Introduction
Weed control is a necessity for corn producers because poor weed control can negatively affect yields. Smith and Scott (Reference Smith, Scott, Espinoza and Kelley2017) demonstrated that one Palmer amaranth plant that goes uncontrolled in corn for 4 wk after emergence can potentially reduce yields by 4%. Therefore, weed competition should be eliminated to allow for maximum yield potential. Weeds can also impede harvest; Bensch et al. (Reference Bensch, Horak and Peterson2003) showed Palmer amaranth can grow up to 2 m tall in less than 40 d, meaning that late-season infestations could result in less than optimal harvest conditions. Whether it is early in the growing season or late, weed control is vital to ensure profitable yields in corn. Troublesome weeds for corn in the southern United States include morningglories (Ipomoea spp.), Texas millet [Panicum texana (Buckley) R. Webster], broadleaf signalgrass [Urochloa platyphylla (Munro ex C. Wright) R. D. Webster], johnsongrass [Sorghum halepense (L.) Pers.], sicklepod [Senna obtusifolia (L.) Irwin and Barneby], nutsedge (Cyperus spp.), and Palmer amaranth (Webster and Nichols Reference Webster and Nichols2012).
In 2016, more than 25 million kg of atrazine were applied in the United States (NASS 2018). Atrazine, a photosystem II (PSII)-inhibiting herbicide, has been the foundation for weed control in corn for over 70 y since its discovery in 1958 (Kramer and Schirmer Reference Kramer and Schirmer2007). PSII-inhibiting herbicides make up Weed Science Society of America herbicide site of action Groups 5, 6, and 7, with the largest portion of PSII-inhibiting herbicides being contained in Group 5. PSII-inhibiting herbicides create oxidative stress to the D1 protein by halting electron flow within the photosynthetic electron transport chain (Aro et al. Reference Aro, Virgin and Andersson1993).
PSII inhibitors act on one of two mechanisms: inactivation and protein damage on the acceptor side or inactivation and damage on the donor side of P680 (Aro et al. Reference Aro, Virgin and Andersson1993). After these mechanisms begin to work, the D1 protein is triggered to begin degradation and is digested by the proteinase of the PSII pathway (Aro et al. Reference Aro, Virgin and Andersson1993). Though each PSII-inhibiting herbicide works by binding with the D1 protein, each group binds somewhat differently.
Atrazine controls an assortment of broadleaf weeds, including common cocklebur (Xanthium strumarium L.), common ragweed (Ambrosia artemisiifolia L.), morningglories, and Palmer amaranth, as well as numerous monocot species (Culpepper and York Reference Culpepper and York1999; Greir and Stahlman Reference Greir and Stahlman1999; Krausz and Kapusta Reference Krausz and Kapusta1998; Sprague et al. Reference Sprague, Kells and Penner1999; Webster et al. Reference Webster, Cardina and Loux1998). Although atrazine can be applied alone, best management practices for slowing resistance evolution suggest using multiple sites of action and residual herbicides (Norsworthy et al. Reference Norsworthy, Ward, Shaw, Llewellyn, Nichols, Webster, Bradley, Frisvold, Powles, Burgos, Witt and Barrett2012). A common addition to atrazine in the Midsouth is mesotrione, which works by inhibiting 4-hydroxyphenylpyruvate dioxygenase, the enzyme that breaks down the amino acid tyrosine, thus hindering weed growth and development (Moran Reference Moran2005). Research has shown that atrazine and mesotrione have synergistic effects when applied together, allowing for broader spectrum weed control (Abendroth et al. Reference Abendroth, Martin and Roeth2006; Sutton et al. Reference Sutton, Richards, Buren and Glasgow2002).
Another herbicide commonly added to atrazine applications is S-metolachlor. This very long chain fatty acid–inhibitor has no POST activity but offers widespread residual control of annual grasses and small-seeded broadleaf weeds (Grichar et al. Reference Grichar, Besler, Brewer and Minton2004). Although there is no documented synergy between S-metolachlor and atrazine, the combination of these two herbicides applied PRE at 1,820 g ha−1 and 1,408 g ha−1, respectively, provided greater than 90% control of giant foxtail (Setaria faberi Herrm.), redroot pigweed (Amaranthus retroflexus L.), and giant ragweed (Ambrosia trifida L.) (Taylor-Lovell and Wax Reference Taylor-Lovell and Wax2001). Combinations of atrazine, mesotrione, and S-metolachlor increase the longevity of use of each of these herbicides by decreasing the risk for target-site resistance evolution.
As discussed previously, atrazine alone and in combination with other herbicides provides corn growers with an unmatched tool for weed control. However, this tool does face potential issues. Numerous reviews have been written supporting the use of atrazine in agriculture as well as addressing its environmental impact (Fan and Song Reference Fan and Song2014; Mudhoo and Garg Reference Mudhoo and Garg2011; Neuberger Reference Neuberger1996; Odukkathil and Vasudevan Reference Odukkathil and Vasudevan2013; Solomon et al. Reference Solomon, Baker, Richards, Dixon, Klaine, La Point, Kendall, Weisskopf, Giddings and Giesy1996; Singh et al. Reference Singh, Kumar, Chauhan, Datta, Wani, Singh and Singh2018). Survey results from Barbash et al. (Reference Barbash, Thelin, Kolpin and Gilliom2006) indicated that atrazine is routinely found in drinking water aquifers and shallow groundwater under agricultural areas, although not at levels considered harmful to humans. Studies have also shown that contamination of groundwater by endocrine disrupters such as atrazine may pose health concerns for the public (Lasserre et al. Reference Lasserre, Fack, Revets, Planchon, Renaut, Hoffmann, Gutleb, Muller and Bohn2009).
Other PSII-inhibiting herbicides, although not as broad spectrum, include ametryn, diuron, fluometuron, linuron, metribuzin, prometryn, propazine, and simazine, among others. Several of these PSII-inhibiting herbicides, including diuron, linuron, metribuzin, and propazine, are generally applied to crops for residual weed control at lower rates than used for atrazine (Shaner Reference Shaner2014). One way to decrease the prevalence of atrazine in groundwater is by reducing the amount used in agriculture, and specifically atrazine use related to corn production. Hence, we tested the tolerance of corn to the aforementioned PSII-inhibiting herbicides alone and in combination with mesotrione and S-metolachlor to determine their potential as replacements for atrazine.
Materials and Methods
Corn Trial Common Methodology
Field experiments were conducted in 2017 and 2018 to test the tolerance of corn to PRE- and POST-applied PSII-inhibiting herbicides. Corn experiments used variety 1197YHR (Pioneer, Johnston, IA 50131), a 111-d maturing, glyphosate- and glufosinate-tolerant hybrid, planted at 79,000 seeds ha−1 into conventionally tilled and raised beds at a 5-cm depth. Plot sizes were 3.7 m wide by 6.1 m long and rows were spaced 91 cm apart. Plots were maintained weed-free with POST applications of glufosinate and glyphosate on an as-needed basis. All corn trials received 56, 73, and 56 kg ha−1 of N, P2O5, and K2O, respectively, before planting and 168 kg ha−1 N when the corn was at V6 (Richie et al. Reference Richie, Hanway and Benson1986). Urea (46-0-0), triple superphosphate (0-45-0), and potash (0-0-60) were the fertilizer sources used. Irrigation in the amount of 2.5 cm was provided via furrow irrigation when 7 d without rainfall in excess of 2.5 cm occurred. Trials were otherwise managed according to the Arkansas Corn Production Handbook (Espinoza and Ross Reference Espinoza and Ross2015).
Experimental Sites
Field experiments were conducted on a Captina silt loam (fine-silty, siliceous, active, mesic Typic Fragiudults) at the Arkansas Agricultural Research and Extension Center in Fayetteville, AR, in 2017 and 2018. The soil at Fayetteville consisted of 34% sand, 53% silt, and 13% clay, with an organic matter content of 1.5% and a pH of 6.8.
PRE Tolerance Study Design and Data Collection
Experiments were designed as a two-factor factorial, randomized complete block, with the factors being (1) PSII-inhibiting herbicide and (2) the herbicide added to create the mixture. The PSII-inhibiting herbicides included ametryn, atrazine, diuron, fluometuron, linuron, metribuzin, prometryn, propazine, and simazine. The second factor consisted of either no herbicide, S-metolachlor, or mesotrione. PSII-inhibiting herbicides were applied at the same rate as they would be applied at in a labeled crop. Herbicide rates and manufacturers are listed in Table 1. All treatments were applied at 140 L ha−1 after corn planting (Table 2). The experimental treatments were replicated four times. Visible crop injury was rated at 14 and 28 d after application (DAA) on a scale of 0% to 100%, with 0% representing no injury and 100% representing crop death (Frans and Talbert Reference Frans, Talbert and Truelove1977). Crop height measurements of three random plants in each plot were measured to the crop canopy, recorded at 28 DAA, and then averaged. Crop density was counted as plants m−1 row 14 DAA. Grain was harvested from the middle two rows of each plot using a small-plot combine, and weights were adjusted to 15.5% moisture and expressed as corn grain yield in kg ha−1.
Table 1. Herbicides, rates, and manufacturers for PRE and POST corn trials in 2017 and 2018 at Fayetteville, AR.
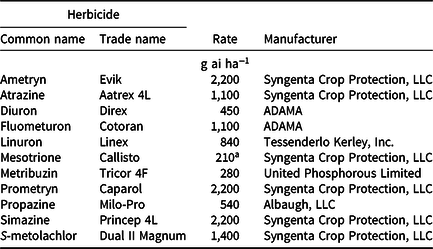
a Applied POST at 105 g ai ha−1.
Table 2. Planting, herbicide application, and harvest dates for PRE- and POST-corn trials in Fayetteville, AR in 2017 and 2018.

POST Tolerance Study Setup and Data Collection
Experiments followed the same treatments and design as the previously discussed PRE trial. However, for the POST experiments, treatments were applied when corn was 30-cm tall (V3 to V4). Visible crop injury was rated at 14 and 28 DAA. Crop height and yield were determined as outlined in the PRE tolerance section.
Statistical Analysis
Data from the trials were analyzed separately by year, given the different planting dates from year to year. All visible estimates of crop injury for the nontreated plots in these studies were zero; thus, the nontreated plots were excluded from the analysis for injury at 14 and 28 DAA. Crop height, crop density, and yield values were converted to be relative to those of the nontreated plots. This was done by dividing the observations for each response variable by the average of the nontreated observations for each respective response variable. Data were then subjected to an analysis of variance using the GLIMMIX procedure in SAS, version 9.4, statistical software (SAS Institute Inc, Cary, NC), assuming a beta distribution for injury assessments and a gamma distribution for all other assessments, to see if the main PSII-inhibiting herbicide, the additive herbicide, or the interaction had an effect (Gbur et al. Reference Gbur, Stroup, McCarter, Durham, Young, Christman, West and Kramer2012). Means were compared for injury, relative crop height, relative crop density, and relative yield using Fisher protected LSD (P = 0.05).
Results and Discussion
PRE Study
Rainfall
The amount and timing of rainfall relative to the PRE applications differed between years (Figure 1). The performance of soil-applied herbicides is affected by numerous factors, including, but not limited to, soil texture, organic matter, and soil moisture (Curran Reference Curran2001; Hartzler Reference Hartzler2002). Given that both experiments were conducted on the same soil texture, with similar organic matter and pH, it is likely that any differences in herbicide performance depended on rainfall timing and rate after herbicide application. Because herbicides applied PRE are taken up through the roots of young, germinating seedlings, 1 to 2 cm of rainfall is required for activation (Rao Reference Rao2000). In 2017, PRE herbicides were applied immediately after planting and received an activating rainfall of 3.5 cm 2 d later (Figure 1). In 2018, PRE herbicides were applied 2 d after planting and received 1.6 cm of rainfall the evening immediately after the application (Figure 1).

Figure 1. Rainfall amounts by day along with corn planting and herbicide application dates at the Arkansas Agricultural Research and Extension Center in Fayetteville, AR, in 2017 and 2018.
Injury
In both years, corn injury 14 DAA was influenced by an interaction of the PSII-inhibiting herbicide and the additive herbicide (P = 0.0305 for 2017; P = 0.0292 for 2018) (Table 3). Injury was in the form of leaf-tip chlorosis with some bleaching of new leaves on plants that received mesotrione-containing treatments. In 2017, applications of ametryn alone, ametryn plus mesotrione, and ametryn plus S-metolachlor caused 9%, 5%, and 7% injury, respectively (Table 4). However, in 2018, ametryn and ametryn plus mesotrione caused no observable injury. Fluometuron-containing treatments caused injury in both years, with fluometuron plus mesotrione causing 10% injury in both years. In 2017, this was the highest injury rate observed for any treatment, but it did not differ from fluometuron alone or ametryn alone. In 2018, fluometuron plus mesotrione injury was higher than that occurring with all other treatments.
Table 3. Significance of P values for interactions and main factors of PSII-inhibiting herbicide and herbicide added on various factors by year for PRE corn trials.

a Abbreviation: DAA, days after application.
b P < 0.05 was considered statistically significant.
Table 4. Average visible estimates of corn injury as influenced by interactions between PSII-inhibiting herbicide and herbicide added applied PRE in Fayetteville, AR, in 2017 and 2018.

a Abbreviation: DAA, days after application.
b Means within a column followed by the same letter are not significantly different according to Fisher protected LSD (P = 0.05).
Corn injury in 2018 was temporary, and by 28 DAA, no differences were detected among treatments. No treatment displayed injury greater than 3% (data not shown). However, corn injury 28 DAA in 2017 was not temporary and was influenced by an interaction of PSII-inhibiting herbicide and the herbicide added (P < 0.0001) (Table 3). In 2017, some plots with injury of 5% or higher 14 DAA did not recover by 28 DAA (Table 4). For example, fluometuron alone, fluometuron plus mesotrione, and fluometuron plus S-metolachlor exhibited 9%, 10%, and 5% injury, respectively, 14 DAA, and then 9%, 16%, and 9% injury, respectively, 28 DAA. However, treatments containing ametryn plus mesotrione, diuron plus mesotrione, prometryn plus mesotrione, and simazine plus S-metolachlor were exceptions to this lack of recovery. Each of these treatments exhibited 5% injury 14 DAA and then exhibited no injury 28 DAA. Overall, injury in both years and at both ratings was less than 20%. Excluding ametryn- and fluometuron-containing treatments, injury was less than 10% at 14 and 28 DAA.
Relative Stand
There was no significant effect for the main effects of PSII-inhibiting herbicide and herbicide added and the interaction (Table 3). Densities in nontreated plots were 8.1 and 7.7 plants m−1 row in 2017 and 2018, respectively (data not shown).
Relative Height
In 2017, corn height was not affected by any factor. Although visible injury symptoms of interveinal chlorosis were not present by 28 DAA in 2018, height was influenced by the PSII-inhibiting herbicides (P < 0.0001) (Table 3). Consistent with injury at 14 DAA, fluometuron-containing treatments, which caused the highest visible injury, also caused the greatest reduction in height (77% of the nontreated plots; Tables 4 and 5). Generally, any PSII-inhibiting herbicide that caused injury 14 DAA reduced height compared with the nontreated plots, except metribuzin- and simazine-containing treatments, which did not reduce height compared with nontreated plots in 2018.
Table 5. Relative corn height as influenced by PSII-inhibiting herbicide applied PRE in Fayetteville, AR, in 2018.

a Height of corn in the nontreated plots averaged 36 cm.
b Means followed by the same letter are not significantly different according to Fisher protected LSD (P = 0.05).
Relative Yield
Although various treatments may have caused visible injury and height reduction in 2017 and 2018, relative yield was not significantly influenced by the main effects of PSII-inhibiting herbicide, herbicide added, or the interaction (Table 3). On average, corn in the nontreated plots yielded 11,000 and 12,510 kg ha−1 for 2017 and 2018, respectively. Corn is a fairly vigorous crop with the ability to recover from early-season injury caused by herbicides. Corn yield components develop at different stages, giving corn the ability to compensate for adverse effects throughout the growing season (Milander Reference Milander2015). Yield components such as kernels row−1, rows ear−1, and kernel weight are each primary yield components that are determined at different times after the V4 growth stage (Fageria et al. Reference Fageria, Baligar and Clark2006). However, ears m−1 is typically correlated with crop density (i.e., plant stands). Because injury in 2017 and 2018 was minimal and, in most treatments, temporary, and because density was not affected, the corn likely was able to compensate for any yield component affected by the herbicides later in the growing season. Curran et al. (Reference Curran, Knake and Liebl1991) found that corn treated PRE with clomazone, chlorimuron, imazaquin, and imazethapyr, although exhibiting injury up to 20%, did not suffer any yield loss. This reinforces that corn treated with PRE herbicides can compensate for early-season injury and still produce optimal yields.
POST Study
Rainfall
Given that corn was already 30-cm tall at application, the herbicides did not need to be activated to provide ideal performance. However, any herbicide that did reach the soil surface would have to be activated before providing residual activity. In 2017, 7.8 and 3.5 cm of rainfall were received 2 and 10 DAA, respectively (Figure 1). In 2018, rainfall events each totaling 1.5 cm were received 2 and 4 DAA (Figure 1).
Injury
In 2017 and 2018, corn injury 14 DAA was influenced by the interaction of PSII-inhibiting herbicide and herbicide added (P = 0.0072 for 2017; P < 0.0001 for 2018) (Table 6). Injury was in the form of leaf-tip chlorosis and necrosis with some bleaching of contacted leaves as well as new growth on plants to which mesotrione-containing treatments were applied. In 2017, linuron plus S-metolachlor caused the highest injury (45%) (Table 7). In general, linuron-containing treatments, along with diuron plus S-metolachlor and prometryn plus S-metolachlor, caused greater injury compared with most other treatments. The Linex (linuron) label (Tessenderlo Kerley, Inc., Phoenix, AZ) does not allow for over-the-top use of the herbicide in corn, because of injury concerns (Anonymous 2017). In 2018, prometryn alone and in combination with S-metolachlor caused 45% and 49% injury, respectively (Table 7). Ametryn plus S-metolachlor, linuron plus S-metolachlor, and prometryn plus mesotrione caused 38%, 38%, and 35% injury, respectively, all of which were comparable. Atrazine-, fluometuron-, metribuzin-, and simazine-containing treatments each caused no more than 15% injury in both years (Table 7).
Table 6. Significance of P values for interactions and main effects of PSII-inhibiting herbicide and herbicide added on various factors by year for POST herbicide study conducted at Fayetteville, Arkansas in 2017 and 2018.

a Abbreviation: DAA, days after application.
b Statistical significance set at P < 0.05.
Table 7. Average visible estimates of corn injury and yield as influenced by interactions between PSII-inhibiting herbicide and herbicide added applied POST in Fayetteville, AR in 2017 and 2018.

a Abbreviation: DAA, days after application.
b Means within a column followed by the same letter are not significantly different according to Fisher protected LSD (P = 0.05).
c Corn yield in 2017 and 2018 averaged 11,000 and 12,500 kg ha−1 in nontreated plots, respectively.
Injury 28 DAA in 2017 was influenced by an interaction between PSII-inhibiting herbicide and herbicide added (P = 0.0009) (Table 6). Linuron plus S-metolachlor caused 29% injury in 2017 and was the most injurious treatment (Table 7). Diuron plus S-metolachlor, linuron plus mesotrione, and prometryn plus S-metolachlor were comparable and caused 17%, 18%, and 18% injury, respectively. No other treatment caused greater than 10% injury in 2017. In 2018, injury 28 DAA was less than 10% (data not shown) and was not affected by PSII-inhibiting herbicide, herbicide added, or the interaction (Table 6). Overall, injury was moderate among treatments in both years, excluding fluometuron-, metribuzin-, and simazine-containing treatments, which caused less than 15% injury (Table 7).
Relative Height
In 2017 and 2018, height 14 DAA was influenced by an interaction between PSII-inhibiting herbicide and herbicide added (P = 0.0051 for 2017; P = 0.0003 for 2018) (Table 6). Generally, height followed the trend of injury. For example, in 2017, linuron plus S-metolachlor resulted in the highest injury rate (45%), and corn height after this treatment was only 77% of that of nontreated plots (Tables 7 and 8). In 2017, plots with greater than 10% injury also had plant heights that were reduced compared with plants in nontreated plots. In 2018, the same was true, excluding plots treated with diuron plus mesotrione and plots treated with propazine alone (Tables 7 and 8). Overall, height 14 DAA generally followed the same trends as injury 14 DAA for a given year.
Table 8. Relative corn height as influenced by PSII-inhibiting herbicide applied POST in Fayetteville, AR in 2017 and 2018.

a Abbreviation: DAA, days after application.
b Means within a column followed by the same letter are not significantly different according to Fisher protected LSD (P = 0.05).
c Height of corn in 2017 and 2018 in the nontreated plots averaged 52 and 46 cm, respectively.
Relative Yield
In 2017 and 2018, relative yield was influenced by an interaction between PSII-inhibiting herbicide and herbicide added (P = 0.0006 for 2017; P < 0.0001 for 2018) (Table 6). Corn in plots treated with ametryn alone, ametryn plus mesotrione, diuron alone, diuron plus mesotrione, metribuzin alone, metribuzin plus S-metolachlor, propazine alone, simazine alone, and simazine plus S-metolachlor had yields comparable to atrazine-containing treatments in 2017 (Table 7). In 2018, corn in plots treated with fluometuron plus mesotrione and S-metolachlor, metribuzin alone, metribuzin plus mesotrione or S-metolachlor, prometryn plus mesotrione, prometryn plus S-metolachlor, and simazine plus mesotrione had yields comparable to corn that receiving atrazine-containing treatments.
These applications were made while the corn was 30-cm tall or at the V3 to V4 growth stage. During this time and subsequent weeks after application, yield components such as kernels row−1 and rows ear−1 were developing (Fageria et al. Reference Fageria, Baligar and Clark2006; Uribelarrea et al. Reference Uribelarrea, Cárcova, Otegui and Westgate2002). Corn hybrid 1197YHR contains a semiflex ear trait, meaning that it has the potential to set a small range of rows ear−1. It is possible the chlorosis and stunting caused by certain herbicides affected the development of these yield components and, therefore, hindered yield in some treatments.
Practical Implications
Determining which herbicides should be tested further to potentially replace atrazine should be based on a combination of visible injury, crop height, and yield. Efforts should be made to avoid herbicides that injure corn beyond a reasonable level, even if yield is not affected, because injury may translate into delayed maturity or increased potential for disease and pest pressure. Therefore, even though yield was not affected for any PRE-applied herbicide, certain ametryn- and fluometuron-containing treatments caused greater than 10% injury and, therefore, no longer should be considered as an atrazine replacement in corn, because safer options were identified.
Although not directly measured, it is possible that herbicides that injured corn or reduced height could delay canopy closure. Any delay in canopy closure would negatively affect weed control (Anderson Reference Anderson2008). Given the negative effects of reduced crop height, prometryn- and propazine-containing treatments should also be eliminated from additional testing. Corn tolerance to diuron-, linuron-, metribuzin-, and simazine-containing treatments applied PRE should be further tested to validate the tolerance observed in this study. Furthermore, weed control trials should also be conducted for these herbicides and herbicide combinations to ensure adequate replacement of atrazine.
The same factors should be considered for POST application of these herbicides. On the basis of crop injury, relative crop height, and relative yield in 2017 and 2018, only metribuzin- and simazine-containing treatments should be further assessed for crop tolerance and weed control when applied POST. Efforts should be made to evaluate these herbicides over as many diverse environments as possible.
Acknowledgements
The authors would like to thank the Arkansas Corn and Grain Sorghum Board for funding this study, and the University of Arkansas System Division of Agriculture for their support. No conflicts of interest have been declared.