Fructus Ligustri Lucidi (FLL), the fruit of Ligustrum lucidum Ait, is a commonly prescribed herb to nourish the endocrine and renal systems and to strengthen the bones in Traditional Chinese Medicine(Reference Neer, Arnaud and Zanchetta1). In the clinical practice of Traditional Chinese Medicine, FLL is a component of many kidney-tonifying Chinese herbal formulae for the treatment of osteoporosis(Reference Zhang, Lai and Leung2). Our previous study demonstrated that the ethanol extract (EE) of FLL could improve bone properties in the aged female rats, mainly by elevating Ca absorption and balance(Reference Zhang, Lai and Leung2, Reference Zhang, Leung and Che3). FLL was proven to have improved efficiency in utilising Ca from diet, mainly via its actions on increasing serum 1,25-dihydroxyvitamin D3 (1,25(OH)2D3) level and vitamin D-dependent Ca binding proteins (CaBP)(Reference Zhang, Lai and Leung2). Our results demonstrated that FLL enhanced the transcellular Ca transport in aged female rats fed with sufficient dietary Ca(Reference Zhang, Lai and Leung2). Our recent study reported that FLL increased the circulating levels of 1,25(OH)2D3 in aged female rats by directly stimulating the activity of its biosynthetic enzyme, 25-hydroxyvitamin D 1-hydroxylase(Reference Dong, Zhang and Favus4). Collectively, our research work on FLL indicated that direct actions of FLL EE on the vitamin D system might act as the initial targets for its protective effects on bone in the aged female rats(Reference Zhang, Lai and Leung2, Reference Dong, Zhang and Favus4).
Ca balance is determined by the relationships between Ca intake, absorption and excretion. Ca homeostasis in vivo is regulated by calciotropic hormones, including parathyroid hormone (PTH) and 1,25(OH)2D3. PTH regulates Ca homeostasis through three aspects, namely, stimulation of Ca release from bone, stimulation of renal Ca reabsorption to decrease urinary Ca loss and stimulation of renal 1,25(OH)2D3 production(Reference Ward, Maldonado-Perez and Hollins5). Ca (re)absorption through the intestine and kidney occurs by way of two main mechanisms, which are normally called paracellular and transcellular Ca transport. The paracellular transport is a passive non-saturable route(Reference Ward, Maldonado-Perez and Hollins5) while the transcellular transport is an active route that includes the action of PTH and vitamin D systems(Reference Hoenderop, Nilius and Bindels6). Intestinal Ca absorption and renal Ca reabsorption are primarily regulated by the genomic actions of 1,25(OH)2D3 on the transcellular pathway(Reference Hoenderop, Nilius and Bindels6) or through its non-genomic pathway on paracellular Ca transport(Reference Tudpor, Teerapornpuntakit and Jantarajit7, Reference Fujita, Sugimoto and Inatomi8). PTH seems to act indirectly on intestinal Ca absorption by stimulating 1,25(OH)2D3 production(Reference Ward, Maldonado-Perez and Hollins5), and the direct effect of PTH on the global process of intestinal Ca absorption has not yet been reported. PTH and 1,25(OH)2D3 interact to organise in a multilevel negative feedback loop for the purpose of maintaining Ca homeostasis.
Despite the fact that FLL could clearly modulate Ca and vitamin D metabolism in female rats, the chemical constituents in FLL that account for its positive effect on bone and mineral metabolism remain unknown. Previous studies(Reference Yim, Wu and Pak9–Reference Li, Zhang and Zhang11) reported that oleanolic acid and ursolic acid are the active compounds that might account for the hepatoprotective and osteoprotective effects of FLL. In addition, FLL contains quantitative constituents of polysaccharides. The polysaccharides in FLL, which are usually extracted by water(Reference Qiu and Li12), are proven to exert anti-oxidation, anti-ageing(Reference Zhang, Ge and Xu13) and immune stimulation effects(Reference Ruan and Lu14) in animal experiments. It is unclear if these previously reported compounds and constituents are responsible for the positive effects of FLL on Ca balance.
The present study aimed to determine the active fractions in FLL that are responsible for its positive effects on Ca balance and to characterise the effects of the FLL active fractions on Ca balance, calciotropic hormone levels as well as bone properties in mature female rats in response to treatment with diets containing different levels of Ca. It is hoped that the present study will further increase our understanding of the molecular actions of the active fractions in FLL that might be useful for increasing Ca bioavailability.
Methods
Preparation and fractionation of Fructus Ligustri Lucidi
Fructus Ligustri Lucidi (FLL) was obtained from Jiangsu province of China. A voucher specimen was deposited in The Hong Kong Polytechnic University. The crude plant (40 kg) was extracted with 70 % ethanol by the reflux method twice; and each lasted 1 h. The mixture was filtered to collect the filtrate, which was evaporated to almost dryness using a rotary evaporator under reduced pressure. The residue was finally lyophilised to dryness to obtain the EE (yield 11·42 %). The EE was then suspended in hot water and partitioned between ethyl acetate and water to obtain the ethyl acetate-soluble fraction (EAF) and the water-soluble fraction (WF). The two fractions were made into dried powders by a process of evaporation and lyophilisation.
Oleanolic acid and ursolic acid are the two main active compounds in FLL; and oleanolic acid is a commonly used chemical marker for the authentication of FLL according to the Chinese Pharmacopoeia (Edition 2010). HPLC detection of the two compounds in the EE of FLL and its two fractions, EAF and WF are shown in the Appendix. A C18 HPLC column (4·6 × 250 mm, 5 μm) was used in the HPLC analysis. A mobile phase consisting of MeOH–0·1 % acetic acid (87:13) was run at a flow rate of 1 ml/min, and the detection wavelength was set at UV 204 nm. Oleanolic acid and ursolic acid were adequately resolved from other unknown compounds and could be clearly identified by the retention time in the EE of FLL and its EAF (see Appendix (a)). WF was further analysed by HPLC under another condition (see Appendix (b)). A mobile phase consisting of acetonitrile and 0·1 % trifluoroacetic acid was run at a flow rate of 0·8 ml/min, and the detection wavelength was set at UV 230 nm. The linear gradient elution was set as follows: from the beginning, acetonitrile increased from 8 to 40 % in 40 min, and then increased to 70 % in the next 20 min. The two most prominent peaks in WF were identified to be nuezhenide and salidroside (see Appendix (b)).
Animal study design
A total of thirty-two 4-month-old mature (average weight 238·5 (se 2·5) g) Sprague–Dawley female rats (Experimental Animal Center of the Hong Kong Chinese University, Hong Kong, China) were used in the first animal study. Upon acclimatisation with a medium-Ca diet (MCD; TD Teklad 98 005, 0·6 % Ca, 0·65 % P) for 5 d, the mature rats were randomly divided into four groups: the control group (C, treated with vehicle (distilled water)) and FLL EE, FLL EAF or FLL WF as the treatment groups and pair-fed with MCD. The dosages of EE, EAF and WF were given according to their actual extraction ratio, namely 700, 126 and 574 mg/kg per d, respectively. Total treatment period was 12 weeks. In the second experiment, the effects of the active fraction on Ca balance and bone properties were studied. A total of sixty 4-month-old mature Sprague–Dawley female rats (220–250 g; Experimental Animal Center of the Hong Kong Chinese University, Hong Kong, China) were randomly divided into three groups with differing dietary Ca levels and treated with either FLL water fraction (WF, 574 mg/kg per d) or its vehicle (distilled water) for 12 weeks. All rats were fed a MCD (TD 98 005, 0·6 % Ca, 0·65 % P) for 5 d before the initiation of the treatment regimen. Diets containing different levels of Ca were low-Ca diet (LCD, TD 05 004, 0·1 % Ca, 0·65 % P), MCD and high-Ca diet (HCD, TD 05 005, 1·2 % Ca, 0·65 % P). All diets were purchased from Harlan Teklad (Madison, WI, USA). All rats had free access to distilled water, and were fed 15 g/d per rat of the respective diet, the minimum average food intake of the rats during the acclimation period. The body weight of the animals was recorded weekly. The rats were housed in a room that provided alternating 12 h of light and 12 h of darkness with the room temperature at 23 ± 1°C and humidity 55 ± 5 %. Husbandry of the animals was based on the National Institutes of Health Guide for Care and Use of Laboratory Animals(Reference Council15). The experimental protocol was approved by the Animal Ethics Committee of The Hong Kong Polytechnic University.
Sample collection
The animals, 2 d before being killed, were housed individually in metabolic cages for collection of urine and faeces. On the day of killing, blood was withdrawn from the abdominal aorta under light diethyl ether anaesthesia, and the serum was prepared. Rat tibias were collected, cleaned of all soft tissue, wrapped in saline-soaked towels and stored at − 20°C for further analysis.
Biochemical analysis of serum, urine and faeces samples
Ca concentration in both serum and urine samples was measured using standard colorimetric methods with commercial kits (Wako Pure Chemical Industries Limited, Osaka, Japan). Urinary creatinine (Cr) was determined using the Jaffe method by kits (Wako Pure Chemical Industries Limited). Urinary Ca excretion was expressed as ratio of urinary Ca to Cr level. The faeces was first dried (at 110°C for 12 h), then incinerated (at 800°C for 12 h) in a muffle furnace and weighed. Faecal ash (50 mg) was then dissolved in 2 ml of 6 m-HCl and diluted appropriately with Milli-Q water for atomisation. The amount of faecal Ca excretion in 24 h was determined by atomic absorption spectrophotometry (AAnalyst 100 Spectrometer; PerkinElmer, Waltham, MA, USA). The Ca absorption rate was calculated from the formula: Ca absorption rate (%) = (intake Ca − faecal Ca)/intake Ca × 100; the Ca net balance was calculated from: Ca net balance (mg) = intake Ca − faecal Ca − urine Ca.
Detection of calciotropic hormones
Serum levels of intact PTH (1–84) were detected using rat bioactive intact PTH ELISA assay (Immutopics, Inc., San Clemente, CA, USA). Serum 1,25(OH)2D3 was extracted with two separate extraction columns and measured by competitive enzyme immunoassay (Immundiagnostik AG, Bensheim, Germany).
Micro-computed tomography analysis of rat tibia
Left tibias were thawed at room temperature before testing. The bone properties of tibia were studied using cone-beam X-ray micro-computed tomography (μCT; vivaCT40; Scanco Medical AG, Basserdrof, Switzerland). The μCT images of the proximal metaphysis in the left tibia head were obtained by scanning at medium resolution with 21 μm increments at 70 kVp and 110 μA with a tube voltage of 50 kV, tube content of 0·1 mA, slice thickness of 13 μm and pixel size of 13 μm. The scanning process was controlled by an OpenVMS (Intel Itanium) workstation. The scanning positions of the tibia head were 2·5–7 mm from the knee joint. A total of 210 consecutive slices from metaphysis bone were obtained for evaluation. After evaluation, a μCT reconstruction model was generated and three dimensional bone parameters were calculated by software. Bone content parameters included apparent bone mineral density, bone mineral content and bone volume:tissue volume ratio (BV:TV). Bone structural parameters included trabecular number, trabecular thickness, trabecular separation, bone surface:bone volume ratio (BS:BV) and connectivity density.
Statistical analysis
Data from these experiments were reported as means with their standard errors. All statistical analyses were performed using PRISM version 4.0 (GraphPad Software Inc., La Jolla, CA, USA). Analysis of the effects of diet, herb and interaction of both factors as grouping variables was performed by two-way ANOVA. Inter-group differences were analysed by unpaired t-test as a post-test. Differences in P value of less than 0·05 were considered statistically significant.
Results
Effects of Fructus Ligustri Lucidi fractions on body weight, serum and urine chemistries
Body weight in the mature female rats increased gradually within the experimental period and the FLL treatment did not alter the weight gain (Table 1). Serum Ca and P were not altered by FLL treatment in mature female rats (Table 1). Urinary Ca:Cr was significantly decreased in rats by treatment with FLL EE and FLL WF (P < 0·05 v. C, Table 1). Urinary P:Cr levels were not significantly altered in rats upon treatment with EE and its fractions (Table 1). EE appeared to increase serum 1,25(OH)2D3 levels; however, the increase was not statistically significant (Table 1). Similarly, serum PTH levels tended to increase in rats upon EE and WF treatment, but the increase did not reach statistical significance (Table 1).
Table 1 Effects of the ethanol extract (EE) of Fructus Ligustri Lucidi (FLL) and its fractions on serum or urine chemistries in mature female rats fed a medium-calcium diet (MCD; 0·6 % calcium, 0·65 % phosphorus) for 12 weeks
(Mean values with their standard errors, n 6–8)

Cr, creatinine; 1,25(OH)2D3, 1,25-dihydroxyvitamin D3; PTH, parathyroid hormone; C, vehicle-treated group; EAF, ethyl acetate-soluble fraction in the EE of FLL; WF, water fraction in the EE of FLL.
* Mean values were significantly different from those of the vehicle-treated group (P < 0·05).
Effects of Fructus Ligustri Lucidi fractions on calcium balance
Faecal Ca excretion in rats was significantly decreased by EE and its WF treatment (P < 0·05 v. C, Fig. 1(a)). Both the calculated Ca net balance and Ca absorption rate in the mature rats were enhancfed simultaneously by EE and WF treatment (P < 0·05 and P < 0·01 v. C, respectively; Fig. 1(b) and (c)).
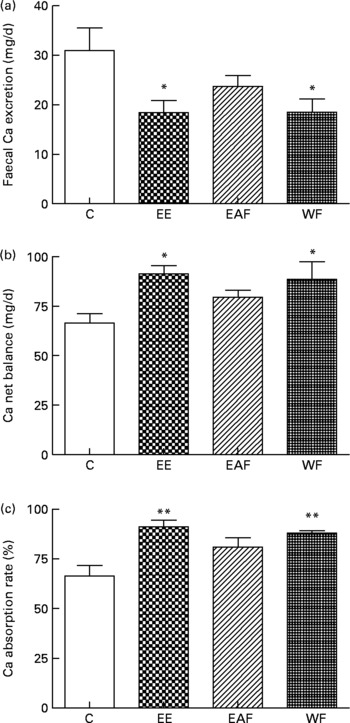
Fig. 1 Contents of (a) faecal Ca, (b) Ca net balance and (c) Ca absorption rate in each treatment group. Sprague–Dawley female rats (4 months old) were treated with vehicle (C; distilled water) and the ethanol extract (EE), ethyl acetate-soluble fraction (EAF) or water fraction (WF) of Fructus Ligustri Lucid (FLL), at the dosage of 700, 126 and 574 mg/kg per d, respectively. Total treatment period was 12 weeks. At the end of experiment, faeces and urine were collected for detection of Ca content. Ca net balance and Ca absorption rate were calculated according to their faecal and urinary Ca excretion. Values are means with their standard errors represented by vertical bars (n 6–8). Mean values were significantly different from those of vehicle-treated group: *P < 0·05, **P < 0·01.
Effects of Fructus Ligustri Lucidi water fraction on body weight, serum and urine calcium and phosphorus levels in rats fed with different levels of dietary calcium
Body weight increased slowly in all six rat groups during the experimental period and weight gain in mature rats was not significantly altered in response to treatment with FLL WF (Table 2). However, two-way ANOVA analysis indicated that dietary Ca level altered the weight gain significantly (P < 0·05, Table 2). In particular, LCD induced more weight gain in both vehicle- and WF-treated female rats (Table 2). Serum Ca did not alter in rats fed LCD or MCD in response to WF treatment (Table 2). However, HCD significantly increased serum Ca levels in the vehicle-treated rats (P < 0·05 v. LCD-fed rats), while WF significantly suppressed the increase in serum Ca levels in the HCD-fed groups (P < 0·05 v. vehicle-treated HCD-fed rats, Table 2). Furthermore, neither WF treatment nor Ca diets influenced serum P levels in our study (Table 2). WF treatment decreased urinary Ca:Cr in the rats that were fed diet containing higher levels of Ca, especially in the HCD groups (P < 0·05 v. vehicle-treated rats, Table 2). Urinary Ca:Cr was significantly increased with increasing dietary Ca levels as demonstrated by two-way ANOVA analysis (P < 0·001, Table 2). Similarly, urinary P:Cr in rats was shown to be significantly altered in response to dietary Ca (P < 0·0001, Table 2). In addition, WF treatment further increased urinary P:Cr in the LCD-fed rats (P < 0·05 v. vehicle-treated rats, Table 2).
Table 2 Effects of water fraction of Fructus Ligustri Lucidi (FLL) on weight gain and chemistries levels of calcium, phosphorus in serum and urine of normal mature female rats fed a low-calcium diet (LCD; 0·1 % calcium, 0·65 % phosphorus), medium-calcium diet (MCD; 0·6 % calcium, 0·65 % phosphorus) or high-calcium diet (HCD; 1·2 % calcium, 0·65 % phosphorus) for 12 weeks
(Mean values with their standard errors, n 6–8)
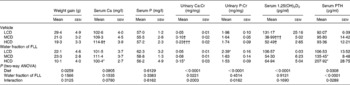
Cr, creatinine; 1,25(OH)2D3, 1,25-dihydroxyvitamin D3; PTH, parathyroid hormone.
* Mean values were significantly different from those of the vehicle-treated group fed with a similar level of dietary Ca (P < 0·05).
Mean values were significantly different from those of the LCD-fed rats in vehicle-treated groups: †P < 0·05, †††P < 0·001.
Effects of Fructus Ligustri Lucidi water fraction on calciotropic hormone levels in rats fed with different levels of dietary calcium
Two-way ANOVA analysis indicated that serum 1,25(OH)2D3 levels in rats were not altered by WF treatment (Table 2); however, they were significantly influenced by the levels of dietary Ca (P < 0·0001, Table 2). As expected, serum 1,25(OH)2D3 levels increased in rats fed LCD compared with those fed MCD or HCD (P < 0·05, P < 0·01, respectively; Table 2). In contrast, WF treatment significantly increased serum PTH levels in MCD- and HCD-fed rats (P < 0·05 v. vehicle-treated rats; Table 2). Furthermore, two-way ANOVA analysis indicated that the regulation of serum PTH was significantly altered by dietary Ca, WF treatment as well as interaction between the dietary Ca and WF (P < 0·05, Table 2).
Effects of Fructus Ligustri Lucidi water fraction on calcium balance in rats fed with different levels of dietary calcium
WF treatment appeared to suppress faecal Ca excretion in the MCD- and HCD-fed rats, though the suppression was not statistically significant (Fig. 2(a)). Net balance of Ca in vivo was significantly improved by WF treatment in both the MCD- and HCD-fed rats (P < 0·05, P < 0·01 v. vehicle-treated rats, respectively; Fig. 2(b)). Similarly, the Ca absorption rate in the MCD- and HCD-fed rats was significantly elevated by WF treatment (P < 0·05 and P < 0·01 v. vehicle-treated rats, respectively; Fig. 2(c)). In addition, high levels of dietary Ca significantly up-regulated faecal Ca and Ca net balance in both vehicle- and WF-treated mature female rats (P < 0·05 and P < 0·001 v. LCD-fed rats, respectively; Fig. 2(a) and (b)). Accordingly, the highest Ca absorption rate was found in the LCD-fed rats (P < 0·001 v. MCD- and HCD-fed rats, Fig. 2(c)).
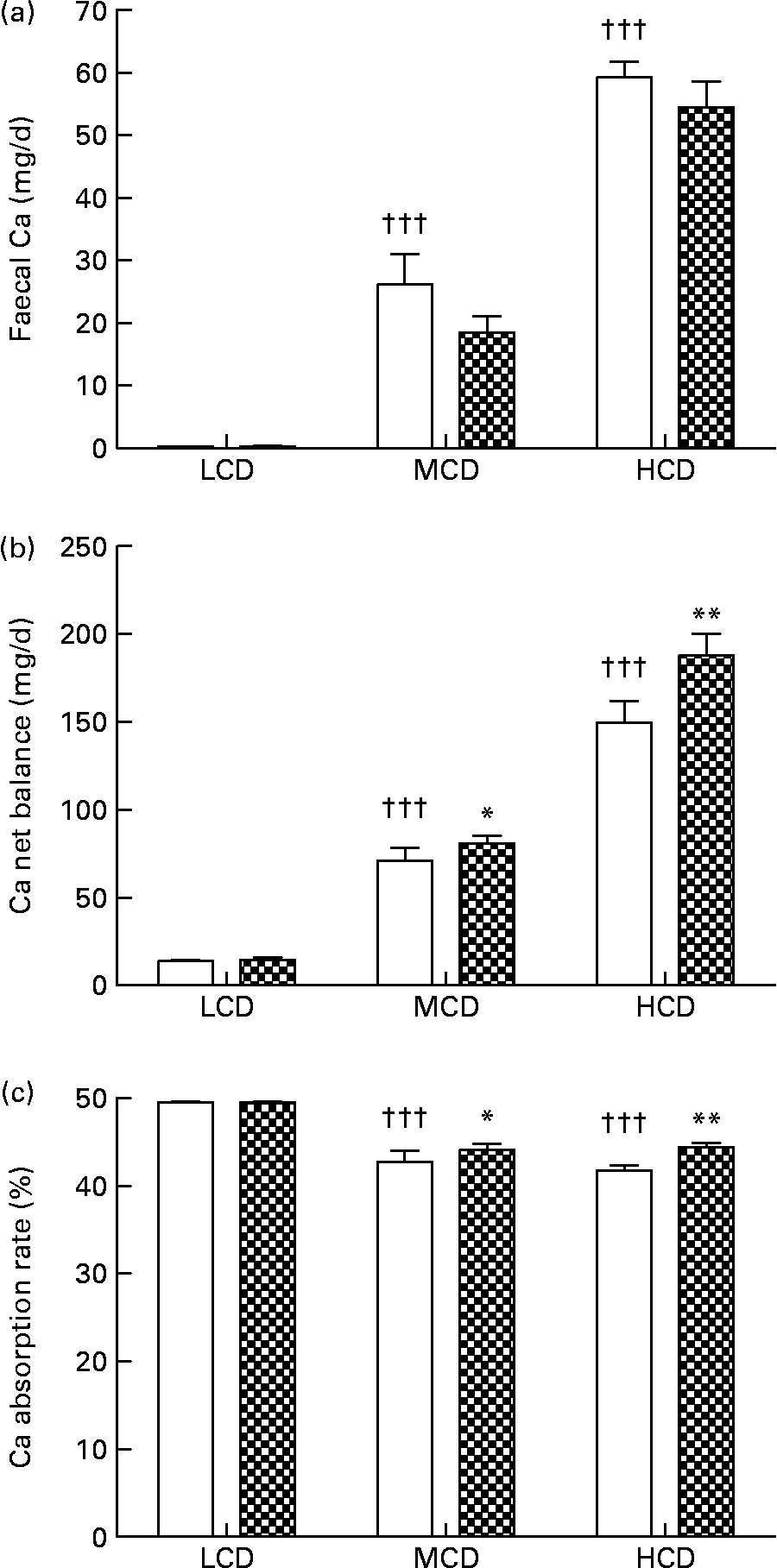
Fig. 2 Contents of (a) faecal Ca, (b) Ca net balance and (c) Ca absorption rate in each group. Sprague–Dawley female rats (4 months old) were treated with vehicle (□; distilled water) and water fraction (WF, ) of Fructus Ligustri Lucidi at the dosage of 574 mg/kg per d, under three different levels of dietary Ca feeding. Total treatment period was 12 weeks. At the end of experiment, faeces and urine were collected for detection of Ca content. Ca net balance and Ca absorption rate were calculated according to their faecal and urinary Ca excretion. Values are means with their standard errors represented by vertical bars (n 10). Mean values were significantly different from those of vehicle-treated group fed with a similar dietary Ca: *P < 0·05, ** P < 0·01. ††† Mean values were significantly different from those of low-Ca diet (LCD; 0·1 % Ca, 0·65 % P)-fed rats in vehicle-treated group (P < 0·001). MCD, medium-Ca diet (0·6 % Ca, 0·65 %P); HCD, high-Ca diet (1·2 % Ca, 0·65 % P).
Effects of Fructus Ligustri Lucidi water fraction on bone properties of rat tibia in rats fed with different levels of dietary calcium
Dietary Ca significantly altered the parameters of bone content and structure in proximal metaphysis of the rat tibia. Our results showed that higher dietary Ca significantly increased trabecular number, trabecular thickness, connectivity density, apparent bone mineral density, bone mineral content and BV:TV (P < 0·05, Table 3) and simultaneously decreased trabecular separation and BS:BV (P < 0·05, Table 3). Conversely, WF treatment seemed to induce negative alterations of bone parameters, including a slight decline of trabecular number, trabecular thickness, connectivity density, apparent bone mineral density, BV:TV and a slight increase of trabecular separation and BS:BV in all dietary groups; however, those slight effects on bone did not reach statistical significance. Two-way ANOVA analysis indicated that only dietary Ca level significantly influenced those bone parameters. Specifically, MCD and HCD were found to induce great improvement of apparent bone mineral density, bone mineral content and BV/TV in mature female rats (P < 0·05 and P < 0·01 v. LCD-fed rats, respectively; Table 3). WF treatment failed to induce apparent alteration of bone content in rats fed with different levels of dietary Ca (Table 3). Higher levels of dietary Ca could significantly ameliorate trabecular bone microarchitecture in rats through increasing trabecular numbers (P < 0·05 v. LCD-fed rats, Table 3), thickness (P < 0·01 v. LCD-fed rats, Table 3) and decreasing separation (P < 0·05 v. LCD-fed rats, Table 3) among them. Moreover, the ratio of bone surface area to total volume (BS:BV) decreased in rats in response to MCD or HCD (P < 0·01 v. LCD-fed rats, Table 3), suggesting a lower rate of bone resorption in these groups. Connectivity density in bone was improved by MCD and HCD treatment in rats (P < 0·05 v. LCD-fed rats, Table 3). Similarly, WF treatment did not result in any significant changes of bone microarchitecture in rats (Table 3).
Table 3 Effects of water fraction of Fructus Ligustri Lucidi (FLL) on the bone structural and bone content parameters in tibia metaphysis of normal mature female rats fed a low-calcium diet (LCD; 0·1 % calcium, 0·65 % phosphorus), medium-calcium diet (MCD; 0·6 % calcium, 0·65 % phosphorus) or high-calcium diet (HCD; 1·2 % calcium, 0·65 % phosphorus) for 12 weeks
(Mean values with their standard errors, n 6–8)
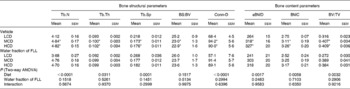
BS, bone surface; BV, bone volume; Conn-D, connectivity density; aBMD, apparent bone mineral density; BMC, bone mineral content; TV, tissue volume.
* Mean values were significantly different from those of LCD-fed rats in vehicle-treated groups (P < 0·05).
Discussion
Our previous studies reported that FLL EE exerted positive effects on Ca metabolism in aged female rats(Reference Zhang, Lai and Leung2). The present study extended our previous findings to demonstrate that FLL (EE) could increase Ca balance and Ca absorption in mature female rats. Furthermore, WF from EE, but not EAF, exhibited a prominent effect on Ca balance, indicating that WF might be the active fraction of EE for Ca regulation. The subsequent experiment confirmed that WF could offer significant enhancing effects on Ca balance and absorption when the dietary Ca was adequate. The positive actions of WF on Ca balance were associated with the rise in serum PTH levels without altering serum 1,25(OH)2D3 levels in mature normal female rats. Furthermore, the increase in PTH levels by FLL treatment did not induce apparent bone loss in the mature-female-rat model. In addition, WF was found to help prevent surges of serum Ca levels with increasing dietary Ca in the mature female rats.
The mechanism of the actions of FLL on vitamin D metabolism in female rats appeared to alter with age. In contrast to our previous study(Reference Zhang, Lai and Leung2) in which serum 1,25(OH)2D3 levels increased in response to FLL treatment in aged female rats, serum 1,25(OH)2D3 levels did not alter in response to treatment with FLL EE and its fractions in the mature female rats. Previous studies by others(Reference Lai, Chau and Cheung16, Reference Armbrecht, Boltz and Bruns17) showed that the levels of serum 1,25(OH)2D3 declined with age. Thus, it is possible that the lower background levels of serum 1,25(OH)2D3 in the aged rats allowed further stimulation of its biosynthesis by FLL in our previous study(Reference Zhang, Lai and Leung2). In contrast, the regulation of 1,25(OH)2D3 biosynthesis in mature rats might be tightly regulated by its stimuli and further stimulation might be inhibited by the physiological feedback mechanisms(Reference Dusso, Brown and Slatopolsky18), thereby preventing further increase of serum 1,25(OH)2D3 levels by FLL in mature rats in the present study.
Our results showed that WF treatment did not alter serum Ca levels in the rats fed LCD or MCD. Serum Ca levels were even down-regulated in the HCD-fed rats in response to WF treatment. Thus, the results indicated that WF treatment could prevent the surge of serum Ca in rats in response to HCD feeding. Moreover, WF treatment improved Ca net balance and Ca absorption rate in both the MCD- and HCD-fed rats through suppressing urinary and faecal Ca excretion. Most importantly, urinary Ca excretion was decreased greatly in the WF-treated HCD-fed rats. Thus, it was intriguing to find that serum Ca levels in WF-treated HCD fed rats were suppressed despite the induction of intestinal Ca absorption rate as well as the suppression of urinary Ca excretion by WF treatment in these animals.
Our results also showed that WF treatment significantly increased serum PTH levels in the MCD- and HCD-fed rats. It is well known that the hormonal regulation of PTH is complex and involves circulating Ca levels and 1,25(OH)2D3 (Reference Carrillo-Lopez, Fernandez-Martin and Cannata-Andia19). Low levels of extracellular Ca stimulate PTH secretion within minutes; while elevated levels of Ca inhibit hormone release and favour the degradation within parathyroid cells(Reference Silver, Kilav and Naveh-Many20). Another major regulator of PTH secretion is 1,25(OH)2D3 in which PTH mRNA expression in the parathyroid gland is regulated by 1,25(OH)2D3 (Reference Silver, Kilav and Naveh-Many20). In addition, 1,25(OH)2D3 is capable of indirectly inhibiting PTH secretion by increasing serum Ca through its actions on the intestine, bone and kidney(Reference Goodman21). As a result, serum PTH levels are normally induced in response to a prolonged decrease in serum Ca and 1,25(OH)2D3 levels, or an increase in serum P levels(Reference Silver, Kilav and Naveh-Many20). However, apart from the significant down-regulation of serum Ca levels by WF treatment in the HCD-fed rats, neither serum 1,25(OH)2D3 levels nor serum P levels were altered in these animals in response to WF treatment. Thus, the dramatic increase in serum PTH levels in both the MCD- and HCD-fed rats in response to WF treatment could not be explained by the changes in circulating levels of Ca, P or 1,25(OH)2D3. Our results suggest that the components in WF might exert direct effects on the regulation of PTH levels, possibly by altering the sensitivity of the parathyroid glands to serum Ca and 1,25(OH)2D3. Future studies will be needed to investigate the mechanism by which WF modulates serum PTH levels.
Elevation of serum PTH levels normally will enhance 1,25(OH)2D3 production, decrease urinary Ca excretion and increase bone resorption, thereby restoring serum Ca levels. Our results showed that the increase in serum PTH levels in mature female rats by WF treatment resulted in a significant reduction of urinary Ca excretion without any induction of serum 1,25(OH)2D3 levels or bone resorption. The loss of association between serum PTH and serum 1,25(OH)2D3 levels was also reported in our previous study that the significant increase in PTH levels in mature ovariectomised rats fed LCD did not result in any further increase in serum 1,25(OH)2D3 levels(Reference Zhang, Lai and Wu22). However, it is of interest to find that the positive actions of WF treatment on Ca balance did not result in any increase in bone mass and bone properties in mature female rats in contrast to our previous reported study on aged female rats(Reference Zhang, Lai and Wu22).
It is well known that secondary hyperparathyroidism is associated with increased bone turnover, decreased bone mineral density and increased fracture risks. On the other hand, intermittent administration of PTH induces bone formation(Reference Qin, Raggatt and Partridge24). Daily injection of PTH as either intact hormone PTH (1–84) or the active fragment PTH (1–34) is an efficacious bone anabolic treatment option for osteoporosis patients(Reference Neer, Arnaud and Zanchetta1, Reference Greenspan, Bone and Ettinger25, Reference Ettinger, San Martin and Crans26). Such injections lead to rapid and transient spikes in hormone exposure levels, a profile that is a prerequisite to form bone effectively(Reference John, Widler and Gamse27). Hence, the newly discovered oral compounds that triggered rapid, transient spikes in endogenous PTH levels in rats or humans would also benefit bone formation(Reference John, Widler and Gamse27, Reference Kumar, Matheny and Hoffman28). Thus, it is intriguing to find that the increase in serum PTH by WF treatment did not result in any negative influence on bone properties in the tibia in mature female rats fed MCD or HCD. However, it should be noted that the time-course responses of PTH levels to daily treatment of WT were not characterised in the present study. Thus, it is possible that the elevation of PTH levels in the WF-treated rats was transient and not continuous; further study is needed to characterise the PTH profile upon treatment with WT in these rats.
The lack of negative effects of high serum PTH levels on bone could be related to the overall Ca status/balance of the WT-treated animals as well as the protective effects of WT on bone. The present study clearly demonstrated that WT fraction could improve Ca balance in rats fed MCD or HCD, with a significant reduction of urinary Ca excretion as well as positive effects on intestinal Ca absorption. Our previous study showed that FLL extract could exert direct effects on the mineralisation process in osteoblast-like cells(Reference Zhang, Lai and Leung2). Thus, the positive effects of WT on bone and Ca balance might counteract the negative effects of high PTH levels in rats in the present study; resulting in no obvious changes in bone properties in the WT-treated animals fed MCD or HCD. Future studies are needed to investigate the mechanism by which WF treatment exerts protective effects on bone in the midst of increased PTH levels in mature female rats.
Currently, the most well-studied non-vitamin D dietary factors that increase Ca balance in humans or animals are non-digestible saccharides (NDS), including monosaccharides(Reference Brommage, Binacua and Antille29–Reference Tardivel, Fournier and Dupuis33) and fructo-oligosaccharides(Reference Takahara, Morohashi and Sano34–Reference Griffin, Davila and Abrams38). Several mechanisms that contribute to the actions of NDS have been proposed: (1) NDS could be fermented by residing bacteria to produce byproducts, such as SCFA in the caecum and colon to result in a decrease in intestinal pH that eventually leads to an increase in Ca solubility(Reference Scholz-Ahrens, Schaafsma and van den Heuvel39); (2) in vitro studies suggested that NDS indirectly open tight junctions in the epithelial cells by increasing intracellular Ca ion concentration, which in turn activates paracellular Ca transport and benefit Ca absorption(Reference Suzuki and Hara40); (3) NDS could increase active Ca transport by the activation of calbindin 9k in large intestine only(Reference Takasaki, Inaba and Ohta41). Our previous results indicated that FLL increased 1,25(OH)2D3-dependent CaBP expression in duodenum(Reference Zhang, Lai and Leung2) as well as renal 1-hydroxylase expression(Reference Dong, Zhang and Favus4) in aged female rats. In addition, the present study clearly demonstrated that FLL increased serum PTH levels in mature female rats. Thus, the mechanism of actions involved in increasing Ca balance by FLL and by NDS appears to be different.
Although FLL also contains quantitative constituents of polysaccharides(Reference Qiu and Li12–Reference Ruan and Lu14), the active constituents in FLL that are responsible for Ca regulation might not be NDS, as the reported mechanism of NDS is different from that of FLL. Moreover, it was found that oleanolic acid and ursolic acid(Reference Yim, Wu and Pak9–Reference Li, Zhang and Zhang11), the commonly reported active ingredients in FLL, are not contained in the identified active fraction of WF in the present study. Further study is required to identify the active components in FLL that exert the reported positive effects on Ca balance in vivo. Nuzhenide and salidroside, two major compounds that can be isolated from WF, might be candidates for explorations in future studies.
In summary, our present study demonstrated that FLL EE could improve Ca absorption and Ca balance, independent of the ages of the female rats. WF might be responsible for the positive effects of EE in regulating Ca absorption and Ca balance. The beneficial effects of WF in the mature female rats when dietary Ca was sufficient might be mediated by its direct or indirect action on PTH production. Most importantly, the increase in PTH levels induced by WF treatment did not result in any bone loss and the surges of serum Ca levels associated with high Ca feeding can also be prevented by WF treatment in mature female rats. These results suggested that WF is a potential agent that can be administrated orally to improve Ca balance and might be useful for the prevention of osteoporosis.
Acknowledgements
The authors thank the State Key Laboratory of Chinese Medicine and Molecular Pharmacology for providing the support in carrying out the present study. This work was supported by the Research Studentship and Niche Area Research Grant from the Research Committee of The Hong Kong Polytechnic University (I-BB8N) as well as the Shenzhen Basic Research Fund. X.-L. D was responsible for most of the experimental work and for drafting the manuscript. M. Z. and K.-K. W. helped in the herb extraction experiment and Micro CT detection work. C.-T. C. and M.-S. W. were responsible for the supervision and direction of the whole project as well as the final review of the manuscript. All authors declare that there are no conflicts of interest.
Appendix
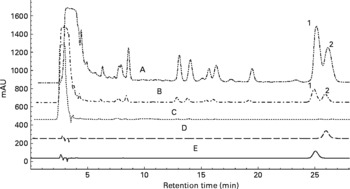
(a) Overlapped HPLC chromatograms for quantitative analysis of oleanolic acid and ursolic acid in the 70 % ethanol extract of Fructus Ligustri Lucidi (FLL), and its sub-fractions. (A) Ethyl acetate fraction of FLL (EAF); (B) 70 % ethanol extract of FLL (EE); (C) water fraction of FLL (WF); (D) ursolic acid standard; (E) oleanolic acid standard; (1) oleanolic acid; (2) ursolic acid.
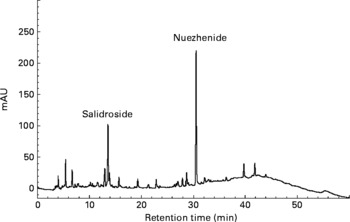
(b) HPLC profile of water-soluble fraction of Fructus Ligustri Lucidi run under a new condition. The two quantitative identified compounds in this fraction were salidroside and nuezhenide, which were shown in the figure, respectively.