Introduction
The Oktyabr'sky deposit plays an important role in solving genetic problems of the unique platinum–copper–nickel ores in the Norilsk region, in addition, it is the largest deposit related to the Kharaelakh layered intrusion, located in the south of the Kharaelakh trough (Fig. 1).
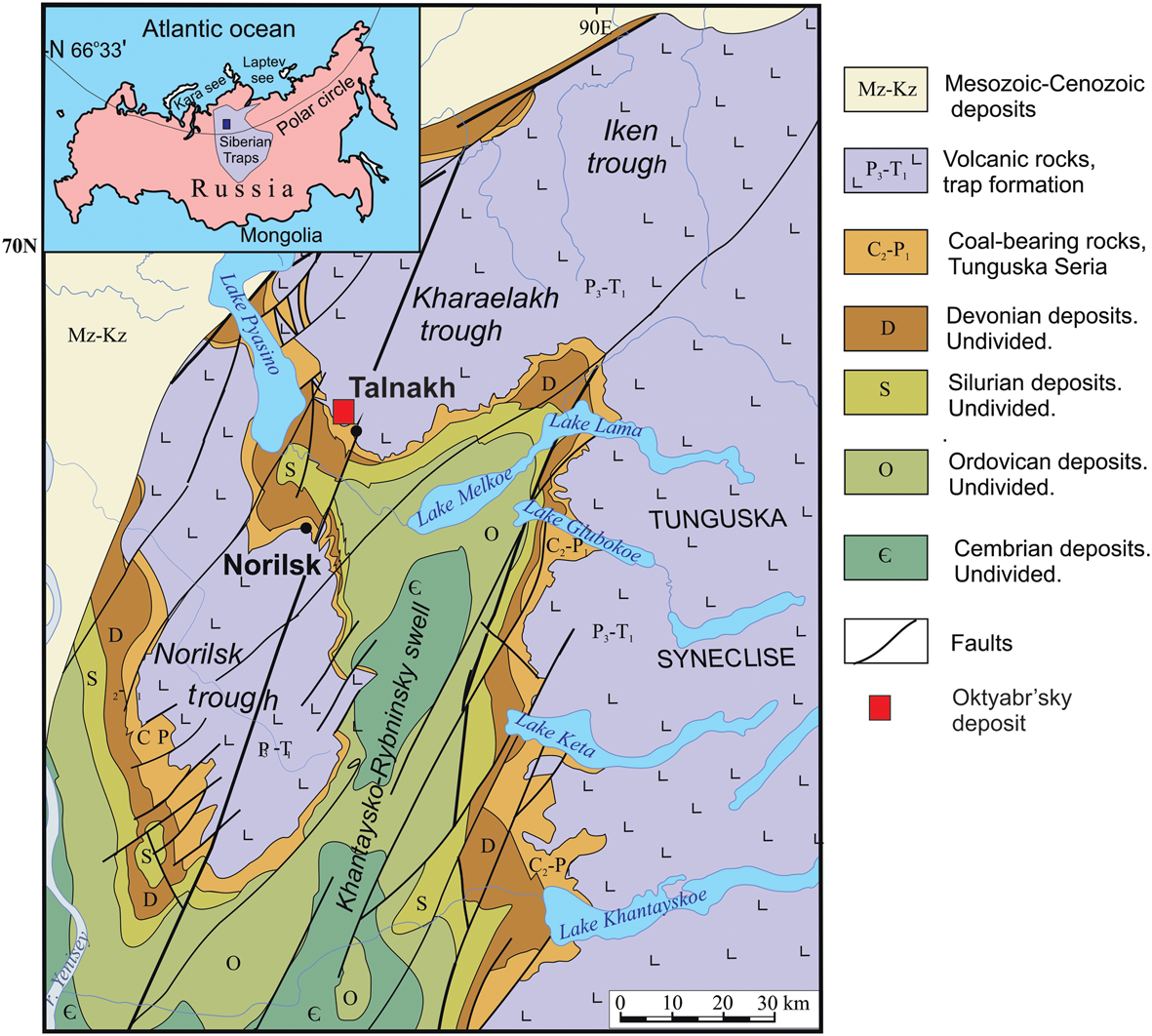
Figure 1. Geological schema of the Noril'sk area (based on Geological map 1:200000, from Strunin, Reference Strunin1994).
The Oktyabr'sky deposit is a collection of at least 15 massive (and overlying disseminated) orebodies or lenses (Dyuzhikov et al., Reference Dyuzhikov, Distler, Strunin, Mkrtychyan, Sherman and Sluzhenikin1992; Stekhin, Reference Stekhin, Lightfoot and Naldrett1994; Torgashin, Reference Torgashin1994). They are variously located close to each other, or separated spatially as in the C-3 and C-4 orebodies, however these orebodies (or lenses) as a whole make up the Oktyabr'sky deposit. A huge amount of analytical data has been obtained for the Main Ore Body – a lode of massive sulfide ore, 4 × 2 km with thickness up to 50 m). The genesis of the Main Ore Body has been the subject of intensive discussions for several decades (Genkin, Reference Genkin1968; Dodin and Batuev Reference Dodin, Batuev and Urvantsev1971; Distler et al., Reference Distler, Genkin, Filimonova, Hitrov and Laputina1975, Reference Distler, Grokhovskaya, Evstigneeva, Sluzhenikin, Filimonova and Dyuzhikov1988, Reference Distler, Kulakov, Sluzhenikin and Laputina1996; Genkin et al., Reference Genkin, Distler, Gladyshev, Filimonova, Evstigneeva, Kovalenker, Laputina, Smirnov and Grokhovskaya1981; Zientek and Likhachev, Reference Zientek and Likhachev1992; Likhachev, Reference Likhachev, Lightfoot and Naldrett1994, Reference Likhachev2006; Naldrett et al., Reference Naldrett, Asif, Gorbachev, Kunilov, Stekhin, Fedorenko, Lightfoot, Lightfoot and Naldrett1994, Reference Naldrett, Fedorenko, Lightfoot, Kunilov, Gorbachev, Doherty and Johan1995, Reference Naldrett, Fedorenko, Shushen, Asif, Kunilov, Stekhin, Lightfoot and Gorbachev1996; Naldrett, Reference Naldrett2004; Sluzhenikin, Reference Sluzhenikin2011; Krivolutskaya et al., Reference Krivolutskaya, Nesterenko, Gongalsky, Korshunov, Bychkova and Svirskaya2018, and many others).
The Main Ore Body has mineralogical zoning: mooihoekite (Cu9Fe9S16) – talnakhite (Cu18(Fe, Ni)18S32) – chalcopyrite (CuFeS2) ore transforms into cubanite (CuFe2S3) ore, and then into pyrrhotite (Fe1–xS) ore from the centre to the flank (Dyuzhikov et al., Reference Dyuzhikov, Distler, Strunin, Mkrtychyan, Sherman and Sluzhenikin1992; Torgashhin, Reference Torgashin1994; Lul'ko et al., Reference Lul'ko, Fedorenko, Distler and Sluzhenikin1994; Naldrett et al., Reference Naldrett, Fedorenko, Lightfoot, Kunilov, Gorbachev, Doherty and Johan1995; Valetov et al., Reference Valetov, Badtiev and Ryabikin2000; Gorbachev, Reference Gorbachev2006; Dodin et al., Reference Dodin, Sluzhenikin and Bogomolov2009). That is, the iron content in the ores increases in this direction. The origin of this ‘reverse’ zoning is one of the main genetic questions. The Main Ore Body cannot be considered as a single lode crystallised in a closed system, as from the point of view of crystallisation differentiation the more fractionated parts of orebodies enriched in Cu and platinum-group elements (PGE), which are incompatible with monosulfide solid solution (Mss), should be located on the periphery (Distler et al., Reference Distler, Genkin, Filimonova, Hitrov and Laputina1975; Zientek et al., Reference Zientek, Likhachev, Kunilov, Barnes, Meier, Carlson, Briggs, Fries, Adrian, Lightfoot and Naldrett1994; Barnes et al., Reference Barnes, Zientek and Severson2011). Experimental (Likhachev and Kukoev, Reference Likhachev and Kukoev1973; Sinyakova et al., Reference Sinyakova, Borisenko, Karmanov and Kosyakov2019) and modelling (Distler, Reference Distler1975; Kalugin and Latypov, Reference Kalugin and Latypov2009) work have confirmed that direct zoning is formed during fractional crystallisation when the more fractionated constituents of sulfide melts are distilled to the periphery.
The horizontal zoning of the Oktyabr'sky deposit is very complex, and reflects the interaction of different-scale genetic factors such as: the separation of the sulfide melt in the intermediate chamber into Fe-rich and Cu-rich fractions that can then be introduced separately (Gorbachev and Nekrasov, Reference Gorbachev and Nekrasov2004; Gorbachev, Reference Gorbachev2006; Likhachev, Reference Likhachev2006); various directions of the flows (streams) of sulfide melts and the pattern of these flows laterally (Stekhin, Reference Stekhin, Lightfoot and Naldrett1994); a single-act or the pulsating nature of their introduction; and the fractionation of the sulfide melt during the flow differentiation, with the distillation of its more cuprous derivatives into the frontal parts.
In comparison, the vertical zoning of orebodies depend on: the degree of fractionation of the sulfide melt in situ and the behaviour of elements that are compatible and incompatible with Mss during its crystallisation; possible degassing of the sulfide melt (Godlevsky, Reference Godlevsky1968; Gorbachev, Reference Gorbachev2006) when surface capillary forces are dominant over gravity (Barnes et al., Reference Barnes, Vaillant, Godel and Lesher2019); and the pulsation mode of incoming melts (Latypov et al., Reference Latypov, Chistyakova and Alapieti2007, Reference Latypov, Hanski, Lavrenchuk, Huhma and Havela2011; Egorova and Latypov, Reference Egorova and Latypov2013).
If we consider the Oktyabr'sky deposit on a large scale, then the influence of the Norilsk-Kharaelakh fault is important for the overall configuration of ore zoning (Stekhin, Reference Stekhin, Lightfoot and Naldrett1994). The zoning of ores is associated primarily with the action of a series of linear flows directed from the fault that provides supply channels for intrusive material (Fig. 2). Each flow is characterised by the distribution of pyrrhotite ores in the root parts and near the fault, and more cuprous ores forming halos around the pyrrhotite ores (Kunilov, Reference Kunilov, Lightfoot and Naldrett1994). The MOB is composed of at least two ore lenses corresponding to the different flows of sulfide melt – pyrrhotite is predominant in the first lens, and a mooihoekite–cubanite–chalcopyrite assemblage is common in the second lens (Torgashin, Reference Torgashin1994; Kalugin and Latypov, Reference Kalugin and Latypov2010, Reference Kalugin and Latypov2012). The vertical zoning of cuprous and pyrrhotite ores are the opposite, i.e. the contents of Cu, PGE and Au increase from the bottom to the top (direct zoning) in cuprous lenses, whereas the contents increase from the top to the bottom in pyrrhotite lenses – reverse zoning (Fig. 3) (Torgashin, Reference Torgashin1994; Gorbachev, Reference Gorbachev2006; Kalugin and Latypov, Reference Kalugin and Latypov2010, Reference Kalugin and Latypov2012).
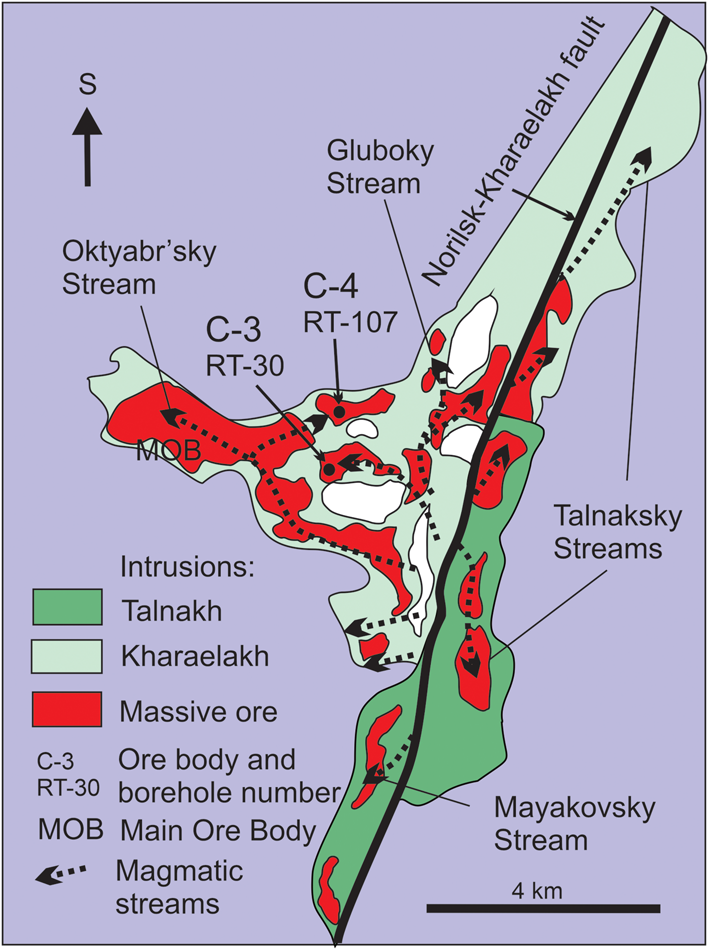
Figure 2. Plan view of the Talnakh ore cluster with contours of massive ore and flow directions of fractionating ore magma. Redrawn from Stekhin (Reference Stekhin, Lightfoot and Naldrett1994).
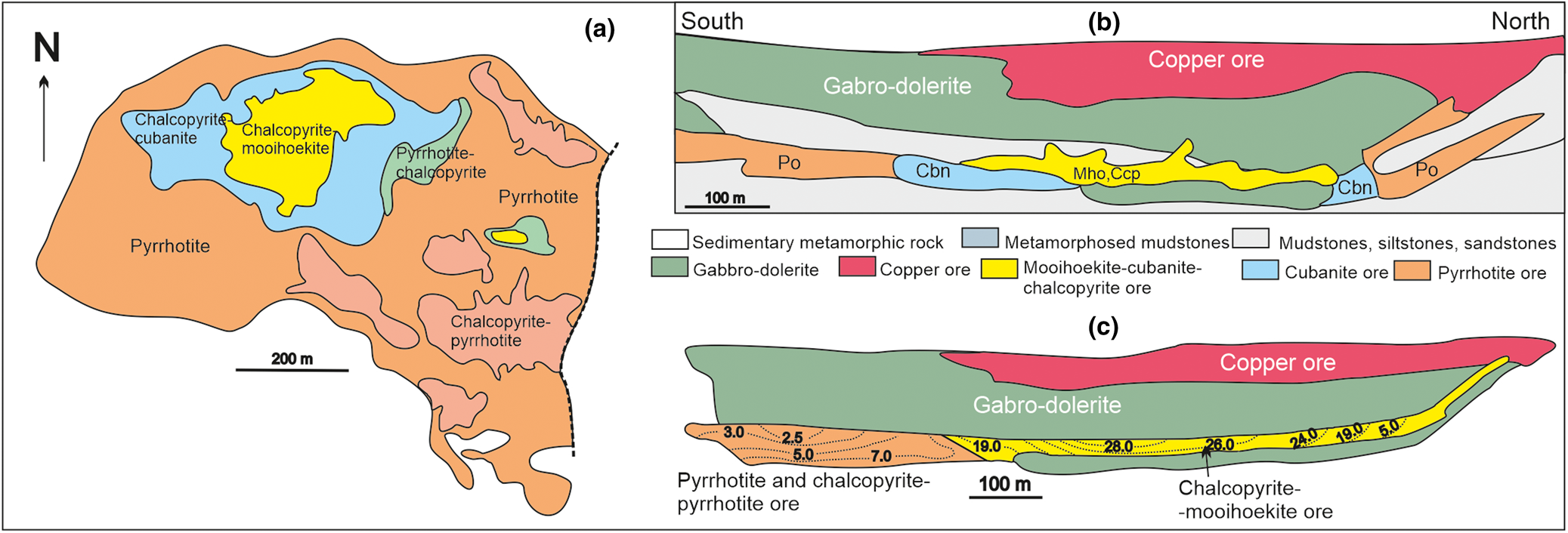
Figure 3. Schematic map of the massive ores (a), and sections (b,c) of the Oktyabr'sky deposit by Torgashin (Reference Torgashin1994) (redrawn with some changes). Details: (b) large scale lateral zoning of massive ores from Cu-rich in the centre of the Main Ore Body to pyrrhotite-rich on the periphery; (c) vertical zoning expressed as a decrease in Cu concentrations (reverse zoning) up the section of the pyrrhotite ore and an increase (direct zoning) in the Cu-rich ore (Torgashin, Reference Torgashin1994).
Lenses C-3 and C-4 belong to different flows of silicate magma and sulfide melt (Fig. 2): the northern lens C-4 belongs to a branch of the extended ‘Oktyabr'sky flow’, and the southern lens C-3 belongs to another flow (unnamed), extending northwest from the regional fault (Stekhin, Reference Stekhin, Lightfoot and Naldrett1994). Their study is very important for understanding the conditions for the formation of the deposit as a whole (Krivolutskaya, Reference Krivolutskaya2014; Krivolutskaya et al., Reference Krivolutskaya, Gongalsky, Kedrovskaya, Kubrakova, Tyutyunnik, Chikatueva, Bychkova, Magazina, Kovalchuk, Yakushev and Kononkova2019; Reference Krivolutskaya, Bychkova, Gongalsky, Kubrakova, Tyutyunnik, Dekunova and Taskaev2021).
We present new mineralogical data from boreholes on two orebodies in the central part of the Oktyabr'sky deposit, including both massive and disseminated ores located at the bottom of the Kharaelakh intrusion: C-3 in the south and C-4 in the north of this area (Fig. 2). These boreholes are characterised by different mineral associations of both sulfides and platinum-group minerals (PGM). This work has two objectives: (1) to demonstrate the mineralogical differences and similarity between disseminated and massive ores; (2) to show typomorphic features of disseminated ores in picritic gabbro-dolerite for C-3 (RT-30 borehole) and C-4 (RT-107 borehole) orebodies to verify the difference in their genesis.
Brief geology of the Norilsk district
The geology of the Talnakh ore cluster and the Oktyabr'sky deposit, related to the Kharaelakh intrusion is described in many publications (for example: Zolotukhin, Reference Zolotukhin1964; Zolotukhin et al., Reference Zolotukhin, Ryabov, Vasil'ev and Shatkov1975; Dyuzhikov et al., Reference Dyuzhikov, Distler, Strunin, Mkrtychyan, Sherman and Sluzhenikin1992; Torgashin, Reference Torgashin1994; Stekhin, Reference Stekhin, Lightfoot and Naldrett1994; Likhachev, Reference Likhachev1996; Turovtsev, Reference Turovtsev2002). This intrusion belongs to the Norilsk intrusive complex (Dyuzhikov et al., Reference Dyuzhikov, Distler, Strunin, Mkrtychyan, Sherman and Sluzhenikin1992) and is located within the carbonate–sulfate–terrigenous rocks, mostly, at the boundary of the Razvedochninsky and Kureysky Formations, and partially in the Manturovsky Formation (western flanks). The Devonian sediments are overlapped by the coal-bearing Tunguska series and P3-T1 tuff-lavas of the Ivakinsky, Syverminsky, Gudchikhinsky, Khakanchansky, Nadezhdinsky, Morongovsky and Mokulaevsky formations (Figs 1, 4). The Kharaelakh intrusion, like other intrusions of the Norilsk complex, is composed of a differentiated series of gabbro-dolerites, with varying amounts of olivine that decrease from bottom to top (Fig. 4), i.e. in the order: contact, taxitic, picritic, olivine, olivine-bearing, olivine-free; leucogabbro, gabbro-diorite, ferrogabbro, upper taxitic, and then upper picritic gabbro-dolerites form the upper intrusive zone. Hereinafter, the nomenclature of rocks adopted in the legend to the 1: 200,000 scale Geological Map is used (Strunin, Reference Strunin1994).
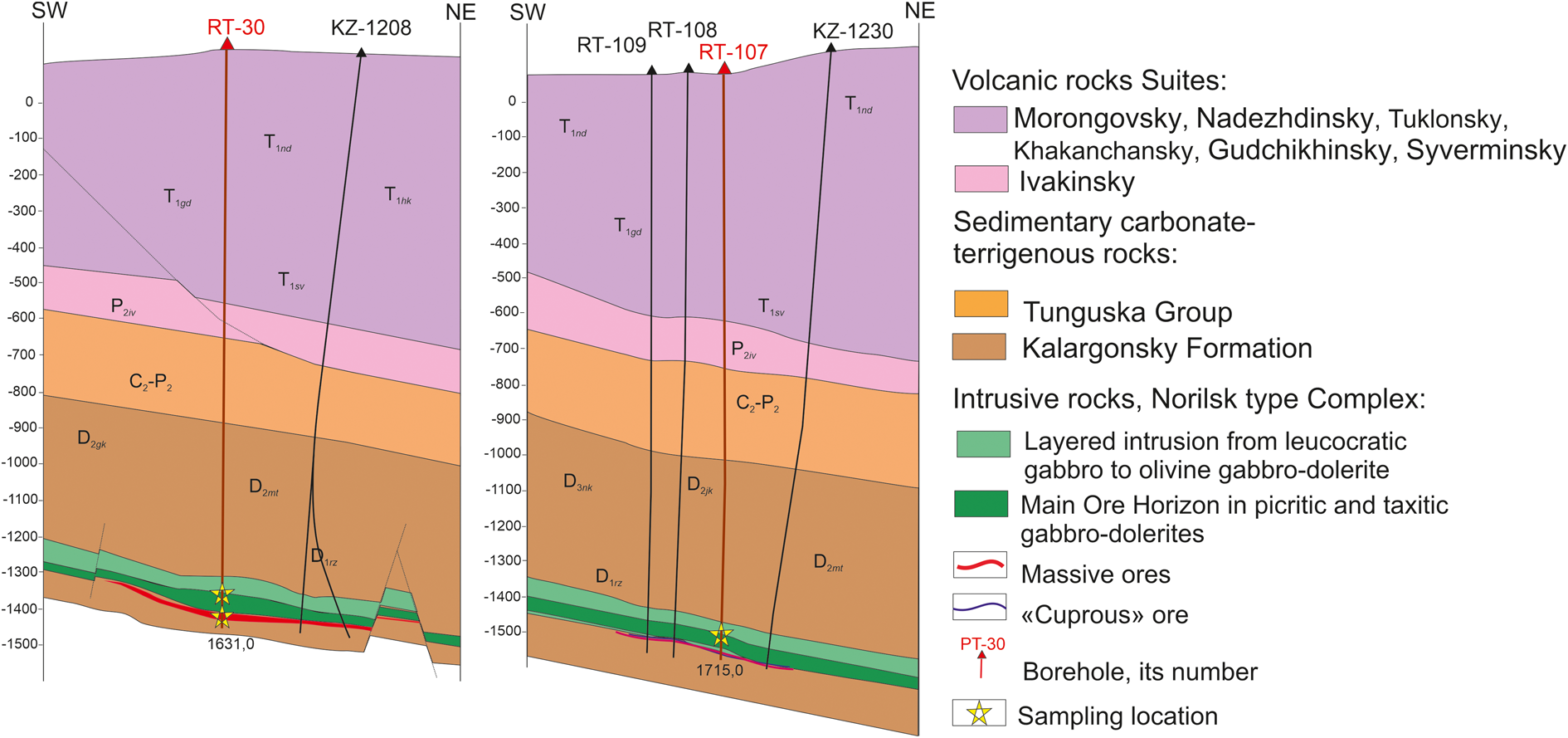
Figure 4. Sections of the intrusion showing the position of boreholes with the studied ores of the Oktyabr'sky deposit according to ‘Norilskgeologiya’ LLC, Norilsk Nickel company.
The picritic gabbro-dolerite are considered a cumulative part of the main layered series (Likhachev, Reference Likhachev1996; Distler et al., Reference Distler, Sluzhenikin, Cabri, Krivolutskaya, Turovtsev, Golovanova, Mokhov, Knauf and Oleshkevich1999). Massive ores of various thicknesses are located at the contact of the intrusion with the host rocks. They are separated from the intrusion by a horizon of host hornfelses, with thicknesses reaching, for example, 19 m in borehole RT-30 and only 3 m in RT-107 (Fig. 5).
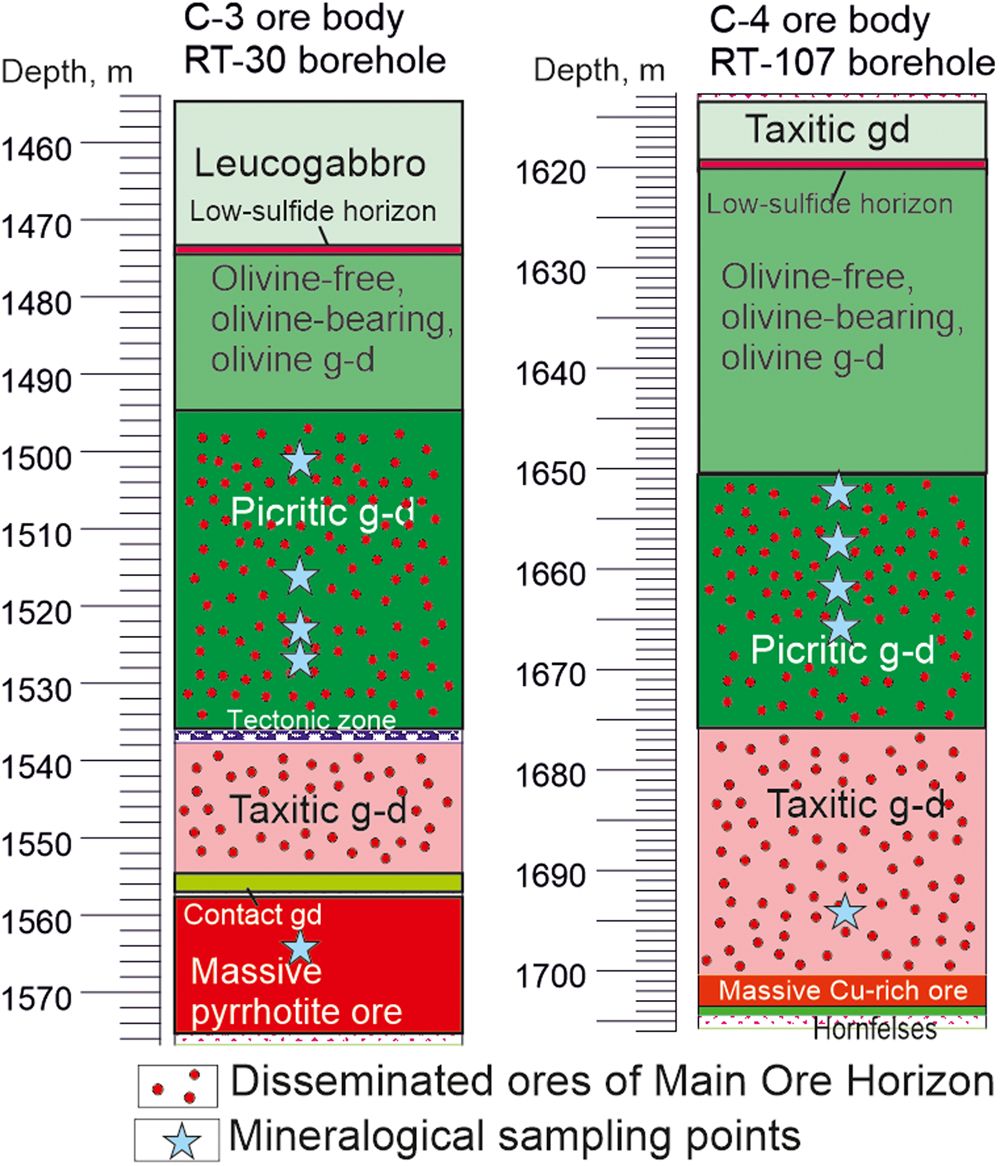
Figure 5. Schematic columns of boreholes RT-30 and RT-107 with sampling points.
Methods of analysis
The analyses of sulfides and minerals of the platinum-group elements and high-resolution electronic imaging were performed at the Analytical Center for Multi-Element and Isotope Research of the IGM of SB RAS, Novosibirsk (N.S. Karmanov) by X-ray spectral methods using microanalysers (SEM-EDS) MIRA 3 LMU (Tescan Ltd) with an INCA Energy 450+ XMax 50+ and Inca Wave 500 microanalysis system (Oxford Instruments Ltd). Analytical conditions were: beam size ~2 μm and accelerating voltage 20 kV; beam current 30–50 nA over the sample surface; and beam diameter ~1 μm. The measurement duration was 20 s for each analytical line. The following standards were used: FeS2 (S), PtAs2 (As), HgTe (Te), metallic Zn, Co, Ni, Cu, Pt, Pd, Au, Ag, Sn, Sb, etc. The limit of detection for the majority of the elements was 0.2–0.3% (3 sigma criterion). The correction of matrix effects was performed using the XPP software algorithm. The accuracy and reproducibility of the analytical procedure were evaluated by comparing the results of EDS and WDS analysis on sulfides (pyrrhotite, pentlandite and chalcopyrite) in the RT-107 borehole, identifying the macrocomponents (Fe, Ni, Cu, Co), and confirmation using special tests (Korolyuk et al., Reference Korolyuk, Usova and Nigmatulina2009). We also carried out a comparison of EDS and WDS analysis of pentlandite from samples of picritic gabbro-dolerites in both boreholes, resulting in convincing compositions of pentlandite obtained by the different methods. Therefore the EDS results were used; all other minerals were analysed by X-ray spectral methods on microanalysers (SEM-EDS) MIRA 3 LMU.
Results
General characteristics of the sulfide ores
Sulfide associations and PGMs were investigated in samples of disseminated ores from picritic and taxitic gabbro-dolerites above the northern body C-4 and southern body C-3 (in boreholes RT-107 and RT-30, correspondingly). Picritic gabbro-dolerites of the Oktyabr'sky deposit, in addition to all other deposits of the Norilsk complex, are represented by fine- to medium-grained massive rocks consisting of (wt.%): olivine (50–80); clinopyroxene (20–30); plagioclase (20–40); and orthopyroxene (~5). Sulfides in the picritic gabbro-dolerite of both boreholes are composed of drops and small segregations of chalcopyrite–pentlandite–cubanite–pyrrhotite composition (Fig. 6a–d). Massive ores of the southern С-3 orebody are essentially pyrrhotite in composition. Pyrrhotite commonly occurs as intergrowths of crystals of various modifications (troilite and hexagonal), and can be found with second generation exsolution inclusions of pentlandite (Fig. 6h). Chalcopyrite grains of various sizes are surrounded by pentlandite rims of the first generation (Fig. 6h,i).
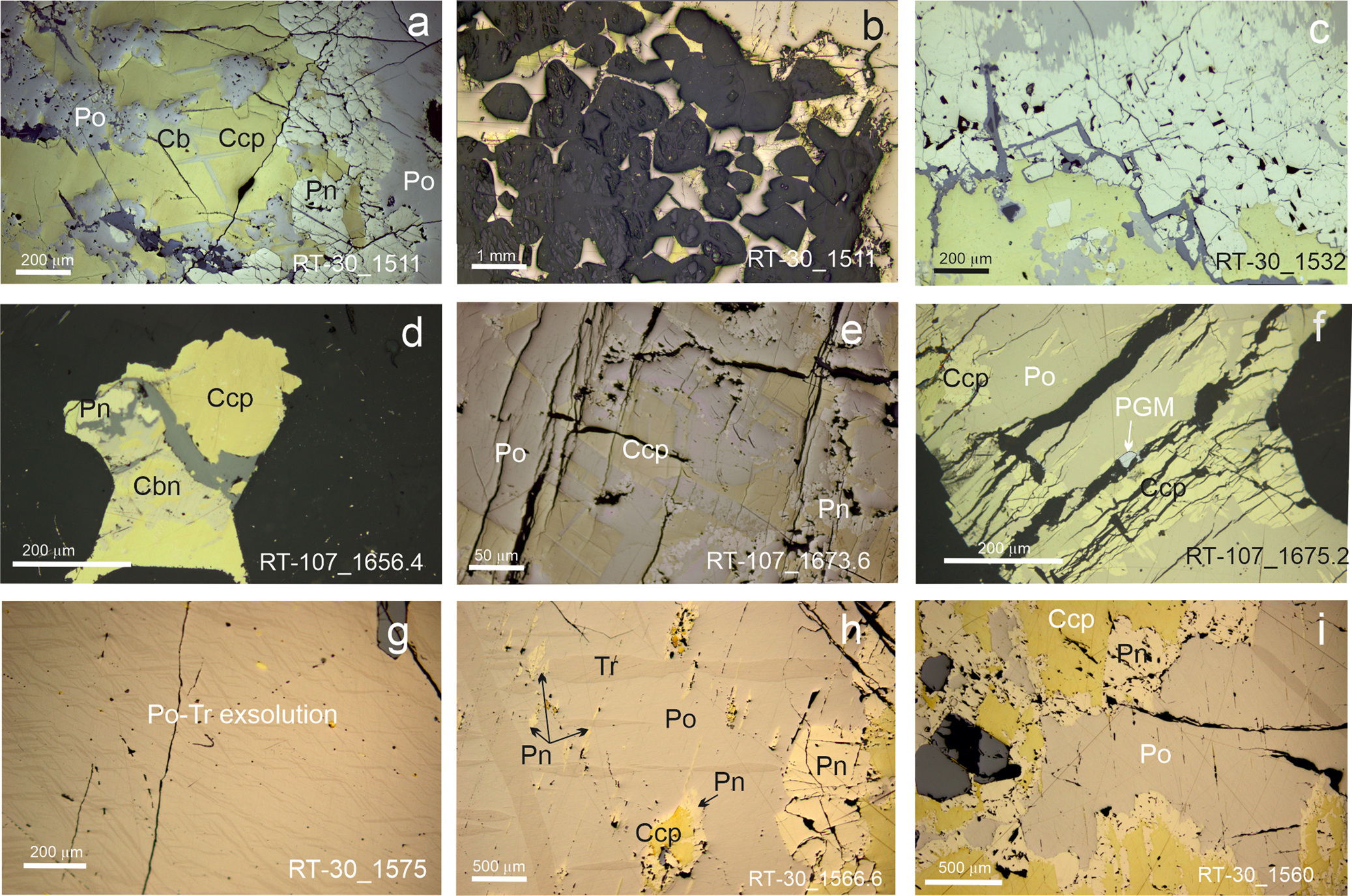
Figure 6. Photomicrograph in reflected light of various types of ores from the southern C-3 (a–c, g–i) and northern C-4 (d–f) orebodies. Details: (a–f) sulfides from disseminated ore in picritic gabbro-dolerite; (g–i) – sulfides from massive ore. Po – pyrrhotite, Ccp – chalcopyrite, Cbn – cubanite, Pn – pentlandite, Mag – magnetite, Tro – troilite, Sil – silicate minerals, PGM – platinum-group minerals.
The sulfur concentration in the disseminated ores does not exceed 6 wt.% in the picritic gabbro-dolerite and 0.5 wt.% in taxitic rocks; PGE contents in both rocks are in single percent levels; and Pd prevails over Pt. The sulfur concentration in massive ore varies between 41.8–47.8 wt.% and the (Pd + Pd) concentrations reach 30 ppm.
Sulfide associations.
We have analysed disseminated sulfides in the following samples taken from different levels of the picritic gabbro-dolerites, i.e. in RT-30: at 1501.2, 1516, 1523 and 1527, and in RT-107: at 1652, 1657.2 and 1665.4 m (Fig. 5). They are composed mainly of pyrrhotite (Po) or troilite (Tr), chalcopyrite (Ccp) and pentlandite (Pn) (Table 1).
Table 1. Representative compositions of sulfides from boreholes RT-30 (orebody C-3) and RT-107 (orebody C-4) of the Oktyabr'sky deposit.
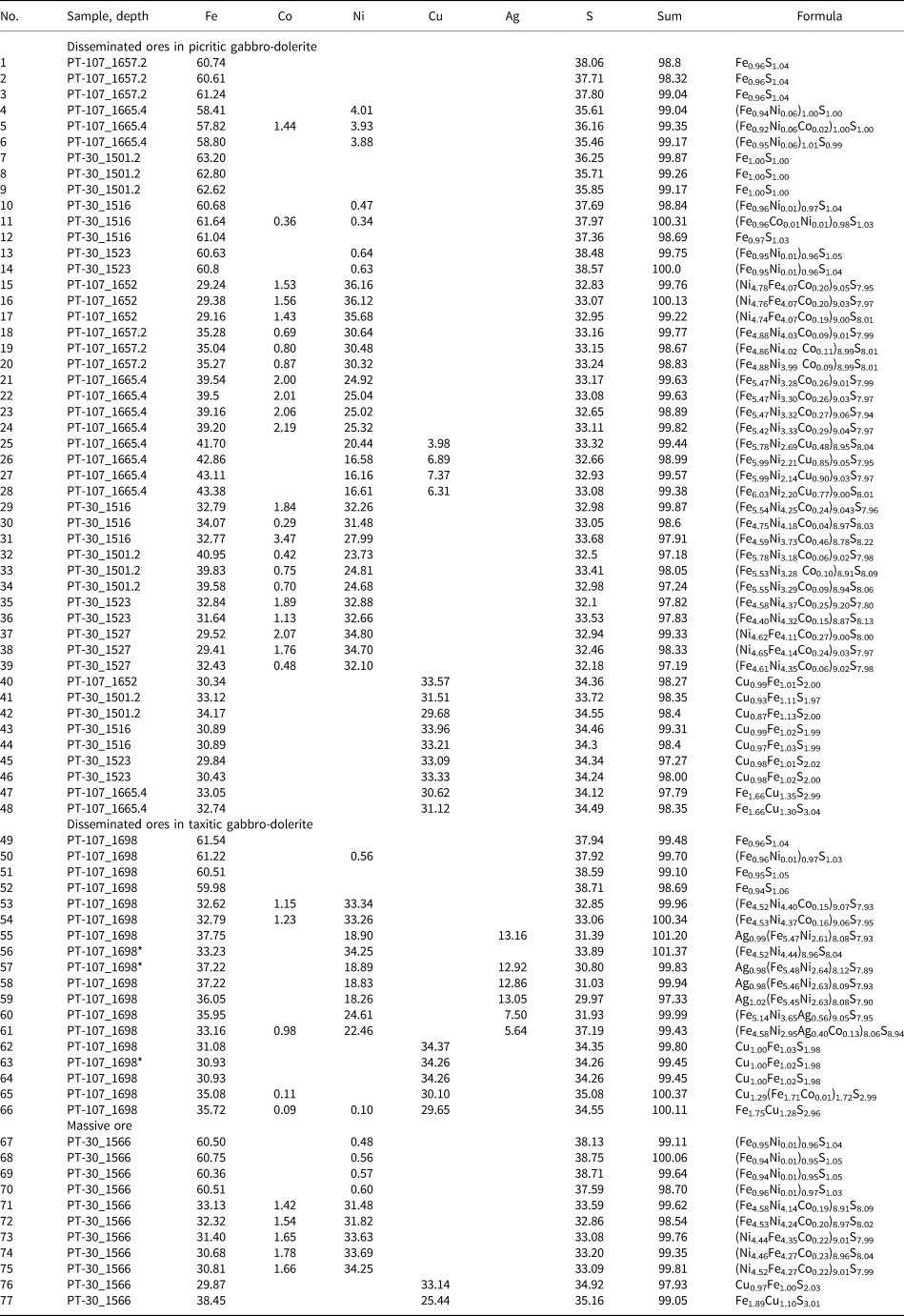
The table presents the sample compositions of sulfides and is constructed on the principle of changing compositions with depth from the upper parts of the picrite layers to the lower ones for each borehole.
Minerals: 1–14, 49–52, 67–70 – pyrrhotite (Fe,Ni,Co)S; 15–24, 29–39, 53–61, 71–75 – pentlandite (Fe,Ni)9S8; 25–28 – sugakiite Cu(Fe,Ni)8S8; 40–46, 62–65, 76 – chalcopyrite CuFeS2; 47–48, 65–66, 77 – cubanite Fe2CuS3; * Sample PT-107–1698* – compositions shown in Fig. 7.
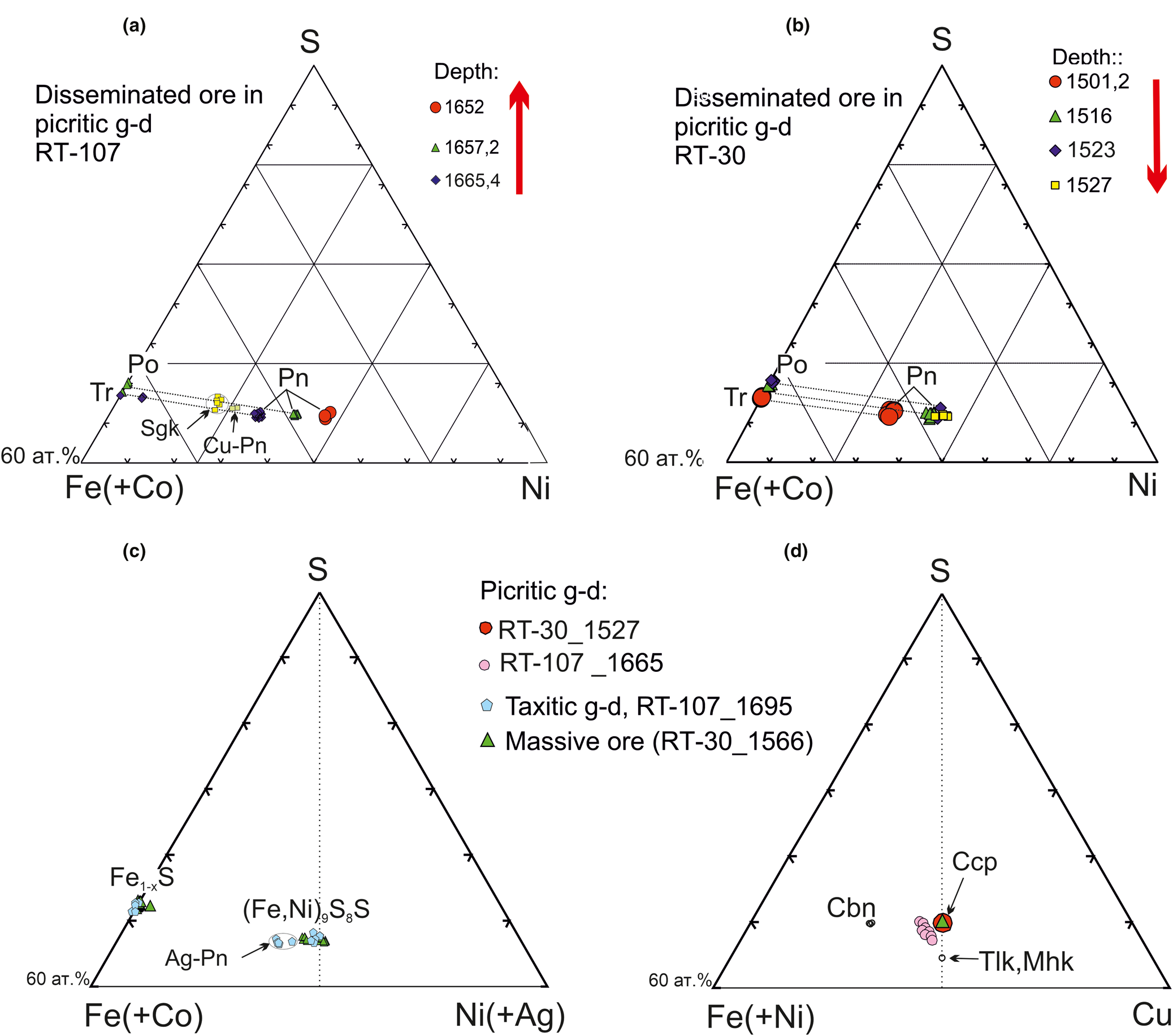
Figure 7. Compositions of sulfides: (a–c) in the Fe(+Co)–S–Ni(+Ag) system; (d) in the Fe(+Ni)–S–Cu system in various types of ores. Details: (a,b) Po and Pn paragenetic associations connected by connodes; (c,d) comparisons of sulfides in picritic, taxitic gabbro-dolerites and massive ores. Po – pyrrhotite, Ccp – chalcopyrite, Pn – pentlandite, Cbn – cubanite, Sgk – sugakiite, Ag-Pn – argentopentlandite, Tlk, Mhk - talnakhite and mooihoekite. The red trend indicates the direction of sulfur fugacity increase.
The iron–nickel ratio in pentlandite varies significantly in the picritic gabbro-dolerite in the sections: both ferruginous and nickel-rich varieties are present however Fe-rich pentlandite predominates (Fig. 7a,b), including Co up to 3.47 wt.% (Table 1, No. 31). The most Fe-rich compositions of pentlandite occur at a deeper horizon (1665.4 m) of picritic ore over the northern orebody C-4, and the content of Ni in pentlandite, as well as S in pyrrhotite, increases up the section towards the 1652 m horizon (Table 1, Fig. 7a). Whereas the opposite direction of these changes occurs in the disseminated ore over the southern orebody: Fe-rich Po and S-poor Po (troilite) are located in the upper part of the picritic layer (1501.2 m), and the most enriched in nickel – at a depth (1527 m) (Table 1, Fig. 7b).
A very important difference between the northern and southern disseminated ores is that the former (in borehole RT-107) contains sugakiite Cu(Fe,Ni)8S8 (Fig. 7a) and sulfides deficient in sulfur of the chalcopyrite group (approaching talnakhite in composition) (Fig. 7d), whereas the latter ores (RT-30) comprise only stochiometric chalcopyrite (tetragonal). Sugakiite, a rare mineral, occurs as granular (60–120 μm) grains intergrown with pyrrhotite at the bottom part of the picritic horizon (1665.4 m). The concentration of copper in sugakiite varies from 0.48–0.90 wt.% (Table 1, Nos. 25–28, Fig 7a) and is similar to that of the first discovery described in Hokkaido, Japan (Kitakaze, Reference Kitakaze2008).
Disseminated ore in the taxitic gabbro-dolerite (RT-107_1698) is represented by monoclinic pyrrhotite, chalcopyrite, cubanite and pentlandite. In detail, Ag-rich pentlandite (0.40–0.56 apfu Ag) and argentopentlandite (0.98–1.02 apfu Ag)], with the Ag concentration in the latter reaching 13.16 wt.%. (Table 1, 56–61), and Fe significantly prevailing over Ni (Table 1, Fig. 7c). Argentopentlandite grains (~100 μm) are included in the chalcopyrite matrix in association with fine Ag-free pentlandite grains framing this chalcopyrite (Table 1, Fig. 8a,b,f).
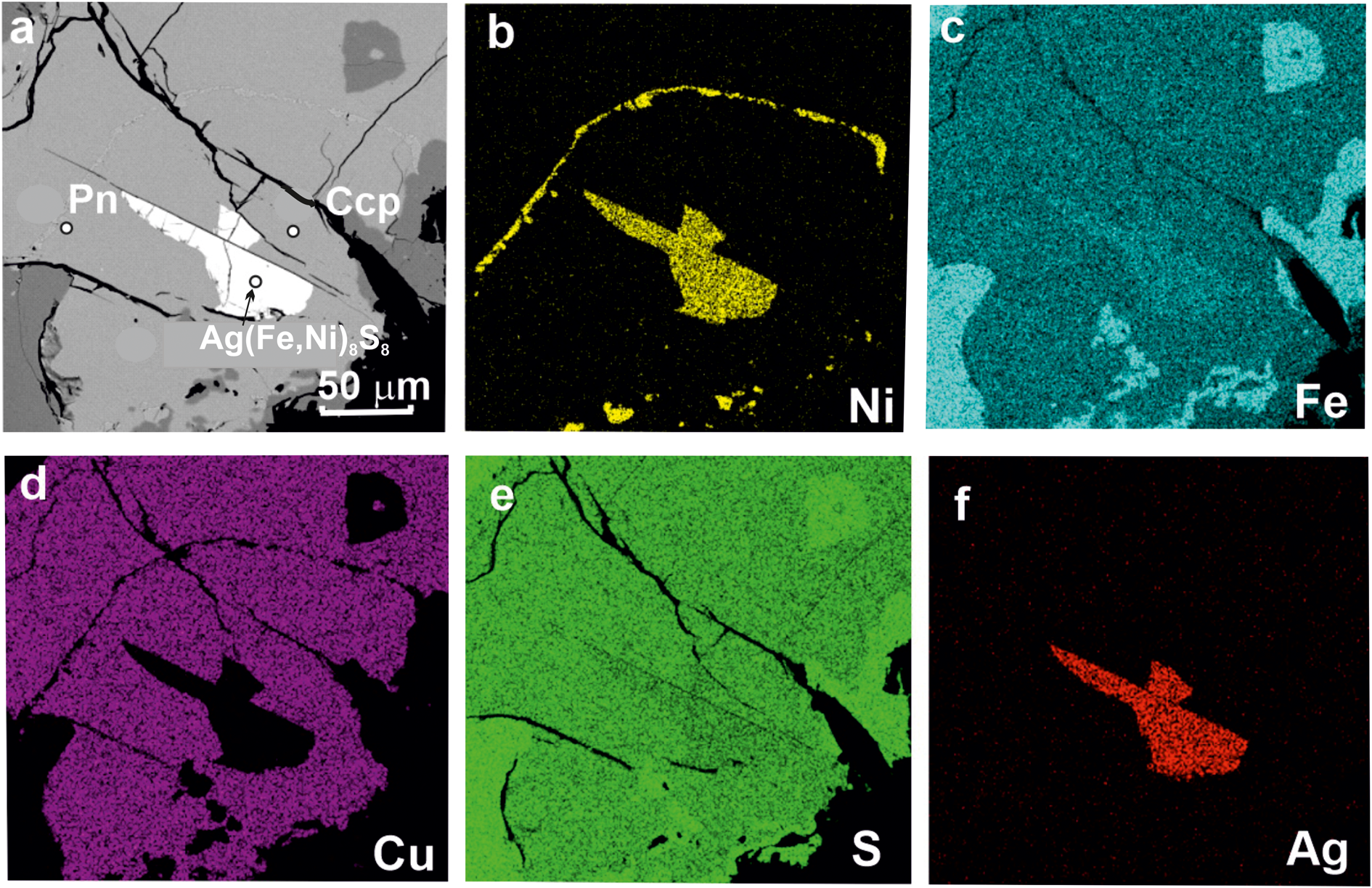
Figure 8. Sulfide microparagenesis in taxitic gabbro-dolerite (RT-107_1695). (a) BSE image of argentopentlandite Ag(Ni,Fe)8S8 and Ag-free pentlandite (Pn), included in chalcopyrite Ccp and (b–f) X-ray maps of the same area showing individual element distributions.
The massive ore of the southern orebody (RT-30_1566) is characterised by a predominance of pyrrhotite over other sulfides. Pentlandite occurs in two generations: Pn1 as rims around chalcopyrite segregations, and Pn2 as thin lamellae – exsolution inclusions in a pyrrhotite matrix. Pentlandite contains ubiquitous Ni, up to 1 wt.%, and Co from 1.42 to 1.80 wt.%. Compositions of pentlandite vary within small limits and belong to both Fe-rich and Ni-rich varieties (Fig. 7c). Compositions intermediate between cubanite and chalcopyrite are, possibly, a fine exsolution texture between these minerals (Fig. 7d).
Association of minerals of the Platinum Group Elements (PGM)
Disseminated ore in the picritic gabbro-dolerite of the southern C-3 orebody (RT-30_1527) contains the following PGMs: sperrylite PtAs2 grains (30–50 μm) intergrown with chalcopyrite and pentlandite (Fig. 9a,c,i); Te- and Sb-bearing sobolevskite Pd(Bi, Te, Sb) (Fig. 9b,f,g); sopcheite Ag4Pd3Te4 as a small (2 μm) inclusion in pentlandite (Fig. 9e); stibiopalladinite Pd5Sb2 intergrown with sobolevskite and stannopalladinite Pd5Sn2Cu (Fig. 9g,h); and single grains of Au–Ag alloys (Fig. 9d). Stannopalladinite from the southern orebody contains Pt up to 7.52 wt.%, sometimes Sb ~2 wt.%, and corresponds to the formula (Pd,Pt)5(Sn,Sb)2Cu (Fig. 10a). Sperrylite and stibiopalladinite correspond to their stoichiometric PtAs2 and Pd5Sb2 formulas, whereas sobolevskite Pd(Bi, Te, Sb) forms solid solutions with kotulskite up to 0.38 apfu Te and sudburyite up to 0.09 apfu Sb (Table 2).
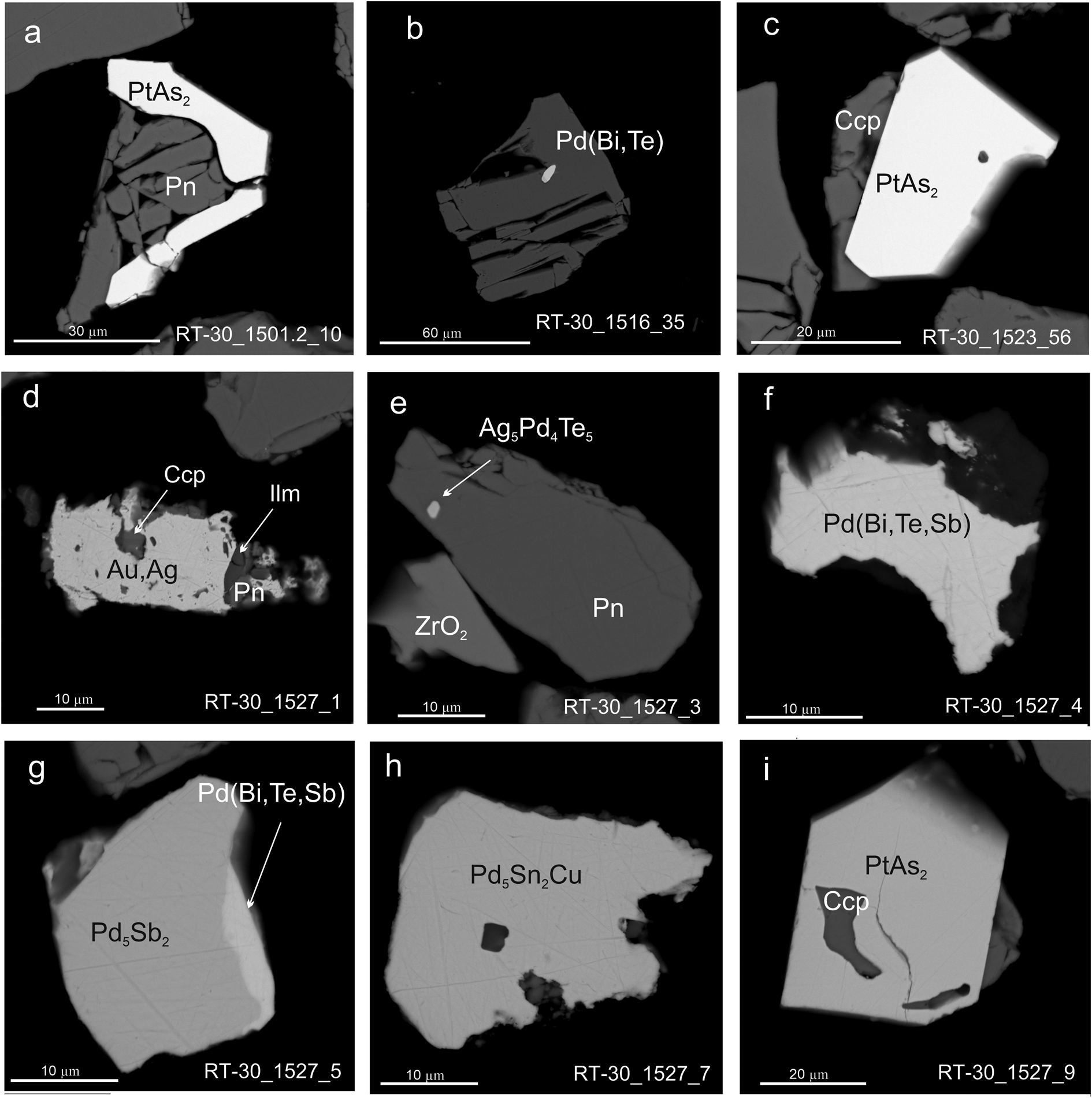
Figure 9. Scanning electron microscope images of PGM and Ag–Au alloys from disseminated ores of picritic gabbro-dolerite of the southern orebody (RT-30): sperrylite PtAs2 intergrown with pentlandite (a) and chalcopyrite (c, i); sobolevskite Pd(Bi,Te,Sb) and Pd(Bi,Te) (b,f); sopcheite Ag5Pd4Te5 included in pentlandite (e); stibiopalladinite Pd5Sb2 intergrown with sobolevskite (g); grain of stannopalladinite Pd5Sn2Cu (h).
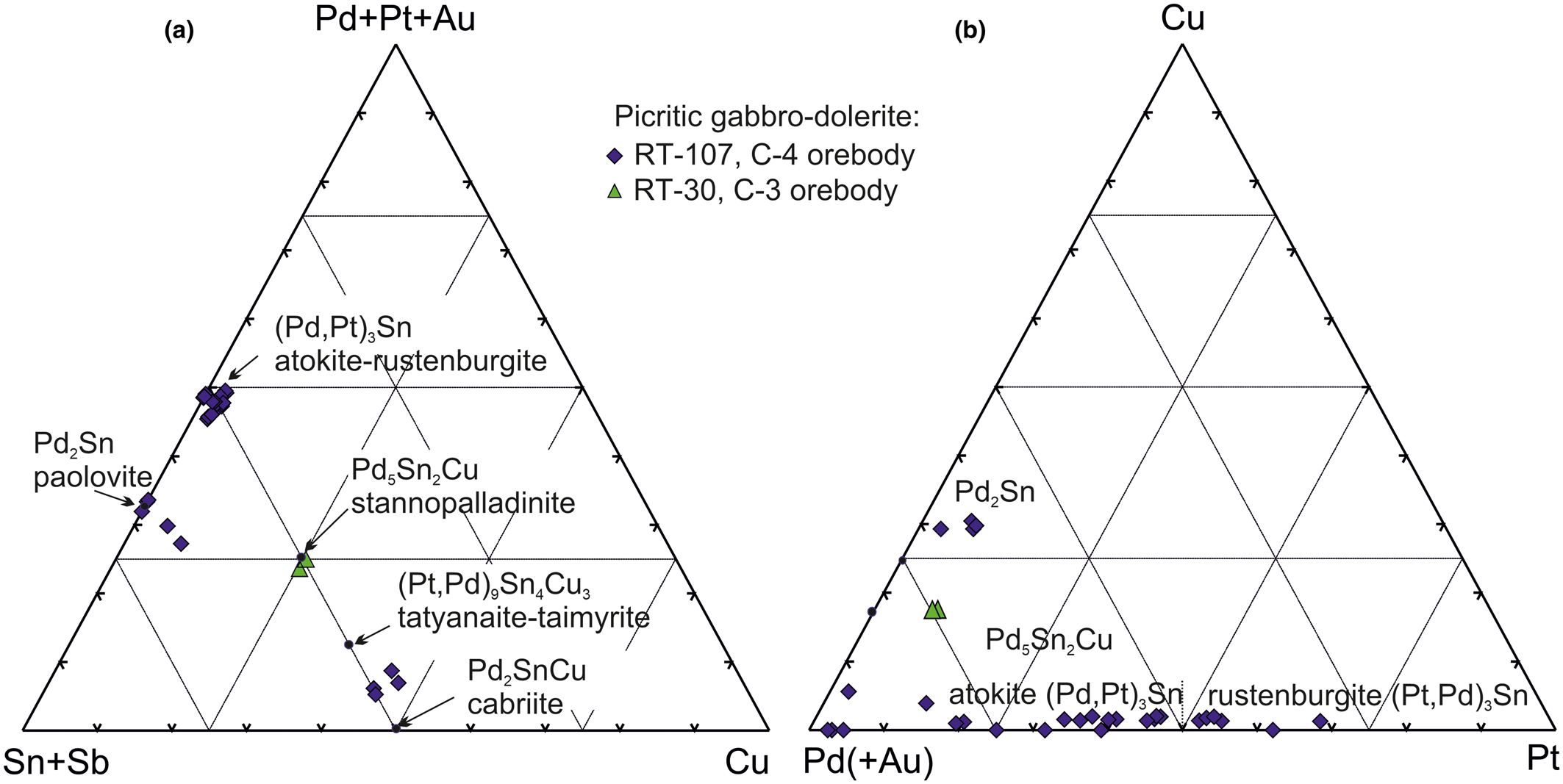
Figure 10. Compositions of PGE minerals in the Sn–(Pd + Pt)–Cu (a) and Pd(+Au)–Cu–Pt (b) systems.
Table 2. Compositions of PGM (wt.%).
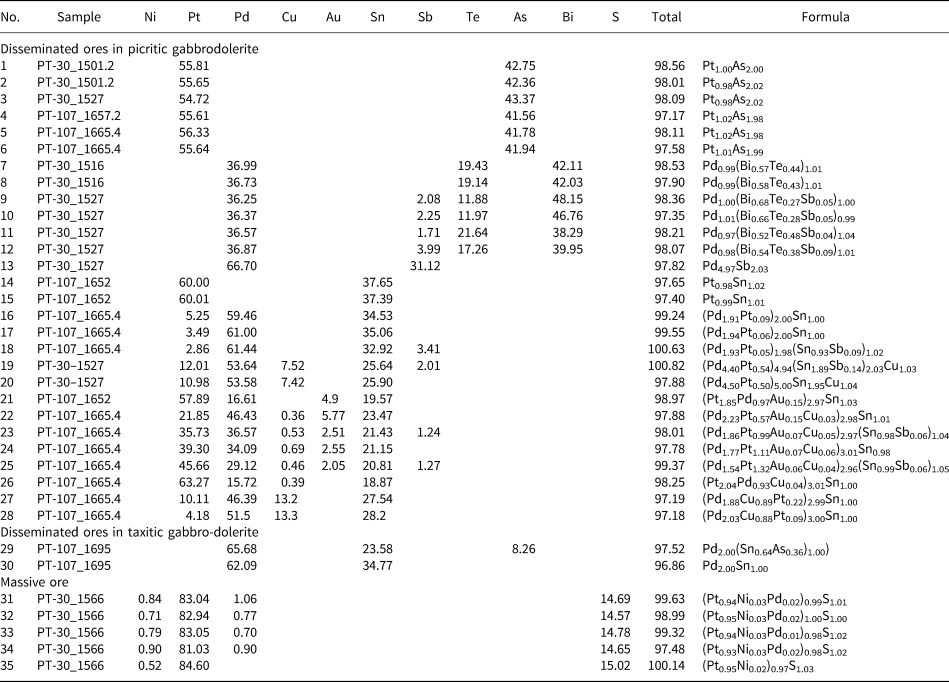
* The total of the mineral analysis after subtracting the composition of the sulfide matrix; Minerals: 1–6 – sperrylite PtAs2; 7–12 – sobolevskite Pd(Bi,Te,Sb); 13 – stibiopalladinite Pd5Sb2; 14, 15 – niggliite PtSn; 16–18, 29–30 – paolovite Pd2(Sn,As,Sb); 19–20 – stannopalladinite Pd5Sn2Cu; 21–26 - rustenburgite-atokite solid solutions (Pd,Pt,Cu)3Sn; 27–28 – taimyrite–cabriite solid solution (Pd,Pt,Cu)3Sn; 31–35 – cooperite PtS.
The PGM association in disseminated ore in the picritic gabbro-dolerite of the northern C-4 orebody (PT-107) differs from those described above and includes niggliite (PtSn) with gold dissemination (Fig. 11a) in the upper part of the horizon; atokite–rustenburgite solid solutions that dominate in the lower part of this horizon (Fig. 11f,i); paolovite (Pd2Sn), sometimes as a fine exsolution texture with sobolevskite (Fig. 11h) and minerals intermediate in composition between taimyrite and cabriite (Fig. 10a). Sperrylite is a common mineral of this association. A characteristic feature of this PGM is Au impurity (up to 5.77 wt.%) in atokite and rustenburgite (Table 2). Native silver, Ag–Au–Cu alloys, and auricupride Cu3Au are common (Table 3, Fig. 12). In the taxitic gabbro-dolerite (in studied samples), only As-bearing paolovite was found up to 0.36 apfu As (Table 2).
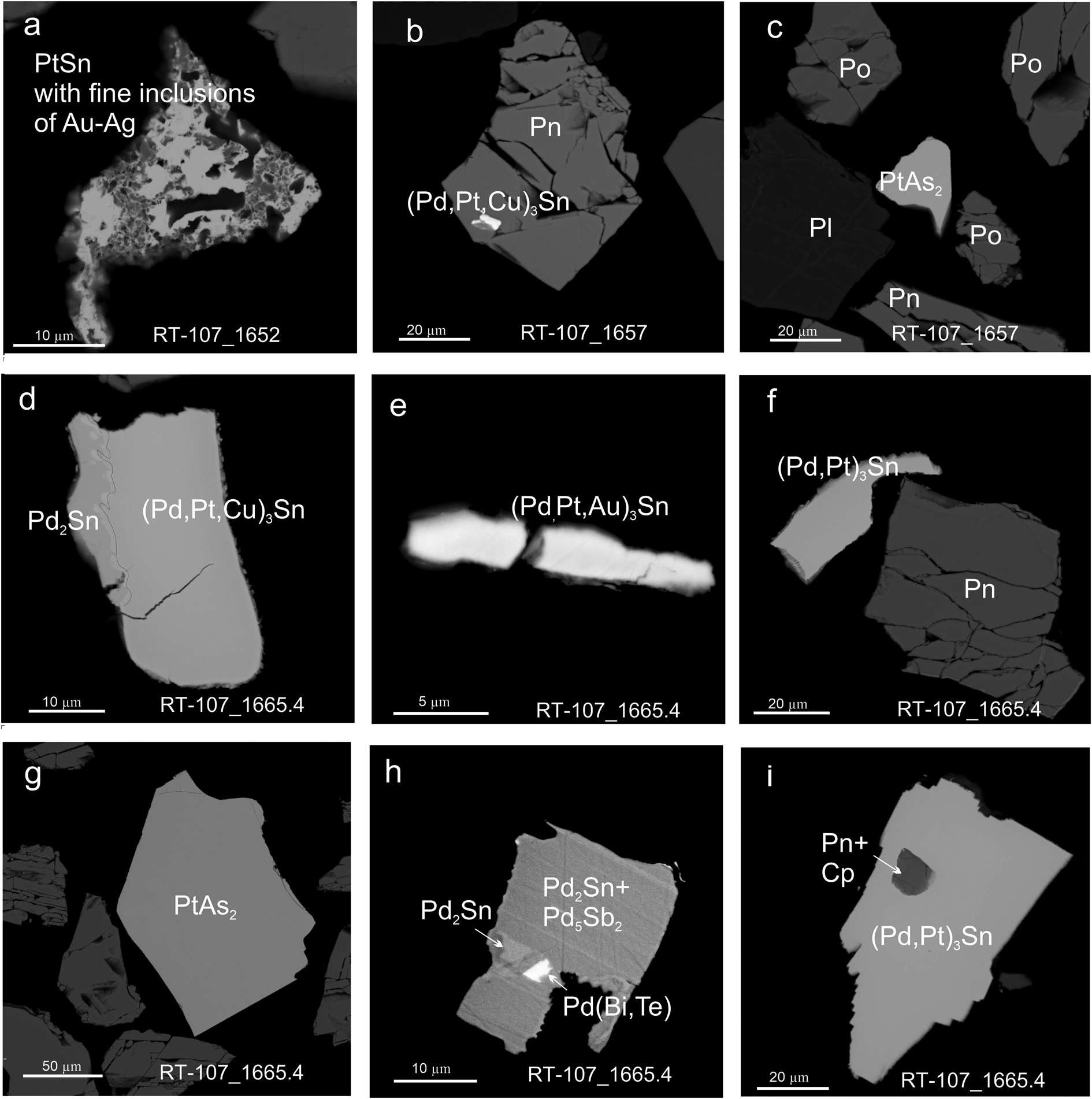
Figure 11. SEM images. PGM from disseminated ore of picritic gabbro-dolerite of the northern orebody (borehole RT-107: (a) niggliite PtSn; (b,d,f,i) – rustenburgite (Pd,Pt,Cu)3Sn; (c,g) – sperrylite PtAs2; (e) stannopalladinite Pd5Sn2Cu; (h) inclusions of sobolevskite Pd(Bi,Te) and paolovite Pd2Sn in the exsolution texture of paolovite and stibiopalladinite Pd5Sb2.
Table 3. Compositions of Au–Ag alloys from the Oktyabr'sky deposit.
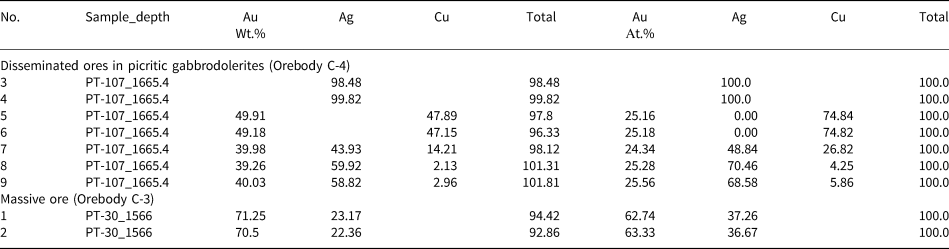
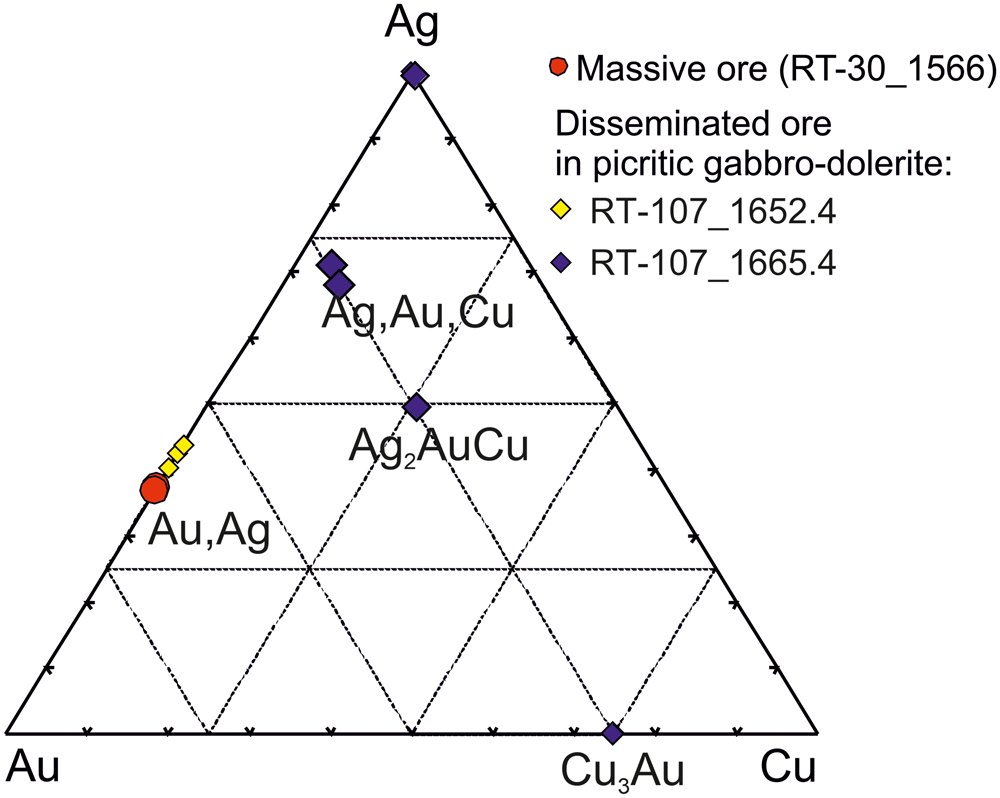
Figure 12. Compositions of Au–Ag–Cu alloys in various types of ores from the C-3 and C-4 orebodies.
Precious metal (PGE and Au) minerals from massive pyrrhotite ores are characterised by platinum specialisation, i.e. all PGMs found are platinum minerals, even though Pd prevails over Pt in all ore samples. They form small (1–3 μm) inclusions in pyrrhotite, less commonly in chalcopyrite. These are represented by Pt–Fe alloys, a S-rich variety of sperrylite PtAsS, sperrylite PtAs2 and cooperite PtS (Fig. 13). The small size of these grains does not always allow determination of the quantitative composition and are hence only identified qualitatively. Only the Au–Ag alloys and cooperite reach sizes of 15–25 μm (Fig. 13a,g). The Au–Ag alloys of this association are of higher grade gold compared to those from the picritic rocks: Au0.64Ag0.36 (Table 3, Fig. 12). The concentrations of both Pd and Ni do not exceed 1 wt.% in cooperite (Table 2). A rare associated mineral is bismuth oxychloride with the formula BiOCl2, close to bismoclite (BiOCl) and acanthite Ag2S, which occur as inclusions up to 10 μm in size in pyrrhotite in massive ores (Fig. 13h,i).
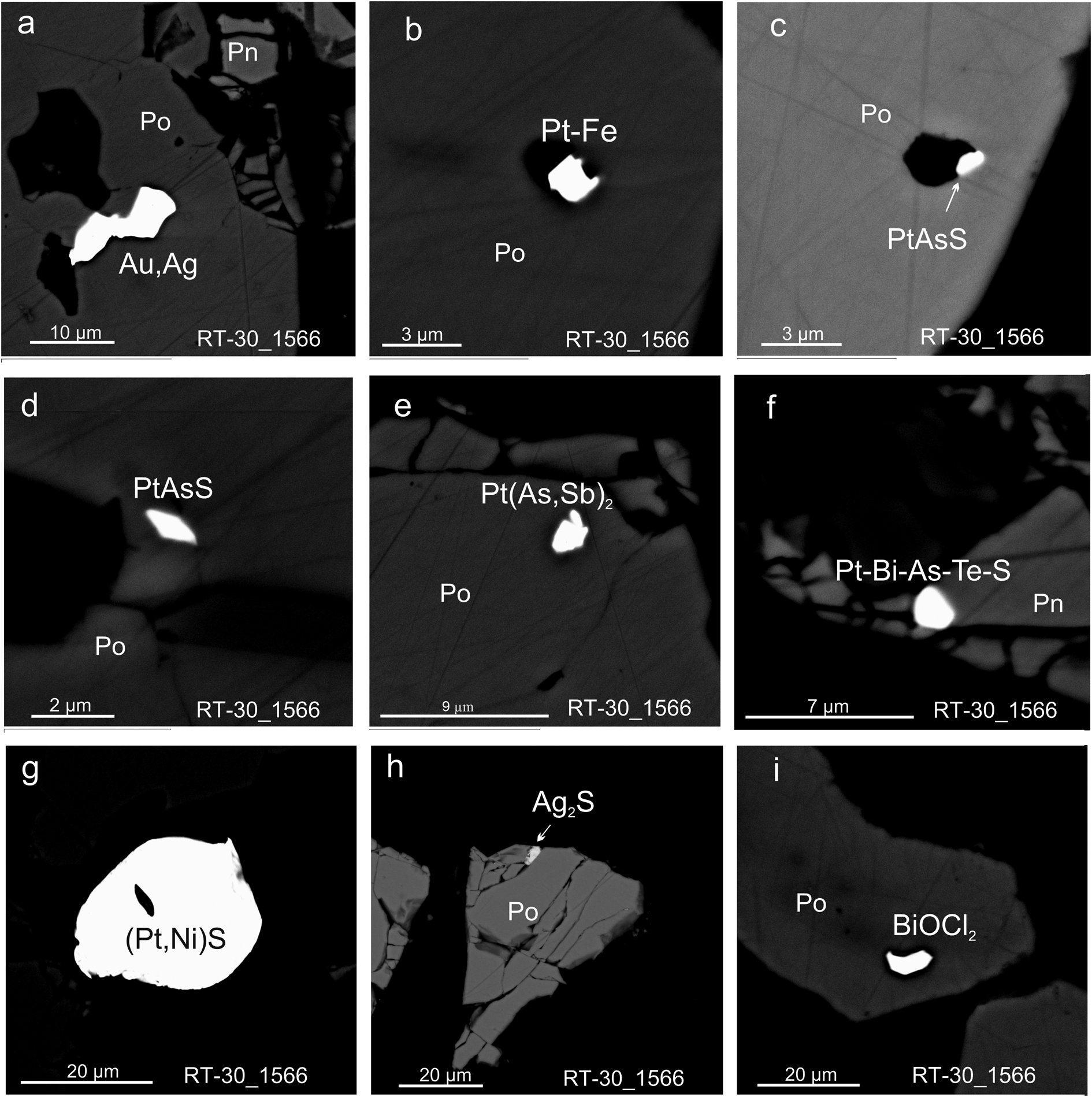
Figure 13. SEM images of PGM, gold and associated minerals from massive ore of the C-3 orebody (RT-30_1566 m). Inclusions of (a) Au–Ag alloy; (b) isoferroplatinum Pt3Fe, (c,d) S-rich variety of sperrylite PtAsS, (e) Sb-sperrylite, (f) Pt–Bi–As–Te–S phase, (g) cooperite grain, (h) acanthite (Ag2S), (i) bismoclite in pyrrhotite.
Discussion
Mineralogical features and zoning in picritic gabbro-dolerite of the C-3 and C-4 orebodies
The mineralogy of various types of ores of the Norilsk deposits has been investigated and discussed for many years (for example, Genkin et al., Reference Genkin, Evstigneeva, Troneva and Vyalsov1969, Reference Genkin, Distler, Gladyshev, Filimonova, Evstigneeva, Kovalenker, Laputina, Smirnov and Grokhovskaya1981; Distler et al., Reference Distler, Genkin, Filimonova, Hitrov and Laputina1975, Reference Distler, Sluzhenikin, Cabri, Krivolutskaya, Turovtsev, Golovanova, Mokhov, Knauf and Oleshkevich1999; Genkin and Evstigneeva, Reference Genkin and Evstigneeva1986; Begizov et al., Reference Begizov, Meschankina and Dubakina1974; Razin et al., Reference Razin, Dubakina and Dubinchuk1976; Evstigneeva and Genkin, Reference Evstigneeva and Genkin1983; Barkov et al., Reference Barkov, Martin, Poirier, Tarkian, Pakhomovskii and Men'shikov2000; Kozyrev et al., Reference Kozyrev, Komarova, Emelina, Oleshkevich, Yakovleva, Lyalinov, Maximov and Cabri2002; Komarova et al., Reference Komarova, Kozyrev, Simonov, Lyul'ko and Cabri2002; Spiridonov et al., Reference Spiridonov, Kulagov and Kulikova2003, Reference Spiridonov, Kulagov and Kulikova2004, Reference Spiridonov, Kulagov, Serova, Kulikova, Korotaeva, Sereda, Tushentsova, Belyakov and Zhukov2015; Likhachev, Reference Likhachev2004; Sluzhenikin, Reference Sluzhenikin2010, Reference Sluzhenikin2011; Sluzhenikin and Mokhov, Reference Sluzhenikin and Mokhov2014; Tolstykh et al., Reference Tolstykh, Krivolutskaya, Safonova, Shapovalova, Zhitova and Abersteinerd2020a, Reference Tolstykh, Shvedov, Polonyankin and Korolyuk2020b, Reference Tolstykh, Garcia and Shvedov2021). Our conclusion here is based on mineralogical data which allow us to evaluate the physicochemical conditions of ore crystallisation, in particular, the fugacity of sulfur in the ore-forming system.
The southern and northern orebodies differ in their mineralogical composition. The southern C-4 orebody is characterised by a high-sulfur association and zoning, expressed by pyrrhotite–chalcopyrite fractionation (Distler et al., Reference Distler, Genkin, Filimonova, Hitrov and Laputina1975), and the northern C-3 orebody contains minerals typical of a low-sulfur association (troilite, talnakhite, sugakiite) which is a characteristic of disseminated ores in the picritic gabbro-dolerite only (Distler et al., Reference Distler, Sluzhenikin, Cabri, Krivolutskaya, Turovtsev, Golovanova, Mokhov, Knauf and Oleshkevich1999; Tolstykh et al., Reference Tolstykh, Shvedov, Polonyankin and Zemlyanssky2017).
In addition, these orebodies show multidirectional zoning of pentlandite compositions (Fig. 7), which varies regularly along the sections of the intrusive rocks. The Fe/Ni ratio of pentlandite reflects the activity of sulfur (log$f_{\rm S_2}$) during its formation, and the Ni content in pentlandite has direct proportional dependence on log$f_{\rm S_2}$
(Kaneda et al., Reference Kaneda, Takenouchi and Shoji1986; Kolonin et al., Reference Kolonin, Orsoev, Sinyakova and Kislov2000; Kosyakov et al., Reference Kosyakov, Sinyakova and Shestakov2003). This dependence is based on experimental data and calculation of k = Ni/(Ni + Fe) in pentlandite (Fig. 14). The value ‘k’ varies from 0.38 (in both boreholes, i.e. RT-30 and RT-107), which corresponds to the lowest values of log$f_{\rm S_2}$
(–13) in the pentlandite stability field, to 0.52 (log$f_{\rm S_2}$
= –10.5) in RT-30 and up to 0.54 (log$f_{\rm S_2}$
= –10) in RT-107. These evolutionary changes are multidirectional in sections of picritic rocks from the different orebodies of the Oktyabr'sky deposit (Fig. 15). Our data shows that the Ni/(Ni + Fe) ratio of pentlandite and S in pyrrhotite increases with depth in the disseminated ore of the southern body (borehole RT-30 from 1501.2 m to 1527 m); consequently, the fugacity of sulfur increases and the evolution of the sulfide melt occurs in the same direction. In contrast, the picritic gabbro-dolerite from borehole RT-107 (northern C-4 orebody) shows an opposite zoning trend with Fe-enriched pentlandite and troilite characteristic of the deeper 1665.4 m horizon, and with the Ni/(Ni + Fe) ratios of pentlandite increasing upwards in the section (Fig. 7a,b). Consequently, the evolution of the sulfide melt increases from the bottom to the top along the picritic rock of the C-3 orebody (i.e. reverse zoning).
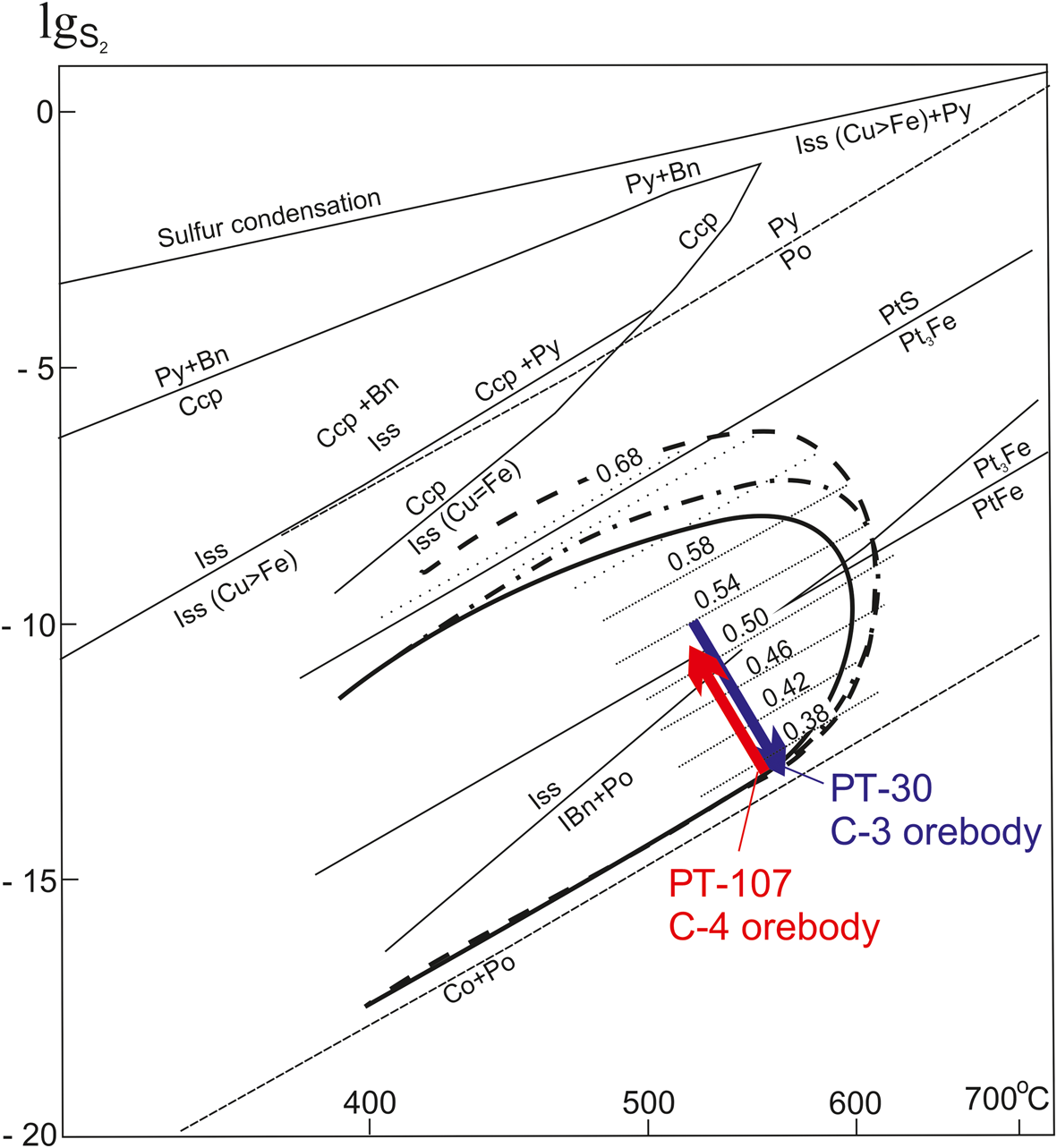
Figure 14. Physical and chemical conditions for the formation of pentlandite and Pt minerals relative to phase equilibria for the Cu − Fe − S and Ni − Fe – S systems (from Kolonin et al., Reference Kolonin, Orsoev, Sinyakova and Kislov2000). The solid elliptical curve is the boundary of the field of stability of pentlandite from Vaughan and Craig (Reference Vaughan and Craig1981), the dotted-dashed curve is from Kolonin et al. (Reference Kolonin, Orsoev, Sinyakova and Kislov2000), and the dotted curve from Peregoedova (Reference Peregoedova1999). The dotted lines within the pentlandite field indicate the changing value of k = Ni/(Ni + Fe) with decreasing temperature and increasing sulphur volatility. The vectors indicate the evolution of pentlandite in the picritic gabbro-dolerite from bottom to top along the cut of layers: RT-107 (red) and RT-30 (blue).
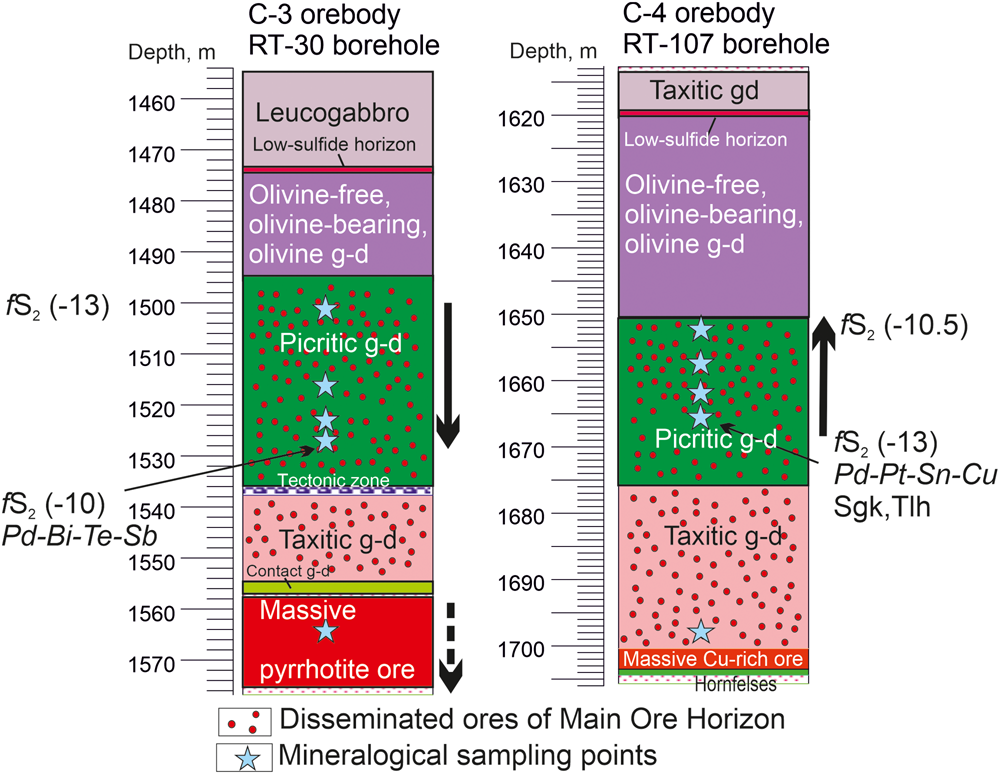
Figure 15. Parameters of sulfur volatility ($ f_{\rm S_2}$) and directions of evolution of the sulfide melt (trends) in the sections of the southern (RT-30) and northern (RT-107) orebodies of the Oktyabr'sky deposit. The distribution of minerals of various systems in the sections is shown by arrows. Sgk – sugakiite and Tlh – talnakhite.
Mineralogical zoning in the picritic gabbro-dolerite has been described previously and shown to be from a low-sulfur association with troilite, talnakhite, cubanite and Fe-rich pentlandite in the upper most magnesian part to a high-sulfur association at its base (Distler et al., Reference Distler, Sluzhenikin, Cabri, Krivolutskaya, Turovtsev, Golovanova, Mokhov, Knauf and Oleshkevich1999). The same direction of evolution of the sulfide parageneses from top to bottom was also observed in the picritic gabbro-dolerite of the Norilsk 1 intrusion (Tolstykh et al., Reference Tolstykh, Shvedov, Polonyankin and Korolyuk2020b, Reference Tolstykh, Garcia and Shvedov2021). Sluzhenikin (Reference Sluzhenikin2010) showed that in picritic rocks zoning is directed in both directions from the central part towards the top and bottom, in addition to relative increase in the sulfur content of parageneses.
The zoning of the disseminated ores in different boreholes as identified in this work coincides with the evolution vectors shown for the massive sulfide lenses with similar composition (Torgashin, Reference Torgashin1994; Fig. 3). These massive lenses also have opposite vertical mineralogical and geochemical zoning. The lenses of cubanite–chalcopyrite ore are characterised by an increase in incompatible elements from the bottom to the top along the section similar to that of the southern C-3 orebody. In contrast, the ores in the pyrrhotite domain evolve from the top to the bottom, along the vertical section as in the northern C-4 orebody.
PGE minerals from disseminated ore in the picritic gabbro-dolerite
The compositions of disseminated ores from different rocks and the paragenesis of sulfide minerals in the picritic and taxitic gabbro-dolerites have been established for the Norilsk 1, Oktyabr'sky and Talnakh deposits (Genkin et al., Reference Genkin, Kovalenker, Smirnov and Muravitskaya1977; Naldrett, Reference Naldrett2004; Distler et al., Reference Distler, Smirnov and Grokhovskaya1979, Reference Distler, Sluzhenikin, Cabri, Krivolutskaya, Turovtsev, Golovanova, Mokhov, Knauf and Oleshkevich1999; Spiridonov, Reference Spiridonov2010; Tolstykh et al., Reference Tolstykh, Shvedov, Polonyankin and Korolyuk2020b). Note that sometimes disseminated ores from the picritic and taxitic gabbro-dolerites are combined during research and considered together as one ‘Main Ore Horizon’ (Komarova et al., Reference Komarova, Kozyrev, Simonov, Lyul'ko and Cabri2002).
Using the example of the Norilsk 1 intrusion, we showed (Tolstykh et al., Reference Tolstykh, Garcia and Shvedov2021) that the picritic and taxitic gabbro-dolerites differ in mineral associations, although they are characterised by the same type of zoning in relation to PGMs. Namely, from Pd–Sn–Cu minerals common in the upper parts of both layers to the minerals of the Pd–Bi–Sb and Pd–Bi systems characteristic of the base of each layer, in accordance with the regressive temperature gradient and the direction of PGE fractionation in the sulfide melt. Such a distribution of PGMs characterises reverse zoning.
The PGM associations in the C-3 and C-4 orebodies are also unevenly distributed. At the base of the picritic gabbro-dolerite of the C-4 orebody, the leading minerals are high-temperature PGMs of the Pd–PT–Cu–Sn system: atokite–rustenburgite (Pd,Pt)3Sn; cabriite Pd2CuSn; and paolovite Pd2Sn, which correspond to direct zoning. Whereas, at the base of picritic gabbro-dolerite of the C-3 orebody, low-temperature PGMs of the Pd–Bi–Te–Sb system, e.g. sobolevskite Pd(Bi,Te), are widespread in accordance with the reverse zoning of the development of the ore-forming system (Fig. 15).
The modes of occurrences of Au and Ag also differ in that only silver telluride (sopcheite) was found in the southern C-3 orebody, whereas native alloys (Ag, Au and Cu) are typical for the northern C-4 orebody and are enriched in Au at a higher level. Thus, the PGE-bearing ore-forming systems of picritic gabbro-dolerite differ in different orebodies in the Oktyabr'sky deposit penetrated by RT-30 and RT-107 boreholes (Fig. 16a,b).

Figure 16. The ratio of platinum-group minerals in disseminated ores from picritic gabbro-dolerite from the southern (RT-30) and northern (RT-107) orebodies of the Oktyabr'sky deposit (a,b) and in massive ores (c).
Mineralogical similarities and differences between disseminated and massive ores in the southern orebodies.
Massive ore in the exocontact of the intrusion and disseminated ore in the picritic gabbro-dolerite of a section along the RT-30 borehole of the southern C-3 orebody have both differences and similarities. The main difference is in the features of PGM mineralisation. Whereas the Pd–Bi–Sb–Te minerals are common in the disseminated ores, the massive ores contain only Pt minerals, among which cooperite PtS is more common (Fig. 16c). This characteristic of massive ores makes them comparable with other pyrrhotite ores of the Norilsk region (Kozyrev et al., Reference Kozyrev, Komarova, Emelina, Oleshkevich, Yakovleva, Lyalinov, Maximov and Cabri2002), though not with disseminated ores in the picritic rocks.
Nevertheless, the similarity lies in the unidirectional mineralogical and geochemical zoning from the top to the bottom along the cross-section, due to the evolution of the sulfide melt (Fig. 15). It is assumed that zoning in the pyrrhotite ores of the C-3 body is analogous to zoning in the pyrrhotite lens of the Main Ore Body of the Oktyabr'sky deposit, where the concentration of Cu and incoherent elements increases down the section (Torgashin, Reference Torgashin1994, Fig. 3).
This unidirectional evolution seems logical if one follows the hypothesis that the massive ores are the result of the deposition of sulfide droplets from disseminated horizons and their infiltration into the underlying rocks through the taxitic and contact gabbro-dolerite. Barren hornfelses at the contact of disseminated and massive ores, sharp changes in sulfide parageneses between picritic and taxitic gabbro-dolerites (Tolstykh et al., Reference Tolstykh, Shvedov, Polonyankin and Korolyuk2020b), as well as a significant difference in PGM associations between disseminated and underlying massive ores do not comform to this hypothesis. If we consider disseminated and massive ores as different episodes of long-term magmatic activity (Zientek and Likhachev, Reference Zientek and Likhachev1992), then unidirectional trends (evolutionary zoning) of massive and disseminated ores can be caused by the action of similar mechanisms of intrusion of silicate magma (with droplets of sulfides) and sulfide melts.
Features of rare sulfides from various types of ores
Argentopentlandite Ag(Ni,Fe)8S8 occurs in taxitic gabbro-dolerite (RT-107_1695) of the C-4 orebody. This mineral was also found in various ores of the Norilsk deposits, and it can be associated concurrently with ordinary pentlandite and Ag-bearing pentlandite (Sluzhenikhin and Mokhov, Reference Sluzhenikin and Mokhov2014), as found in this study. Silver can only occupy an octahedral site in argentopentlandite, thus its amount should not exceed 1 apfu of Ag in the structure of the mineral (Hall and Stewart, Reference Hall and Stewart1973; Rudashevskii et al., Reference Rudashevskii, Mintkenov, Karpenkov and Shiskin1977), which corresponds to the formula Ag(Fe,Ni)8S8 or, more precisely, AgFe2+6Ni2S8, in which Fe predominates three times over Ni, which is also characteristic of the argentopentlandite investigated (Table 1). Argentopentlandite might be a product of exsolution from an Ag-bearing intermediate solid solution during the cooling of the ore-forming system according to one of the models described in Melfos et al. (Reference Melfos, Vavelidis and Arikas2001). Taking into account its morphological character of segregations as relatively large grains (60–70 μm) in the chalcopyrite matrix, located in the halo of small Ag-free pentlandite grains (Fig. 8), it can be assumed that argentopentlandite is an early mineral and crystallises under the less sulfuric conditions of the ore-forming system of the taxitic gabbro-dolerite. With a decrease in temperature and an increase in sulfur fugacity, small grains of more nickel-rich pentlandite crystallised from residual melts in the intergranular space surrounding chalcopyrite grains, the composition of which corresponds to the log$f_{\rm S_2}$ = –10 to –10.5.
Sugakiite as large (~80 μm) granular grains is common in the picritic gabbro-dolerite of the C-4 orebody. It is assumed that sugakiite crystallised due to the peritectic reaction of Mss with melt, similar to early pentlandite Pn1 (Distler et al., Reference Distler, Kulakov, Sluzhenikin and Laputina1996), though only under conditions of low sulfur activity, which is noted at the base of the picritic rocks in the RT-107 borehole. Sugakiite is not an exsolution product, as this mineral contains up to 0.9 apfu Cu that is in excess in the residual melt, but does not fractionate in Mss. The ratio Ni/(Ni + Fe) of sugakiite is the lowest (0.26–0.27) compared to pentlandite of all horizons of the picritic rock in borehole RT-107. Compositions of sugakiite are mostly moved to the ferruginous area of the diagram (Fig. 7a). This mineral intergrows with troilite, so it can be assumed that it crystallised under conditions of the lowest sulfur fugacity that were achieved at the base of the picritic layer of the C-4 orebody. Sugakiite is a very rare mineral of low sulfur association discovered in sulfide aggregates in a peridotite of the Horoman massif, Hokkaido, Japan (Kitakaze, Reference Kitakaze2008). Sugakiite has also been noted in picritic gabbro-dolerite of the C-6 orebody located in the northeastern part of the Oktyabr'sky deposit, in which the massive ores are also essentially pyrrhotite (Ketrov et al., Reference Ketrov, Yudovskaya, Shelukhina, Velivetskaya and Palamarchuk2022).
Origin of different types of sulfide ores
There is a hypothesis that the Kharaelakh intrusion is a single horizontal channel of magma supplied to the surface (chonolith), in which, upon interaction with the host rocks, a sulfide melt segregated and formed the orebodies (Rad'ko, Reference Rad'ko1991). Others have suggested two-stage (Zolotukhin et al., Reference Zolotukhin, Ryabov, Vasil'ev and Shatkov1975), three-stage (Turovtsev, Reference Turovtsev2002; Malitch et al. Reference Malich, Belousova, Griffin, Badanina, Pearson, Presnyakov and Tuganova2010) or multipulse (Zientek and Likhachev, Reference Zientek and Likhachev1992; Likhachev, Reference Likhachev1996) formation of the Kharaelakh intrusion. An extended period of magmatic activity from Middle Palaeozoic to Early Mesozoic consistent with multiple magmatic events during the evolution of the Kharaelakh intrusion has been assumed. The interaction of juvenile and crustal magmatic sources with a mantle origin based on Hf–Nd isotope data has been established (Malich et al., Reference Malich, Belousova, Griffin, Badanina, Pearson, Presnyakov and Tuganova2010). The multipulse concept is consistent with our mineralogical studies of the central part of the Oktyabr'sky deposit, where the intrusion and crystallisation of differently fractionated melts was occurring in the southern and northern orebodies. Each magma pulse generated its own cooling front on a local scale, forming forward zoning in the northern C-4 orebody, and reverse zoning in the southern C-3 orebody. The high ore/silicate imbalance and complex mineralogical and geochemical zoning confirms a dynamic mode for the origin of ore-bearing intrusions and a pulsed nature of magma intrusion with different evolutionary histories of the sulfide melts in the С-3 and C-4 orebodies.
It is assumed that the disseminated ores were formed as a result of the intrusion of silicate magma containing sulfide liquid, the segregation of which occurred throughout the entire period of existence of the magmatic melt (Zientek and Likhachev, Reference Zientek and Likhachev1992; Likhachev, Reference Likhachev1973, Reference Likhachev2006). Volatiles in the magma played a significant role in the carrying capacity of rising magma in transporting sulfide droplets. To explain the formation of globular disseminated ores in picritic gabbro-dolerites, models of the so-called ‘segregation vesicles’ attached to sulfide liquid droplets have been proposed. Segregation vesicle disruption is considered to have occurred in situ at low pressures, resulting in the release of sulfide liquids that could accumulate in traps. However, ‘bubble-rafting’ according to Yao et al. (Reference Yao, Mungall and Qin2019) and Barnes et al. (Reference Barnes, Vaillant, Godel and Lesher2019) was not the predominant mechanism of ore formation. It has been noted (Yao et al., Reference Yao, Mungall and Qin2019) that the mechanisms of evaporite assimilation in situ and high R-factor do not need to be involved for the formation of disseminated ores.
The formation of massive ores, according to Likhachev (Reference Likhachev2006) is the result of sulfide liquid settling in widened sections of magma channels, where magma flow slowed. In our case, it is also unlikely that massive ores could be a result of permanent settling of sulfide droplets to the bottom of the formation chamber and the penetration of the accumulated sulfide melt through the layers of taxitic and contact gabrodolerites into the underlying rocks. The arguments are: (1) disseminated ores in picritic gabbro-dolerites and massive ores have completely different sulfide assemblages and PGM compositions; (2) there is a distinct geochemical and mineralogical contrast between picritic and the underlying taxitic ores (Tolstykh et al., Reference Tolstykh, Shvedov, Polonyankin and Zemlyanssky2017, Reference Tolstykh, Shvedov, Polonyankin and Korolyuk2020b, Reference Tolstykh, Garcia and Shvedov2021), rather than gradual changes in composition, as would occur with sequential droplet deposition; and (3) there is a gap between disseminated and massive ores, containing a layer of barren rocks. There is also a hypothesis that disseminated ore in the gabbro-dolerites are enriched in ‘fractional sulfide fluid coming from underlying massive sulfide ores’ (Naldrett, Reference Naldrett2004). This seems unlikely as there is a layer of barren hornfelses between the massive ore and taxitic gabbro-dolerite (Fig. 5).
It is obvious that the formation of disseminated and massive ores are fragments of two different intrusion processes from one intermediate chamber as the geochemical features, namely the PGE distribution patterns, are the same for all types of ores, but with different degrees of element fractionation (Tolstykh et al., Reference Tolstykh, Shvedov, Polonyankin and Korolyuk2020b). It can be assumed that the magmatic system first functioned as an open system and filled the pools in a single act in the case of direct zoning in the C-4 orebody or multi-act in the case of reverse zoning in the C-3 orebody. Subsequently, the magmatic system could close, giving preference to crystallisation fractionation at the final stage.
The formation of reverse zoning in the marginal parts of layered intrusions is a controversial issue (Latypov et al., Reference Latypov, Chistyakova and Alapieti2007, Reference Latypov, Hanski, Lavrenchuk, Huhma and Havela2011; Latypov and Egorova, Reference Latypov and Egorova2012; Egorova and Latypov, Reference Egorova and Latypov2013; Egorova and Shelepayev, Reference Egorova and Shelepaev2020 and references therein). These authors summarised numerous reasons potentially leading to the reverse zoning, suggesting, among other ideas, that top-down crystallisation of ore matter was due to: (1) the floating of early Mss crystals towards the roof; (2) a progressive decrease in the amount of trapped residual liquid; or (3) top-down solidification from a cold roof rock. However, these factors are debatable and in each specific case they might vary in importance.
In our opinion, the most appropriate petrological mechanisms for reverse zoning formation is the filling of the magma chamber with the continuous arrival of increasingly primitive melt, which was fractionated in the supply channel or in the intermediate chamber (Egorova and Latypov, Reference Egorova and Latypov2013). This mechanism is most consistent with the zoning in the C-3 orebody, and observed in the rocks in the Norilsk 1 intrusion (Tolstykh et al., Reference Tolstykh, Shvedov, Polonyankin and Korolyuk2020b).
The process of fractional crystallisation was most probably the leading one, as both types of zoning (direct and reverse) correspond to the evolution of the sulfide melt. In the first case in situ (in the chamber of formation), and in the second in an intermediate chamber or supply channel, if we accept the hypothesis of a continuous flow of increasingly primitive melts (Latypov et al., Reference Latypov, Chistyakova and Alapieti2007, Reference Latypov, Hanski, Lavrenchuk, Huhma and Havela2011).
Conclusions
The southern C-3 orebody of massive ores penetrated by borehole RT-30, is composed mainly of pyrrhotite, and is comparable to the pyrrhotite lenses, whereas the northern C-4 orebody, composed of talnakhite–chalcopyrite ores penetrated by the RT-107 borehole, can be compared with the Cu-rich lenses of the Main Ore Body of the Oktyabr'sky deposit.
The southern and northern orebodies differ in their mineral compositions. The former is characterised by a high-sulfur association, and the latter contains minerals with a deficit of sulfur. Multidirectional evolution of the ore-forming systems is observed in the disseminated ores of these deposits. In the northern C-4 orebody direct crystallisation zoning is observed, where the fugacity of sulfur increases from the bottom to the top, whereas in the southern C-3 orebody $f_{\rm S_2}$ decreases in this direction. The zoning coincides with the evolutionary vectors of various ‘blocks’ of the massive Main Ore Body ores of the Oktyabr'sky deposit. The presence of the rare mineral, sugakiite, in the lower part of the picritic gabbro-dolerite of the northern orebody is confirmed by the extremely low values of sulfur fugacity, which increase upwards in the section.
Platinum-group mineral associations and modes of occurrence of Au and Ag in the northern and southern orebodies also differ and correspond to sulfide zoning. In the lower horizon of picritic gabbro-dolerite of the northern C-4 orebody. The leading compounds are high-temperature PGM of Pd–PT–Cu–Sn systems in accordance with direct zoning, whereas in the lower horizon of the southern C-3 orebody the low-temperature PGMs of the Pd–Bi–Te–Sb system are widespread in accordance with the reverse zoning of the evolution of ore-forming systems.
Disseminated and massive ores within a single cross-section of borehole RT-30 of the southern orebody are different in mineral associations in that the former is characterised by minerals of the Pd–Bi–Sb–Te system, whereas the latter is characterised only by Pt-minerals. The similarity of these ores lies in the unidirectional reverse mineralogical and geochemical zoning along the cross-sections in both disseminated and massive ores, which are the result of different magmatic events. However, unidirectional zoning of both types of ores might be due to the action of the same type of mechanism.
All the above data indicate that the formation of the southern C-3 and northern C-4 orebody occurred under different physico-chemical conditions as the result of the intrusion of various portions of both ore-silicate magmas, from which disseminated ores were formed, together with sulfide melt, previously separated into copper-rich and iron-rich fractions in the intermediate chamber. Each of the portions entered the formation chambers through different magma channels.
Acknowledgments
We thank analysts M. Khlestov for carrying out analytical procedures and providing quantitative analyses, and N. Belkina and M. Podlipsky for technical assistance in the manuscript preparing. Funding: This research was carried out within the framework of the state assignment of the IGM SB RAS financed by the Ministry of Science and Higher Education of the Russian Federation, and project of the Ministry of Science and Higher Education of the Russian Federation No. 13.1902.21.0018. The authors thank the Associate Editor David Good and the reviewers for their assistance in the evaluation of this paper.
Competing interests
The authors declare none.