Introduction
Cover crops play an important role in arable organic cropping systems because they improve soil fertility parameters such as soil organic matter, soil structure, nutrient availability, and soil organism activity. It has also been reported that cover crops can suppress weeds in the period between cash crop growing (named ‘post-harvest period’), particularly if they establish quickly, have high growth rates, provide good soil coverage and produce ample biomass, all typical attributes of leafy and tall-growing species and varieties (Dorn, Jossi and van der Heiden, Reference Dorn, Jossi and van der Heiden2015; Teasdale, Reference Teasdale and Zimdahl2018; Osterholz et al., Reference Osterholz, Culman, Herms, Joaquim de Oliveira, Robinson and Doohan2021). Undoubtedly, competitive cover crops can be effective at suppressing the weed canopy in the post-harvest period, but they may not necessarily have an effect on perennial weeds that are detectable in the next growing season.
Perennial weeds still pose a significant problem in organic cropping systems in Northern Europe (Andert et al., Reference Andert, Guguin, Hamacher, Valantin-Morison and Gerowitt2023). Mechanical methods, such as weed harrowing, brush weeding and inter-row hoeing, used directly in the crops have either no or very limited effect against perennial weeds (Melander, Rasmussen and Barberi, Reference Melander, Rasmussen and Barberi2005). Growth of organic crops can be hampered by nitrogen limitations, which can reduce their competitive ability against weeds and allow perennial weeds to proliferate substantially until crop harvest (Rasmussen et al., Reference Rasmussen, Melander, Askegaard, Kristensen and Olesen2014; Melander, Rasmussen and Olesen, Reference Melander, Rasmussen and Olesen2016). Effective control campaigns against perennials are only possible in the post-harvest period where intensive tillage can be applied (Melander et al., Reference Melander, Holst, Rasmussen and Hansen2012). However, the success of these campaigns heavily depends on repetition of tillage events and weather conditions.
Cover crops hinder intensive disintegration and exhaustion of root and rhizome structures through cultivation events whereby belowground propagules can retain their resprouting capacity (Rasmussen et al., Reference Rasmussen, Melander, Askegaard, Kristensen and Olesen2014). Moreover, perennial species such as Sonchus arvensis L. (perennial sowthistle) and partly Cirsium arvense (L.) Scop. (Canada thistle) have endodormant restrictions of bud growth on roots in the post-harvest period (Brandsæter et al., Reference Brandsæter, Fogelfors, Fykse, Graglia, Jensen, Melander, Salonen and Vanhala2010; Andersson et al., Reference Andersson, Boström, Forkman, Hakman, Liew and Magnuski2013; Boström et al., Reference Boström, Andersson, Forkman, Hakman, Liew and Magnuski2013; Liew et al., Reference Liew, Andersson, Boström, Forkman, Hakman and Magnuski2013) and insignificant production of new aboveground shoots exposed to suppression from cover crops. Only older shoots that have established in the preceding main crop are prone to suppression from cover crops that can prevent a further build-up of food reserves in the belowground propagules. The studies of Rasmussen et al. (Reference Rasmussen, Melander, Askegaard, Kristensen and Olesen2014) and Melander, Rasmussen and Olesen (Reference Melander, Rasmussen and Olesen2016) did not identify any suppressive effects on perennial weeds of cover cropping in a long-term organic crop rotation experiment conducted at three Danish sites when analyzing the dynamics of C. arvense and Elytrigia repens (L.) Nevski (quackgrass) over the course of 11–12 yrs. At one site, C. arvense responded indifferently to cover crops, while at another site E. repens was actually promoted by cover cropping and the associated absence of tillage to weaken rhizome growth. The third site at Foulumgaard, which had the highest soil fertility, had no outbreaks of perennial weeds even 12 yrs after the experiment began. (The long-term experiment at Foulumgaard is the one analyzed in this study).
The establishment of cover crops in a Scandinavian climate is not always successful (Kumar et al., Reference Kumar, Thomsen, Eriksen, Vogeler, Mäenpää and Hansen2023), but undersowing the cover crop in the main crop in spring is usually most effective. The cover crop stand can be thin with little ground cover, and a lack of rain or harsh weather conditions may slow autumn growth. Competition for light is probably the primary factor when cover crops and weeds interact in North European conditions. The addition of a suppressive attribute other than a shadowing canopy might improve the overall suppressive ability of cover crops against weeds. Forage plantain (Plantago lanceolata L.) used in grazed pastures contains active secondary metabolites that can inhibit nitrification and reduce nitrification rates, and consequently N2O emission (a greenhouse gas) and NO3 leaching from soils (Skiba et al., Reference Skiba, George, Baggs and Daniell2011; Simon et al., Reference Simon, de Klein, Worth, Rutherford and Dieckow2019). Furthermore, P. lanceolata when in a grass-clover mixture, for example, can increase the standing root biomass of the mixture (Cong et al., Reference Cong, Jing, Rasmussen, Søegaard and Eriksen2017) and thereby enhance soil nutrient exploitation. However, whether changes in nitrogen dynamics in the soil following the inclusion of P. lanceolata in cover crop mixtures can affect the growth of perennial weeds is unclear.
The situation with insignificant growth of perennial weeds in the organic crop rotation experiment at Foulumgaard lasted until 2009, but afterwards outbreaks of several perennial weed species were observed (Melander, personal observations). The objective of the present study was to describe and explain the dynamic behavior of perennial weeds at Foulumgaard in the period 2011–2022 not previously reported, and in particular to focus on the effects of cover crops on the growth of perennial weeds. The data from Foulumgaard are unique by coming from up to 25 yrs of cultivation of two organic crop rotations with and without cover crops, providing enough time to expect solid interpretations of the responses of perennial weeds to cover cropping (Osterholz et al., Reference Osterholz, Culman, Herms, Joaquim de Oliveira, Robinson and Doohan2021). As an additional study, the importance of mixing P. lanceolata into the cover crop mixture was also studied in the experiment in the period 2020–2022. The objective of this additional study was to investigate whether P. lanceolata affects the growth of perennial weeds and whether its effect lasts into the following growing season.
Materials and methods
Crop rotation experiment
Assessments of perennial weed growth were undertaken from the organic crop rotation experiment at Foulumgaard (56°30′N, 09°35′E) in Central Jutland, Denmark. Several papers treating different cropping subjects have been published from this experiment (e.g. Olesen et al., Reference Olesen, Hansen, Askegaard and Rasmussen2007, Reference Olesen, Askegaard and Rasmussen2009; Melander, Rasmussen and Olesen, Reference Melander, Rasmussen and Olesen2016, Reference Melander, Rasmussen and Olesen2020; De Notaris et al., Reference De Notaris, Rasmussen, Sørensen and Olesen2018). The experiment commenced in 1996 and the design is explained in more detail in Olesen, Askegaard and Rasmussen (Reference Olesen, Askegaard and Rasmussen2000). Melander, Rasmussen and Olesen (Reference Melander, Rasmussen and Olesen2016) described the dynamics of perennial weeds in the period 1997–2008 and Rasmussen et al. (Reference Rasmussen, Askegaard, Olesen and Kristensen2006) analyzed the annual weed flora during the first course of the rotations (1997–2000) and found no major weed proliferation in the first four years, mainly thanks to physical weed control interventions. Melander, Rasmussen and Olesen (Reference Melander, Rasmussen and Olesen2020) estimated weed seed bank sizes after 19 yrs (1997–2015) and revealed that the crop rotation including grass-clover for green manuring was particular effective at reducing the seed bank size.
Two organic crop rotations were established in 1997. The major difference between the rotations was that one (O2) mostly had a one-year green manure crop (grass-clover) while the other (O4) had an annual grain legume crop. The purpose of the legumes in O2 and O4 was to provide N input through biological N fixation (Pandey et al., Reference Pandey, Li, Askegaard and Olesen2017). The experiment had three experimental factors: (i) crop rotation [O2 and O4], (ii) cover crop [with (+CC) and without (−CC)], and (iii) manure [with (+M) and without (−M)] combined into six treatments as O2/+M/+CC, O2/+M/−CC, O2/−M/+CC and O4/+M/+CC, O4/+M/−CC, O4/−M/+CC, all arranged in a complete factorial design with a plot size of 12 × 18 m. The experiment was designed with only two blocks (replicates). Although more replicates would have provided greater statistical power, land limitations and financial costs prevented additional replication. Priority was given in the design of the experiment to include relevant treatments and to have all four crops in the rotations present every year. In addition to crop rotation treatments O2 and O4, only the manured treatments (+M/−CC, +M/+CC) were included in the present study of temporal dynamics of perennial weeds. The crops grown in both crop rotations O2 and O4 from 1997 to 2009 are explained in Melander, Rasmussen and Olesen (Reference Melander, Rasmussen and Olesen2016, Reference Melander, Rasmussen and Olesen2020). The crop compositions of O2 and O4 for the period 2010–2022 are given in Table 1. In 2010–2014, crop rotation O2 had a legume-based green manure crop lasting for two years before being terminated. Crop rotation O4 still had a grain legume either solely or in mixture with spring barley, but during 2011–2014 hemp (Cannabis sativa L.) was also grown (Table 1).
Table 1. The cash crops grown in the four entry points of crop rotations O2 and O4 at Foulumgaard in the period 2010–2022

‘+M’ denotes actual manure N applications (kg total-N ha−1), with target N rates in parentheses for each crop. Crops succeeded by a cover crop (CC) are shown with a ‘+’.
Each crop rotation had four crop sequence entry points (Table 1), meaning that sampling on the temporal dynamics of perennials was performed in 32 plots in total (2 rotations × 2 cover crop treatments × 4 entry points × 2 blocks = 32 plots). Due to the original experimental design, four crops were represented each year, but with all the plots receiving the full crop sequence for four (2015–2018 and 2019–22) or five years (2010–2014). In rotation O2, the crops were spring wheat (Triticum aestivum L.), potato (Solanum tuberosum L.), spring oat (Avena sativa L.), winter rye (Secale cereale L.) and spring barley (Hordeum vulgare L.) with undersown lucerne (Medicago sativa L.) or grass-clover [a mixture consisting mainly of perennial ryegrass (Lolium perenne L.), white clover (Trifolium repens L.) and red clover (Trifolium pratense L.)]. The crops in rotation O4 were spring barley, spring oat, winter rye, spring wheat, potato, faba bean (Vicia faba L.), hemp and mixtures of peas (Pisum sativum L.) and spring barley, termed ‘pea:barley’, or mixtures of lupine (Lupinus luteus L.) and spring barley, termed ‘lupine:barley’. All cropping procedures followed Danish guidelines for organic farming. The lucerne and grass-clover were treated in two ways:
1) In the treatments receiving animal manure (+M), the lucerne and grass-clover were cut 3–4 times per year and the plant materials removed simulating harvest for biogas treatment. The concept was to use the cuttings for biogas production and return an equivalent amount of N in digestate to the cropping system (Brozyna et al., Reference Brozyna, Petersen, Chirinda and Olesen2013). The cuttings were thus regarded as a mobile fertilization source meant for distribution at farm level, for example to other fields within the crop rotation. Finally, the green manure crop was incorporated by moldboard plowing in spring at the time of termination.
2) In the treatments without animal manure (−M), lucerne and grass-clover were cut 3–4 times per year and mulched by leaving the plant materials on the soil surface. Finally, the green manure crop was incorporated by moldboard plowing in spring at the time of termination.
For the remainder of this paper, the lucerne and grass-clover crops are referred to as ‘green manure crops’.
Cover crops were either undersown in May or after the harvest of potato, winter rye and the main crop in cases where perennial weeds needed to be controlled by stubble cultivation. Moldboard plowing in spring was the primary tillage method to incorporate cover crop biomass and prepare the soil before sowing the main crop except before winter rye where plowing was done in autumn. In the period 2010–2018, a cover crop mixture of perennial ryegrass (Lolium perenne L.), chicory (Chicorium intybus L.), white clover and red clover was used for undersowing in both rotations O2 and O4, whereas a mixture of winter rye (Secale cereale L.), winter vetch (Vicia villosa Roth) and winter oilseed rape (Brassica napus L.) was used for post-harvest establishment. In the period 2019–2022, a cover crop mixture of perennial ryegrass, chicory and red clover was used for undersowing in spring barley in rotation O4, a cover crop mixture of perennial ryegrass and chicory for undersowing in lupine:barley in rotation O4, and a cover crop mixture of fodder radish (Raphanus sativus L. var. Oleiformis), winter vetch, purple tansy (Phacelia tanacetifolia Benth.) and spring vetch (Vicia lathyroides L.) in rotations O2 and O4 for post-harvest establishment.
Animal manure was applied in the treatments receiving manure (+M) at the N rates shown in Table 1. Manure was not applied to green manure crops, grain legume crops or grain legume:cereal mixtures.
Experiment on the effects of P. lanceolata in the cover crop mixture
The study on the effects of P. lanceolata in the cover crop mixture was conducted in rotation O4 with grain legumes. Plantago lanceolata was mixed into the cover crop blend consisting of perennial ryegrass, chicory, and red clover at a rate of 1 kg ha−1. (The seed rate was higher than the one used in some Danish commercial cover crop mixtures where only 200 g ha−1 of P. lanceolata seeds are sown [www.dlf.dk]). The cover crop blend with P. lanceolata was undersown in plots in spring barley in 2020 in entry point 3 (Table 1). More specifically, the cover crop mixture with P. lanceolata (CCP) was placed in one subplot, while the same mixture without P. lanceolata (CC) was established in another subplot within the same plot. In 2021, P. lanceolata was again established (1 kg ha−1) in the same subplots as in 2020, but now undersown in subplots in the lupine:barley crop in entry point 3 (Table 1). The cover crop mixture for lupine:barley consisted of perennial ryegrass and chicory. In addition, and also in 2021, P. lanceolata was established in subplots in spring barley in entry point 4 using the same cover crop mixture as in 2020. This arrangement allowed an analysis of the carry-over effects of having P. lanceolata for one and two years in a row, respectively, when analyzing the effects on perennial weed growth in 2022. All treatments with P. lanceolata were made in cover crop plots (CC) with (+M/+CC) and without manure (−M/+CC) application.
Management of perennial and annual weeds in the crop rotation experiment
Weed harrowing and occasionally inter-row hoeing were applied in cereals and grain legumes for the control of annual weeds in 2010–2014. From 2015, all cash crops were constantly established at inter-row spaces sufficiently wide to allow for inter-row hoeing. On occasion, this was supplemented by weed harrowing.
Potato ridges were cultivated once or twice close to crop emergence using a rotary cultivator to control annual weeds and build up the ridges. However, perennial weeds were also hampered by this treatment, and the harvest of potato tubers in particular caused considerable uprooting of E. repens rhizomes that were subsequently removed from the plots. Post-harvest cultivation was generally conducted in plots after the grain legume crops, cereal crops (other than spring barley with undersown green manure crop or cover crops), potato crops and when abandoning the green manure, in plots without cover crops and occasionally also with cover crops, as mentioned above. Decisions to employ post-harvest cultivations were based on a visual assessment of the weed infestation of each plot. Post-harvest cultivations were followed by moldboard plowing to 20 cm soil depth in spring. A stubble cultivator with goosefoot shares mounted on vibrating S-shaped tines slicing the soil across the full working width was generally used. However, in a few cases a power rotary cultivator or shallow moldboard plowing to 15 cm depth, intensifying root and rhizome fragmentation and uprooting, was used before stubble cultivation. In general, post-harvest tillage was more intense in rotation O4 because the green manure crops and barley with an undersown ley in O2 prevented cultivation.
Assessment of perennial weeds in the crop rotation experiment
Aboveground biomass of perennial weeds was recorded at the end of June for all crops and for the years 1997–2022, except for the green manure crops in O2. Biomass samples were not taken in 2010. Three 0.25 m2 quadrats were randomly placed in each plot, and all aboveground plant material within each quadrat was cut at ground level. Perennial weeds were separated from other plant material, and the biomass was oven-dried for 24 h at 80°C to obtain dry matter (DM). In addition, all plots were visually scored in late July each year in the period 2012–2021 for the occurrence of perennial weeds at species level. A scale from 0 to 10 was used to score the infestation level of the tall growing species C. arvense, S. arvensis and E. repens, where 10 was given in cases where the whole plot was completely covered by the species considered. All scoring was undertaken by the same person in all years to avoid biases from using different scorers. The whole plot was inspected thoroughly by looking at the plant canopy from above and horizontally before making the score.
Assessment of perennial weeds and cover crops in the study on P. lanceolata
Perennial weeds were counted at species level in three 0.5 m2 quadrats per subplot in early August 2020, 2021, and 2022. Cover crop growth was recorded in early November 2020 and 2021 by cutting all aboveground plant materials at ground level to obtain biomasses of cover crop, P. lanceolata (only in 2021), perennial weeds, annual weeds, and volunteers.
Data analyses
Perennial weed biomass in the period 2011–2022 was analyzed using a generalized linear mixed model with a Gaussian distribution of errors. A similar model was used to analyze scorings of the abundance of perennials (2012–2021), but with a Poisson distribution of errors. Fixed effects were crop rotation, entry point, cover crop, and two-way and three-way interactions. Random terms were year, block and year × block. Both the data on biomass and scoring represented time series with recordings made over time in the same plots. This was accounted for by including year as a repeated effect and plot as the subject, with the assumption of an autoregressive correlation structure and variance between years.
The data on weed counts and cover crop and weed biomasses in the study on P. lanceolata in the cover crop mixtures were also analyzed using a generalized linear mixed model; with a normal distribution of errors for biomasses and a Poisson distribution of errors for count data. Analyses were made year-wise and the fixed effects were entry point, manuring and cover crops with (CCP) or without (CC) P. lanceolata. Random effects were block and plot.
The parameters of the linear models were estimated using residual likelihood estimations. The models were simplified by excluding non-significant effects based on likelihood ratio tests and Akaike's information criterion (Akaike, Reference Akaike1974). Calculations on discrete data (scorings and counts) were made with the glimmix procedure of SAS that features a Poisson distribution (SAS release 9.4, SAS Institute Inc., Cary, N.C.). For the non-discrete data (weed biomasses), calculations were performed using the mixed procedure of SAS, with means calculated as least square means (LSM). Pair-wise comparisons between LSMs were based on t-tests, with probability values adjusted according to the Tukey method. The denominator degrees of freedom (DDF) in F-tests and t-tests for mean separations were calculated according to Kenward and Roger (Reference Kenward and Roger1997).
Biomass data were log-transformed while a square root link-function was used for all discrete data (scores and counts). In all cases following visual inspection of the residuals.
Results
The dynamic behavior of perennial weeds 2011–2022
The general population with perennial weeds increased from 2011, reaching a higher infestation level for the 2011–2022 period compared with the 1997–2009 period (P < 0.0001) (Fig. 1). The perennial weed flora was dominated by S. arvensis, C. arvense and E. repens. Outbreaks of T. farfara, Artemisia vulgaris L. (common wormwood), Mentha arvensis L. (wild mint), Cirsium vulgare (Savi) Ten (bull thistle), Stachys palustris L. (marsh hedgenettle) and Equisetum arvense L. (field horsetail) were also observed, but the numbers of each species were not sufficient for the performance of robust analyses.
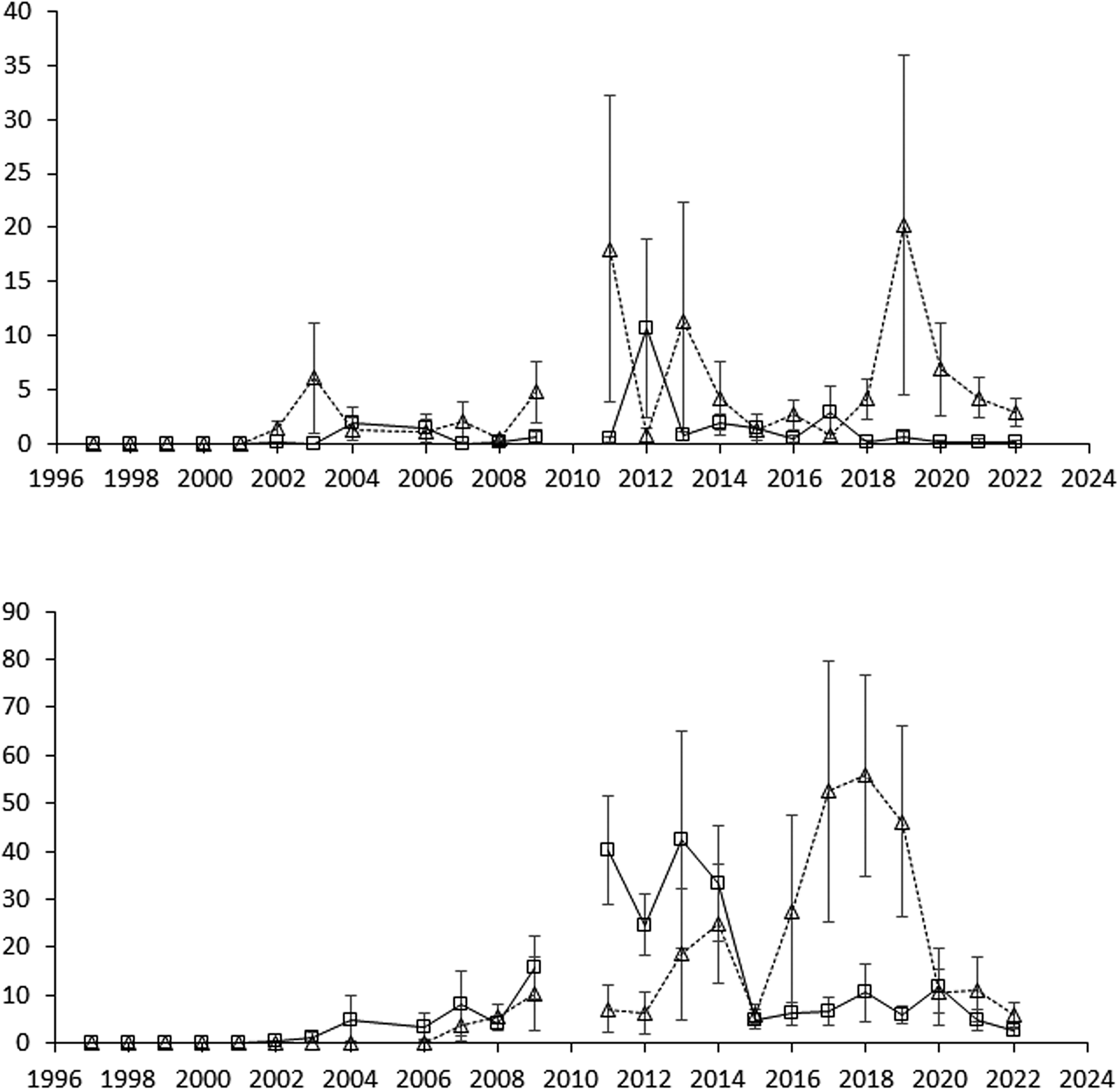
Figure 1. The development of perennial weed biomass in total from 1997 to 2022, shown as means for rotations O2 and O4 with and without cover cropping but across entry points. Error bars show standard errors of means (n = 8).
Entry point had generally negligible effects in the analyses, and consequently was excluded from the models (Table 2). Crop rotation O4 was generally more infested with perennials than O2 in the 2011–2022 period, both when assessing the total population by biomass (2011–2022) and when scoring (2012–2021) (Table 2 and Figs. 1 and 2). Cover cropping interacted with crop rotation, showing that the infestation level was greater with cover crops in rotation O2 than without cover crops, while there was no difference between the two levels of cover cropping in rotation O4 (applying to assessments of both scoring and biomass).
Table 2. Analyses of the effects of crop rotation, cover crop and their interaction on total perennial biomass (g m−2) and scores of Sonchus arvensis, Cirsium arvense, Elytrigia repens and the three species in total respectively
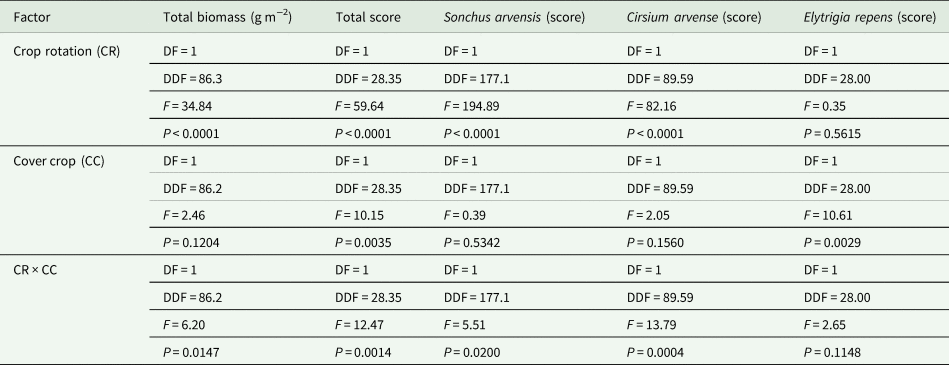
DF, degrees of freedom; DDF, denominator degrees of freedom; F, F-value; P, probability.
In the analyses, biomass data were normally distributed following log-transformation, while all discrete data on scoring followed a Poisson distribution using a square root link-function. The analyses on biomass data reflected the period 2011–2022, while data on scoring covered the period 2012–2021.
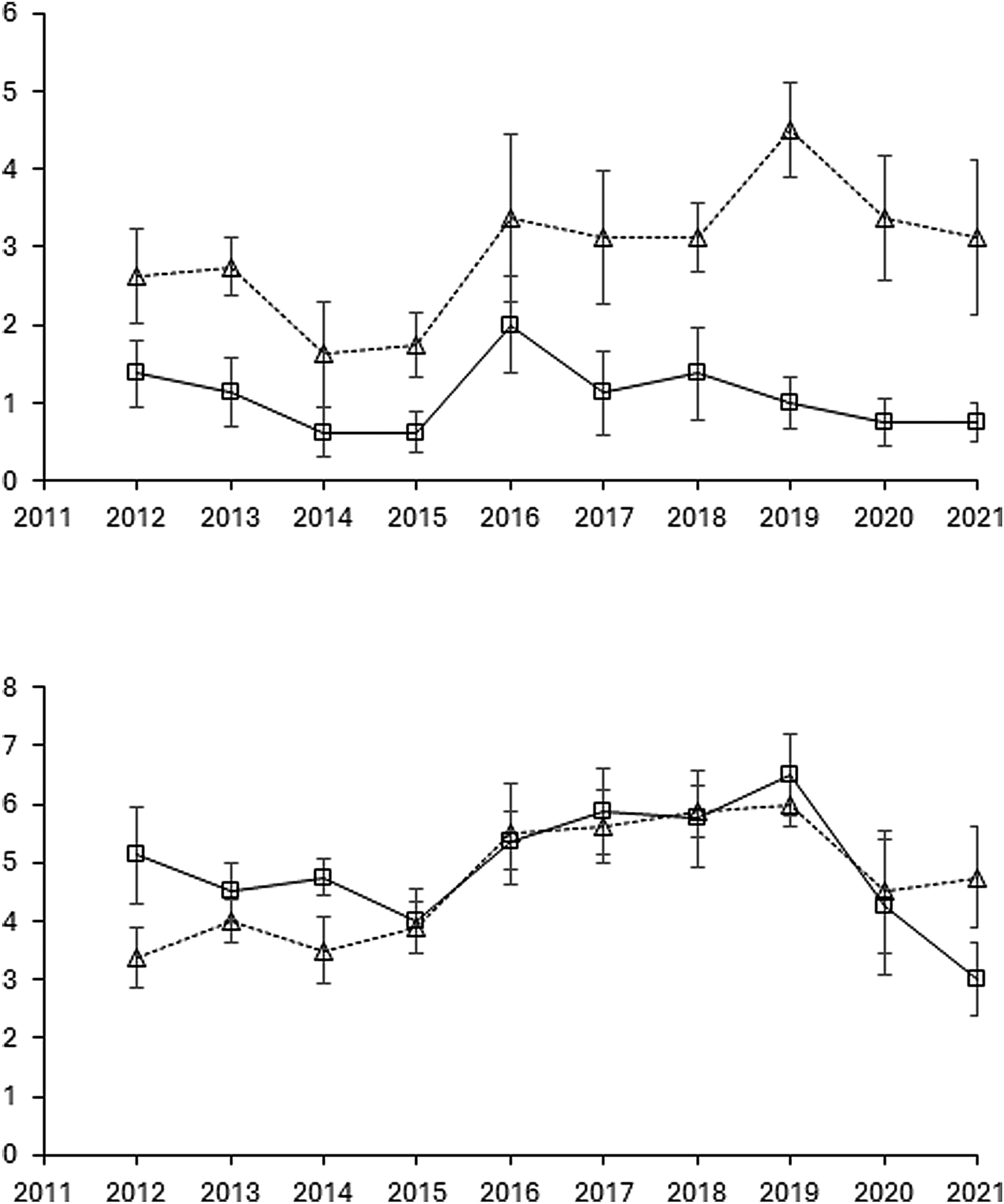
Figure 2. The development of perennial weeds in total (Sonchus arvensis, Cirsium arvense, and Elytrigia repens) from 2011 to 2021 based on visual scoring and shown as means for rotations O2 and O4 with and without cover cropping, but across entry points. Error bars show standard errors of means (n = 8).
In rotation O2 and in the period 2011–2014, the cash crop potatoes generally had more perennial weeds in total based on scoring than the other crops grown (P < 0.001, data not shown), while differences between the other crops within cover crop level (+CC, −CC) were minor. Differences in total infestation levels with perennial weeds were less pronounced between cash crops in rotation O4 across cover-crop level in the same period. In the four-year rotation cycle 2015–2018, green manure crops suppressed the growth of perennial weeds significantly more (based on scorings) than any other crop in rotation O2 (P < 0.01, data not shown). Differences between the other crops within cover-crop level were minor and non-significant in O2 in the same period. No differences were seen between crops in rotation O4, irrespective of the cover-crop level. Responses of perennial weeds similar to 2015–2018 were seen for the last four-year rotation cycle (2019–2022).
The dynamic behavior of perennials was largely driven by S. arvensis, C. arvense and E. repens, although S. arvensis was not affected by cover cropping (Table 2 and Figs. 3 and 4). The infestation score with C. arvense was significantly (P < 0.05) lower without cover crops in rotation O2, whereas cover crops had no effect in O4 across the period 2012–2021 (Fig. 3). However, treatment −CC (no cover crops) had more C. arvense infestation (P < 0.05) when looking specifically on the period 2012–2014. E. repens was not affected by crop rotation, but was strongly affected by cover crop, particularly in rotation O2 (Table 2) where E. repens scores were markedly greater with cover crops compared to without cover crops (Fig. 4).
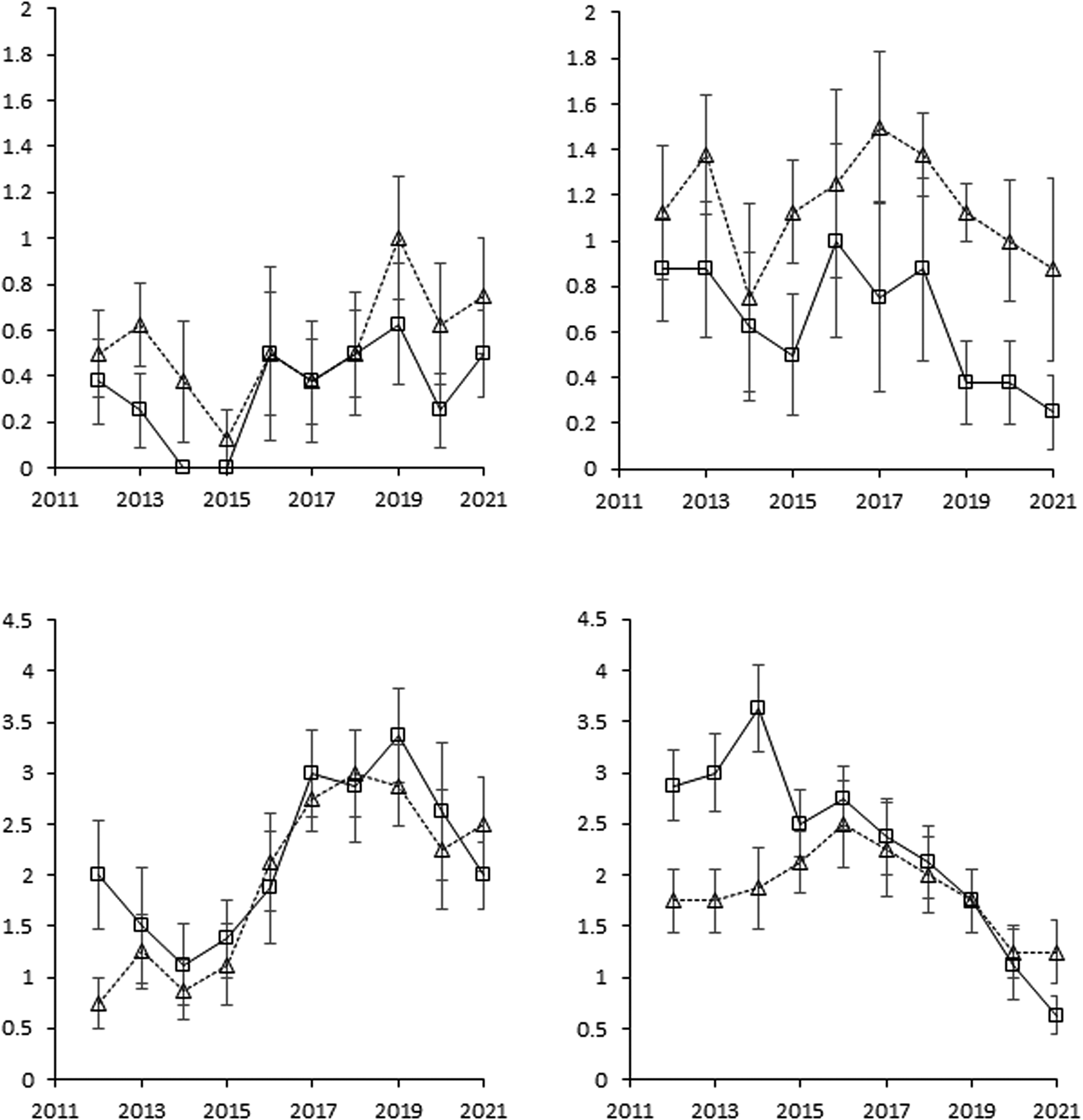
Figure 3. The development of Sonchus arvensis and Cirsium arvense from 2011 to 2021 based on scoring and shown as means for rotations O2 and O4 with and without cover cropping, but across entry points. Error bars show standard errors of means (n = 8).
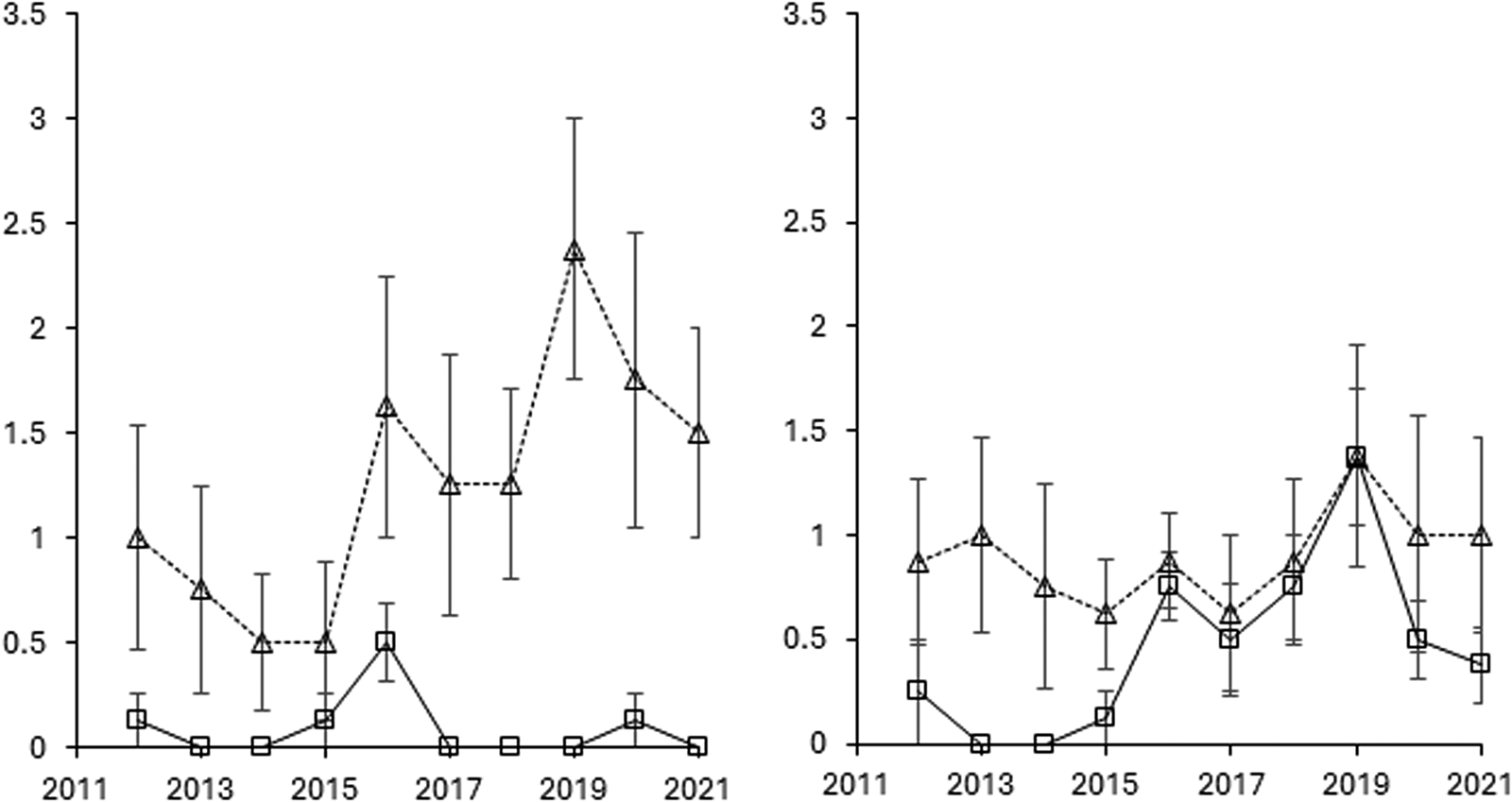
Figure 4. The development of Elytrigia repens from 2011 to 2021 based on scoring and shown as means for rotations O2 and O4 with and without cover cropping, but across entry points. Error bars are standard errors of means (n = 8).
Cover crops with and without P. lanceolata
The cover crop treatment with P. lanceolata (CCP) in 2020 did not affect the biomass production of the cover crop in total, perennial weeds, annual weeds, or volunteers, respectively, in early November 2020 when compared with CC without P. lanceolata (data not shown). The main effects of the factor P. lanceolata (CCP and CC) and its interaction with manuring were not significant in the analyses of biomasses. Perennial weeds were almost absent in the biomass samples, which reflected the very low initial shoot numbers of perennials assessed in early August 2020 (data not shown). Shoot numbers of perennials counted again in early August 2021 were still low and were unaffected by P. lanceolata or manuring. Treatment effects averaged 0.0 (±0.00) – 1.3 (±0.86) shoots m−2, with standard errors shown in parentheses.
Cover crop biomasses recorded in early November 2021 were generally lower than in 2020 (roughly 100 vs 50 g m−2), but with 10% more biomass produced in CCP (main effect P < 0.01). The factor P. lanceolata did not interact with fertilization or duration, irrespective of whether cover crop mixtures with P. lanceolata had been grown only in 2021 or for two years in a row (2020 and 2021). The other plant biomasses (perennial weeds, annual weeds, and volunteers) were negligibly affected by P. lanceolata and its interactions with fertilization and duration (data not shown). However, shoot counts of perennials in total in early August in 2022 revealed a suppressive effect of P. lanceolata that was particularly prevalent where manure had been applied (Table 3). This effect was mainly seen for S. arvensis.
Table 3. Inverse link transform to least square means (LSMILINK) with inverse standard errors in parentheses of total shoots (no m−2) of perennial weed species counted in 2022 and shown for the effects of duration (entry point, one or two years with P. lanceolata), P. lanceolata (CCP/CC) and the interaction between fertiliser (−M/ + M) and P. lanceolata (CCP/CC)
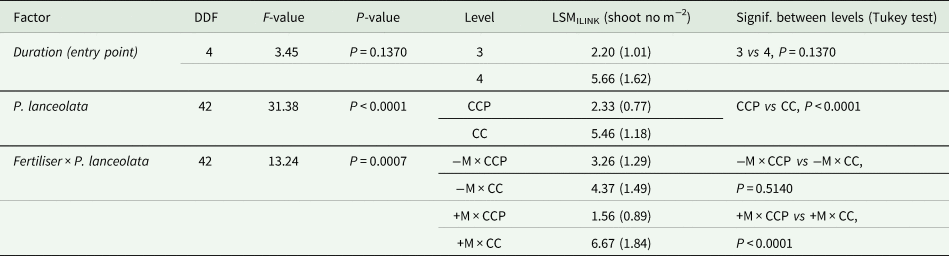
DDF, denominator degrees of freedom; P, probability; CCP, cover crop mixture with P. lanceolata; CC, cover crop mixture without P. lanceolata; −M, without animal manure; +M, with animal manure.
Discussion
The dynamics of perennials 2011–2022
The results of this study reflect several years of cultivation and are in line with previous studies by Rasmussen et al. (Reference Rasmussen, Melander, Askegaard, Kristensen and Olesen2014) and Melander, Rasmussen and Olesen (Reference Melander, Rasmussen and Olesen2016). Overall, cover cropping with its limitations in post-harvest tillage did not provide long-lasting suppression of perennial weeds in the organic crop rotations studied (Figs. 1 and 2). Indeed, E. repens can be promoted by cover crops, as seen in rotation O2, because green manure crops and barley with undersown cover crops prevent the employment of intensive post-harvest tillage campaigns to combat E. repens (Fig. 4). E. repens sprouts easily from rhizomes during the post-harvest period and is sensitive to repeated cultivation events depleting storage sugars in the rhizomes (Brandsæter et al., Reference Brandsæter, Fogelfors, Fykse, Graglia, Jensen, Melander, Salonen and Vanhala2010). Nonetheless, if cover cropping had been compared with an untilled post-harvest period without cover crops, perennial weed populations would probably have been lower with cover cropping. Competition from the cover crops would then have hampered the growth of aboveground shoots and thereby reduced the upload of storage sugars to the rhizome and root structures.
Cirsium arvense and S. arvensis grow less readily in the post-harvest period in Scandinavian climates (Andersson et al., Reference Andersson, Boström, Forkman, Hakman, Liew and Magnuski2013; Brandsæter et al., Reference Brandsæter, Fogelfors, Fykse, Graglia, Jensen, Melander, Salonen and Vanhala2010; Liew et al., Reference Liew, Andersson, Boström, Forkman, Hakman and Magnuski2013). Post-harvest cultivation against species with seasonal growth restrictions has a limited effect (Brandsæter et al., Reference Brandsæter, Mangerud, Andersson, Børresen, Brodal and Melander2020, Andersson et al., Reference Andersson, Boström, Forkman, Hakman, Liew and Magnuski2013) and may even cause an increase, as seen for S. arvensis (Melander et al., Reference Melander, Holst, Rasmussen and Hansen2012). Nevertheless, cultivation prevents the uptake of storage sugars by older shoots that have survived from the main crops, presumably reducing the perennials regenerative capacity. It is not possible in this type of study to unravel further the causes of the effects observed since this would require a more reductionist approach to the experimental design.
The suppressive effects of green manure crops in O2 supplemented by mechanical post-harvest control (O2 without cover crops) may be the main causes of the lack of any noteworthy outbreaks of perennial weeds in this treatment combination during the entire period of 1997–2022 (Fig. 1). The perennial weed population in this study contained most of the problematic perennial weed species, such as C. arvense and E. repens, reported for organic spring cereals in northern Europe (Hofmeijer et al., Reference Hofmeijer, Melander, Salonen, Lundkvist, Zarina and Gerowitt2021). The experimental results should be regarded as the combined effects of treatments and interspecific competition between the individual perennial weed species. For example, when green manure crops with cutting reduce C. arvense and S. arvensis infestations while E. repens is unaffected, more space is left for E. repens growth as also observed by Graglia, Melander and Jensen (Reference Graglia, Melander and Jensen2006). Defoliation by shoot cutting needs to be more frequent and cutting height to be lower to suppress E. repens rhizome growth significantly in a green manure crop (Kolberg et al., Reference Kolberg, Brandsæter, Bergkvist, Solhaug, Melander and Ringselle2018).
Cover crops with and without P. lanceolata
The inclusion of P. lanceolata in the cover crop mixtures reduced the number of perennial weeds in the year following the last period with a cover crop, and the effect was most pronounced where manure had been applied (Table 3). There were no differences in the amounts of aboveground perennial weed biomasses in cover crops with P. lanceolata vs without P. lanceolata assessed at the time when decreasing temperatures normally begin to slow plant growth in November in Denmark. Thus, competition for light, nutrients, and water did not appear to influence the canopy growth of perennial weeds in total during the autumn cover crop phase. Belowground propagules of the perennials, however, seem to have responded to the presence of P. lanceolata, with lower shoot numbers emerging from belowground buds in the following spring of 2022 (Table 3). The present study is not enough detailed to disentangle the mechanisms behind the effects seen with P. lanceolata. This would require more in-depth studies on N dynamics and other ecosystem processes in the post-harvest period.
Conclusions
Green manure crops that are cut and last for one or more years in organic cropping systems are important for the management of perennial weeds with restricted bud growth in the post-harvest period. However, cropping systems should also make room for repeated mechanical weed control in the post-harvest period to target perennial weed species that grow readily and are sensitive to tillage. Cover crops may hinder intensive cultivation strategies and generally do not seem to be a relevant tactic for the suppression of perennial weeds via competition for resources. Cover crops are crucial to the improvement of soil fertility and prevention of nitrate leaching.
A search for cover crop attributes other than fast growth rates, good ground cover and abundant biomass production might improve the potential for cover crops to become more suppressive against weeds with effects that last longer. This study indicates that adding a specific herb (P. lanceolata) to the cover crop blend might weaken the growth of perennial weeds. However, the topic needs considerably more elaboration to assess its potential in organic crop production in future.
Acknowledgements
We would like to thank technicians Erling Nielsen, Eugene Driessen and Karen Bjørn Heinager for their skillful technical assistance.
Author contributions
Bo Melander: Conceptualization, data curation, formal analysis, investigation, methodology, project administration, validation, visualization, writing—original draft, writing—review and editing. Jim Rasmussen: Conceptualization, project administration, funding acquisition, resources, supervision, writing—review and editing. Peter Sørensen: Conceptualization, planning and operating the long-term experiment, resources, supervision, writing—review and editing.
Funding statement
This study was part of the CCRotate project no 34009-19-1559 funded under Organic RDD 5. The grant was provided by the Green Growth and Development Programme (GUDP) under the Danish Ministry of Environment and Food and coordinated by the International Centre for Research in Organic Food Systems (ICROFS).
Competing interests
Authors Bo Melander, Jim Rasmussen and Peter Sørensen declare no conflicts of interest.