INTRODUCTION
The faecal–oral pathway is considered to be the most important route of transmission of salmonella organisms in domestic animals. However, the ability of some Salmonella enterica serovars to survive in aerosols for prolonged periods of time [Reference McDermid and Lever1] suggests that airborne transmission might occur. Infection with Salmonella after exposure to contaminated aerosols has already been demonstrated in some animal species [Reference Tannock and Smith2–Reference Lever and Willians5]. It is quite probable that airborne transmission in pigs also occurs [Reference Heard and Linton6], and the role of nasal associated lymphoid tissues (NALT) in pigs as important sites of invasion of Salmonella Typhimurium [Reference Fedorka-Cray, Kelley, Stabel, Gray and Laufer7] supports this hypothesis. Moreover, seroconversion of specific pathogen-free (SPF) pigs receiving airflow from a room containing S. Typhimurium-infected pigs has been reported, even though no Salmonella was recovered from the exposed pigs [Reference Proux, Cariolet, Fravalo, Houdayer, Keranflech and Madec8].
This study tested the hypothesis of a short-distance airborne transmission of Salmonella Agona and S. Typhimurium in weaned pigs from commercial farms using controlled disease transmission isolation cabinets.
METHODS
Animals
Twelve cross-bred male weaned pigs were used. In each trial, six animals originating from the same litter were obtained from a commercial pig farm. In order to ensure that piglets were not infected by Salmonella, a monitoring phase was conducted on both farms. It comprised three visits during gestation and two visits after parturition, in which 10-g faecal samples from the sow and individual rectal swabs from the piglets were analysed for S. enterica by bacteriological isolation and PCR detection. In the first trial (Trial I), piglets were acquired from farm A after weaning (28 days), no Salmonella had been isolated from this farm in a previous study [Reference Oliveira, Carvalho, Fernandes, Tavechio, Menezes and Domingues9]. Piglets were further monitored for 11 days before being inoculated and placed in the isolation cabinets.
In the second trial (Trial II), piglets were obtained from farm B after weaning (13 days). They were also kept under strict isolation and individually monitored for Salmonella until 18 days of age, when they were inoculated and placed in the isolation cabinets. Salmonella prevalence in this farm had not been previously assessed.
Isolation cabinets
Considering the ubiquity of S. enterica and the high number of contamination sources, three identical stainless-steel/glass transmission disease isolation cabinets (Marconi Equipamentos para Laboratório, Piracicaba, SP, Brazil) were designed to assure that aerosols were the only possible mechanism of transmission of Salmonella and to prevent other possibilities of cross-contamination through vectors and vehicles. Each cabinet measured 0·80×0·80×1·30 m and was equipped with water and feed containers, which could be externally filled and were hermetically sealed. The animals received autoclaved meal feed and water throughout both trials. A fumigation chamber with an internal and an external door permitted sampling without the need to open the system. The cabinets had a slotted floor and slurry was removed through a tube connected to a plastic sack. Animals were handled and sampled with the aid of rubber gloves laterally coupled to the cabinets. The cabinets were connected to each other with a 0·8-m-long duct with 0·2 m diameter. Ventilation rate in both trials was 550 m3/h (∼1 m3/pig per min). Both exhauster (ExaustFlow500, Filtracom Ltda, Campinas, SP, Brazil) and fan (SterilFlow500, Filtracom Ltda) units included pre-filters and HEPA filters, with 99·97% of efficiency for 0·3-μm particles.
Bacteria and inoculum
S. Agona (accession no. IAL 0845/01) and S. Typhimurium (IAL 1007/01) were originally isolated from pigs [Reference Oliveira, Carvalho, Domingues, Menezes, Fernandes and Tavechio10] and kindly provided by Instituto Adolfo Lutz (São Paulo, SP, Brazil). Both strains contained a nalidixic acid resistance marker (S. Agonanal+ and S. Typhimuriumnal+). These serotypes were selected because they have been isolated from healthy pigs in Brazil [Reference Oliveira, Carvalho, Domingues, Menezes, Fernandes and Tavechio10] and are among the leading serotypes isolated from human sources in this country [Reference Tavechio, Fernandes, Neves, Dias and Irino11].
The inoculum was prepared as described by Wood et al. [Reference Wood, Rose, Coe and Ferris12]. Scrapings from pure stock cultures were first streaked onto nutrient agar (CM003, Oxoid, Basingstoke, Hampshire, UK) and then onto modified Brilliant Green agar (CM329, Oxoid) supplemented with nalidixic acid (50 μg/ml). A fresh culture was prepared inoculating a single colony in Luria–Bertani broth (LB) and a 3-ml aliquot was slowly administered into the pharyngeal region with a plastic syringe. The number of S. Agonanal+ and S. Typhimuriumnal+ present in the inoculum, as determined by the drop-counting technique [Reference Miles and Misra13], were 1·2×109 and 2·0×109 colony-forming units per 1 ml (c.f.u./ml) respectively. Pigs were fasted for 18 h (overnight) before inoculation and feed and water were only given 30 min after inoculation.
Experimental design
Before each trial, isolation cabinets were cleaned with detergent solution and disinfection was carried out with 70% alcohol and then connected as shown in the Figure. A solution containing 2% glutaraldehyde and quaternary ammonium (AVT500, Polysell, Campinas, SP, Brazil) was sprayed into the cabinets and air ducts and the system was kept closed for 48 h. Glutaraldehyde was chosen because it is not corrosive and is considered a high-level disinfectant and chemical sterilant [Reference Rutala, Murray, Baron, Pfaller, Tenover and Yolken14]. Swabs from internal parts of the cabinets and air ducts were taken for bacteriological analysis after the cleaning and disinfection procedures and before the animals were housed.
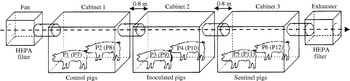
Fig. Arrangement of isolation cabinets. In Trial I (Salmonella Agona), control pigs (P1, P2), inoculated pigs (P3, P4) and sentinel pigs (P5, P6) were housed in cabinets 1, 2 and 3 respectively. In Trial II (Salmonella Typhimurium), control pigs (P7, P8), inoculated pigs (P9, P10) and sentinel pigs (P11, P12) were placed in cabinets 1, 2 and 3 respectively.
In both trials, animals were randomly chosen and placed in the cabinets. In Trial I, control (P1 and P2) and sentinel (P5 and P6) pigs received 3 ml of sterile LB and were placed in cabinets 1 (C1) and 3 (C3) respectively. Pigs 3 (P3) and 4 (P4) were inoculated with S. Agonanal+ and placed in the isolation cabinet 2 (C2). The system was closed and the animals were housed until 15 days post-infection (DPI). The relative humidity of the air ranged between 65% and 80% and temperature ranged between 24 and 28°C.
In Trial II, control (P7 and P8), and sentinel (P11 and P12) pigs received sterile LB (3 ml) and were put in C1 and C3 respectively, while S. Typhimuriumnal+-inoculated pigs (P9 and P10) were placed in C2. The system was opened at 19 DPI, when the animals were necropsied. The relative humidity of the air ranged between 45% and 65%, while temperature ranged between 26 and 30°C.
Sampling
Individual rectal swabs, faeces from the floor of cabinets and air samples were collected daily in both trials. Screw-cap tubes containing enrichment broth, sterile plastic bags for faecal sampling and agar plates were placed in the fumigation chamber through its external door and sprayed with a solution containing 2% glutaraldehyde and quaternary ammonium (AVT500, Polysell). After 2 h, the internal door of the fumigation chamber was opened and sampling was performed. A similar disinfection procedure was conducted before taking the samples out of the system. Blood samples were collected at the beginning (0 DPI) and at the end of each trial (15 DPI and 19 DPI). After centrifugation, serum samples were separated and stored at –20°C until analyses.
Necropsy
At the end of each trial, pigs were necropsied according to the euthanasia procedure approved by the Animal Welfare Commission of Faculdade de Ciências Agrárias e Veterinárias de Jaboticabal (CEBEA-FCAVJ). Palatine tonsils, mandibular, mesenteric, ileocolic, and colic lymph nodes, liver, lung, portions of jejunum and ileum (10-cm segments), and caecal content samples were aseptically taken using disposable gloves and sterile surgical instruments for each tissue. Samples were placed in sterile plastic bags (BO1065WA, Whirl-pak, Nasco, Fort Atkinson, WI, USA), kept under refrigeration and transported to the laboratory for bacteriological analysis within 1 h of sampling.
Bacteriology
Rectal swabs were first enriched in GN-Hajna broth (GN broth, Hajna, 248610, Difco, Franklin Lakes, NJ, USA) overnight at 37°C. Aliquots of 1·0 and 0·1 ml were then transferred to 9 ml of Müller–Kauffmann tetrathionate (MKT) broth (CM343, Oxoid) and 9·9 ml of Rappaport–Vassiliadis soya peptone (RVS) broth (CM866, Oxoid) which were incubated at 37°C for 48 h and at 42°C for 24 h respectively. Afterwards, a loopful of each broth was plated onto xylose-lysine-tergitol (Bacto XLT-4, 223420, Difco) and modified Brilliant Green agar (CM329, Oxoid) plates, both containing nalidixic acid (50 μg/ml). The pooled faecal samples from the floor of the cabinets were analysed as previously described [Reference Oliveira, Carvalho, Domingues, Menezes, Fernandes and Tavechio10].
Air samples were collected through an impaction method previously described [Reference Mitchell, Buhr, Berrang, Bailey and Cox15]. Duplicates of modified Brilliant Green agar plates were placed for 10 min at the entrance of the air duct in each cabinet and incubated at 37°C for 24 h. Tissue samples were processed as described by Hurd et al. [Reference Hurd, Gailey, McKean and Rostagno16].
All strains presumptively identified as S. enterica were confirmed by slide agglutination test using Salmonella Group B antiserum (Probac do Brasil, São Paulo, SP, Brazil) and serotyped at a reference laboratory (Instituto Adolfo Lutz, São Paulo, SP, Brazil).
Polymerase chain reaction (PCR)
Faecal samples enriched in RVS were analysed by PCR, because of the high efficiency of the PCR cultivation method using this broth [Reference Oliveira, Freschi, Carvalho and Leontides17]. Aliquots of enriched RVS (1 ml) were taken and submitted to DNA extraction by a boiling-centrifugation technique. After initial centrifugation (13 000 g, 10 min), the pellet was resuspended in 100 μl of sterile double-distilled water and boiled for 10 min. Extraction products were centrifuged (4000 g, 4 min) and 5 μl was added to 25 μl of the reaction mixture containing 10 mM Tris–HCl (pH 8·3), 1·5 mM MgCl2, 50 μ M of each dNTP (Biotools do Brasil, Rio de Janeiro, RJ, Brazil), 1 μ M of each oligonucleotide primer and 1 U of Taq DNA polymerase (Biotools do Brasil). The pair of primers was specific for the rfb gene of S. enterica Group B (5′-AGA ATA TGT AAT TGT CAG and 5′-TAA CCG TTT CAG TAG TTC) [Reference Kongmuang, Luk and Lindberg18]. The reaction was performed in a thermocycler (Mastercycler Personal, Eppendorf, Germany) and comprised of a 10-min denaturation step at 94°C, 40 cycles of amplification at 94°C for 40 s, 53°C for 30 s and 72°C for 2 min and a final extension at 72°C for 10 min. Aliquots of the PCR products (10 μl) were electrophoresed in 1·5% agarose gels and visualized after ethidium bromide staining. Samples showing an amplified fragment of 882 bp were considered positive [Reference Kongmuang, Luk and Lindberg18].
Serology (ELISA)
Salmonella antibody titres in serum samples were evaluated by an ELISA test based on LPS antigens of S. Typhimurium [Reference Kich, Cardoso, Coldebella, Piffer, Vizzoto, Silva, Castanha and Leontides19], which was developed at a reference centre (Embrapa Suínos e Aves, Concórdia, SC, Brazil). The results were expressed as mean calibrated optical density (MCOD).
Results analyses
Considering the use of controlled isolation cabinets coupled to absolute HEPA filters, it was assumed that airborne movement was the only mechanism of Salmonella spreading to other cabinets in the system. Thus, recovery of nalidixic acid-resistant S. Agona (Trial I) or S. Typhimurium (Trial II) from sentinel pigs was considered sufficient to reject the null hypothesis (H0: airborne transmission is not possible) and corroborate the alternative hypothesis (H1: airborne transmission is possible).
Agreement between isolation and PCR for the samples analysed by both detection methods was determined by the coefficient of association kappa and interpreted as poor (<0·0), slight (0 to⩾0·2), fair (0·21 to⩾0·4), moderate (0·41 to⩾0·6), substantial (0·61 to⩾0·8) and almost perfect (0·8 to 1), according to Landis & Koch [Reference Landis and Koch20].
Results
Bacteriology
No Salmonella was cultured or detected by PCR from faecal samples collected during the monitoring period prior to the inoculation. Swab samples collected from the isolation cabinets and air duct surfaces after the disinfection procedures were also negative.
In both trials, no Salmonella was detected in rectal swab samples from the control pigs (P1, P2, P7 and P8) in C1 as well as from air and faecal samples from this cabinet (Tables 1 and 2). In Trial I, S. Agonanal+ was frequently isolated from individual rectal swabs collected from the inoculated pigs (P3 and P4) as well as from the pooled faecal samples taken from the floor of C2 (Table 1). S. Agonanal+ was also isolated from air samples collected from C3 from 1 to 4 DPI and from faeces on the floor of this cabinet at 2, 3, 5, 6 and 7 DPI. The pathogen was detected by PCR in the pooled faecal samples at 5, 6, 12 and 15 DPI. Positive rectal swabs were detected by culture at 5 DPI from P5 and from 11 to 14 DPI from P6. In some cases, positive samples were detected by PCR but not by culture, or vice versa (Table 1).
Table 1. Results of Salmonella Agona isolation (ISO) and PCR detection (PCR) from samples collected in Trial I
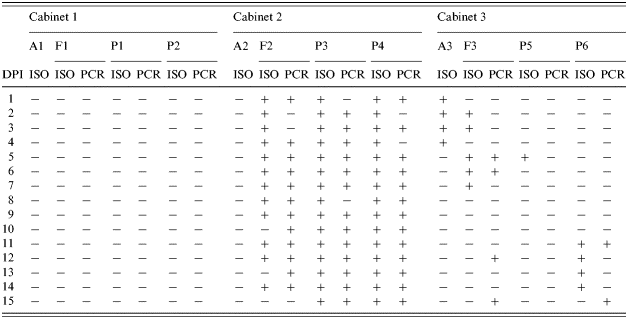
DPI, days post-infection;A, air samples; F, pooled faecal samples from the floor; P, rectal swabs from pigs.
Table 2. Results of Salmonella Typhimurium isolation (ISO) and PCR detection (PCR) from samples collected in Trial II

DPI, days post-infection; A, air samples; F, pooled faecal samples from the floor; P, rectal swabs from pigs.
* Sampling was not performed on 17 DPI.
In Trial II, positive samples were frequently isolated from individual rectal swabs collected from the inoculated pigs (P9 and P10) and from the pooled faecal samples on the floor of C2 (Table 2). However, no Salmonella was isolated from air samples entering C3. S. Typhimuriumnal+ was isolated from the pooled faecal samples from C3 at 1, 4, 5, 8 and 11 DPI and detected by PCR at 1, 5, 8, 12 and 16 DPI (Table 2). It was also isolated from a rectal swab sample collected from P11 at 5 DPI but not from P12 throughout the experiment. Nevertheless, rectal swabs from P12 were positive for Salmonella by PCR at 2, 5 and 18 DPI.
Necropsy samples
No Salmonella was isolated from samples of control pigs in both trials (Table 3). In Trial I, S. Agonanal+ was isolated from all tissues from the infected pigs, except for lungs and liver. In this trial, S. Agonanal+ was recovered only in caecal contents from P5, while ileocolic and colic lymph nodes, liver, lung and caecal contents from P6 were positive. Ileocolic lymph nodes from P5 and mesenteric lymph nodes from P6 were positive by PCR but negative by isolation (Table 3).
Table 3. Results of isolation (ISO) and PCR detection (PCR) of Salmonella Agona and Salmonella Typhimurium from tissues collected in Trials I and II respectively

In Trial II, Salmonella Typhimuriumnal+ was isolated from all tissues from P9 and P10, except for lung and liver samples from both inoculated animals and from segments of jejunum-ileum and colic lymph nodes from P10. Salmonella Typhimurimnal+ was not isolated from P11. Both isolation and PCR were able to detect the pathogen in fragments of jejunum-ileum and ileocolic lymph nodes collected from P12 at the end of the trial.
Serology
Antibody titres against Salmonella at the beginning and at the end of each trial can be seen in Table 4. No variation in MCOD of serum samples was detected in control animals. The inoculated animals from Trial I (P3 and P4) showed increased MCOD at 15 DPI. However, both inoculated pigs in Trial II (P9 and P10) showed decreased MCOD at 19 DPI. One sentinel pig in each trial (P6 and P12) showed marked increase in serum MCOD at the end of the experimental period.
Table 4. ELISA results of serum samples at inoculation (0 DPI) and at the end of Trial I (15 DPI) and Trial II (19 DPI). Results are expressed in mean calibrated optical density (MCOD)
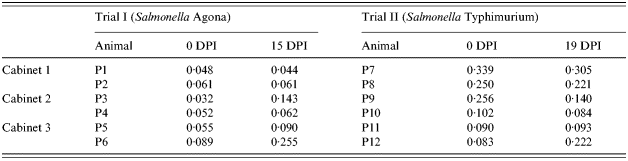
DPI, days post-infection.
DISCUSSION
Considering that the animals were housed in disease transmission isolation cabinets, the recovery of S. Agonanal+ and S. Typhimuriumnal+ from sentinel pigs in the trials gives evidence that such pathogens can be spread through the air and infect weaned pigs over a short distance. Furthermore, macrorestriction of chromosomal DNA and plasmid profiling of isolates recovered from sentinel and inoculated pigs in each trial showed indistinguishable patterns compared to the respective strain used as inoculum (data not shown). This was sufficient to reject the null hypothesis and, therefore, other statistical analyses were not attempted. These findings confirm previous indications about the possibility of airborne transmission of S. enterica in swine [Reference Heard and Linton6, Reference Fedorka-Cray, Kelley, Stabel, Gray and Laufer7, Reference Stärk21]. Furthermore, aerosol transmission of S. enterica has been already demonstrated in poultry [Reference Lever and Willians5, Reference Gast, Mitchell and Holt22], calves [Reference Wathes, Zaidan, Pearson, Hinton and Todd4, Reference Hardman, Wathes and Wray23], sheep [Reference Tannock and Smith2] and mice [Reference Tannock and Smith3, Reference Wathes, Zaidan, Pearson, Hinton and Todd4].
Although similar experiments have been performed to test airborne transmission of other pathogens in pigs [Reference Torremorell, Pijoan, Janni, Walker and Joo24, Reference Brockmeier and Lager25], the present report is, according to our knowledge, the first to describe airborne transmission of S. enterica in pigs using isolation cabinets.
Samplings of individual rectal swabs were performed to determine when pigs started shedding Salmonella. According to our results, Salmonella was isolated from the inoculated pigs in both trials early on the first day post-infection (Tables 1 and 2) which is in agreement with previous reports about rapid Salmonella shedding after experimental inoculation [Reference Fedorka-Cray, Kelley, Stabel, Gray and Laufer7, Reference Fedorka-Cray, Whipp, Isaacson, Nord and Lager26, Reference Van Winsen, van Nes and Keuzenkamp27].
In Trial I, one sentinel pig (P6) showed a consistent shedding pattern of S. Agonanal+ between 11 and 15 DPI. In Trial II, however, S. Typhimurium was isolated from P11 at 5 DPI detected only by PCR in rectal swabs from P12 at 2, 5 and 18 DPI. In the only available report about airborne transmission of S. Typhimurium in swine, no bacteria were isolated from sentinel animals exposed to airflow from a room with infected pigs and only seroconversion was observed [Reference Proux, Cariolet, Fravalo, Houdayer, Keranflech and Madec8].
Despite the large variation of time and frequency of Salmonella shedding that is seen in exposed pigs [Reference Fedorka-Cray, Whipp, Isaacson, Nord and Lager26, Reference Van Winsen, van Nes and Keuzenkamp27], S. Typhimurium can be rapidly isolated from faeces soon after they are commingled with animals shedding this pathogen [Reference Fedorka-Cray, Whipp, Isaacson, Nord and Lager26], or when they are placed in a contaminated environment [Reference Hurd, Gailey, McKean and Rostagno16]. However, when infected and sentinel pigs had only nose-to-nose contact, the majority of the exposed pigs shed S. Typhimurium after 5 weeks [Reference Proux, Cariolet, Fravalo, Houdayer, Keranflech and Madec8]. These findings could support the hypothesis that contaminated faeces are a major source for cross-contamination between pens [Reference Heard and Linton6].
The recovery of S. Agonanal+ from air samples collected at the entrance of C3 during four consecutive days in Trial I support the assumption that sentinel pigs acquired the infection after receiving contaminated air from C2. The fact that S. Typhimuriumnal+ was not recovered from air samples in Trial II does not refute the hypothesis of airborne transmission. Although brilliant green agar has been considered to give superior results compared to other selective media in isolating Salmonella from air samples [Reference Gast, Mitchell and Holt28], it must be remembered that isolation of specific pathogenic bacteria from environmental air in animal facilities is always difficult, and results are frequently negative for extensive contamination by saprophytic microorganisms [Reference Torremorell, Pijoan, Janni, Walker and Joo24].
Considering the 387 samples that were analysed by both isolation and PCR, there was substantial agreement between the two tests. The kappa coefficient (0·77) found herein confirms the successful use of enriched Rappaport–Vassiliadis broth as source of DNA template for PCR as an additional method to improve Salmonella detection sensitivity in porcine faecal samples [Reference Oliveira, Freschi, Carvalho and Leontides17, Reference Kongmuang, Luk and Lindberg18]. It has been suggested that enrichment allows for dilution of inhibitory substances and also increase salmonella numbers in faeces [Reference Kongmuang, Luk and Lindberg18]. Nineteen samples were positive by PCR and negative by isolation, whereas 21 samples were positive by isolation and negative by PCR. These discrepant results and the fact that isolation of bacteria is required to confirm transmission hypothesis in experimental trials suggest that enrichment broth cultivation PCR might be used only as a support diagnostic tool, and not as a substitute for bacterial isolation.
The experimental design used herein does not allow conclusions about the invasion sites of Salmonella in the sentinel pigs. The frequency of recovery of Salmonella in both trials, i.e. the rapid environmental contamination in C3 before identification of Salmonella shedding by sentinel pigs, might suggest that these animals acquired Salmonella through ingestion, probably from environmental surfaces contaminated by aerosols from C2, including feed. This hypothesis is supported by the fact that slaughter-age pigs can be rapidly infected when exposed to relatively low numbers of S. Typhimurium in the environment [Reference Hurd, Gailey, McKean and Rostagno16].
On the other hand, the hypothesis of inhalation of Salmonella by sentinel pigs cannot be excluded. Using oesophagotomized pigs, Fedorka-Cray et al. [Reference Fedorka-Cray, Kelley, Stabel, Gray and Laufer7] showed that S. Typhimurium can rapidly accumulate in the gut by routes other than the classical oral route of inoculation and elucidated the importance of NALT as an important portal of entry of S. Typhimurium in pigs. These tissues can also constitute important sites of invasion to bacteria present in particles of food and faeces, since the behaviour of pigs ensures that such particles usually enter the nasal cavity during feeding and rooting [Reference Baskerville and Dow29].
Interestingly, the isolation of S. Agonanal+ from lung and liver samples from P6, as well as tissues from the digestive tract, are suggestive of systemic infection. Systemic infections in pigs have been frequently documented in experiments using the intranasal respiratory route of inoculation [Reference Fedorka-Cray, Kelley, Stabel, Gray and Laufer7] but have not been usually described in trials using oral exposure [Reference Wood, Pospischil and Rose30, Reference Wood and Rose31]. The sequence of exposure, i.e. oral–respiratory vs. respiratory–oral, could also affect the immune response, which could in turn affect the severity of the disease [Reference Fedorka-Cray, Kelley, Stabel, Gray and Laufer7].
The differences in animal ages, animal origin, mean humidity and temperature do not permit direct comparisons between the two trials. The most important difference between the two trials lies in the different immune status of the animals before the inoculation (Table 4). The mean MCOD in animals from farm A (Trial I) was only 0·056, while it was 0·187 in animals from farm B (Trial II). Three animals in Trial II (P7, P8 and P9) showed higher MCOD than the standard cut-off value for the test (0·169), which indicates the presence of maternal antibodies against Salmonella. Despite the fact that S. Typhimuriumnal+ has been isolated from tissues collected from one sentinel pig (P12) in Trial II, the presence of lactogenic immunity could explain the lack of Salmonella shedding by the sentinel pigs throughout the experimental period. Indeed, the presence of maternal antibodies against Salmonella may protect piglets during the first weeks [Reference Proux, Houdayer and Humbert32].
Two sentinel pigs (P6 and P12) showed increased titres of antibodies against Salmonella. These results corroborate previous findings on humoral immune response detection after aerosol exposure in pigs [Reference Proux, Cariolet, Fravalo, Houdayer, Keranflech and Madec8].
Titre decrease seen in infected pigs in Trial II could be due to the depletion of maternal antibodies after challenge. Interestingly, infected pigs in Trial I showed no marked increase in antibody titres. Such low serological response could be attributed to the relatively low age of the pigs and the relatively short experimental period. In fact, seroconversion has been shown to vary substantially among inoculated pigs (6–37 days) and seroconversion usually peaks around 30 DPI [Reference Nielsen, Baggesen, Bager, Haugegaar and Lind33]. In naturally infected pigs, this lag-time between infection and seroconversion may be even longer [Reference Kranker, Alban, Boes and Dahl34].
The lack of knowledge and the complexity of the epidemiology of Salmonella in modern pig production systems are considered as the greatest problems to control these bacteria on farms. Numerous reports about the impact of nutrition, production systems, management, and transport/lairage strategies on the reduction of Salmonella in swine have been published and the faecal–oral cycle of infection is usually taken as the basis for epidemiological analysis. On the other hand, little attention has been paid to the spread, contamination and infection of Salmonella through aerosols in pigs. Whether or not this transmission pathway may also be a matter of concern in the epidemiology of Salmonella in intensive pig production systems remains to be clarified. In poultry, however, contaminated aerosols have already been demonstrated to play an important role on the transmission of Salmonella [Reference Nakamura, Takagi, Takahashi, Suzuki, Sato and Takehara35] and methods to reduce aerosolized dust levels have been applied successfully to diminish transmission of this pathogen in hatching cabinets and broiler breeding facilities [Reference Mitchell, Buhr, Berrang, Bailey and Cox15, Reference Mitchell and Waltman36, Reference Richardson, Hofacre, Mitchell and Wilson37].
In this sense, environment and management improvements focusing on aerosol reduction might play an important role on the prevention of Salmonella infection in swine as well. In order to reduce airborne disease transmission in pigs, the air volume per animal, as well as the ventilation and humidity rates should be adequate, and high stock densities of rearing pigs and excessive movement of animals should be avoided [Reference Stärk21]. It is worth noting that such measures not only decrease infectious aerosols, but also contribute to decreased animal stress. Moreover, spraying oil or a mixture of oil and water in the environment can efficiently reduce dust concentrations in pig barns [Reference Pedersen, Nonnenmann, Rautiainen, Demmers, Banhazi and Lyngbye38]. Finally, as feed is the major source of airborne dust [Reference Honey and McQuitty39], feeding strategies should also be considered as options to reduce dust in barns. Fermented liquid feeding has been associated with decreased Salmonella prevalence in swine, probably as a result of the combined effect of large amounts of organic acids and large numbers of lactic acid-producing bacteria present in these feeds [Reference Van der Wolf, Wolbers and Elbers40]. On the other hand, the reduced production of dust when using this feeding method should also be considered.
In conclusion, short-distance airborne transmission of S. Agona and S. Typhimurium in weaned pigs is possible. Further studies on the impact of aerosol and dust reduction on the control of Salmonella in intensive pig raising systems should be conducted.
Acknowledgements
This study was supported by Fundação de Amparo à Pesquisa do Estado de São Paulo – FAPESP, which provided a grant (proc. 01/05098-1) and a Ph.D. scholarship to C. J. B. Oliveira (proc. 01/05099-8). The authors are grateful to Dr Sueli A. Fernandes and Dr Ana T. Tavechio from the Instituto Adolfo Lutz, São Paulo – SP for the serotyping of Salmonella isolates and providing strains, and to Dr Jalusa D. Kich from Emprapa Suínos e Aves, Concórdia – SC, for performing the ELISA test. We are indebted to Dr Stefan Schwarz and Geovana B. Michael from the Institute of Animal Breeding, Federal Agricultural Research Centre (FAL) in Neustadt-Mariensee, Germany, for kindly providing reagents and technical support for plasmid profiling and PFGE analyses of isolates.