1 Introduction
Due to its good spectroscopic properties, high solubility to rare earth ions, large damage threshold and easier to be produced with large size,
$\text{Nd}^{3+}$
-doped phosphate glasses are widely used as gain media for high-peak-power lasers for inertial confinement fusion (ICF) research[Reference Campbell, Hayden and Marker1–Reference Campbell and Suratwala3]. In this laser system with Million Joules energy output, laser glass must bear high energy density above
$10~\text{J}~\text{cm}^{-2}$
at 1053 nm wavelength.
$\text{P}_{2}\text{O}_{5}\text{-}\text{Al}_{2}\text{O}_{3}\text{-}\text{M}_{2}\text{O}\text{-}\text{MO}$
is the most suitable metaphosphate glass system till now for the application in high-peak-power laser facility, in which
$\text{M}_{2}\text{O}$
can be either
$\text{K}_{2}\text{O}$
or the mixture of alkali oxides. MO can be one of alkaline oxide or the mixture of alkaline oxides. Commercial phosphate laser glasses, such as LG-770 (from Schott of America) and LHG-8 (from Hoya of Japan), have been used in NIF (US) and LMJ (France) laser ICF facilities[Reference Arbuzov, Fyodorov, Kramarev, Lunter, Nikitina, Pozharskii, Shashkin, Semyonov, Ter-Nersesyants, Charukhchev, Sirazetdinov, Garanin and Sukharev4]. N31 glass developed in Shanghai Institute of Optics and Fine Mechanics (SIOM), China, has been used in Shen Guang laser facilities[Reference Hu, Chen, Tang, Wang, Meng, Chen, Wen, Hu, Li, Xu, Jiang, Zhang and Jiang5]. All these phosphate glasses have the emission cross section about
$(3.6{-}3.9)\times 10^{-20}~\text{cm}^{2}$
and nonlinear refractive indices
$(1.02{-}1.18)\times 10^{-13}$
esu. Mass production of LHG-8, LG-770 and N31 phosphate laser glasses has been realized by continuous melting technique[Reference Hu, Chen, Tang, Wang, Meng, Chen, Wen, Hu, Li, Xu, Jiang, Zhang and Jiang5, Reference Campbell, Suratwala, Thorsness, Hayden, Thorne, Cimino, Marker, Takeuchi, Smolley and Ficini-Dorn6].
Table 1. Thermal–mechanical properties of silico-phosphate glasses and N31 glass.

With the development of laser technology, the application requirement is increasing in many fields, such as laser processing working at lower repetition rate, ultrafast laser system with Exawatt (simplified as EW) output, and even high-peak-power demand in ICF laser facility. The laser with 1-5Hz/50-100J output has been widely used in laser peening field[Reference Dane, Hackel, Daly and Harrisson7, Reference Dane, Hackel, Halpin, Daly, Harrisson and Harris8]. Glass laser with 0.1-10Hz/30-200J has been used as pump laser source for CPA or Ti: sapphire laser system to construct ultra-short Petawatt (simplified as PW) system[Reference Kuzmin, Kulagin, Khazanov and Shaykin9–Reference Zou, Le Blanc, Papadopoulos, Chériaux, Georges, Mennerat, Druon, Lecherbourg, Pellegrina, Ramirez, Giambruno, Fréneaux, Leconte, Badarau, Boudenne, Fournet, Valloton, Paillard, Veray, Pina, Monot, Chambaret, Martin, Mathieu, Audebert and Amiranoff11], and hybrid glass (laser glasses with different emission bandwidths) laser was proposed as the most promising approach to realize EW laser[Reference Gaul, Martinez, Blakeney, Jochmann, Ringuette, Hammond, Borger, Escamilla, Douglas, Henderson, Dyer, Erlandson, Cross, Caird, Ebbers and Ditmire12]. In addition, concerning the even higher output energy requirements in high-peak-power ICF laser facility, the nonlinear optical damage will be a bottle neck to the whole laser system. Gain medium with low nonlinear refractive index
$n_{2}$
is needed to decrease the B integral (a parameter that represents the cumulative nonlinear phase retardation over the optical path length)[Reference Wen and Fan13] in Megajoule ICF laser facility. Therefore, fluorophosphate laser glass with low
$n_{2}$
was developed to meet the future development of high-peak-power laser system. On the other hand, new
$\text{Nd}^{3+}$
-doped laser glasses should be developed to meet the different requirements from the different laser applications.
Through compositional designs and identification of special post-processing treatments, new active glasses with tailored properties have been continuously developed for specific laser architectures around the world[Reference Hu, Chen, Tang, Wang, Meng, Chen, Wen, Hu, Li, Xu, Jiang, Zhang and Jiang5, Reference Hayden14]. In recent years, three kinds of new
$\text{Nd}^{3+}$
-doped laser glasses have been studied and developed in SIOM, China. In this work, the compositions, main properties and applications of these neodymium laser glasses have been discussed.
2 Working principle
Phosphate glasses were prepared by conventional pot-melting technique in air atmosphere. All starting materials were introduced with high purity powder reagent. 2000 g raw materials of phosphate glass were weighted and mixed thoroughly and then melted in quartz crucibles at different temperature according to the compositions, and dehydrated with
$\text{O}_{2}$
or
$\text{N}_{2}$
, and then poured into an airtight platinum crucible for refining and stirring. Afterward the melts were casted onto a preheated steel plate and annealed in a furnace around the glass transition temperatures and cooled gradually to room temperature. Similarly, fluorophosphate glasses were prepared in airtight platinum too, but the humidity of atmosphere should be controlled in the melting process. All samples were cut and polished carefully with the size of
$25~\text{mm}\times 15~\text{mm}\times 5~\text{mm}$
for optical property measurements.
The densities were measured through the Archimedes method using distilled water as an immersion liquid, and refractive indices were achieved by the prism minimum deviation method. The absorption spectra were recorded with a PerkinElmer Lambda 900 UV/VIS/NIR spectrophotometer in the range of 200–1000 nm, while the emission spectra were obtained with a Triax 320 type spectrometer in the range of 1000–1200 nm excited at 808 nm by Xenon lamp. All measurements were carried out at room temperature. The thermo-optic parameters were determined using Mach–Zehnder interferometer with a 632.8 nm He–Ne laser on glass samples sized
$\unicode[STIX]{x1D711}6\times 50$
mm. The stimulated emission cross section (
$\unicode[STIX]{x1D70E}_{\text{em}}$
) and radiative lifetime for the
$^{4}\text{F}_{3/2}\rightarrow ^{4}\text{I}_{11/2}$
transition are calculated by the J–O theory based on measured refractive index of glass, ion concentration of rare earth ions as well as absorption and fluorescence spectra[Reference Judd15].
3 Silico-phosphate neodymium laser glasses with high thermal shock resistance
Nd:phosphate glass with high thermal shock resistance is usually used in high-average-power laser system. Typical application is laser peening processing. Figure of merit of high-average-power Nd:phosphate glass is shown in


where
$E$
is Young’s modulus,
$\unicode[STIX]{x1D70E}_{\max }$
is fracture strength,
$K$
is thermal conductivity,
$v$
is Poson’s ratio.
$\unicode[STIX]{x1D6FC}$
is linear thermal expansion coefficient.
$K_{\mathit{Ic}}$
is the fracture toughness,
$a$
is the flaw depth in glass.
For applications in high-average-power laser, the Nd:phosphate glass works in lower repetition rate, such as 0.1–15 Hz, then a high thermal shock resistance is needed. According to Equation (1), the solution is to maximize the thermal conductivity and fracture toughness, minimize the coefficient of thermal expansion and Young’s modulus. On the other hand, emission cross section should be optimized to ensure the gain property. In addition, glass should have low temperature coefficient of the optical path length to reduce the thermal distortion effect in Nd:glass in repetition case. Consequently, considerable efforts have been done on optimizing the glass compositions of this kind of Nd:glass[Reference Chen, He, Hu, Li and Cheng16, Reference Li, He, Li, Chen and Hu17].
Table 2. Emission cross section and thermal–optical properties of
$\text{Nd}^{3+}$
-doped
$\text{P}_{2}\text{O}_{5}\text{-}\text{Al}_{2}\text{O}_{3}\text{-}\text{BaO}\text{-}\text{K}_{2}\text{O}\text{-}\text{Li}_{2}\text{O}$
phosphate glass.

In order to increase the thermal conductivity and reduce the thermal expansion coefficient, phosphate glass system,
$\text{P}_{2}\text{O}_{5}\text{-}\text{Li}_{2}\text{O}\text{-}\text{MgO}\text{-}\text{Al}_{2}\text{O}_{3}\text{-}\text{SiO}_{2}$
, was chosen. Six kinds of (65-
$x$
)
$\text{P}_{2}\text{O}_{5}\text{-}x\text{SiO}_{2}\text{-}15\text{Al}_{2}\text{O}_{3}\text{-}10\text{Li}_{2}\text{O}\text{-}10\text{MgO}$
glasses (
$x=0$
, 4, 8, 12, 16, 20 mol%) with different combinations of
$\text{P}_{2}\text{O}_{5}$
and
$\text{SiO}_{2}$
were designed to achieve high thermal shock resistance and modest laser properties, and designated as P-Si0, P-Si4, P-Si8, P-Si12, P-Si16 and P-Si20. Table 1 shows their thermal shock properties, and as a reference, the corresponding properties of N31 glass are also given. It is known that through composition optimization, thermal conductivity can be improved by 2 times and the thermal expansion coefficient decreased to
$1/2$
, and then the thermal shock resistance was improved 4 times compared with that of N31 glass.
In phosphate laser glass, the thermal–optical properties were also studied by adjusting the content of alkali oxides and alkaline oxides. Table 2 shows the emission cross section and thermal–optical properties of
$\text{P}_{2}\text{O}_{5}\text{-}\text{Al}_{2}\text{O}_{3}$
-BaO-
$\text{Li}_{2}\text{O}$
phosphate glass. In (60-70)
$\text{P}_{2}\text{O}_{5}$
-(7-10)
$\text{Al}_{2}\text{O}_{3}$
-(22-x)BaO-
$x\text{Li}_{2}\text{O}$
(
$x=0$
, 6, 11,16) glass system, the thermal–optical coefficient can be reduced from
$3.8\times 10^{-6}$
to
$2.7\times 10^{-6}~\text{K}^{-1}$
with little effect on thermal expansion coefficient, just by the substitution of
$\text{Ba}^{2+}$
for
$\text{Li}^{+}$
, which were marked as Ba-Li0, Ba-Li6, Ba-Li11 and Ba-Li16.
(60-65)
$\text{P}_{2}\text{O}_{5}\text{-}(3\text{-}7)\text{Al}_{2}\text{O}_{3}\text{-}(10\text{-}18)\text{BaO}\text{-}x\text{K}_{2}\text{O}\text{-}(15\text{-}x)\text{Li}_{2}\text{O}$
series glasses (
$x=0$
, 3, 6, 9, 12, 15), which were labeled as K-Li0, K-Li3, K-Li6, K-Li9, K-Li12 and K-Li15, the replacement of
$\text{Li}^{+}$
by
$\text{K}^{+}$
was also studied. It can be found that the thermo-optical coefficient can be obviously reduced to zero and even negative (can obtain the athermal laser glass further), which benefits to compensate the laser beam distortion caused by thermal lens effect[Reference Jackel, Kaufman and Lallouz18]. In addition, it can be seen that the stimulated emission cross section can be improved from
$4.1\times 10^{-20}$
to
$4.5\times 10^{-20}~\text{cm}^{2}$
.
Based on the previous studies, two kinds of high-average-power Nd:phosphate glasses, NAP-2 and NAP-4, are developed in SIOM. Glass composition design, pot-melting technology, surface etching processing and thermal shock resistance of NAP-2 glass have been systematically investigated[Reference Chen, He, Hu, Li and Cheng16, Reference Li, He, Li, Chen and Hu17]. The main properties of NAP-2 and NAP-4 from SIOM with those of HAP-4 (Hoya), APG-1 and APG-2 (Schott) glasses are given in Table 3. Comparing the data in Tables 1 and 2, it is found that the thermal conductivity and the thermal expansion coefficient of NAP-2 are equivalent with those of APG-1, while the temperature coefficient of the optical path length of NAP-2 is smaller than those of HAP-4 and APG-1, which is helpful to decrease the thermal lens effect of laser beam for the applications in repetition-rate laser.
Table 3. Main parameters of high-average-power neodymium phosphate laser glasses from Hoya[Reference Campbell and Suratwala3], Schott[Reference Campbell and Suratwala3] and SIOM.
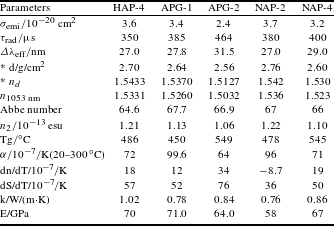
* Parameters which may vary with the
$\text{Nd}_{2}\text{O}_{3}$
concentration in glass.

Figure 1. The absorption (left) and emission (right) spectrum of phosphate, silicate and aluminate glass.
Table 4. Range of optical parameters of
$\text{Nd}^{3+}$
in different host glasses[Reference Weber21].

4 Silicate and aluminate neodymium laser glasses with ultra-broad emission bandwidth
The Texas Petawatt Laser has proved that it is possible to combine two laser glasses with different gain spectra together to create laser power exceeding the world’s largest Ti:Sapphire systems at a fraction of the cost[Reference Gaul, Martinez, Blakeney, Jochmann, Ringuette, Hammond, Borger, Escamilla, Douglas, Henderson, Dyer, Erlandson, Cross, Caird, Ebbers and Ditmire12]. While this technology is a proved route to high-power, compressed-pulse PW lasers, but the emission bandwidth of laser glasses had limited the further scaling to EW level[Reference Hays, Gaul, Martinez and Ditmire19].
Laser glasses used in PW systems must meet two conflicting requirements: broad emission bandwidth and low saturation fluence. PW lasers require gain media with broad spectral bandwidth (
$\unicode[STIX]{x1D6E5}\unicode[STIX]{x1D710}$
) to accommodate the short pulse width. This limitation is generally expressed by the product of pulse-length (
$t_{p}$
) times bandwidth (
$\unicode[STIX]{x1D6E5}\unicode[STIX]{x1D710}$
), which for a chirped Gaussian-shape pulse is[Reference Campbell, Hayden and Marker1]:

Therefore, laser glasses used to amplify very short temporal pulse must have broad gain (or emission) bandwidths. For CPA systems, the merit function of laser glass selection should shift from emission cross section and low nonlinear coefficient parameters to a broader gain spectrum[Reference Gaul, Hays, Martinez and Ditmire20]. Table 4 summarizes the spectroscopic properties in different host glasses[Reference Weber21]. From a knowledge of emission bandwidth, neodymium-doped silicate, germanate and aluminate glass are suitable to meet these needs. In the investigations of Texas Petawatt Laser, two kinds of laser glasses have been identified specially from nearly 250 glass compositions due to their significantly broader spectrum. These two glasses are designated as K-824 Nd:Ta-Silicate and L-65 Nd:Aluminate glass. However, neither of them was commercially available because of the serious crystallization problem during fabrication process[Reference Hays, Gaul, Martinez and Ditmire19, Reference Baesso, Bento, Miranda, de Souza, Sampaio and Nunes22].
In CaO-BaO-
$\text{Al}_{2}\text{O}_{3}$
glass, the effects of
$\text{SiO}_{2}$
and
$\text{Ga}_{2}\text{O}_{3}$
on the spectra properties have been studied[Reference Kang, He, Gao, Huang, Ding and Hu23]. The spectroscopic properties of ACS (
$\text{Al}_{2}\text{O}_{3}$
-CaO-BaO-
$\text{SiO}_{2}$
) and ACG (
$\text{Al}_{2}\text{O}_{3}$
-CaO-BaO-
$\text{Ga}_{2}\text{O}_{3}$
) glasses have been given in Table 5. The ACS series glasses have relatively broader bandwidth and long fluorescent lifetime, and the ACG glass has better glass-forming and fabrication properties.
Table 5. Bandwidth and Emission cross section of
$\text{Nd}^{3+}$
-doped aluminate glasses.

In SIOM, NSG-2 silicate glass and ACS-1 aluminate glass with broadband emission spectra have been developed. The main parameters of these two laser glasses were given in Table 6 and compared with those of LG-680 from Schott, K-824 Nd:Ta-silicate, L-65 Nd:aluminate glass from LLNL reports[Reference Hays, Gaul, Martinez and Ditmire19]. Fluorescence peak wavelengths of these glass locate at wavelength larger than 1060 nm, which is red shifted from the Nd:phosphate glass peaked at 1053 nm, and this feature will benefit the broadening of gain bandwidth to support the shorter pluses of hybrid glass laser system. Figure 1 shows the absorption and emission spectra of
$\text{Nd}^{3+}$
-doped phosphate, silicate and aluminate glasses. It indicates that emission peak wavelengths of
$\text{Nd}^{3+}$
ions at three different glasses change within 8–12 nm range, which provides the laser glass a solution to the mixed glass technique for EW laser system.
Table 6. Main parameters of laser glasses from Schott, LLNL and SIOM.

Table 7. Emission cross section and nonlinear refractive index in commercial silica/silicate and FP glasses.

Table 8. Properties of
$\text{Nd}^{3+}$
-doped fluorophosphate glasses from Schott, Hoya and SIOM.
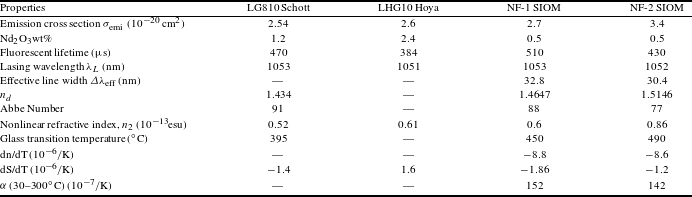
5 Fluorophosphate glass with low nonlinear refractive index
Concerning of the much higher laser energy demanded in ICF laser facility, the risk of nonlinear optical damage will increase. B integral[Reference Wen and Fan13], which is closely related to nonlinear refractive index, should be controlled to be as lower as possible. Intensity ripples (noise) that occur at certain spatial frequencies grow exponentially with B. B integral is calculated according to the equation[Reference Shao, Fu, Lin and Qiao24]:

where
$I(0,t)$
is the intensity of the incident light,
$z$
is the propagation distance of laser,
$l$
is length of the gain medium, and
$\unicode[STIX]{x1D706}$
is the incident light wavelength),
$n_{2}$
is the nonlinear refractive index. The gain medium with low
$n_{2}$
is needed in order to decrease the B integral. Compared with other laser glass systems, fluorophosphate glass is a special one that combines the advantages of fluorides and phosphate glasses: higher thermal and chemical stability than fluoride glass; higher
$\text{OH}^{-}$
resistance ability than phosphate glass; athermal behavior, low refractive index; low dispersion, and most importantly, low nonlinear refractive indices; long fluorescence lifetime and tailorable properties by varying the F/P ratio[Reference Gan25, Reference Zhang, Hu and Jiang26]. Table 7 gives the
$n_{2}$
and
$\unicode[STIX]{x1D70E}_{\text{emi}}$
data of
$\text{Nd}^{3+}$
-doped silica/silicate and FP glasses, in which LG810 (from Schott) and LHG10 (from Hoya) are
$\text{Nd}^{3+}$
-doped fluorophosphate glasses with obviously lower
$n_{2}$
compared with other
$\text{Nd}^{3+}$
- doped glasses.
Table 9. Simulation results with total 18 pieces of NF-1 and N31 glass slabs, pulse width 5 ns.

Two kinds of
$\text{Nd}^{3+}$
:fluorophosphate glasses with small
$n_{2}$
have been developed in SIOM. NF-1 focuses on low
$n_{2}$
and NF-2 aims to achieve higher emission cross section and better thermal–mechanical properties. Both glasses are with low phosphate content and fluoride alkalis free to maintain lower
$n_{2}$
but higher glass transition temperature. The base glass composition is (5-13 mol%) phosphates-(15-27)
$\text{AlF}_{3}$
-(7-11)
$\text{MgF}_{2}$
-(15-24)
$\text{CaF}_{2}$
-(8-16)
$\text{SrF}_{2}$
-(8-12)
$\text{BaF}_{2}$
-(0-5)
$\text{YF}_{3}$
. Both NF glasses perform good glass-forming ability and higher thermal stability as well as large
$\unicode[STIX]{x1D6E5}\unicode[STIX]{x1D706}_{\text{eff}}$
value because of the inhomogeneous broadening feature of the mixed glass formers in this kind of glass. Table 8 shows properties of
$\text{Nd}^{3+}$
: fluorophosphate glasses from Schott (LG810) Hoya (LHG10) and SIOM (NF-1 and NF-2). Lifetime of NF-1 can keep
$335~\unicode[STIX]{x03BC}\text{s}$
when
$\text{Nd}_{2}\text{O}_{3}$
wt% is 2.8%.
A simulation base on the conditions of
$\unicode[STIX]{x1D6E5}\text{B}~(\text{rad})\leqslant 1.8$
(
$\unicode[STIX]{x1D6E5}\text{B}$
is the B integral accumulated by the beam passing between two pinholes)[Reference Yang, Zhang, Hu, Wang and Su27] and
$\unicode[STIX]{x1D6F4}\text{B}~(\text{rad})\leqslant 3.5$
(
$\unicode[STIX]{x1D6F4}\text{B}$
is the B integral for the entire chain from beam injection to the input of frequency converter)[Reference Wang, Su and Wang28] has been carried out to evaluate the contribution of
$\text{Nd}^{3+}$
-doped fluorophosphate glass to the maximum output energy of a laser system with different combinations of N31 and NF-1 glasses. The restrictive conditions that
$\unicode[STIX]{x1D6E5}\text{B}~(\text{rad})\leqslant 1.8$
and
$\unicode[STIX]{x1D6F4}\text{B}~(\text{rad})\leqslant 3.5$
were set to maintain a better beam quality. The stimulated emission cross section of N31 glass is
$3.8\times 10^{-20}~\text{cm}^{2}$
, and
$n_{2}$
of N31 glass is
$1.18\times 10^{-13}~\text{esu}^{5}$
. Table 9 shows the simulation results. The replacement of NF-1 glass to N31 glass showed that the output energy improves 7%–27% with the contribution of small
$n_{2}$
of NF-1 glass.
6 Conclusion
Three kinds of neodymium laser glasses have been developed in SIOM, China. In phosphate laser glass, the thermal shock resistance, emission cross section and thermal–optical properties were studied and NAP-2 and NAP-4 glasses with improved thermal–mechanical properties and suitable laser properties were developed for high-average-power laser system. The
$\text{Nd}^{3+}$
-doped silicate (NSG-2) and aluminate (ACS-1) glasses with broad effective emission bandwidth of 34 and 50 nm, respectively, having potential applications in the EW-class laser facility, have been developed. The properties of two kinds of
$\text{Nd}^{3+}$
-doped fluorophosphate glasses (NF-1 and NF-2) with low nonlinear refractive index (
$n_{2}=0.6{-}0.86$
) and long fluorescence lifetime (
$430{-}510~\unicode[STIX]{x03BC}\text{s}$
) were presented, and simulation work showed that the replacement of NF-1 glass to N31 glass in high-power laser device results in 7%–27% improvement of output energy.