Ulcerative colitis (UC) and Crohn's disease (CD), collectively known as inflammatory bowel disease (IBD), are chronic diseases with unknown aetiologies. The clinical course is characterised by episodes of flare-up, i.e., patients are symptomatic and exhibit active intestinal inflammation and tissue injury, followed by periods of remission, i.e., patients are asymptomatic and display no active intestinal inflammation or tissue injury. There is evidence that the active inflammation experienced in IBD is, at least in part, due to an inappropriate and dysregulated immune response to luminal gut microbiota.
The luminal-associated microbiota move through the large bowel with peristaltic movements and appear in the faeces, while mucosa-associated microbiota are static and are present at the mucous interface between the epithelium and lumen(Reference Probert and Gibson1). Harper et al. (Reference Harper, Lee and Kettlewell2) demonstrated that a small bowel effluent (containing luminal bacteria) introduced into the defunctioned colon of patients with CD treated by loop ileostomy was far more pro-inflammatory than a (sterile) ultrafiltrate of the effluent. Additionally, diversion of the intestinal luminal contents into the surgically excluded ileum of patients with CD induced inflammation in previously unaffected regions(Reference D'Haens, Geboes and Peeters3). Thus, gut microbiota appear to play a key pathogenic role in both initiation and clinical course of IBD.
The luminal microbiota community can be simplistically divided into helpful/beneficial and harmful bacteria. ‘Helpful’ bacteria inhibit the growth of harmful bacteria, beneficially stimulate the immune system, induce production of SCFA, aid in digestion and absorption of nutrients and synthesise vitamins(Reference Gibson and Roberfroid4). ‘Harmful’ bacteria induce protein purification, inhibit production of SCFA and promote growth of injurious bacteria or production of their pro-inflammatory and injurious products. Thus, factors that enhance the establishment of helpful bacteria in the gut lumen, while inhibiting the harmful ones, may prevent initiation and/or progression of the intestinal inflammatory cascade experienced in IBD patients during disease flare-up and, therefore, induce and maintain remission.
Maintenance of a healthy gut microbiota relies on indigestible carbohydrates or dietary fibre. Under adequate dietary fibre intake, gut microbes receive a sufficient supply of carbohydrate to fulfil energy needs. During saccharolytic fermentation, bacteria produce SCFA (e.g., acetate, propionate and butyrate) and gases (e.g. CO2 and hydrogen) and are able to proliferate. SCFA help maintain a (relatively) low colonic pH, preventing the growth of potentially harmful bacteria(Reference Cummings, Christie and Cole5). Acetate and propionate can be absorbed into the blood stream, and influence glucose and lipid metabolism(Reference Scheppach6), while butyrate is utilised almost completely by colonic epithelial cells and represents their major source of energy(Reference Cummings and Englyst7). With respect to IBD, butyrate is of particular importance because it additionally acts as an anti-inflammatory agent(Reference Saemann, Bohmig and Osterreicher8). Therefore, it appears that adequate dietary fibre intake is important for maintaining healthy gut microbiota community and for the prevention or alleviation of colonic inflammation.
It is important to note, however, that not all dietary fibres are created equal. Most dietary fibres that can be utilised by bacteria (i.e., fermentable fibres) are rapidly degraded at the terminal ileum and caecum, leaving very little carbohydrate substrate for both luminal and especially mucosal bacteria in more distal regions of the colon(Reference Cummings and Macfarlane9). This results in a decrease in SCFA (and thus an increase in pH) and an increase in undesirable, even toxic compounds, such as phenol and NH3 in distal regions of the colon(Reference Macfarlane, Gibson and Cummings10), resulting in a less healthy colonic environment. Thus, intake of slowly fermentable fibres may help increase beneficial fermentative products in the distal colon and improve colonic health.
We have developed a novel source of dietary fibre, termed starch-entrapped microspheres, wherein electrostatically cross-linked alginate forms a web-like structure that is filled with starch(Reference Hamaker, Venkatachalam and Zhang11). The alginate thus creates a physical barrier that impedes digestion such that over 90 % of the starch in uncooked starch-entrapped microspheres remains intact after simulated upper-gastrointestinal digestion (i.e. mouth, stomach and small intestine) using an in vitro procedure(Reference Rose, Keshavarzian and Patterson12). Furthermore, this product has shown potential as a slowly fermentable fibre with high production of butyrate and the ability to change the colonic microbiota in vitro (Reference Rose, Keshavarzian and Patterson12). Thus, the purpose of this research was to test the hypothesis that slow fermenting starch-entrapped microspheres would produce more favourable fermentation profiles and microbial shifts than a rapidly fermenting fibre, fructo-oligosaccharides (FOS), during in vitro fermentation with the faecal microbiota obtained from patients with IBD.
Experimental methods
Fermentation substrates
Starch-entrapped microspheres were made by dissolving 0·2 kg of sodium alginate in 8·8 kg water. One kilogram of waxy maize starch was then added, and the suspension was mixed until homogenous. The slurry was then pumped through an atomiser into 20 l of stirred 4 % (w/v) calcium chloride dihydrate. The microspheres were then harvested by filtration. After extensive washing, the microspheres were mixed with ethanol and filtered again. The ethanol was necessary to prevent the microspheres from sticking together. The microspheres were allowed to dry in air until the odour of ethanol could not be detected (about 4 h) and then moved to a forced draft oven at 45°C to finish drying (about 24 h).
Total starch was determined using a colorimetric method (Total Starch (α-amylase/amyloglucosidase method), Megazyme, Wicklow, Ireland) after disruption of the alginate matrix with a ball mill (Brinkman, Westbury, NY, USA). Moisture content was measured by loss in weight after drying in a forced draft oven at 105°C for 18 h. Short-chain FOS was obtained from Encore Technologies (Plymouth, MN, USA). As per the manufacturer's spec sheet, the product contained 94 % FOS, 0–4 % glucose and 4–8 % sucrose.
Faeces
Fresh stools were collected from six patients with inactive CD and six patients with active UC, who had not received antibiotics within the last 4 weeks. CD and UC patients were selected from the IBD Clinic at Rush University Medical Center (Chicago, IL, USA). The diagnosis of UC and CD diseases was made based on established clinical, endoscopic and histological criteria. Patients with inactive CD had a CD activity index of < 150, and had no diarrhoea, abdominal pain or bleeding. Patients with active UC had rectal bleeding, diarrhoea (liquid stool and stool frequency >3/d) and friable and ulcerated rectosigmoid mucosa on their sigmoidoscopy at the time of stool collection. All the patients were taking daily 5-aminosalicylic acid, but none were taking prednisone at the time of stool collection. The healthy controls consisted of faculty and staff of the university who were not involved in the study. Faeces were collected in gas-tight bags containing Anaerocult C (to maintain anaerobosis; Merck, Darmstadt, Germany), frozen in liquid nitrogen and then stored at − 80°C until used for fermentation experiments (approximately 1 month).
To validate our use of frozen stool in fermentation experiments, we analysed a fresh microbiota and the same microbiota stored at − 80°C. Microbial composition of the fresh and frozen microbiotas was checked periodically over 44 weeks of storage using denaturing gradient electrophoresis as described previously(Reference Venema, Van Nuenen and Smeets-Peeters13). Activity of the fresh and frozen microbiotas (8 d, − 80°C) was assessed by measuring SCFA production during in vitro fermentation as described below, except the total fermentation time was 196 h, and the microbiota were maintained on the standard ileal efflux medium during the entire experiment rather than switching to the test carbohydrates after 16 h.
Fermentation
Faeces were defrosted in an anaerobic cabinet, and samples from six individuals in each of the three categories were combined to yield a microbiota representative of that group. The amounts used from each individual were not equal due to differing amounts of stool collected (Table 1). Pooling was important to reduce the possibility of anomalous results(Reference Christl, Katzenmaier and Hylla14) due to the highly variable nature of gut microbiota among individuals(Reference McBurney and Thompson15). The faeces were homogenised with nine volumes of diluted standard ileal efflux medium (modified from(Reference Gibson, Cummings and Macfarlane16); Table 2). Ninety millilitres of this homogenate were used to inoculate the Toegepast Natuurwetenschappelijk Onderzoek in vitro model of the large intestine: the Toegepast Natuurwetenschappelijk Onderzoek gastro-intestinal model-2 (TIM-2) system (TNO, Zeist, The Netherlands)(Reference Minekus, Smeets-Peeters and Bernalier17). This system has been validated against sudden death individuals(Reference Venema, Van Nuenen and Smeets-Peeters13) and recently investigated using molecular RNA technology and assessed as closely simulating the microbiota in the colon(Reference Kovatcheva-Datchary, Egert and Maathuis18).
Table 1 Amount of faecal material used from each donor
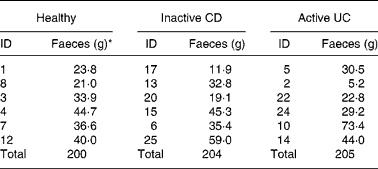
CD, Crohn's disease; UC, ulcerative colitis; ID, identification.
* Wet weight.
Table 2 Composition of standard ileal efflux medium (SIEM) and diluted SIEM per litre
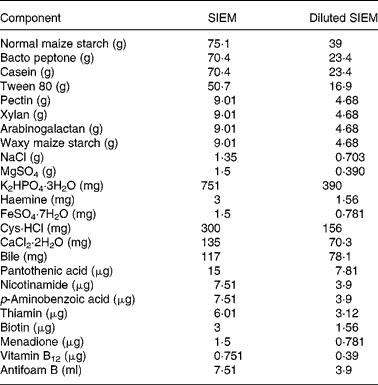
The TIM-2 system was allowed to equilibrate for 16 h after inoculation, during which time the microbiota (representing healthy, inactive CD or active UC) were fed a standard ileal efflux medium (Table 2) at a rate of 2 ml/h (containing 5 g of carbohydrate per 24 h). After equilibration, the feeding line was shut off, and the microbiotas were starved for 2 h. The test period (t = 0 h) was then begun by introducing the test carbohydrates (starch-entrapped microspheres or FOS) into the TIM-2 units. Carbohydrates were administered with a standard ileal efflux medium prepared without the carbohydrates listed in Table 2. FOS was dissolved in the medium, and starch-entrapped microspheres, due to their insoluble nature, were administered through a second syringe that was kept dry. Test carbohydrates were given at a rate of 10 g of fermentable carbohydrate per 24 h for 72 h.
The bacteria were constantly supplied with carbohydrate; therefore, this model more closely resembles the proximal rather than the distal colon. To approximate the distal colon more closely, during the last 4 h of fermentation (t = 68–72 h), the feeding line was shut off, and the pH was monitored to indicate whether the saccharolytic fermentation continued, or if the bacteria switched to putrefactive fermentation due to lack of carbohydrate. Samples were collected every 24 h for SCFA quantification, and microbial analyses were performed at the initial (t = 0 h) and final (t = 72 h) times. Fig. 1 shows a timeline of these events.
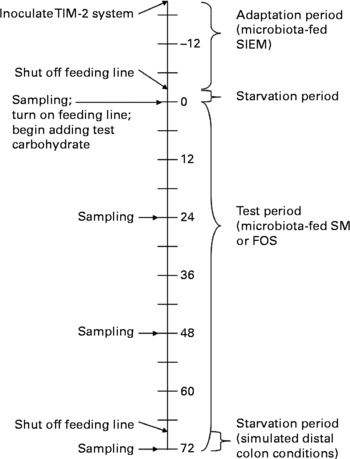
Fig. 1 Timeline for Toegepast Natuurwetenschappelijk Onderzoek gastro-intestinal model-2 (TIM-2) experiments; numbers represent hours; SIEM, standard ileal efflux medium; SM, starch-entrapped microspheres; FOS, fructo-oligosaccharides.
SCFA determination
For SCFA, lumen or dialysate samples were centrifuged (12 000 rpm, 5 min). A mixture of formic acid (20 %), methanol and 2-ethyl butyric acid (internal standard, 2 mg/ml in methanol) was added to the supernatant. A 0·5 μl sample was injected onto a GC column (Stabilwax-DA, length 15 m, inner diametre 0·53 mm, film thickness 0·1 mm; Varian Chrompack, Bergen op Zoom, The Netherlands) in a Chrompack CP9001 GC using an automatic sampler (Chrompack liquid sampler CP9050; Varian Chrompack)(Reference Jouany19).
Microbial analysis
Analysis of the microbiota with the intestinal-chip was performed at TNO (Zeist, The Netherlands), described by Schuren et al. (Reference Maathuis, Hoffman and Evans20). DNA was isolated from luminal samples at the start (t = 0 h) and at the end of the TIM-2 experiments (t = 72 h). Signals were quantified by calculating the mean of all pixel values of each spot and calculating the local background about each spot. Signal intensity was dependent on the position of the probe within the 16S rRNA gene; for that reason, concentrations of individual bacterial classes could not be assessed without setting up standard curves using quantitative PCR for each probe. However, signal intensities could be compared within probe to determine relative changes in abundance of the bacterial class represented by that particular probe(Reference Maathuis, Hoffman and Evans20). Because the primary aim was to determine the propensity of each test carbohydrate to alter individual classes of bacteria, fold differences between the signals at the initial and final times were useful for this determination. Standard deviations were relatively large among runs; therefore, each replicate was analysed twice.
Data analysis
Data were analysed with SAS software (version 9.1; SAS Institute, Cary, NC, USA) using a mixed model ANOVA (PROC MIXED). Main effects were microbiota source (1, healthy; 2, inactive CD; 3, active UC) and carbohydrate source (1, starch-entrapped microspheres; 2, FOS). For those analyses that included a time element (i.e., comparison between the initial and final times), a time effect (0 and 72 h) was also included. Fisher's least significant different test was used to determine significant differences among treatment combinations. All the treatment combinations were performed in quadruplicate, except when the active UC microbiota were fermented with FOS, which were performed in triplicate.
Ethical approval
The present study was conducted according to the guidelines laid down in the Declaration of Helsinki and all procedures involving human subject/patients were approved by the Institutional Review Board of Rush University Medical Center. Written informed consent was obtained from all the subjects/patients.
Results and discussion
Use of frozen microbiota
Fig. 2 shows that after 44 weeks of storage at − 80°C, the composition of the microbiota in terms of viable cells was not substantially affected, as evidenced by similar banding patterns on denaturing gradient electrophoresis gels. Furthermore, the stored, frozen samples still contained the microbial diversity of the fresh faecal sample, indicating that freezing had minimal influence on the microbiota composition.
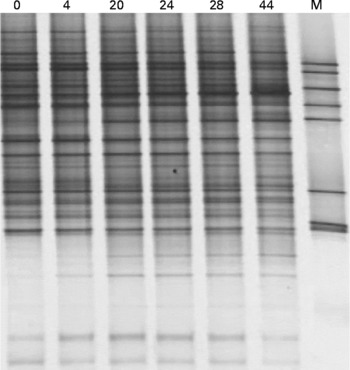
Fig. 2 Denaturing gradient gel electrophoresis profiles of a fresh faecal sample (0) and the same faecal sample after frozen storage ( − 80°C) for 4, 20, 24, 28 and 44 weeks. M, marker.
The production of SCFA during 196 h of fermentation was also measured using a fresh microbiota and the same microbiota that had been frozen at − 80°C for 8 d. On comparison, the fresh and frozen microbiotas showed similar SCFA production over at least 150 h of fermentation (Fig. 3). Most notably, during the first 72 h, which is the time period used in the present study, SCFA production was nearly identical. Moreover, the ratios of SCFA produced during fermentation (acetate:propionate:butyrate) were not significantly different for fresh and frozen microbiotas (data not shown).
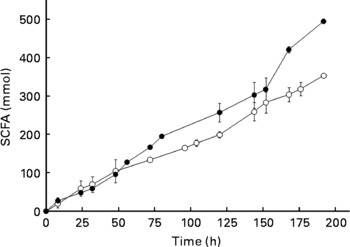
Fig. 3 Production of SCFA (acetate+propionate+butyrate) from a fresh microbiota (○) and the same microbiota after frozen storage (8 d, − 80°C; ●). Error bars show standard deviation; some error bars are too small to see; n>3.
Previously, others have reported using frozen microbiotas from animals(Reference Stanco, Di Meo and Piccolo21, Reference Hervas, Frutos and Giraldez22). For example, Hervas et al. (Reference Hervas, Frutos and Giraldez22) discovered that storage of rumen fluid at − 18°C for 24 h did not affect fermentation parameters when starch and cellulose were fermented; although average fermentation rate and the extent of polysaccharide degradation were affected, when barley straw neutral-detergent fibre and alfalfa hay were fermented. Stanco et al. (Reference Stanco, Di Meo and Piccolo21) showed that it was possible to use frozen inoculum from the caecum of rabbits (stored at − 18°C) for at least 3 months without affecting gas production, degradation of organic matter and SCFA production by the microbiota during in vitro fermentation. In a review aimed at examining factors that influence microbial activity of the rumen microbiota, Mould et al. (Reference Mould, Klien and Morgan23) concluded that during storage and sample transport, exposure to air should be excluded. For this reason, we maintained anaerobosis during storage and transport using Anaerocult C. These reports, together with the data provided in Figs. 2 and 3, provided sound evidence that the use of a frozen microbiota provides results that are comparable to a fresh microbiota for in vitro fermentation studies.
SCFA production
SCFA may be of particular importance to colonic health. They reduce colonic pH, thereby inhibiting the growth of some opportunistic, pathogenic bacteria(Reference Cummings, Christie and Cole5), decreasing the activity of co-carcinogenic enzymes such as glucuronidases, glycosidases and 7α-hydroxylases(Reference Goldin and Gorbach24, Reference Thornton25). Butyrate, one of the SCFA produced during fermentation, may be especially important in IBD. In vitro studies have revealed that butyrate suppresses the immuno-inflammatory response by inhibiting activation of the transcription factor NF-κB(Reference Inan, Rasoulpour and Yin26) and its downstream products like pro-inflammatory cytokines TNF and IL-12 as well as up-regulating the production of the anti-inflammatory cytokine IL-10(Reference Saemann, Bohmig and Osterreicher8). Patients treated with butyrate enemas have shown decreased inflammation due to a reduction in macrophages positive for activated NF-κB(Reference Luhrs, Gerke and Muller27).
Patients with IBD have not only shown decreased SCFA production(Reference Packey and Sartor28), they may also have impaired ability to harvest their beneficial effects. Higher concentrations of sulphate-reducing bacteria have been reported in patients with IBD compared with controls(Reference Loubinoux, Bronowicki and Pereira29). An increase in sulphate-reducing bacteria increases hydrogen sulphide production, which blocks colonocyte oxidation of butyrate(Reference Roediger, Duncan and Kapaniris30). Therefore, dietary fibres that can increase butyrate production by enhancing growth of butyrate producing bacteria, or fibres that can improve butyrate utilisation by inhibiting the growth of sulphate-reducing bacteria may be helpful in alleviating colonic inflammation.
When comparing among faecal microbiotas grown on starch-entrapped microspheres, the healthy microbiota produced more butyrate than microbiotas from IBD stools (Table 3), demonstrating impaired butyrate production by faecal microbiota in IBD patients. This was most pronounced in the inactive CD microbiota. In faecal microbiota grown on FOS, only the microbiota from patients with inactive CD showed significant inhibition. Of note is that starch-entrapped microspheres resulted in significantly higher butyrate production than FOS when fermented with the healthy and active UC faecal microbiotas.
Table 3 Butyrate and total SCFA production from healthy, inactive Crohn's disease (CD) and active ulcerative colitis (UC) microbiotas when administered starch-entrapped microspheres (SM) or fructo-oligosaccharides (FOS); values reported as mmol produced per amount of carbohydrate administered
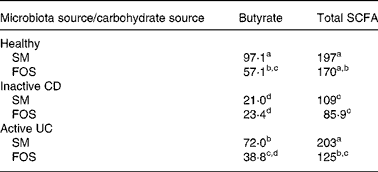
a,b,c,d Mean values within a column with unlike superscript letters were significantly different (P < 0·05).
Total SCFA production was similar when comparing between carbohydrate sources, except when the faecal microbiota from active UC were used (Table 3). In this case, starch-entrapped microspheres produced significantly more SCFA. Moreover, starch-entrapped microspheres were able to induce a total SCFA production in this diseased microbiota that were similar to the healthy faecal microbiota. The same was not true for FOS.
During the adaptation period (t = − 16–0 h), the faecal microbiota were fed a standard ileal efflux medium, which contained a mixture of carbohydrates that approximates the range of substrates that colonic bacteria may be exposed to under normal conditions(Reference Minekus, Smeets-Peeters and Bernalier17). Therefore, the molar ratios of SCFA produced during this time period could be compared to the ratios at the end of the test period (t = 72 h) to determine whether there was a shift in the molar ratios of SCFA produced. In particular, an increase in the molar proportion of butyrate produced would suggest an increase in butyrate producing bacteria, which may be beneficial due to the anti-inflammatory nature of butyrate.
In the healthy faecal microbiota, a significant increase in the proportion of butyrate produced was only experienced when the faecal microbiota were exposed to starch-entrapped microspheres (Fig. 4; with a concomitant decrease in acetate production, data not shown). The microbiota from patients with inactive CD showed a shift toward butyrate production with both starch-entrapped microspheres and FOS (with concomitant decrease in acetate production, data not shown), while that of active UC showed no significant shift toward butyrate production.
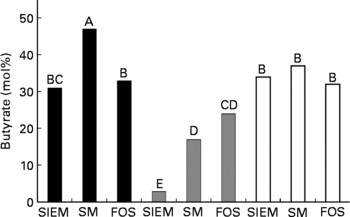
Fig. 4 Molar percentage of butyrate (of total SCFA) produced from healthy (■), inactive Crohn's disease () and active ulcerative colitis (□) microbiotas when administered a standard ileal efflux medium (SIEM, Table 2) during the adaptation period (t = − 16–0 h, Fig. 1) compared with starch-entrapped microspheres (SM) or fructo-oligosaccharides (FOS) during the test period (t = 0–72 h, Fig. 1); bars marked with the different capital letters are significantly different (P < 0·05).
Thus, in light of the trophic effects of SCFA, and in particular butyrate, on the colon, our data suggest that starch-entrapped microspheres may be more beneficial than FOS to promote gut health in healthy subjects and to promote anti-inflammatory milieu in the colon of patients with active UC.
Fermentation rate and pH changes
As mentioned, the TIM-2 system more closely approximates the proximal rather than the distal colon because the microbiotas are constantly supplied with test carbohydrates during fermentation. To approximate conditions that may occur in more distal regions of the colon, the feeding line of the TIM-2 system was shut off during the last 4 h of fermentation. Fig. 5 shows the changes in pH during the last 4 h of fermentation when starch-entrapped microspheres and FOS were inoculated with the faecal microbiota from healthy individuals or patients with IBD. SCFA from the saccharolytic fermentation cause a decrease in pH that is detected by the automatic titrator that prevents the pH from dropping below 5·8 by addition of sodium hydroxide. Therefore, a jagged line indicates the saccharolytic fermentation, and the more jagged the line (crests closer together), the more rapid the fermentation rate.
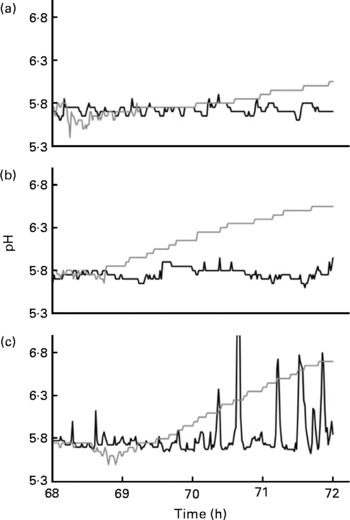
Fig. 5 Changes in pH during the last 4 h of fermentation (when the feeding line had been shut off) of faecal microbiotas from healthy individuals (a), patients with inactive Crohn's disease (b) or active ulcerative colitis (c) that had been previously fed starch-entrapped microspheres (—) or fructo-oligosaccharides ().
During the first hour after the feeding line was shut off (68–69 h), all the samples showed saccharolytic fermentation. The starch-entrapped microspheres clearly showed a less jagged line than FOS, indicating slower fermentation. The faecal microbiota from healthy subjects showed continued the saccharolytic fermentation after the feeding line was shut off (t = 69–72 h) when grown on starch-entrapped microspheres. Conversely, FOS showed a gradual increase in pH during this time period, which is indicative of a shift toward putrefactive fermentation, which results in NH3 production. This supports our previous study showing slow digestion and fermentation of the starch-entrapped microspheres(Reference Rose, Keshavarzian and Patterson12) and suggests this material could provide beneficial fermentable carbohydrates in the distal colon, whereas FOS would not.
As with the healthy microbiota, the microbiota from IBD patients showed continuous fermentation when fermented with starch-entrapped microspheres (Fig. 5). The large peaks for the active UC microbiota when fed starch-entrapped microspheres were due to inadequate mixing. For an unknown reason, when starch-entrapped microspheres were fermented with the active UC microbiota, the slurry became very thick and the mixing laboured. Although this situation was not ideal, this curve still did not show a gradual upward trend (as seen with FOS), indicating the occurrence of saccharolytic fermentation with the starch-entrapped microspheres.
In contrast to starch-entrapped microspheres, the microbiota from patients with IBD when incubated with FOS, showed more pronounced increases in pH during the final 4 h of fermentation compared with the stool from healthy individuals. This suggests that slowly fermentable dietary fibres, such as starch-entrapped microspheres, may be of particular importance in IBD due to their ability to maintain a low pH during prolonged fermentation.
Fermentation rate is important for the maintenance of gut health. As carbohydrates are fermented and their amount diminishes, putrefactive fermentation becomes significant, leading to an increase in pH due to lack of SCFA and production of NH3 (Reference Macfarlane, Gibson and Cummings10). The higher pH may allow the proliferation of undesirable bacteria(Reference Cummings, Christie and Cole5). The distal colon also contains higher concentrations of toxic metabolites(Reference Macfarlane, Gibson and Cummings10) due to fermentation of protein instead of carbohydrate. These undesirable conditions in the distal colon may exacerbate inflammation and explain the high rate of colonic neoplasia in left side of the colon. Thus, improving colonic milieu in the distal colon by delivering slow fermentable carbohydrate could be a beneficial intervention for promotion of gut health and prevention of colonic diseases like colonic polyps and cancer in healthy subjects as well as controlling colonic inflammation in inflammatory disorders of colon like UC. Our data provide compelling evidence that starch-entrapped microspheres are the ideal substrate to achieve this goal, although further in vivo, long-term studies in healthy subjects and patients with IBD are required to confirm these gut-health promoting effects of starch-entrapped microspheres.
Initial composition of the microbiotas from healthy individuals and patients with inactive Crohn's disease and active ulcerative colitis
Table 4 shows the initial (t = 0 h) fold differences among the diseased and healthy faecal microbiotas of some of the major genera of bacteria found in the large intestine(Reference Macfarlane, McBain, Gibson and Roberfroid31, Reference Conway, Mackie, White and Isaacson32). Therefore, changes in these classes of bacteria would likely represent a change in the gut metabolism as a whole. The microbiota from patients with inactive CD displayed far weaker signals for Bacteroides, Clostridium and Eubacterium compared with the healthy microbiota. In contrast, the faecal microbiota from patients with active UC displayed only slightly different signals for these three genera of bacteria compared with the faecal microbiota from healthy individuals. This suggests that microbiota from patients with active UC more closely resembled the healthy microbiota than did the inactive CD microbiota, as these three genera of bacteria represent the major proportion of the gut microbiota as a whole(Reference Vernazza, Rabiu, Gibson, Gibson and Rastall33). Additionally, these are the most important butyrate producers in the gut. The fact that these genera were so much higher in the healthy and active UC microbiota compared with inactive CD at least partially explains why butyrate production was so low in the inactive CD microbiota (Table 3).
Table 4 Fold differences in signal for select bacterial classes in the initial microbiota from patients with inactive Crohn's disease (CD) or active ulcerative colitis (UC) compared with the healthy microbiota; values reported as fold difference from healthy faecal microbiota, with negative numbers indicating a lower signal in the diseased stool and a positive number signifying a higher signal in the diseased stool
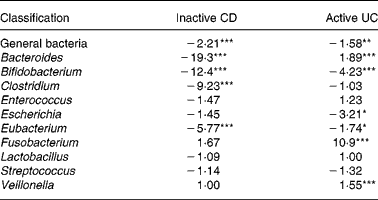
Mean values were significantly different from those of healthy microbiota: *P < 0·05, **P < 0·01, ***P < 0·001.
For the probiotic genera Lactobacillus and Bifidobacterium, no substantial differences were found in the concentrations of Lactobacillus among microbiotas, but the healthy microbiota had a far greater quantity of Bifidobacterium than the microbiota from active UC and inactive CD patients. These genera of bacteria are reported to be beneficial to gut health(Reference Gibson and Roberfroid4); and their lack may be related to the diseased condition.
When comparing the general bacterial signals, the microbiota from patients with inactive CD showed a 2-fold lower concentration than that of healthy individuals. However, signals from a number of the genus probes shown in Table 4 were substantially lower for the inactive CD microbiota compared with the healthy stool. In particular, the major genera, Bacteriodes, Clostridium and Eubacterium, were far more than 2-fold lower. This suggests that many of the bacteria in the inactive CD microbiota belonged to genera not represented in Table 4. This supports the findings of Seksik et al. (Reference Seksik, Rigottier-Gois and Gramet34), who demonstrated that a high percentage (30 %) of the dominant microbiota in CD belongs to unusual phylogenetic groups.
Changes in the faecal microbiota of healthy subjects during fermentation
After 72 h of fermentation, FOS induced a major increase in the concentration of Eubacterium in faecal microbiota from healthy subjects, whereas starch-entrapped microspheres did not (Table 5). While Eubacteria are important butyrate producers(Reference Duncan, Belenguer and Holtrop35), the significantly higher butyrate production from the healthy microbiota when administered starch-entrapped microspheres compared with FOS (Table 3) suggests that starch-entrapped microspheres must have increased the growth of other butyrate producing bacteria more than FOS increased the growth of Eubacterium.
Table 5 Fold changes in the signals of selected bacterial classes in the healthy, inactive Crohn's disease (CD) and active ulcerative colitis (UC) microbiotas after 72 h of fermentation with starch-entrapped microspheres (SM) or fructo-oligosaccharides (FOS); values reported as fold difference from the initial respective faecal microbiota, with negative numbers indicating a lower signal after fermentation, and positive numbers signifying a higher signal after fermentation
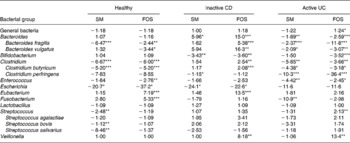
Mean values were significantly different from those of initial microbiota: *P < 0·05, **P < 0·01, ***P < 0·001.
Concentrations of Streptococci were decreased with starch-entrapped microspheres, but left largely unchanged with FOS (Table 5). Prior studies reported that Streptococcus species exhibit mixed effects on the colonic environment. While some species, such as Streptococcus agalactiae and Streptococcus bovis, display negative effects on colonic melieu(Reference Reynolds, Silva and McCormack36–Reference Santos-Sierra, Golenbock and Henneke38), other species such as S. salivarius, show positive characteristics(Reference Sartor39, Reference de Palencia, Lopez and Corbi40). In the present study, of these three species, S. bovis decreased slightly and S. salivarius decreased substantially after fermentation with starch-entrapped microspheres. This suggests that FOS has greater benefit than starch-entrapped microspheres with respect to Streptococcus growth in the healthy faecal microbiota.
Clostridium concentrations were substantially affected by the test carbohydrates to about the same degree for the two treatments (Table 5). Clostridium is sometimes recognised as a harmful or undesirable genus of the gut microbiota(Reference Gibson and Roberfroid4). For example, Clostridium difficile produces a toxin that causes colitis-like inflammation(Reference Freeman41), and infection with C. difficile exacerbates inflammation in patients with IBD(Reference Sartor, Rath and Sellon42). Unfortunately, we did not have a probe for C. difficile and were unable to determine how the test carbohydrates affected this bacterium. Two Clostridia that we did have probes, for Clostridium perfringens and Clostridium butyricum, were decreased by starch-entrapped microspheres and FOS by roughly the same degree. C. perfringens may have detrimental effects, suggested by significantly more C. perfringens found in faecal samples from patients with IBD compared with controls(Reference Mangin, Bonnet and Seksik43). In contrast, C. butyricum may be beneficial through its high SCFA (including butyrate) production and ability to alleviate dextran sodium sulphate-induced colitis in rats(Reference Araki, Andoh and Takizawa44). In fact, many clostridia are major contributors to the colonic butyrate pool(Reference Packey and Sartor28), which indicate that they may have an anti-inflammatory effect.
Escherichia concentrations were decreased during fermentation on starch-entrapped microspheres and FOS (Table 5). Escherichia, like clostridia, have mixed effects on the colonic environment, with some species exhibiting benefits and others exhibiting detrimental effects(Reference Gibson and Roberfroid4). While a virulent strain of Escherichia coli (adherent-invasive E. coli) has been isolated from the ileal mucosa of rats with CD(Reference Darfeuille-Michaud, Boudeau and Bulois45), and high concentrations of E. coli have been associated with colitis in rats(Reference Onderdonk, Richardson and Hammer46), other strains of E. coli (e.g. Nissle 1917) are established probiotics(Reference Schultz47).
In the healthy microbiota, the concentrations of Bifidobacterium and Lactobacillus were unaffected during fermentation, independent of carbohydrate source (Table 5). It is surprising that there was not an increase in Bifidobacterium on FOS, as FOS is known to be bifidogenic(Reference De, Vanhoutte and Huys48). A likely explanation is that the concentration of Bifidobacterium in the faecal microbiota community in healthy subjects, as suggested by Table 4, was initially quite high. If such was the case, previous research has demonstrated that it is almost impossible to then increase Bifidobacterium even further(Reference Roberfroid, Van Loo and Gibson49).
Changes in the microbiota from patients with inactive Crohn's disease during fermentation
The faecal microbiota from patients with inactive CD showed a substantial increase in Bacteroides concentration during fermentation (Table 5). Because faecal microbiota from patients with inactive CD had an extremely low concentration of Bacteroides at the initial time compared with the healthy microbiota (Table 4), an increase would represent a shift toward the healthy microbiota; thus suggesting a beneficial shift. The fact that FOS was able to increase Bacteroides more than starch-entrapped microspheres suggests that FOS was more desirable than starch-entrapped microspheres in this regard; however, some species of Bacteroides are undesirable. For instance, Bacteroides fragilis is found in much larger numbers in the mucosal biofilm in patients with CD compared with healthy individuals, and thus may play a role in the pathogenesis of this disease(Reference Swidsinski, Weber and Loening-Baucke50). Also, Bacteroides vulgatus expresses a membrane protein that induces inflammation(Reference Bamba, Matsuda and Endo51). There was an increase in B. fragilis during fermentation with FOS, whereas no change was observed with starch-entrapped microspheres (Table 5). B. vulgatus were increased with both carbohydrate treatments, but only FOS showed a significant increase. Therefore, starch-entrapped microspheres may be superior to FOS at preventing the growth of potentially harmful Bacteroides species.
Eubacterium and Veillonella concentrations were also affected differently by starch-entrapped microspheres compared with FOS. Eubacterium concentration was increased during fermentation with FOS, while no change was observed with starch-entrapped microspheres (Table 5). As mentioned, the major benefit of Eubacterium is high butyrate production(Reference Duncan, Belenguer and Holtrop35), yet the production of this metabolite was not significantly different among starch-entrapped microspheres and FOS when fermented with the microbiota from inactive CD patients (Table 3). This indicates that the increase in the concentration of Eubacterium seen with FOS must not have exceeded the increase in other butyrate producers induced by starch-entrapped microspheres. Veillonella was increased with FOS, whereas starch-entrapped microspheres had little effect. It is noteworthy that Veillonella is a harmful gut genus(Reference Gibson and Roberfroid4), because it is not saccharolytic and produces NH3 and hydrogen sulphide(Reference Rogosa52). Our finding suggests that starch-entrapped microspheres may be preferred over FOS by limiting growth of Veillonella.
Starch-entrapped microspheres and FOS induced similar effects on Enterococcus, Escherichia and Bifidobacterium concentrations (Table 5). Enterococcus signals were not increased with either carbohydrate. This is likely beneficial because high concentrations of Enterococcus have been associated with colitis in rats(Reference Onderdonk, Richardson and Hammer46) and Enterococcus facialis leads to distal colitis in mice(Reference Kim, Tonkonogy and Albright53). Escherichia concentrations were decreased with starch-entrapped microspheres and FOS. The mixed effects that this may have on the gut microbiota have been discussed earlier. Shifts in Bifidobacterium were unexpected. Significant decreases in this genus were observed when the microbiota from patients with inactive CD was exposed to starch-entrapped microspheres or FOS. One possible explanation is that stool microbiota community in patients with CD had an initially low concentration of Bifidobacterium; therefore, it is likely that these bacteria were unable to compete with the other bacteria for substrate, and thus were unable to proliferate. Alternatively, this decrease may simply be due to differences among healthy and diseased microbiota, i.e., a carbohydrate that is beneficial for one type of microbiota, may not be beneficial for another.
Changes in the microbiota from patients with active ulcerative colitis during fermentation
Starch-entrapped microspheres and FOS both resulted in similar decreases in Bacteroides signals during fermentation (Table 5). Unlike the microbiota from inactive CD, the microbiota from patients with active UC showed a much higher concentration of Bacteroides than the faecal microbiota in healthy subjects before fermentation; therefore, a decrease in Bacteroides after fermentation may be beneficial. Of the two detrimental Bacteroides that we had probes for, B. fragilis and B. vulgatus, FOS induced far greater decreases than starch-entrapped microspheres. This suggests that FOS may result in more favourable changes in Bacteroides than starch-entrapped microspheres in active UC due to more substantial decreases in these potentially harmful species belonging to this genus.
Clostridium concentrations were affected largely to the same extent regardless of carbohydrate source. Within the clostridial genera, the beneficial bacterium, C. butyricum, and the detrimental bacterium, C. perfringens, were also substantially decreased by both the carbohydrates (Table 5).
The effects of starch-entrapped microspheres and FOS were different with respect to their influence on Enterococcus, Fusobacterium, Streptococcus and Veillonella. Both the carbohydrates decreased Enterococcus concentrations, but FOS only showed a 2·5-fold decrease, while starch-entrapped microspheres showed a 4·5-fold decrease (Table 5). In light of the possible detrimental effects of Enterococcus on gut health(Reference Onderdonk, Richardson and Hammer46, Reference Kim, Tonkonogy and Albright53), these data would suggest a greater benefit of starch-entrapped microspheres compared with FOS. Fusobacterium is another genus of bacteria that is likely detrimental to gut health; Fusobacterium varium has been suggested as a causative agent for UC(Reference Ohkusa, Okayasu and Ogihara54). Even though we did not have a probe for this specific bacterium, a decrease in its genera may be beneficial. Starch-entrapped microspheres decreased the concentration of this genus, while FOS did not significantly affect Fusobacterium concentrations. Streptococcus increased with FOS and exhibited no substantial change with starch-entrapped microspheres; however, no change in the signals from individual Streptococcus species probes was observed. Veillonella was unchanged by starch-entrapped microspheres, but exhibited an increase with FOS. These data indicate that starch-entrapped microspheres may be better than FOS at preventing the proliferation of this purported harmful bacterial genus(Reference Gibson and Roberfroid4).
There were unexpected changes in Bifidobacterium. Starch-entrapped microspheres did not substantially affect the Bifidobacterium concentration, but FOS decreased its concentration (Table 5). This supports the conclusions from the inactive CD microbiota that Bifidobacterium were unable to compete with other bacteria for substrate, or that a carbohydrate that is beneficial in one case may not be beneficial in all instances.
Conclusions
Although the aetiology of IBD remains unknown, it is now thought that gut microbiota play a key role in initiation and/or progression of gut inflammation and associated tissue injury. For example, it is known that luminal bacteria of IBD patients differ significantly from healthy subjects(Reference Macfarlane, McBain, Gibson and Roberfroid31), and the abnormal microbiota are likely to play a role in the disease progression(Reference D'Haens, Geboes and Peeters3). Prebiotics and other dietary fibre substrates may be able to correct microbial abnormalities in IBD by selectively stimulating the growth of beneficial bacteria(Reference Gibson and Roberfroid4). In the present study, starch-entrapped microspheres, with long lasting and controlled fermentation properties, were tested against FOS, a known prebiotic, in regard to their influence on fermentation profiles and the stool microbiota of healthy subjects and patients with IBD.
Starch-entrapped microspheres showed clear advantages over FOS with respect to fermentation rate. Starch-entrapped microspheres fermented more slowly than FOS, as indicated by continued production of SCFA during the final starvation period (t = 70–72 h). This supports our previous findings(Reference Rose, Keshavarzian and Patterson12) and suggests that, unlike FOS, starch-entrapped microspheres should provide fermentable substrate into the distal colon, where carbohydrates, and consequently the beneficial fermentative metabolites, SCFA and particularly butyrate, are generally scarce.
Starch-entrapped microspheres resulted in higher butyrate production than FOS when fermented with the stool microbiota from healthy subjects and the stool microbiota from patients with active UC. This may have particular application for treatment of UC flare-ups and maintenance of remission due to the anti-inflammatory properties of butyrate. However, starch-entrapped microspheres did not outperform FOS in every aspect. For example, FOS resulted in lower concentrations of the potentially harmful bacteria, B. fragilis and B. vulgatus, after fermentation compared with starch-entrapped microspheres. Nevertheless, FOS resulted in higher concentrations of other potentially harmful bacteria: Enterococcus; Fusobacterium; Veillonella.
Starch-entrapped microspheres also showed benefits on the microbiota profile of patients with inactive CD. After fermentation with starch-entrapped microspheres, we noted lower concentrations of the potentially harmful Bacteroides species, B. fragilis and B. vulgatus, in the stool microbiota from patients with inactive CD compared with fermentation with FOS. Additionally, while FOS enhanced the growth of the harmful bacterial genus, Veillonella, starch-entrapped microspheres did not.
One limitation of the present study is that we used two different types of IBD to study active and inactive states of inflammation. Although UC and CD are inflammatory diseases of intestine and have many similar clinical and mechanistic features, they are two different types of inflammatory disorders. Our original aim was to compare the healthy microbiota to both active and inactive CD and UC. Unfortunately, during faecal collection, many patients were unable to produce a stool and thus we were unable to collect stools from all the groups. Since our primary aim was to compare the impact of fermentable substrates on microbiota composition and SCFA production between the healthy subjects and those with active and inactive colonic inflammation, we believe the use of inactive CD and active UC still yielded useful results.
Our findings suggest that different fermentable substrates affect the microbiota from individuals in healthy and diseased states differently, and that starch-entrapped microspheres induce a higher production of beneficial fermentation products and a decrease in the growth of potentially harmful gut bacteria more than FOS. Further in vivo studies are needed to confirm these findings and to determine whether starch-entrapped microspheres can promote gut health in healthy subjects and attenuate colonic inflammation in IBD and promote remission.
Acknowledgements
This research received no specific grant from any funding agency in the public, commercial or not-for-profit sectors. We wish to express gratitude to Annet Maathuis for her help with the TIM-2 apparatus and SCFA analyses. D. J. R. and K. V. performed the laboratory work. D. J. R. drafted the manuscript, except for the section on microbial analysis, which was written by K. V. All the authors were actively involved in experimental design, interpretation of the results, and editing of the manuscript. Authors (D. J. R., A. K., B. R. H.) have a pending (non-issued) patent for the microsphere product. However, the product is not commercialised and the purpose of the research was to determine the effects of delayed and slowly released starch on gut bacterial composition and bacterial fermentation.