Introduction
The glial fibrillary acidic protein (GFAP) is an intermediate filament widely known as a molecular marker for astroglia (Middeldorp & Hol, Reference Middeldorp and Hol2011). GFAP expression is strikingly enhanced in conditions of stress, inflammation or injury (Anderson, Ao, & Sofroniew, Reference Anderson, Ao and Sofroniew2014; Li et al., Reference Li, Liu, Liu, Liu, Tong, Jia and Wang2019; Middeldorp & Hol, Reference Middeldorp and Hol2011), and mutations in this filament are associated to Alexander disease (Messing, Reference Messing2018). However, the structure and function of this filament remain mostly unknown. This is due, at least in part, to the intolerance of GFAP to tags at the N- or C-terminus. Most GFAP studies were restricted to fixed cells and immunocytochemistry (Hsiao et al., Reference Hsiao, Tian, Long, Der Perng, Brenner, Quinlan and Goldman2005; Perng et al., Reference Perng, Wen, Gibbon, Middeldorp, Sluijs, Hol and Quinlan2008), and early attempts to tag human GFAP (hGFAP) had mixed success, as cells often showed GFAP aggregation or poor filament formation (Bachetti et al., Reference Bachetti, Caroli, Bocca, Prigione, Balbi, Biancheri, Filocamo, Mariotti, Pareyson, Ravazzolo and Ceccherini2008; Perng et al., Reference Perng, Wen, Gibbon, Middeldorp, Sluijs, Hol and Quinlan2008; Tulyeu et al., Reference Tulyeu, Tamaura, Jimbo, Shimbo, Takano, Iai, Yamashita, Goto, Aida, Tokuhiro, Yamagata and Osaka2019). Very recently, mouse GFAP (mGFAP) was successfully tagged with EGFP by introducing a linker between the filament and the fluorescent protein (Mignot et al., Reference Mignot, Delarasse, Escaich, Della Gaspera, Noé, Colucci-Guyon and Pham-Dinh2007). Although mGFAP shares 95% homology to hGFAP (Anderson et al., Reference Anderson, Ao and Sofroniew2014; Middeldorp & Hol, Reference Middeldorp and Hol2011), there are relevant differences that could determine different mechanisms of regulation and function.
Objective
Here, we developed new tools for the study of GFAP behavior and function in living cells. First, we created an EGFP-tagged version of hGFAP where the tag is within the GFAP sequence, and not at its N- or C-terminus, in order to avoid the issues related to terminal GFAP tags (Bachetti et al., Reference Bachetti, Caroli, Bocca, Prigione, Balbi, Biancheri, Filocamo, Mariotti, Pareyson, Ravazzolo and Ceccherini2008; Perng et al., Reference Perng, Wen, Gibbon, Middeldorp, Sluijs, Hol and Quinlan2008; Tulyeu et al., Reference Tulyeu, Tamaura, Jimbo, Shimbo, Takano, Iai, Yamashita, Goto, Aida, Tokuhiro, Yamagata and Osaka2019). Secondly, we used a previously reported mGFAP construct tagged with EGFP at the C-terminus (Mignot et al., Reference Mignot, Delarasse, Escaich, Della Gaspera, Noé, Colucci-Guyon and Pham-Dinh2007) to insert a Halo Tag in substitution of EGFP. The Halo tag is far more versatile than EGFP (England, Luo, & Cai, Reference England, Luo and Cai2015) and will allow super-resolution, single-molecule and protein–protein interaction studies on GFAP.
Methods
A commercial transposase was used to insert a kanamycin resistance gene and EGFP into the human GFAP sequence in a random manner (dx.doi.org/10.17504/protocols.io.77fhrjn). The final selected construct had EGFP inserted after amino acid 183 of the GFAP sequence, but the transposase reaction produced an unavoidable repetition of amino acids 181–183 right after the EGFP sequence, as previously described (Sheridan et al., Reference Sheridan, Berlot, Robert, Inglis, Jakobsdottir, Howe and Hughes2002). EGFP was substituted by a Halo tag in the pEGFP-N3-mGFAP plasmid (dx.doi.org/10.17504/protocols.io.77fhrjn). Single R236H or R239C Alexander disease-related mutations were inserted by site-directed mutagenesis into our mGFAP or hGFAP constructs, respectively (dx.doi.org/10.17504/protocols.io.77fhrjn). Human U251 cells and rat C6 glioma cells were maintained, transiently transfected with the corresponding plasmids and treated as described in dx.doi.org/10.17504/protocols.io.77ehrje. Imaging and image analysis were carried out as described in dx.doi.org/10.17504/protocols.io.77ghrjw. Protein extraction and western blotting was carried out as described previously (Herrera et al., Reference Herrera, Chen, Fischer, Maher and Schubert2009). Statistical analysis and graphical representation of data were performed using Sigmaplot software (Systat Software, Inc., San Jose, CA, USA). Sample data are represented as mean ± standard deviation of at least 3 independent experiments. Statistical significance was evaluated by means of a one-way ANOVA followed by a Tukey’s test. Results were considered significant when p < 0.05.
Results
Both EGFP-hGFAP and mGFAP-Halo constructs formed normal filaments in U251 glioma cells, but the mGFAP-Halo construct failed to do so in rat C6 glioma cells (Fig. 1A). They were suitable for obtaining super-resolution-like pictures by means of super-resolution radial fluctuations (SRRF) (Fig. 1B). None of them formed normal fibers in HEK293 cells (Fig. 1D). The site of insertion of EGFP in hGFAP was not suitable for hosting a Halo tag or bimolecular fluorescence complementation tags (Fig. 1C). While the Alexander disease-related mutation R239C induced aggregation of hGFAP in 48% of cells, the equivalent R236H mutant mGFAP showed disorganization of fibers with signs of aggregation in only 14% of cells (Fig. 2A-C). The expression levels of mutant GFAP were similar to their wild type counterparts (Fig. 2E). However, we cannot rule out the possibility that mGFAP-Halo constructs are expressed at lower overall levels than hGFAP-EGFP constructs, which could explain a lower level of aggregation in mutant mGFAP-Halo. The anti-amyloidogenic curcumin derivative CNB-001 (10 μM)(Liu, Dargusch, Maher, & Schubert, Reference Liu, Dargusch, Maher and Schubert2008) partially prevented hGFAP aggregation (Fig. 2D). Single-molecule analysis of mGFAP-Halo dynamics confirmed that the R236H mutation produced a higher proportion of free-moving GFAP molecules, and indicated that pharmacological interference with microtubules or actin filaments significantly disrupted mGFAP dynamics (Fig. 3).
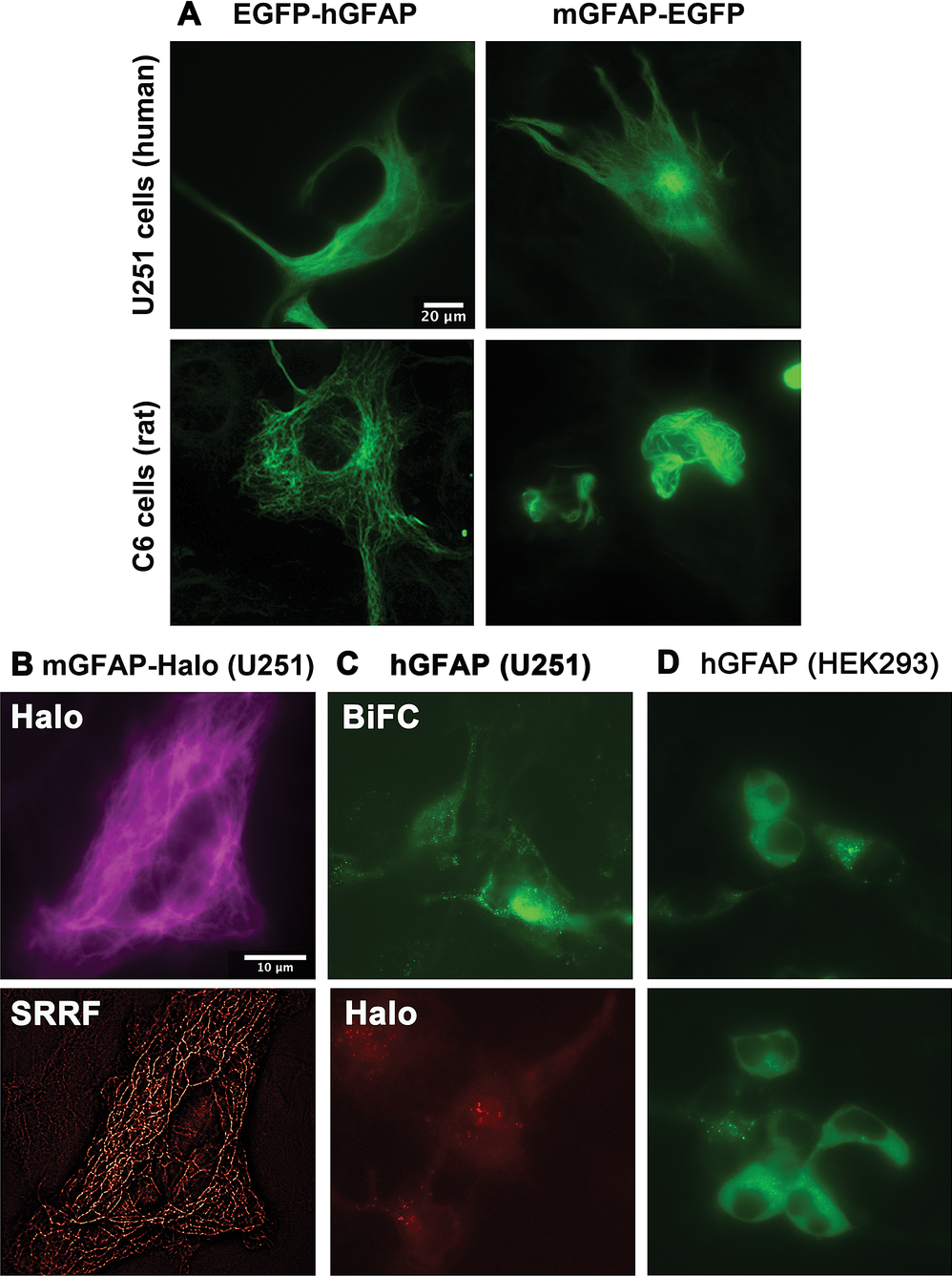
Figure 1. Tagged versions of human and mouse GFAP form normal fibers in glioblastoma cells. Human U251 or rat C6 glioblastoma cells were transiently transfected with different human or mouse GFAP constructs, and imaged 24 h later. A, When transfected into U251 human glioblastoma cells EGFP-hGFAP and mGFAP-EGFP exhibited a normal GFAP filamentous network. However, when the same constructs were transfected into C6 rat glioma cells, only EGFP-hGFAP formed a regular filamentous network. B, mGFAP-Halo constructs (incubated with the JF549 Halo ligand, 100 nM) also produced normal filaments only in U251 cells. Widefield images of the mGFAP-Halo construct were further analyzed using the ImageJ software with the NanoJ SRRF plug-in to obtain a more defined image of the intermediate filament network. C, Our attempts to substitute EGFP for bimolecular fluorescence complementation (BiFC) tags Venus 1 (amino acids 1–157) and Venus 2 (amino acids 158–238) or Halo Tag were unsuccessful. These are representative images of U251 cells transfected with these constructs, where residual fluorescence can be observed but has no recognizable pattern (i.e. filaments, bundles or aggregates). D, Transfection of HEK293 cells with the EGFP-hGFAP construct produced either homogenous fluorescence or aggregates, but no filamentous network.

Figure 2. Alexander disease-related mutations cause filament disorganization of GFAP. A, U251 cells were transiently transfected with either wild type (WT) or Alexander disease (AxD)-related versions of EGFP-hGFAP or mGFAP-Halo (R239C or R236H, respectively), and pictures were taken 24 h later. In the case of the mGFAP-Halo constructs, cells were incubated with the JF647 Halo ligand (100 nM) prior to imaging. WT hGFAP or mGFAP assembled into filaments (left panels), but AxD mutations changed this pattern (right panels). hGFAP R239C produced protein aggregates in the cytoplasm, while mGFAP R236H formed a homogeneous pattern throughout the cytoplasm without apparent filament structures. B-C, Quantification of the various patterns observed in U251 cells transfected with (B) WT EGFP-hGFAP (black bars) and mutant EGFP-hGFAP (white bars) or (C) the equivalent mGFAP-Halo constructs. **, significant vs WT, p < 0.01; ***, p < 0.001. D, Quantification of U251 cells transfected with the EGFP-hGFAP R239C mutant that displayed a normal filamentous network (black bars) or aggregates (white bars) after treatment with increasing concentrations (0.1–10 μM) of the neuroprotective compound CNB-001. All groups were statistically significant versus WT EGFP-hGFAP, p < 0.001. #, significant vs EGFP-hGFAP R239C, p < 0.05; ###, p < 0.001. E, Western blots showing similar levels of expression in U251 cells transfected with hGFAP-EGFP and mGFAP-Halo constructs.

Figure 3. Genetic or pharmacological interference with the diffusion properties of mGFAP molecules. Average diffusion coefficient of rapid-diffusion molecules (A) and fraction of slow-diffusion GFAP molecules (B) calculated for individual cells (20 cells/group) by means of the Spot-On online tool (https://spoton.berkeley.edu/SPTGUI/docs/latest). The AxD-related mutation R236H or incubation of cells with Leukemia Inhibitory Factor (LIF, 100 ng/ml), Nocodazole (10 μM) or Latrunculin B (10 μM) for 2 hours changed the diffusion properties of single mGFAP molecules. LIF is a cytokine that induces the expression and polymerization of GFAP; Nocodazole is a drug that interferes with the formation of microtubules; and Latrunculin B is a drug that disrupts actin filaments. *, significant versus WT, p < 0.05; *** p < 0.001.
Discussion
Our tagged hGFAP and mGFAP constructs behaved mostly as expected for endogenous GFAP when they were expressed in living glioma cells and challenged with previously known genetic and pharmacological modifiers of GFAP fibrillization. The only anomalies detected are the lack of GFAP aggregates in the R236H mGFAP-Halo mutant and its inability to form fibers in rat glioma cells. These are facts that we cannot currently explain and in which the performance of the EGFP-hGFAP version was superior. Interestingly, the only functional site of EGFP insertion in hGFAP is located in a region (amino acids 183–184) that shows very little frequency of mutations related to Alexander disease (Messing, Reference Messing2018).
Conclusions
We reported a successful attempt to EGFP-tag hGFAP and a new version of mGFAP tagged with Halo. The EGFP-hGFAP construct is adaptable to different species and behaves as expected when it is mutated, but it is not suitable for single molecule analysis (although it can be used for super-resolution imaging in combination with the SRRF plug-in (Gustafsson et al., Reference Gustafsson, Culley, Ashdown, Owen, Pereira and Henriques2016). On the other hand, the mGFAP-Halo construct is more sensitive to the biological background and does not aggregate when mGFAP is mutated, but it allows a wider spectrum of applications, including super-resolution, single-molecule and protein–protein interaction analyses in living cells. We hope these new tools help the GFAP and astrocyte community to advance our understanding of physiological and pathological functions of this intermediate filament in living cells.
Acknowledgements
The authors thank the Advanced Imaging Unit from Gulbenkian Science Institute for support with bioimaging and flow cytometry. The pEGFP-N3-mGFAP plasmid was a kind gift from Dr. Cécile Leduc (Institut Pasteur, Paris, France), the pcDNA-hGFAP construct was a generous gift by Dr. Michael Brenner (University of Alabama, Birmingham, USA), and the template transposon construct with kanamycin resistance and EGFP was kindly provided by Dr. Mika Ruonala. The anti-amyloidogenic compounds J147 and CNB001 were kindly provided by Dr. David Schubert (The Salk Institute, La Jolla, CA, USA). The photoactivatable Janelia Fluor 549 nm (PA-JF549) HaloTag ligand was a kind gift from Dr.Luke Lavis (HHMI’s Janelia research campus, Ashburn, VA, USA).
Author contributions
RLV and RQ had equal contribution to this work. RLV developed the EGFP-hGFAP construct and carried out the experiments related to this construct (formation of aggregates and prevention by anti-amyloidogenic drugs). RQ developed the mGFAP-Halo construct and carried out the experiments related to this construct (single-molecule and SRRF analyses). Both of them worked in close collaboration at all cloning and mutagenesis steps. FM tested the formation of GFAP fibrils in different cellular contexts. Alvaro Crevenna and Zach Hensel supervised imaging experiments by RQ and the subsequent data analysis. FH supervised experiments by RLV and RQ, helped with statistical analysis and coordinated the team. FH and RQ wrote the main draft, with contributions from ZH and RLV.
Funding information
FH was supported by national funds through Fundação para a Ciência e Tecnologia (FCT, Ref. IF/00094/2013/CP1173/CT0005 and PTDC/MED-NEU/31417/2017). FH, ZH and AC were supported by Project LISBOA-01-0145-FEDER-007660 (Cellular Structural and Molecular Microbiology) funded by FEDER funds through COMPETE2020 - Programa Operacional Competitividade e Internacionalização (POCI). RLV and FM were supported by fellowships from FCT (Refs. PD/BD/128163/2016 and SFRH/BD/133220/2017, respectively).
Data availability statement
The data that support the findings of this study will be made available in a public repository upon acceptance of the manuscript.
Conflicts of interest
The authors declare that they have no conflicts of interests.
Abbreviations:
- hGFAP
human glial fibrillary acidic protein;
- mGFAP
mouse glial fibrillary acidic protein;
- EGFP
enhanced green fluorescent protein;
- AxD
Alexander disease;
- LIF
Leukemia Inhibitory Factor;
- SRRF
Super-Resolution Radial Fluctuations.
Comments
Comments to the Author: Previous tagged versions of GFAP have mixed reputations, with some arguing that the tags interfere with normal function. These new versions may offer better options. But exactly where in the hGFAP sequence does the tag reside? Could the difference in aggregation between the two constructs derive from different levels of expression?