Introduction
The head neck site is the most prevalent site for the malignant neoplasm, mainly affecting oral tissues. Reference Ferlay, Steliarova-Foucher and Lortet-Tieulent1,Reference Ferlay, Soerjomataram and Dikshit2 In most cases, treatment options are either surgery or radiotherapy, and in some cases, combination therapy is required. In radiotherapy, ionising radiation destroys tumour cells and some normal cells too. Reference Baskar, Dai, Wenlong, Yeo and Yeoh3,Reference Kawashita, Soutome, Umeda and Saito4
The oral management of patients undergoing orofacial radiotherapy requires a challenging multidisciplinary approach to restoring dental caries. Reference Beech, Robinson, Porceddu and Batstone5,Reference Cho and Kumar6 Restoration of dental caries before radiotherapy is of utmost importance to prevent dental caries’ progression and reduce the load of microorganisms. Reference Murdoch-Kinch and Zwetchkenbaum7 This perspective of dentists towards restoring dental caries before radiotherapy is vital because adhesion between the enamel/dentin and restorative material will be weakened by ionising radiation so that post-radiotherapy restoration might be unsuccessful treatment. Reference Naves, Novais, Armstrong, Correr-Sobrinho and Soares8
Metallic restorations, namely dental amalgam and indirect cast restorations, potentially increase the incidence of radio-mucositis caused by backscattering of secondary radiation; thus, adhesive materials, i.e., resin composites and glass ionomer cement (GIC) are preferred in such patients. Reference Biscaro, Moraes, Correr, Almeida, Bóscolo, Soares and Correr-Sobrinho9 Resin composites are aesthetic, require a conservative tooth preparation and have proven to prevent recurrent decay with sufficiently long retention periods in irradiated patients. Reference Bodrumlu, Bodrumlu, Avşar and Meydan10 However, because of polymerisation shrinkage, unreacted monomer and biocompatibility issues with composites are the main reasons not to be the first choice of dentists. Reference Soares, Faria-e-Silva and Rodrigues11
Conventional GICs are the first choice for dentists because of anti-cariogenic activity, better bonding to enamel/dentine and long-term fluoride release. Reference Menezes-Silva, Cabral and Pascotto12 However, low tensile strength and low fracture toughness are the main constraints because of dehydration. Reference Khoroushi and Keshani13
Studies have been conducted to evaluate the bond strength or adaptation of restorative materials with irradiated or non-irradiated tooth surfaces. Reference Carvalho, van Amerongen, de Gee, Bönecker and Sampaio14 Miranda et al. Reference de Miranda, Silva, Dantas, Soares and Novais15 evaluated the effect of in vivo radiotherapy on the chemical properties of human dentine by Fourier-transform infrared spectroscopy (FTIR) and Raman analysis. They observed that radiotherapy altered the chemical composition of human dentin and has higher concentration of organic components after radiotherapy because of the exchange of phosphate-carbonate ions in the hydroxyapatite. Alternatively, the effect of irradiated restorative materials on these very few studies has evaluated the effect of irradiation on the tooth–restoration interface of already placed restorations. Reference Davidson16,Reference De Andrade, Moura, Reis, Loguercio, Garcia and Grande17
This study was thus carried out to evaluate the effect of gamma radiation on the shear bond strength and marginal adaptation of three posterior resin composites and two GIC to coronal dentin of extracted human teeth. The null hypothesis was that gamma radiation would not alter the shear bond strength and marginal adaptation of material used.
Materials and Methods
One hundred freshly extracted human permanent mandibular molar teeth were collected and cleaned of debris and stored in 0·2% thymol solution. These were randomly divided into two groups of fifty each to be tested for shear bond strength of restoration to dentin and the marginal gap at the tooth–restoration interface.
Shear bond strength test
The teeth were bisected in the buccolingual direction using a diamond disk. One-half of each tooth was allocated to the radiotherapy group and the other half to the non-radiotherapy group. The exposed dentin surface of each half was polished with 200-, 400- and 600-grit silicon carbide abrasive papers. The roots of the teeth were mounted in self-cure acrylic resin, with an occlusal surface parallel to the floor. The specimens of each of the groups (with and without radiotherapy) were divided into five subgroups of ten samples each based on the restorative materials used
-
– Group Ia – Restored with Filtek Z250 Universal Restorative resin composite (3M, ESPE, USA).
-
– Group Ib – Restored with Filtek Z350XT Universal Restorative resin composite (3M, ESPE, USA)
-
– Group Ic – Restored with Filtek Bulk Fill Posterior Restorative resin composite (3M, ESPE, USA)
-
– Group IIa – Restored with Ketac Molar GIC (3M, ESPE, USA)
-
– Group IIb – Restored with Ketac Universal GIC (3M, ESPE, USA)
Resin composite build-up for shear bond strength evaluation
Inner dentin surface was etched using Scotch bond™ Etchant (3M, ESPE, USA) for 15 s, rinsed with water for 10 s and blow-dried. Before the application of the adhesive, a transparent plastic tube (2 mm in diameter and 5 mm in height) was placed on the prepared inner dentin surface, and the outer diameter was marked for the application of the bonding agent. The plastic tube was removed, and adhesive Adper™ Single Bond Plus (3M ESPE, USA) was light cured for 10 s. Resin composite build-up was done in increments of 2·5 mm to fill transparent plastic tube and cured with LED light (3M, ESPE, USA) for 20 s.
Glass ionomer cement build-up
After placing the transparent plastic tube on the inner dentin surface, GIC was mixed and placed according to the manufacturer’s instructions.
Radiotherapy was initiated 24 h after the completion of restorations. Fifty out of 100 prepared specimens were subjected to fractionated gamma radiation, and the rest stored in distilled water till the completion of radiotherapy. For radiotherapy, specimens were placed in a plastic container filled with distilled water to a level 0·5 mm above resin blocks (in order to bring the maximum dose to the level of the specimen) and exposed to Co-60 gamma rays from the telecobalt therapy unit (Theraton 780 C, AECL, Ottawa) at field size of 10 × 8 cm2 and standard SSD (Source water distance) 80 cm. For six weeks, a fractionated dose of 60 Gy, using 2 grays per fraction five times/week, was given. After completion of radiotherapy, both irradiated and non-irradiated specimens were stored in distilled water at room temperature till further tests.
Evaluation of shear bond strength
After a total of 7 weeks of restoration (6 weeks of radiotherapy and 1 week of storage), shear bond strength tests were performed on a universal testing machine (Instron, Unitek 9450, USA). The specimens were oriented in a holding device, and the loading head was perpendicular to the composite cylinder, less than 0·25 mm from the tooth surface. Tests were performed at a cross-head rate of 1 mm/minute until the tooth’s restorative material cylinder was dislodged. Shear bond strength was calculated as the fracture load and bonding area ratio, expressed in megapascals (Mpa).


where π is the constant = 3·14 and r is the radius of restoration = 1 mm
Marginal adaptation
For evaluation of marginal gap at the interface, cavities on the gingival one-third of buccal surface (Class V) were prepared and restored with either resin composites or GIC (Figure 1 ). The roots of the teeth were mounted in acrylic resin, with an occlusal surface parallel to the floor. A rectangular box of dimensions 3 mm long and 2 mm wide was cut in a matrix band for standardised Class V cavities preparation. The depth was predetermined using a rubber stopper fixed to the bur and drilled to 1·5 mm. Finally, the prepared cavities of 3 mm length in mesiodistal direction, 2 mm width in occlusal-cervical direction and 1·5 mm depth were obtained. After preparation, the teeth were stored in distilled water in glass containers until restorations. The standardised class V cavities were restored with respective materials (resin composite or GIC) according to the manufacturer’s instructions, either radiotherapy or non-radiotherapy group.
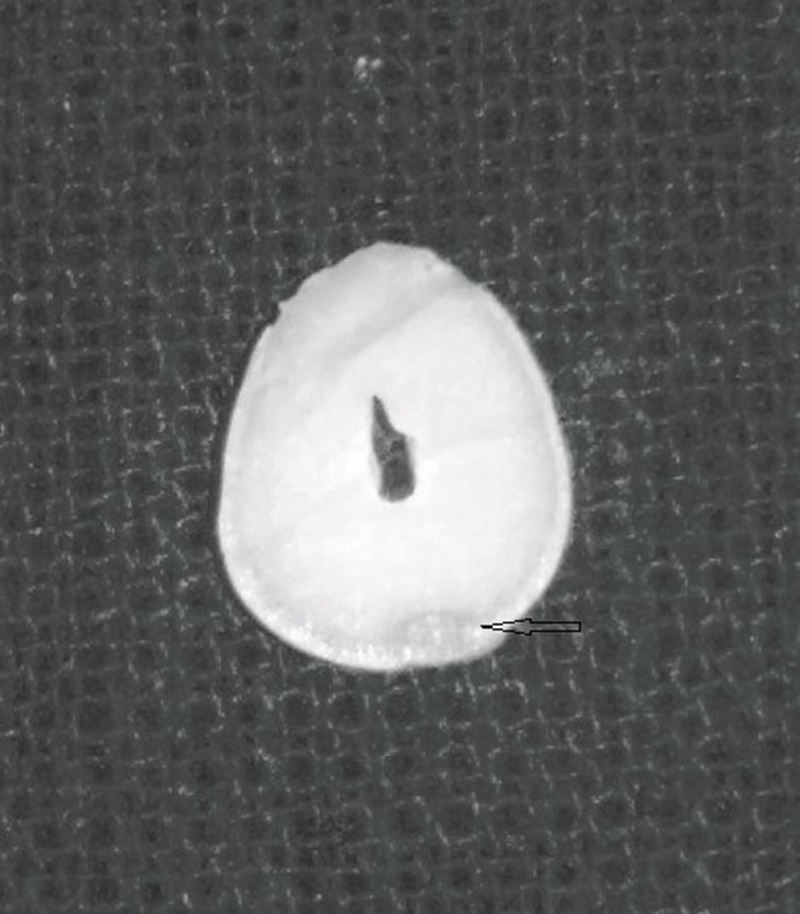
Figure 1. Transverse section of restored molar for SEM analysis. The arrow shows the tooth–restoration interface.
The specimens (n = 50) were divided into five subgroups (n = 10/sub-group) based on the restorative material used, as before.
All specimens with class V restorations were sectioned horizontally at the lower margin of restoration to obtain 2 mm thick disc-like sections, and tooth–restoration interfaces were examined under a 1000× scanning electron microscope (SEM). The photomicrographs were evaluated for the marginal gap between different restorative materials and dentin using the IMAGE J software system (developed at the National Institutes of Health). Measurements at fifty randomly selected sites across the gap were made to evaluate marginal gap at interface followed by statistical evaluation of their means, in accordance with de Siqueira et al., who suggested that 50 measurements give adequate information about gap size regardless of whether the measurement sites are selected in a systematic or random manner. Reference De Siqueira Mellara, Palma-Dibb and de Oliveira18
Statistical analysis
Data were summarised as Mean ± SD (standard deviation). Groups were compared by two-way analysis of variance (ANOVA), and the significance of mean difference within (intra) and between (inter) the groups was done by Tukey’s HSD post-hoc test after ascertaining normality by Shapiro–Wilk’s test and homogeneity of variance by Levene’s test. A two-tailed (α = 2) p-value less than 0·05 (p < 0·05) was considered statistically significant. Analysis was performed on SPSS version 16 (SPSS Inc., Chicago, IL, USA).
Results
The shear bond strength (Mpa) of two groups (without radiotherapy and with radiotherapy) is summarised in Table 1. For all restorations, the mean shear bond strength was higher without radiotherapy as compared with radiotherapy as follows Ketac Universal<Ketac Molar< Bulk Fill< Z350 < Z250 and with radiotherapy Ketac Molar<Ketac Universal<Z350 < Z250 < Bulk Fill. For each group, comparing the mean shear bond strength between the restorations, Tukey’s test showed significantly (p < 0·001) different and higher shear bond strength without radiotherapy. However, without radiotherapy, the mean shear bond strength does not differ (p > 0·05) between Ia and Ib, Ia and Ic, Ib and Ic and IIa and IIb, i.e., found to be statistically the same.
Table 1. The shear bond strength (Mean ± SD) of two groups (without radiation and with radiation) for five different restorative materials

With radiotherapy, the mean shear bond strength of Ia, Ib and Ic was also found to be significantly (p < 0·001) different and higher as compared with both IIa and IIb. With radiotherapy, the mean bond strength of both Ia and Ic was also significantly (p < 0·001) different and higher than Ib. However, with radiotherapy, the mean shear bond strength did not differ (p > 0·05) between Ia and Ic and IIa and IIb, i.e., found to be statistically the same.
Marginal gap evaluation: ANOVA showed a significant effect of both radiotherapy (F = 40·33, p < 0·001) and restorations (134·00, p < 0·001) on the marginal gap at the interface.
The marginal gap at the interface (μm) of tooth restoration of two groups (without radiotherapy and with radiotherapy) for five different restorations is summarised in Table 2. Without radiotherapy, the mean marginal gap at interface of restoration was Z250 < Z350 < Bulk Fill<Ketac Universal<Ketac Molar (Figures 2a and 2b) and with radiotherapy: Bulk Fill<Z250 < Z350 < Ketac Universal<Ketac Molar (Figures 3a and 3b). For each restoration, mean marginal gap at interface between the groups, Tukey test showed significantly (p < 0·001) different and the higher marginal gap at the interface with radiotherapy as compared to without radiotherapy at all restorations except Filtek Bulk Fill (Figure 4).
Table 2. The marginal gap at the interface (Mean ± SD) of two groups at five different restorations (μm)


Figure 2. (a) SEM image depicting sample with the least marginal gap (without irradiation) in Z250. (b) SEM image depicting maximum marginal gap (without irradiation) in Ketac Molar.

Figure 3. (a) SEM image depicting least marginal gap (with irradiation) in Filtek Bulkfill. (b) SEM image depicting maximum marginal gap (with irradiation) in Ketac Molar.

Figure 4. Mean marginal gap at interface of radiated and non-irradiated groups for five different restorations.
Discussion
The specimens were irradiated with 60 Gy in the present study, using a fractionated dose of 2 grays five times a week, simulating therapeutic irradiation. Reference Geoffroy and Tochon-Danguy19 To simulate oral clinical conditions, specimens were irradiated in distilled water. Reference Gogineni, Kargiotis, Klopfenstein, Gujrati, Dinh and Rao20 Other media used include 0·9% saline solution, Reference Grotz, Duschner, Kutzner, Thelen and Wagner21 artificial saliva or buffered phosphate solution. Reference de Miranda, Silva, Dantas, Soares and Novais15
Higher shear bond strength of resin composites than GIC without radiotherapy agrees with previous studies. Reference Harhash22,Reference Hersek, Canay, Akça and Çiftçi23 Biscaro et al. Reference Biscaro, Moraes, Correr, Almeida, Bóscolo, Soares and Correr-Sobrinho9 reported the dose-dependent detrimental effect on the bond strength of resin composite restorations to dentin post x-ray radiation. The increasing doses may reduce the frictional forces between the polymer chains, leading to reduced material strength. This radiotherapy-induced damage of collagen fibers could result in impaired bond strength between composite and dentin. Reference Naves, Novais, Armstrong, Correr-Sobrinho and Soares8 Interestingly, a significant increase in the bond strength values for nano-composite restorative materials after gamma irradiation has also been reported, owing to the continued polymerisation arising from the incident therapeutic radiotherapy beam, leading to the increased degree of polymerisation. Reference Korkmaz, Gurgan, Firat and Nathanson24
In the present study, the least effect of irradiation on shear bond strength to dentin was observed in Filtek Bulk fill (23·2% reduction), which was, however, statistically insignificant (p > 0·05) than the effect seen on Filtek Z250 (28·1% reduction). Also, the increase in the marginal gap at the interface after radiotherapy was statistically insignificant (p > 0·05) in Filtek Bulk Fill resin composite compared with without radiotherapy. The presence of a high molecular weight aromatic dimethacrylate (AUDMA) in Bulk Fill decreases the number of reactive groups in the resin, reducing the volumetric shrinkage and the stiffness of the developing and final polymer matrix.
Radiotherapy-induced damage to collagen fibres impairs bond strength between dentin and composite, thus affecting the marginal adaptation. Reference Bodrumlu, Bodrumlu, Avşar and Meydan10,Reference Hegde, Hegde, Suchetha Kumari and Ganesh Sanjeev25 Cruz et al. Reference Cruz, Sinhoreti, Ambrosano, Rastelli, Bagnato and Boscolo26 investigated the influence of therapeutic doses of X rays on the microhardness and degree of conversion of resin composites. They concluded that when the irradiation occurred before the photo-activation, excitation points were created in the organic matrix of the material. Due to high mobility among these chemical groups, more bond links occurred, resulting in an increase in microhardness. While, when the irradiation occurred after photo-activation, there were excitations, but as the chains were already linked, there was a breaking of bonds due to high radiation energy and rigid structural configuration. Thus, impaired bonding and breakage of formed bonds can possibly explain the increase in marginal gap in resin composite groups in the present study.
Radiotherapy is associated with the upregulation of matrix metalloproteinases (MMPs) in the lung, brain and colon. Reference Gogineni, Kargiotis, Klopfenstein, Gujrati, Dinh and Rao20,Reference Araya, Maruyama and Sassa27 This radiotherapy-induced activation of MMPs in the dentin matrix could lead to debonding at the tooth–restoration interface and result in a higher marginal gap post-radiotherapy. Reference Satoyoshi, Kawata, Koizumi, Inoue, Itohara, Teranaka and Mikuni-Takagaki28
GICs have a typical double-setting reaction whereby the initial setting occurs within few minutes, but the subsequent reaction is slow. This second phase involves ion exchange and can continue for weeks or even months. Reference Davidson16 Gamma irradiation may disrupt the chemical exchange, thus affecting final maturity. This hampers bonding between leached ions from the glass ionomer matrix and calcium and phosphate ions in dentin. In this study, GIC restorations were exposed to gamma irradiation after 24 h of being restored onto the tooth surface. This might have led to incomplete ion exchange and maturity of the bond. Thus, a significant increase in marginal gaps and decreased bond strength post-radiotherapy were noted. Yesilyurt et al., Reference Yesilyurt, Bulucu, Sezen, Bulut and Celik29 in an in vitro study, concluded that if irradiation was applied after GIC is bonded to dentin, radiotherapy might affect the chemical bonding of GIC to dentin; however, on examination of the effect of gamma irradiation on set GIC using FTIR, no statistically significant difference was found in the spectra of radiotherapy and non-radiotherapy specimens.
Conclusion
Within the limitations of this study, composites showed better results than GIC both in terms of marginal adaptation and shear bond strength and may be considered to restore carious lesions before the onset of radiotherapy in patients with head and neck cancer. The adverse effect of radiotherapy on the physical properties of these restorations warrants that the treating radiation oncologist and restorative dentist pre-discuss the dental restorative needs of the patient before the onset of radiotherapy. More clinical studies with suitable sample size and adequate follow-up time are warranted to evaluate the effects of irradiation in patients undergoing radiotherapy.
Acknowledgements
None.
Financial Support
None.