference Chen, Long and Zhang55). Acetate is a fermentation product for most gut anaerobes and consistently reaches the highest concentrations among the SCFA in the colon. Approximately, 70 % of the acetate is used as an energy source by the liver, but is also used as a substrate for the synthesis of cholesterol and long-chain fatty acids(Reference den Besten, van Eunen and Groen56). Moreover, it was revealed to act as an appetite regulator and thus aid in reducing obesity(Reference Frost, Sleeth and Sahuri-Arisoylu57).
Thus, the amount and relative abundance of SCFA may be considered as biomarkers of a healthy status(Reference Martínez, Stegen and Maldonado-Gómez12).
De Filippis et al. showed that vegetarians and vegans had a high-level adherence to the Mediterranean diet, which positively associated to Prevotella and increased levels of SCFA (acetic, propanoic or butanoic acids) and negatively correlated to urinary trimethylamine N-oxide (TMAO) levels(Reference De Filippis, Pellegrini and Vannini13). In contrast, consumption of proteins and fat in omnivorous diets positively associated to higher levels of branched-chain fatty acids (valerate, caproate) and urinary TMAO(Reference De Filippis, Pellegrini and Vannini13). TMAO is a metabolite which has been recently shown to increase the risk of cardiovascular and atherosclerosis in both mice and humans independently of traditional cardiometabolic risk factors and through inhibiting hepatic bile acid synthesis(Reference Zhu, Gregory and Org58,Reference Schugar, Shih and Warrier59) . TMAO results from carnitine and choline found in animal products (eggs, beef, pork and fish), which are converted by the gut microbiota to trimethylamine, oxidised in the liver and released into circulation as TMAO(Reference Zhu, Gregory and Org58). In addition, the content of choline and l-carnitine in the traditional Mediterranean diet is more than 50 % lower than in a typical Western diet(Reference Martínez, Stegen and Maldonado-Gómez12).
Interestingly, Chen et al. showed in a study on mice that TMAO levels were decreased by resveratrol, a natural polyphenol found in grapes, berries and wine, which attenuated TMAO-induced atherosclerosis(Reference Chen, Yi and Zhang60). The authors further explained that resveratrol increased hepatic bile neosynthesis via gut microbiota remodelling (increased levels of Lactobacillus, Bifidobacterium, Akkermansia, but decreased levels of Prevotella)(Reference Chen, Yi and Zhang60).
Thus, while it is still unknown what constitutes a healthy gut microbiome, some studies have linked a microbial dysbiosis to some disease states. High levels of Prevotella were linked to obesity(Reference Moreno-Indias, Sánchez-Alcoholado and García-Fuentes61) and significantly associated with BMI(Reference Hu, Park and Jang62), insulin resistance in non-diabetic people(Reference Moreno-Indias, Sánchez-Alcoholado and García-Fuentes61,Reference Pedersen, Gudmundsdottir and Nielsen63) , hypertension(Reference Li, Zhao and Wang64) and non-alcoholic fatty liver disease (NAFLD)(Reference Michail, Lin and Frey65). Furthermore, Prevotella were also linked to high blood pressure and impaired glucose metabolism(Reference Egshatyan, Kashtanova and Popenko46). However, other studies did not find correlations between obesity(Reference De Filippis, Pellegrini and Vannini13,Reference Haro, Garcia-Carpintero and Alcala-Diaz66,Reference Borgo, Verduci and Riva67) , type 1 or type 2 diabetes(Reference Mejía-León, Petrosino and Ajami68,Reference Forslund, Hildebrand and Nielsen69) and lower levels of Prevotella. In fact, Hjorth et al. reported that subjects with increased waist circumference and high Prevotella/Bacteroides ratio could easier lose body fat on diets high in fibre and whole grain than subjects with a low Prevotella/Bacteroides ratio(Reference Hjorth, Roager and Larsen70). In addition, Prevotella and Bacteroides levels were decreased at baseline in MetS subjects and increased after an intervention with Mediterranean diet and low-fat diet. In contrast, no significant microbiota changes after the dietary intervention were observed in the group of obese subjects without the MetS(Reference Haro, Garcia-Carpintero and Alcala-Diaz66). Furthermore, studies in mice indicate that Prevotella can determine features of the MetS. Colonisation of germ-free mice with a Prevotella-rich microbiota from patients with hypertension induced higher blood pressure compared with mice receiving microbiota from a normotensive donor(Reference Li, Zhao and Wang64).
P. copri colonisation in mice on a high-fat diet promoted increased insulin resistance(Reference Pedersen, Gudmundsdottir and Nielsen63). Furthermore, Kovatcheva-Datchary et al. (Reference Kovatcheva-Datchary, Nilsson and Akrami71) showed that after a dietary intervention with barley, kernel-based bread (BKB), blood glucose and serum insulin responses improved compared with the group that received white wheat bread. Moreover, they compared the faecal microbiota of individuals who showed the least or no improvement in glucose or insulin responses (non-responders) to those who showed the most pronounced improvement (responders). They observed an abundance of Bacteroidetes (Prevotella) and high P/B ratio after BKB intervention in responders, but not in non-responders. Dorea and Roseburia initially increased at baseline but decreased in responders after BKB intervention. Regarding the SCFA profile, no significant differences were observed between the two groups. To investigate the effect of Bacteroides and Prevotella species on glucose metabolism, germ-free mice were colonised with human faeces-derived Bacteroides thetaiotaomicron and P. copri for 14 d and fed a standard chow diet. The levels of Prevotella were higher in mice colonised with responder versus non-responder donor microbiota, which suggested that Prevotella may contribute to the BKB-induced improvement in glucose metabolism(Reference Kovatcheva-Datchary, Nilsson and Akrami71). Similarly, De Vadder et al. (Reference De Vadder, Kovatcheva-Datchary and Zitoun72) showed that mice colonised with the succinate producer P. copri exhibited metabolic benefits, which could be related to succinate-activated intestinal gluconeogenesis (IGN). They concluded that the activation of IGN is positively correlated with Prevotella, which could in fact account for the beneficial metabolic effects such as improved glucose metabolism, by increased glucose tolerance(Reference De Vadder, Kovatcheva-Datchary and Zitoun72).
Likewise, Vitaglione et al. showed that after an intervention with whole grain products, levels of Prevotella increased and a significant positive correlation was identified between SCFA and the abundance of Bacteroidetes in obese subjects(Reference Vitaglione, Mennella and Ferracane73).
Furthermore, levels of Prevotella, Bacteroides and Dorea were favourably correlated with an improved CVD risk factor profile, such as BMI, waist circumference, triglyceride levels and blood pressure, in a randomised crossover study design with 3 g of high molecular weight β-glucan for 5 weeks(Reference Wang, Ames and Tun22). In contrast, Kelly et al. highlighted recently that some species of Prevotella (Alloprevotella, Paraprevotella, Prevotella 7) were associated with CVD risk(Reference Kelly, Bazzano and Ajami74).
Moreover, Henao-Meija et al. elucidated the role of Prevotella in non-alcoholic fatty liver disease, which is a hepatic manifestation of the MetS and has a leading role in triggering chronic liver disease in the Western world. They outlined that a Prevotella-rich dysbiosis was finally associated with pathology of the liver(Reference Henao-Mejia, Elinav and Jin75).
Discussion
The objective of this qualitative systematic review was to evaluate the available data revealing the modulation of the Prevotella genus from the gut ecosystem by different dietary habits as well as its implications as a potential biomarker for homeostasis or disease state through its metabolite signature. Moreover, the association with other bacterial species like Bacteroides and its role as a dietary fibre fermenter in the gut were also assessed.
The results of this review indicate that some Prevotella strains such as P. copri or P. stercorea were associated with plant-based diets, rich in polysaccharides and fibres, typical for rural communities, whereas the Bacteroides genus was correlated with a high-fat, high-protein modern diet, typical in Western countries. Moreover, P. copri was highlighted as an important biomarker for diet and lifestyle, due to its abundance in different populations across the world(Reference De Filippis, Pellegrini and Vannini13,Reference Gorvitovskaia, Holmes and Huse36) . The abundance of Prevotella species were shown in many populations with fibre-rich diets, due to its potential to digest and breakdown complex carbohydrates from foods(Reference Graf, Di Cagno and Fåk76). Therefore, observational studies outlined high levels of Prevotella species in Indian(Reference Dehingia, Talukdar and Talukdar31), Chinese, Kazaks(Reference Li, Fu and Yang32), Turks, Moroccans(Reference Deschasaux, Bouter and Prodan33), Indonesian, Thai(Reference Martínez, Stegen and Maldonado-Gómez12), Filipinos(Reference Nakayama, Yamamoto and Palermo-Conde14), African (Malawian, Egyptian(Reference Shankar, Gouda and Moncivaiz34), Tanzanian and BaAka hunter–gatherers(Reference Schnorr, Candela and Rampelli15,Reference Gomez, Petrzelkova and Burns18) ) and Venezuelan rural or isolated populations(Reference Yatsunenko, Rey and Manary35), whereas Bacteroides were abundant in populations from Western countries in Europe and America(Reference Conlon and Bird11–Reference Nakayama, Yamamoto and Palermo-Conde14,Reference Dubois, Girard and Lapointe19,Reference Wu, Chen and Hoffmann29,Reference Shankar, Gouda and Moncivaiz34) . In addition, vegetarian, vegan and Mediterranean diets were also linked with high levels of Prevotella and a high Prevotella/Bacteroides ratio(Reference Martínez, Stegen and Maldonado-Gómez12).
Evidence reported that these diets might have beneficial effects on human health, in terms of protecting against several diseases such as obesity, type 2 diabetes, inflammatory diseases and CVD(Reference Lopez-Legarrea, Fuller and Zulet39,Reference Santoro, Pini and Scurti41) . These diets were positively correlated with increased levels of SCFA, which are produced by the intestinal microbiota during fermentation of undigested polysaccharides and have well-documented beneficial roles(Reference Gargari, Taverniti and Balzaretti77,Reference Shortt, Hasselwander and Meynier78) . Prevotella species were shown to be positively associated with propionate production that has important roles in preventing weight gain by reducing serum cholesterol and decreasing hepatic lipogenesis(Reference Chambers, Viardot and Psichas52).
Prevotella strains are classically considered commensal bacteria due to their extensive presence in the healthy human body and their rare involvement in infections. However, emerging studies have linked increased Prevotella abundance and specific strains to inflammatory disorders, suggesting that at least some strains exhibit pathobiontic properties(Reference Larsen23).
Several limitations should be considered when interpreting the results of this study. First, assessing the way dietary habits or specific nutrients like dietary fibre impact the gut microbial composition could lead to different bias, as the bacterial communities have a great diversity and the interactions between specific nutrients and commensal bacteria from the gut are still not very well known. Moreover, the great inter-individual variability between different populations, the lack of in-depth metagenomic studies and small sample sizes for interventional studies could also increase the risk of bias.
A review from 2017 underlined the interaction between P. copri and the immune system, revealing pathobiontic properties such as releasing inflammatory mediators from immune and stromal cells and promoting inflammatory diseases (periodontitis, rheumatoid arthritis, asthma, bacterial vaginosis, HIV infection, the MetS and inflammatory bowel disease)(Reference Larsen23). At the same time, inflammatory diseases are highly heterogeneous and the complex interactions between host genetic risk factors and environmental exposures are also important.
Some studies positively correlated Prevotella levels with obesity, type 2 diabetes and NAFLD(Reference Michail, Lin and Frey65), while others did not find any correlations between these diseases and abundance of Prevotella (Reference Bibbò, Dore and Pes80,Reference Frost, Sleeth and Sahuri-Arisoylu81) . In addition, high levels of Prevotella were positively associated with glucose improvement in mice or improved CVD risk factor profile in humans(Reference De Filippis, Pellegrini and Vannini13,Reference Haro, Garcia-Carpintero and Alcala-Diaz66,Reference Borgo, Verduci and Riva67) .
Another recent review by Ley(Reference Ley25) analysed the possible beneficial and detrimental implications of Prevotella strains from the human gut on host health. It emphasises the importance of better understanding of Prevotella’s wide genetic diversity, ecology and interaction with other commensal bacteria from the gut, in order to be able to modulate its levels(Reference Ley25).
Prevotella may only play a part in certain disease endotypes, since they may exhibit different properties due to a high genetic diversity within and between species. These discrepancies in different studies may be due to the complex interrelatedness of the diseases, and additional investigations of immune mechanisms in metabolic disease are needed in humans. Thus, there is a need for more studies in humans to ascertain a causal and potential disease-triggering role for Prevotella (Reference Larsen23).
Conclusion
As Prevotella is a genus with high genetic diversity within and between species, they could explain its abundance in human healthy microbiota and only certain strains may exhibit pathobiontic properties. Nevertheless, there is a need for more studies in humans to ascertain a causal and potential disease-triggering role for Prevotella and more in-depth metagenomic studies are needed in order to reveal the health- or disease-modulating properties.
Acknowledgements
The authors thank Dr Vasile Coman for his constructive suggestions and linguistic review which contributed to the refinement of the manuscript.
This work was supported by a grant of Ministry of Research and Innovation, CNCS-UEFISCDI, project number PN-III-P1-1.1-TE-2016-0661, within PNCDI III.
G. P. and D. V. investigated the references. G. P. wrote the review and D. V. provided suggestions through critical reading of the manuscript and coordinated the writing process starting from the content on. All authors read and approved the final manuscript.
The authors declare that they have no competing interests.
Supplementary material
For supplementary material/s referred to in this article, please visit https://doi.org/10.1017/S0007114519000680