Introduction
An understanding of agricultural systems is key for investigating both the food systems underpinning past urban societies and the impact of imperialism on rural agricultural communities. Urbanization based on domesticated cereals has been linked to periods of agricultural extensification in several periods and regions across Eurasia and has been associated with the growing importance of traction animals and the rise of social inequality (Bogaard et al., Reference Bogaard, Fochesato and Bowles2019). Many case studies are of early state societies, with regionally defined hinterlands, such as Bronze Age Mesopotamia (Styring et al., Reference Styring, Charles, Fantone, Hald, McMahon and Meadow2017a) and Hittite Hattusha (Diffey et al., Reference Diffey, Neef, Seeher and Bogaard2020).
In contrast, the Roman world is a prime example of an empire spanning wide climatic and environmental regions, where staple foods (olives, wine, meat, and cereals) were moved over long distances. The supply of cereals to urban populations through the annona system, and to the military through tax-in-kind and various other strategies (Erdkamp, Reference Erdkamp2005: 209–44) was vital for maintaining social and political stability. Villa landscapes, regions with villas and farmsteads orientated towards cereal production, are found across the north-western provinces, including distinctive regions of open chalk hills or downland (Smith et al., Reference Smith, Allen, Brindle and Fulford2016; Reddé, Reference Reddé2018). Here, shifts to market-orientated cereal production have been recognized in the construction of large-scale above-ground granaries, processing structures such as grain-drying ovens and powered mills, and their specialized preparation as bread and beer (Lodwick, Reference Lodwick, Allen, Lodwick, Brindle, Fulford and Smith2017). The crop husbandry practices by which cereals were cultivated in these landscapes have begun to be addressed (Lodwick, Reference Lodwick, Allen, Lodwick, Brindle, Fulford and Smith2017; Lepetz & Zech-Matterne, Reference Lepetz, Zech-Matterne and Reddé2018), but such progress as has been made has not been subject to multi-proxy analysis, or coupled with an exploration of their socio-economic implications for rural communities and the understanding of provincial society in Roman Britain (Taylor, Reference Taylor2013a).
Crop husbandry practices encapsulate the relationships between land, labour, and resources, namely animal manure, traction, and water. The labour intensity of farming practices has major implications for both the organization and seasonality of agricultural labour, and the sustainability of agro-ecological systems within a given environment. Current understandings of Roman crop husbandry systems range from intensive farming—increasing the productivity of soils through labour-intensive techniques such as manuring (Heinrich et al., Reference Heinrich, Hansen and Erdkamp2021)—to more extensive systems integrated with animal husbandry through rotation or ley farming (Kron, Reference Kron2000; Marzano, Reference Marzano, Hollander and Howe2020). The Roman agronomists, such as Cato and Columella, were aware of a range of fertilizers and crop rotations including legumes, grasses, and barley (Kron, Reference Kron2000). These sources do not, however, provide a sound basis for reconstructing crop husbandry systems through the provinces of the Roman Empire given their specific cultural and regional context in the central Mediterranean. How crop husbandry practices changed, and what the socio-economic and environmental consequences of these changes were, are vital questions for understanding the impact of the Roman empire on rural communities and how market-orientated production of cereals was pursued as the empire expanded into new environmental regions. An area of downland landscape, the Hampshire Downs in southern England, provides the necessary high-resolution archaeobotanical and archaeological evidence to undertake such an exploration due to numerous excavated rural settlements.
This article presents the results of an archaeobotanical, isotopic, and functional weed ecological analysis of plant remains from a series of sites spanning the Middle Iron Age (from c. 400 bc) to late Roman (to c. ad 410) period in the Hampshire Downs. Its aim is to investigate agricultural change in a well-understood villa landscape. By establishing the crop husbandry system underpinning villa systems, I explore the socio-economic organization of a villa landscape, arguably the most marked impact of imperialism on rural society, and the environmental and socio-economic impacts of Roman market-orientated cereal production.
Cereal Cultivation Strategies in the North-Western Roman Provinces
Whilst small-scale arboriculture, horticulture, and viticulture were practised in Gaul and Britain during the Roman period (Lodwick, Reference Lodwick, Allen, Lodwick, Brindle, Fulford and Smith2017; Lepetz & Zech-Matterne, Reference Lepetz, Zech-Matterne and Reddé2018), the bulk of plant-based agriculture in the north-western provinces was based on cereals. Thanks to research projects collating evidence from development-led archaeology (Smith et al., Reference Smith, Allen, Brindle and Fulford2016; Reddé, Reference Reddé2018), a rich dataset of charred plant remains has been used to show key trends in crop choice within temperate Europe north of the Alps. In villa landscapes, the crop spectra have been identified as spelt wheat, barley, and emmer in the southern part of Germania Inferior (Brüggler et al., Reference Brüggler, Jeneson, Gerlach, Meurers-Balke, Zeri, Herchenbach and Reddé2017), free-threshing wheat in the Paris basin (Lepetz & Zech-Matterne, Reference Lepetz, Zech-Matterne and Reddé2018: 343), and spelt wheat and hulled barley in central and southern Britain (Lodwick, Reference Lodwick, Allen, Lodwick, Brindle, Fulford and Smith2017). In contrast, in the non-villa landscape of the Dutch river area, there was a continuation of Iron Age crops, i.e. barley, emmer, oat, and millet (Groot et al., Reference Groot, Heeren, Kooistra and Vos2009). The Roman period saw a decrease in cereal diversity across the north-western provinces, with a shift towards free-threshing or spelt wheat (Lodwick, Reference Lodwick, Allen, Lodwick, Brindle, Fulford and Smith2017; Lepetz & Zech-Matterne, Reference Lepetz, Zech-Matterne and Reddé2018).
It is the methods of cultivation of crops which are key controllers of land, labour, and resources, the three main aspects of the agricultural economy (Erdkamp, Reference Erdkamp2005: 12–54). The most detailed work in north-eastern Gaul has suggested the rotation of cereals and pulses, targeted manuring of free-threshing wheat at some sites, and an overall balance of intensive and extensive practices attuned to the local environment (Aguilera et al., Reference Aguilera, Zech-Matterne, Lepetz and Balasse2018; Lepetz & Zech-Matterne, Reference Lepetz, Zech-Matterne and Reddé2018). In Britain, archaeobotanical studies have postulated the continuation of crop husbandry innovations—monocropping and spring sowing—from the Late Iron Age onwards (Campbell, Reference Campbell and Bird2016; Van der Veen, Reference Van der Veen, Millett, Revell and Moore2016). Some have argued for a Roman infield-outfield system of intensive manuring of core cereals fields and occasional outer cultivation (Fowler, Reference Fowler2002: 216–17; Gerrard, Reference Gerrard2013: 102). Ceramic scatters have been used to propose an intensification of arable cultivation through manuring (Fulford & Holbrook, Reference Fulford and Holbrook2011). In contrast to these varying perspectives, a picture of extensive farming, with fields tilled by traction animals and low inputs of human labour, has been hypothesized on the basis of a large-scale synthesis of faunal and archaeobotanical data (Allen et al., Reference Allen, Lodwick, Brindle, Fulford and Smith2017). The archaeobotanical studies cited above have used a range of autecological techniques (studying individual weed species) and coarse data analysis, which have well-established limitations. A more accurate, comparable technique of identifying crop husbandry practices requires functional weed ecology analysis in combination with stable isotope analysis (Bogaard et al., Reference Bogaard, Hodgson, Nitsch, Jones, Styring and Diffey2016).
The study area
To undertake such analysis, a region that benefits from a high-quality dataset is the Hampshire Downs, a villa landscape of chalk downland or open chalk hills in central-southern Britain (Figure 1). This region has been central to understandings of Middle Iron Age society in southern Britain (c. sixth century bc to c. 50 bc), with hillforts, especially Danebury, interpreted as either hierarchical central places or heterarchical communities (Davis, Reference Davis2013). In the Late Iron Age (c. 50 bc to ad 43, oppida developed to the south-east at Silchester and Winchester (Sharples, Reference Sharples2010). Communities on the Downs continued to reside in small farmsteads, before and after the Claudian conquest of ad 43, despite the Roman road network passing nearby in the early Roman period (c. ad 43 to second century ad) (Allen, Reference Allen, Smith, Allen, Brindle and Fulford2016: 130). No military sites are known in this region, and the closest towns were the civitas capitals located at Venta Belgarum (Winchester) and Calleva Atrebatum (Silchester).

Figure 1. Location maps. A: Roman Empire, B: Roman Britain, C: Hampshire Downs with sites studied.
Third- and fourth-century ad (mid- to late Roman) villas typically contained residential suites and agricultural processing buildings. Large-scale cereal production, a major aspect of the regional economy, is well evidenced by grain-drying ovens and mills (Allen, Reference Allen, Smith, Allen, Brindle and Fulford2016: 122–23). The diversity of wealth displayed in these villas through different levels of architectural investment and food consumption is thought to have been built on the production of agricultural surplus (Cunliffe, Reference Cunliffe2008: 48).
Methods
Excavations at Danebury hillfort from 1968 to 1989 included extensive sampling for charred plant remains. Samples from earlier seasons were fully analysed by Martin Jones (Reference Jones and Cunliffe1984). Excavations of the other sites analysed here were undertaken as part of the Danebury Environs Programme, again with extensive sampling and reporting. Charred plant remains were analysed by Gill Campbell (Reference Campbell and Cunliffe2000, Reference Campbell and Cunliffe2008). Published datasets were digitized and analysed using R software (R Core Team, 2020). Archaeobotanical data was analysed in R using crop ratios and discriminant analysis, followed by correspondence analysis to explore patterns within the dataset. For isotopic analysis, ten grains per archaeobotanical sample were homogenised in order to account for variation within a cereal ear and field. Samples were selected from five sites (Danebury, Nettlebank Copse, Suddern Farm, Dunkirt Barn, Grateley) where sufficient material was available. The methodology for the functional ecological study of weed flora followed Bogaard et al. (Reference Bogaard, Hodgson, Nitsch, Jones, Styring and Diffey2016); that study differentiated, on the basis of the functional traits of the weeds recorded within the fields, two types of traditional farming systems (high-input fields in the Asturias in Spain and low-input fields in Haute Provence in France). This produced a model with which archaeobotanical samples can be compared, as undertaken here. A more detailed methods statement is provided in the Supplementary Material. Data and script to reproduce analysis is available in a Zenodo repository (https://doi.org/10.5281/zenodo.6923308).
Results
Archaeobotanical evidence for crop husbandry change
The archaeobotanical assemblage from the Danebury environs region shows a picture of continuity in crop spectra, with shifts in the abundance of different crops. A decline in emmer wheat though time was observed, with a focus on six-row hulled barley and spelt wheat, and a minor presence of rye, pea, and free-threshing wheat grains (Campbell, Reference Campbell and Cunliffe2008). The dominance of spelt wheat and barley are summarized per site in Supplementary Table S2. This mirrors Roman crop choice across villa landscapes of southern, central, and eastern Britain (Lodwick, Reference Lodwick, Allen, Lodwick, Brindle, Fulford and Smith2017).
However, variation in sample taphonomy and composition led to difficulties in identifying husbandry practices in the original study (Campbell, Reference Campbell and Cunliffe2008: 53, 68, Reference Campbell and Bird2016: 136). Samples from Danebury hillfort are dominated by grain-rich deposits from hermetic crop storage siloes. Late Iron Age settlement samples represent crop-processing by-products, whilst mid- and late Roman villa samples represent high density grain samples from processing waste in crop dyers (Campbell, Reference Campbell and Cunliffe2008: 70). Underpinning these changes is the shift from the centralized storage of crops at hillforts, to crops potentially being mobilized across the landscape in the Late Iron Age (Van der Veen & Jones, Reference Van der Veen and Jones2006), before large-scale crop processing in the Roman period provided surplus cereals for urban and military populations (Campbell, Reference Campbell and Bird2016; Van der Veen, Reference Van der Veen, Millett, Revell and Moore2016). Whilst these depositional differences provide insights into the post-harvest treatment of cereals, they hinder the ability to look at long-term trends in crop choice and crop husbandry practices.
Based upon the percentage of barley and wheat grains in grain-rich samples dating to the Late Iron Age, Campbell (Reference Campbell and Cunliffe2000: 51) identified a change from the cultivation of spelt and barley as a maslin (mixed crop) to monocultures at this time. Monocultures persisted into the Roman period, before a return to maslins in the fourth century ad (Campbell, Reference Campbell and Cunliffe2008: 68). However, when the proportion of wheat and barley grains in all samples through time is considered, no change through time is seen (Figure 2). Furthermore, disentangling the cultivation of barley and glume wheats as maslins is difficult as these crops may be separated at different stages of crop processing (Jones & Halstead, Reference Jones and Halstead1995).
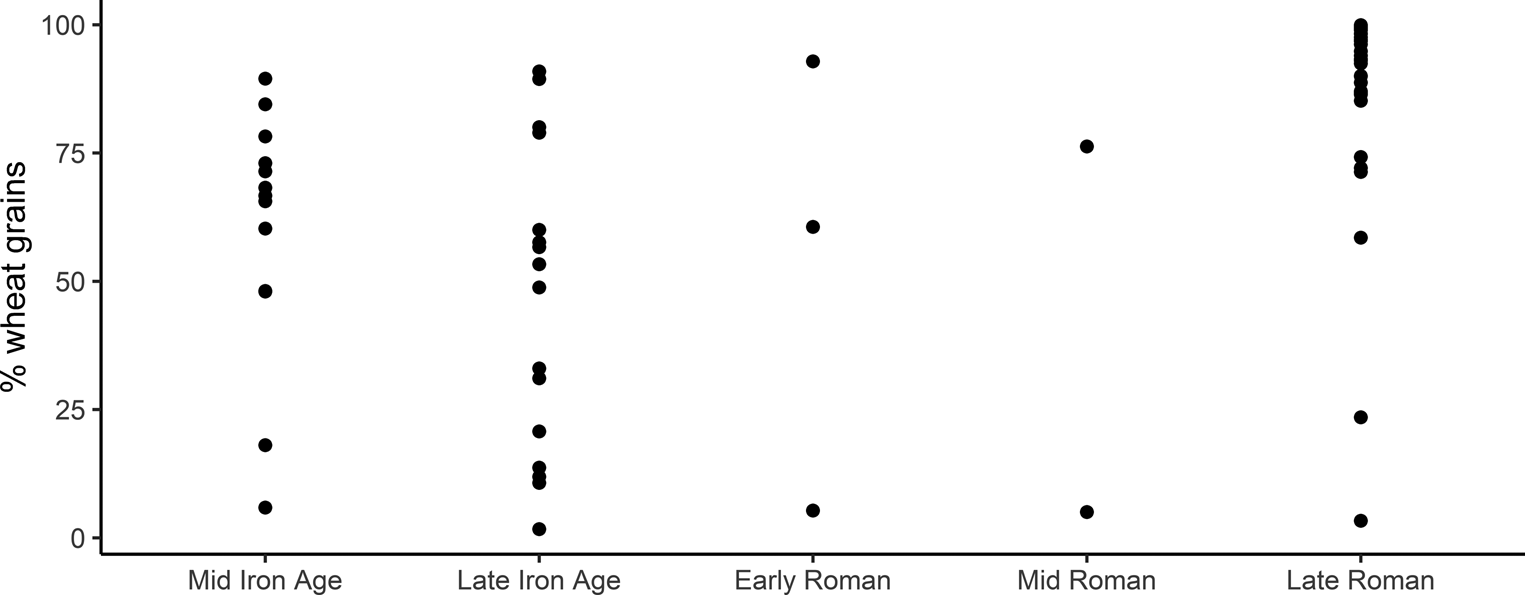
Figure 2. Percentage of wheat grains within the total of identified barley and wheat grains (only samples containing over fifty identified cereal grains). For sample phasing, see Supplementary Table S1, and for summed counts per site phase Supplementary Table S2.
Campbell (Reference Campbell and Cunliffe2000: 50) postulates a shift from autumn to spring sowing, linked with the suggested Late Iron Age move to monocultures and based on the relative percentage of the weed seeds Avena sp. and Bromus sp. A more rigorous way to assess sowing time is through the proportion of short and early flowering weed taxa (indicating autumn sowing) vs long and late flowering weed taxa, indicating spring sowing (Bogaard et al., Reference Bogaard, Jones, Charles and Hodgson2001). A correspondence analysis plot of samples from comparative crop-processing stages (only fine-sieve products and pre-sieved fine-sieve by-products that resemble them in weed composition; Supplementary Table S3) shows that late Roman samples from Grateley may indicate the practice of spring sowing of wheat (Figure 3a); but, when all weed flora are considered beyond Bromus and Avena (Figure 3b), no coherent picture of change in sowing time in the Late Iron Age can be observed. The late Roman samples from Grateley account for much of the variation, due to their association with Avena sp. and Agrostemma githago—weeds that rose to prominence in the Roman period (Figure 4).
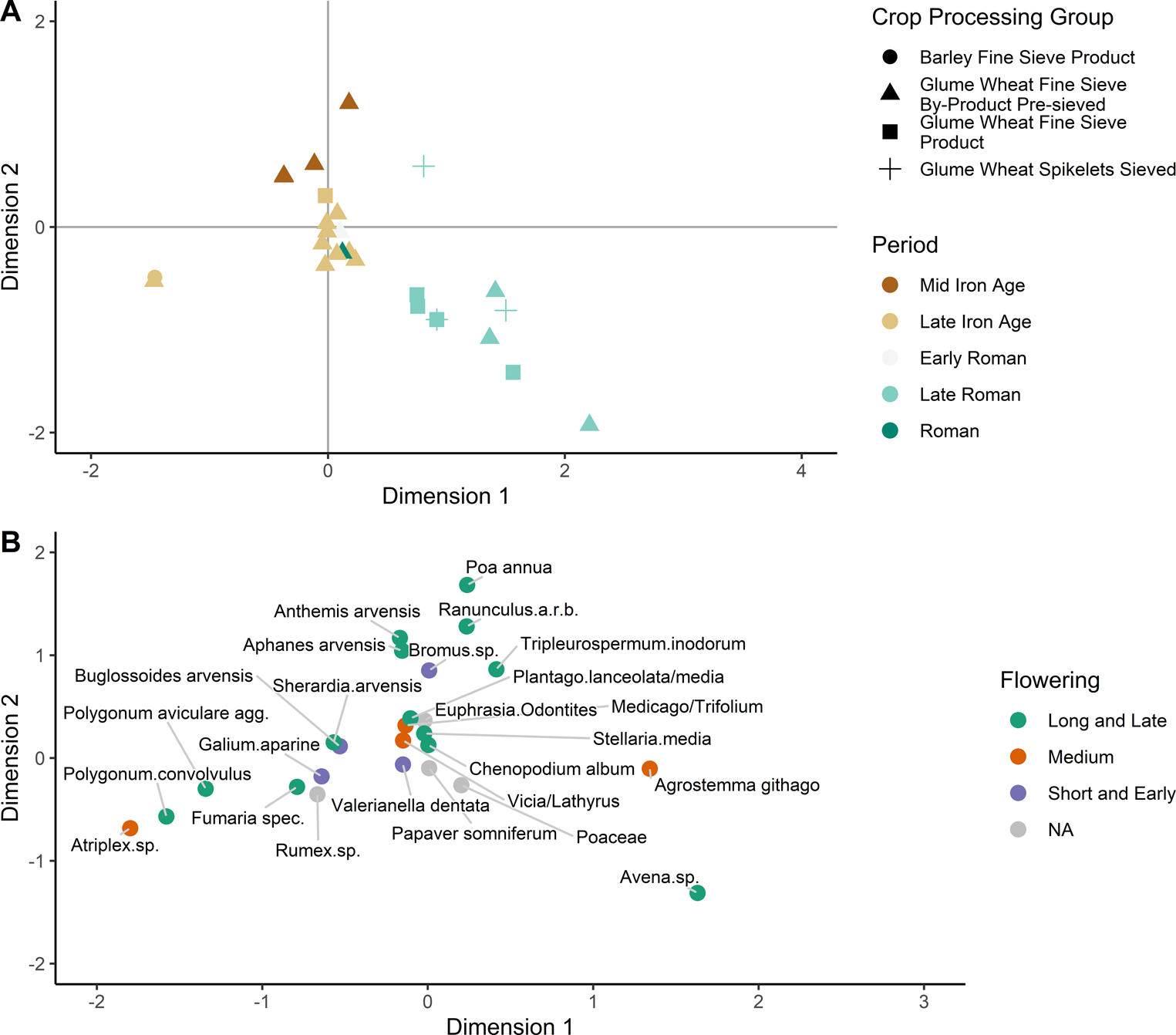
Figure 3. Correspondence analysis plot of A) samples and B) taxa. All samples were classified by crop- processing group, taxa were assigned to sowing time: twenty-nine samples, twenty-five taxa, where Dimension 1 accounts for 25.1 per cent, and Dimension 2 accounts for 21.3 per cent of variation in the contexts.
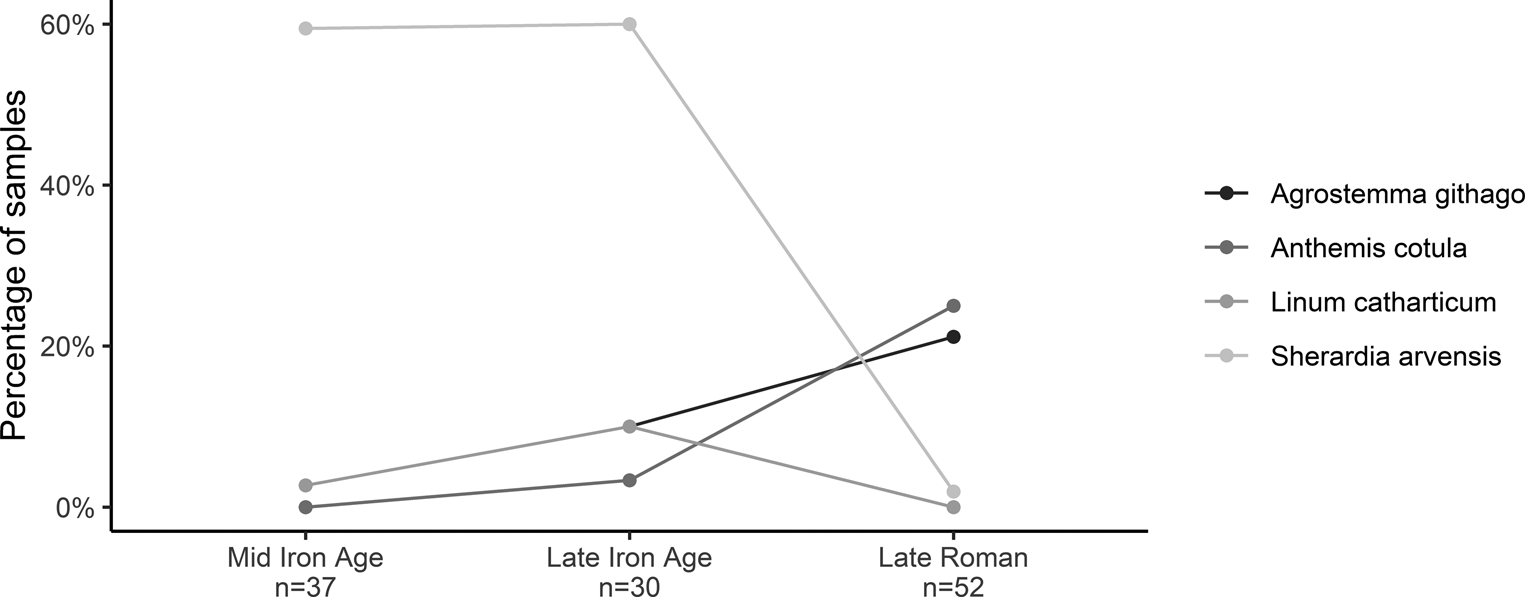
Figure 4. Ubiquity of key weed taxa within all samples per period.
Finally, Campbell (Reference Campbell and Cunliffe2008: 68) highlighted a decline in weeds of calcareous grassland taxa—Sherardia arvensis and Linum catharticum—in the Roman period, and a rise in the presence of Agrostemma githago and Anthemis cotula. Anthemis cotula is a weed associated with the cultivation of clay soils (Kay, Reference Kay1971) and Agrostemma githago becomes widespread as a successful grain mimic in the north-western provinces (Lodwick, Reference Lodwick, Allen, Lodwick, Brindle, Fulford and Smith2017: 20). These patterns are summarized in Figure 4. There are clear indications that cultivation of calcareous grassland declined and shifted instead to clays, but these conclusions must be tempered by the date of introduction of these taxa in the Late Iron Age/Roman period, and their increased presence in cleaned crop samples processed in corn-drying ovens contra small weed seeds.
Clearly, the taphonomic complexities of charred cereal assemblages limit us from drawing firm conclusions on these aspects of crop choice and husbandry. Hence, the application of functional weed ecology and stable isotope analysis is used here to explore aspects of crop status and cultivation practices.
Weed ecology analysis
Functional weed ecology analysis was undertaken by comparing the composition of samples from the Danebury and environs sites (Supplementary Table S8) with modern studies in Haute Provence (extensive farming practices) and fields in Asturias (intensive), following Bogaard and colleagues (Reference Bogaard, Hodgson, Nitsch, Jones, Styring and Diffey2016). Samples from the Middle Iron Age span the extensive, low-input end of the spectrum (Figure 5) and those from the Late Iron Age also span the same area of the axis. Whilst the samples from the Roman period also span the extensive end of the spectrum, when samples from Grateley are highlighted, they lie towards the far end of the axis; they are nearly all more negative than the centroid of the modern Provence fields and contemporary samples from the Fullerton villa. Unfortunately, insufficient weeds were present in samples from Dunkirt Barn to be included in the analysis. Hence, cultivation conditions at Grateley can be inferred as indicating very low disturbance and low soil fertility. This confirms that crop husbandry practices were extensive from the Middle Iron Age to the late Roman period. Furthermore, practices at late Roman Grateley became more extensive, with a reduction in levels of soil disturbance and fertility.
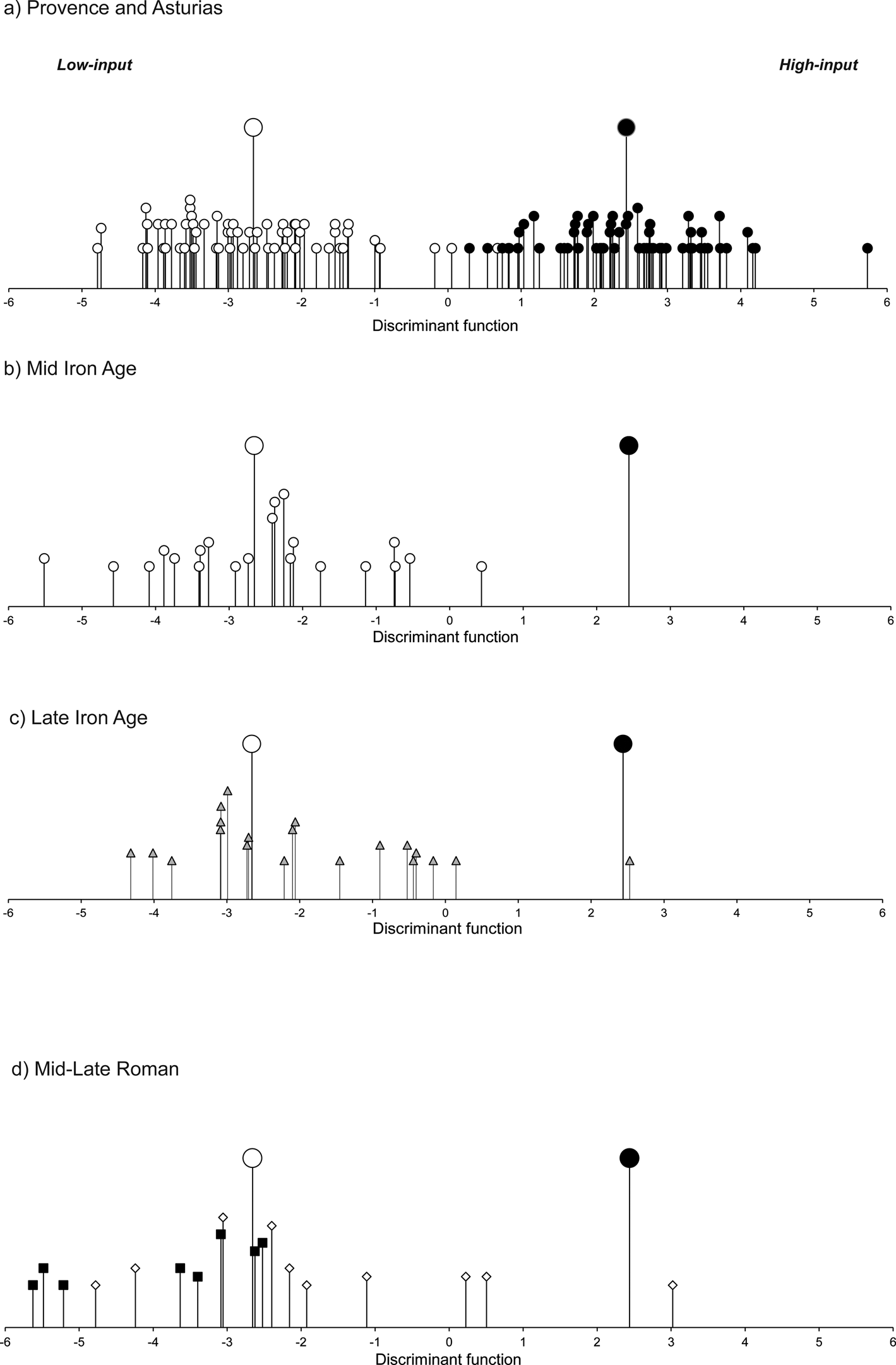
Figure 5. a) Relationship of fields in Haute Provence (open circles) and fields in Asturias (filled circles) with the discriminant function extracted to distinguish these two groups (larger symbols indicate group centroids). b–d) Relationship of archaeobotanical samples to the discriminant function (larger symbols indicate centroids for the modern groups). b) Middle Iron Age samples. c) Late Iron Age samples. d) Mid–late Roman Grateley (squares) and other samples (diamonds).
Crop stable isotope analysis
Given the small numbers of samples from continuous sub-phases at some sites (Danebury, Dunkirt Barn, Grateley), all samples from each site were grouped as one analytical unit other than Grateley; at the latter site, the samples from the Late Iron Age banjo enclosure have been separated from the mid to late Roman villa phases. Isotopic results are summarized in Table 1 and provided in the Supplementary Material (Tables S4–S7).
Table 1. Summary of crop stable isotope values.

Overall, the range of δ 15N values spans –0.2 to 6.3‰, and for δ 13C from –25.3‰ to –21.7‰, which is consistent with values obtained from other studies of cereal crop isotopes from temperate Europe (Aguilera et al., Reference Aguilera, Zech-Matterne, Lepetz and Balasse2018; Lodwick et al., Reference Lodwick, Campbell, Crosby and Müldner2021). Values also vary within each site, consistent with the wide-ranging faunal isotopic values of the Danebury region in the Iron Age (Stevens et al., Reference Stevens, Lightfoot, Hamilton, Cunliffe and Hedges2013). At Danebury hillfort, twenty-four barley and twenty-seven spelt samples show that, whilst the ranges for barley (5.8‰) and spelt (4.4‰) are wide, the mean δ 15N values are not statistically different from each other (3.2‰ for barley, and 3.4‰ for spelt), indicating that the two crops were grown in comparable soil conditions. The mean δ 13C for barley is wider (2.2‰) than spelt (1.5‰), but again the mean values (–23.9‰ for barley and –22.5 ‰ for spelt) have the expected ~1‰ spacing, due to differing phenology and timing of the growth cycle (Wallace et al., Reference Wallace, Jones, Charles, Fraser, Halstead and Heaton2013), as also observed in north-eastern France (Aguilera et al., Reference Aguilera, Zech-Matterne, Lepetz and Balasse2018) and elsewhere in Iron Age and Roman Britain (Lodwick et al., Reference Lodwick, Campbell, Crosby and Müldner2021). Hence, the cultivation of barley and spelt were undertaken in comparable conditions, although barley was potentially grown under more varied conditions.
Whilst the smaller datasets available from the two Late Iron Age farmsteads (Suddern Farm and Thruxton) and two late Roman villas (Thruxton and Fullerton) do not allow the same level of comparison within one site, two consistent patterns emerge. The absence of statistical difference in δ 15N values of barley and spelt at individual sites (Supplementary Table S6) and a consistent spacing of 1.2–1.5‰ in the δ 13C of barley and spelt at individual sites (Figure 6). The only settlements where this pattern differs is at Grateley, where the δ 13C separation is only 0.8‰, suggesting distinct crop growing conditions, with barley perhaps now grown in slightly drier conditions.
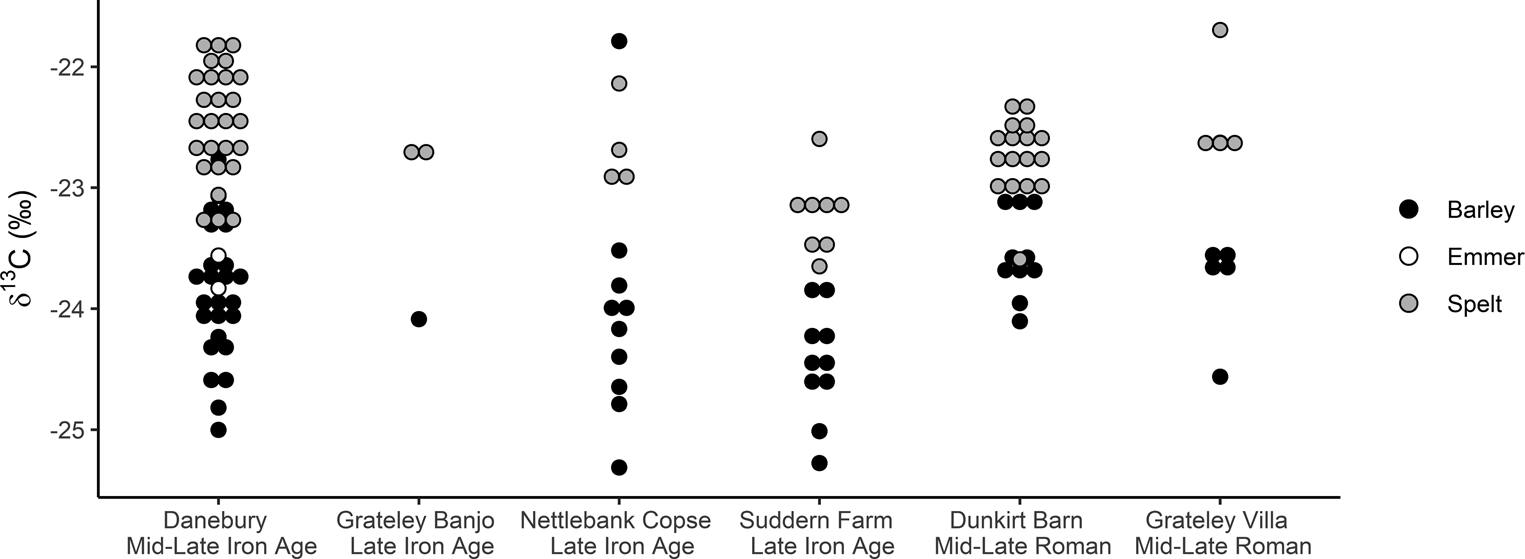
Figure 6. δ 13C stable isotope values from Middle Iron Age to late Roman sites in the Danebury environs.
Moving from individual sites to considering the cultivation within the landscape over time, δ 13C values are lowest during the Late Iron Age at Nettlebank Copse and Suddern Farm. The δ 13C values for spelt wheat at Suddern Farm are statistically significantly lower than earlier values at Danebury, and later values at the villas of Dunkirt Barn and Grateley (Figure 6). The same distinction is also seen in barley between Suddern Farm and the two villa sites (Supplementary Table S7). In arid and semi-arid environments, water availability is considered the greatest influence on δ 13C values (Wallace et al., Reference Wallace, Jones, Charles, Fraser, Halstead and Heaton2013), but in temperate environments the drivers behind shifts in crop carbon values are less well investigated; more elevated δ 13C values are considered to indicate drier and/or less shaded growing conditions (Bonafini et al., Reference Bonafini, Pellegrini, Ditchfield and Pollard2013). Light and nutrient availability as well as temperature may, however, also play a greater role (Aguilera et al., Reference Aguilera, Zech-Matterne, Lepetz and Balasse2018). In a study of southern Germany, lower δ 13C values at two Late Neolithic lakeshore sites (–2‰ to –28‰) were considered to be due to cultivation in more shaded and potentially wetter soils (Styring et al., Reference Styring, Rösch, Stephan, Stika, Fischer and Sillmann2017b).
Multiproxy analysis of palaeoenvironmental records across Britain (Charman, Reference Charman2010: 1548) has identified periods of wetter weather in all phases of settlement considered here (310–90 bc, 50 bc–ad 80, and ad 280–580) and hence cannot explain alone the lower values during the Late Iron Age. Furthermore, the landscape on the Hampshire Downs is considered to have been largely open at this point, with woodland restricted to areas of clay and steep hills (Cunliffe, Reference Cunliffe2000: 43–45). A more likely reason for changes in the carbon isotope values is soil variation, as recently suggested for southern Scandinavia, where an increase in soil organic content and water-holding abilities was linked to lower δ 13C values (Gron et al., Reference Gron, Larsson, Gröcke, Andersen, Andreasen and Bech2021: 201). Here I suggest that changes in carbon values are due to shifts in areas of the landscape that were cultivated. Suddern Farm and Nettlebank Copse are both on chalk spurs overlooking valleys (Figure 7). Here, lower δ 13C values could indicate the location of fields on damper valley soils. Grateley and Dunkirt Barn, where higher values are recorded, are on the edge of clay plateaus. The δ 13C values at Grateley are comparable in range to those recorded at late Roman Stanwick in Northamptonshire, where fields were located on claylands (Lodwick et al., Reference Lodwick, Campbell, Crosby and Müldner2021), and could indicate the cultivation of seasonally dry clay soils.

Figure 7. Soil map of the study area.
Long-term variations can also be observed in the nitrogen results. Most of the drivers for change in δ 15N values (sea spray, burning, waterlogging, and aridity) are not relevant in this landscape (Szpak, Reference Szpak2014). Hence is it suggested that fluctuations in manuring levels are the main cause of changes in the δ 15N values through time. The intensity of manuring can be inferred from using wild faunal values to estimate the δ 15N values of unmanured wild vegetation (Styring et al., Reference Styring, Rösch, Stephan, Stika, Fischer and Sillmann2017b). The ‘wildest’ herbivore represented in the Iron Age and Roman faunal assemblages is the horse. As immature horse bones are rare, the animals are considered to have lived in feral herds (Allen, Reference Allen, Allen, Lodwick, Brindle, Fulford and Smith2017: 126). Isotopic data from 113 Middle and Late Iron Age horses from Danebury and surrounding settlements (Stevens et al., Reference Stevens, Lightfoot, Hamilton, Cunliffe and Hedges2013; Hamilton et al., Reference Hamilton, Sayle, Boyd, Haselgrove and Cook2019), with 4‰ subtracted as an estimate of trophic level, produce an unmanaged ‘wild’ herbivore baseline value of δ 15N 0.1‰ ±1.2 SD for unmanured crops (Fraser et al., Reference Fraser, Bogaard, Heaton, Charles, Jones and Christensen2011). Given that this may be an over-estimation of the wild herbivore baseline, due to horses eating some cereals, it points to a low δ 15N value for unmanured crops. The δ 15N values of crops receiving moderate and high levels of manure can be extrapolated from experimental studies (Fraser et al., Reference Fraser, Bogaard, Heaton, Charles, Jones and Christensen2011). This indicates most crops were receiving no or low levels of manure.
Through time, there are minor fluctuations in δ 15N values at the Middle and Late Iron Age settlements (Figure 8), followed by an elevation in δ 15N values at Dunkirt Barn in barley and spelt, and a decrease in values at the villa at Grateley, with a mean value of 1.5‰ for barley and of 1.6‰ spelt, the latter statistically different from the majority of earlier sites (Supplementary Table S6). Here the low δ 15N values, in tandem with the indication from functional weed ecology of low-input husbandry practices, indicate an extensive farming system.

Figure 8. δ 15N values from Middle Iron Age to late Roman sites in the Danebury environs. Unmanured baseline calculated from δ 15N values of 113 Middle and Late Iron Age horses reported in Stevens et al., Reference Stevens, Lightfoot, Hamilton, Cunliffe and Hedges2013 and Hamilton et al., Reference Hamilton, Sayle, Boyd, Haselgrove and Cook2019.
Continuity in maslin crop cultivation compliments this picture of long-term change in crop husbandry. The mean δ 15N values for barley and spelt are not statistically different from each other at any site (Supplementary Table S6). The difference between the means of δ 13C values of wheat and barley is also consistent. At Grateley, however, the δ 13C spacing is closer, at 0.8‰, than at all other sites, suggesting a move towards the separate cultivation of barley and spelt as monocrops.
Crop Stable Isotope Values: Further Insight into Animal Ecology
The detailed faunal isotope studies in our region make it possible to consider the integration of plant and animal husbandry. The low δ 15N values of herbivores on calcareous soils have previously been reported for fauna from the Hampshire chalk downlands and nearby Berkshire Downs (Hamilton et al., Reference Hamilton, Sayle, Boyd, Haselgrove and Cook2019; Schulting et al., Reference Schulting, le Roux, Gan, Pouncett, Hamilton and Snoeck2019: 204). Suggested reasons for low δ 15N values include lower bacterial activity (Schulting et al., Reference Schulting, le Roux, Gan, Pouncett, Hamilton and Snoeck2019), a tighter nitrogen cycle reducing nitrogen loss, and/or a higher proportion of legumes in the animal diet (Hamilton, Reference Hamilton2016: 42, 167). The low δ 15N values reported here indicate that the low δ 15N values are also present in the cereal crops.
Figure 9 shows the estimated rachis/stalk values of cereals, calculated by subtracting 2.4‰ from grain δ 15N values (Fraser et al., Reference Fraser, Bogaard, Heaton, Charles, Jones and Christensen2011). These are ~4‰ below mean cattle (4.2‰) and sheep (4.4‰) δ 15N values for Danebury, and mean cattle (3.8‰) and sheep (4.3‰) δ 15N values for Suddern Farm, indicating straw and chaff was likely to have been a major dietary resource, confirming previous findings (Lightfoot & Stevens, Reference Lightfoot and Stevens2012).

Figure 9. Comparison of faunal and crop nitrogen isotope values at Danebury and Suddern Farm. Diamonds indicate extrapolated values of spelt and barley straw and chaff at each site.
Discussion
Crop husbandry in a downland landscape
The combination of archaeobotanical, functional weed ecology, and isotopic analysis provides a long-term picture of continuity in crop choice and crop husbandry practices. The agricultural system was based on spelt wheat and barley, cultivated in relatively extensive conditions from at least the Middle Iron Age. Whilst the δ 15N values for both crops span the low and medium manuring bands at Danebury, Nettlebank Copse, and Suddern Farm, two distinct cultivation practices can be seen at the two villas. At Dunkirt Barn, the isotopic samples cluster in the medium manuring band, suggesting extensive practices with some manure input. At Grateley, by contrast, the δ 15N values of crops are barely higher than the unmanured wild vegetation baseline, and the analysis of the weed ecology shows samples clustering at the extensive end of the axis (Figure 5), indicating a move to lower disturbance and fertility practices.
The Iron Age agricultural system on the chalk downlands is considered to have been based on the integration of crops and sheep, the dominant animal, whose role included providing manure for crops (Albarella, Reference Albarella, Haselgrove and Moore2007). This is thought to have involved direct manuring, whereby sheep were folded in fields to graze on stubble after harvest (Cunliffe, Reference Cunliffe2000: 43; Hamilton, Reference Hamilton and Cunliffe2000: 61). Direct manuring is considered characteristic of farming settlements on the downlands as a strategy to maintain fertility on thin calcareous soils that lasted into the post-medieval period (Kerridge, Reference Kerridge1967: 42–51; Cunliffe, Reference Cunliffe2000: 44, 141; McOmish et al., Reference McOmish, Field and Brown2002: 3, 107). As crops were harvested by uprooting in the Early Iron Age (Campbell, Reference Campbell and Cunliffe2000), i.e. leaving no stubble, this system of sheep folding on stubble must have developed later, in the Middle Iron Age. Crop isotope studies and previous faunal isotope studies provide direct support for the persistence of sheep folding into the Late Iron Age (Figure 9).
There are no modern studies to show the isotopic signature of direct manuring from animals penned within a field. Traditional farming practices on the chalk downs folded flocks at densities of c. 1000 sheep per acre per night, with two sheep per 2.5–3.5 m2 (Kerridge, Reference Kerridge1967: 44–45). If each sheep produces 500 dung pellets a day (Wallace & Charles, Reference Wallace and Charles2013), this results in 170 pellets per m2, equivalent to c. 150 g per m2 of fresh dung (Welch, Reference Welch1982). Hence this would fall below the ‘medium’ manuring bands of annual application 20/30 tonnes per ha, equivalent to 1–1.5 kg of manure per m2 (Fraser et al., Reference Fraser, Bogaard, Heaton, Charles, Jones and Christensen2011) and equates well with the low–medium δ 15N values of crops from the Middle and Late Iron Age settlements. Whilst faunal isotopic data are not available for the late Roman period, I suggest here that a decrease in δ 15N values at Grateley indicates a breakdown of the sheep-folding system as the villa maximized cereal production. Cereal by-products were increasingly removed from the fields to be used as fuel in grain-drying ovens (Campbell, Reference Campbell and Cunliffe2008), perhaps leaving insufficient stubble in the fields to maintain the sheep-folding system.
Crops were cultivated in maslins throughout the period under study. There is no evidence for Late Iron Age monocropping from either the isotopic analysis or the archaeobotanical re-analysis. Whilst the spacing between δ 13C values of barley and spelt fluctuates between sites, it is unclear whether an increase or decrease in the barley–wheat offset indicates cultivation in distinct conditions. Adjustments in sowing time, and hence the cereal growth period, may also account for changes at Grateley. A tightening of the barley–wheat spacing at Grateley could indicate a shift towards separate sowing times for each crop. Spring-sown cereals would reach maturity around a month later than autumn-sown crops. Currently, potential evaporation values are highest in June and July on the Hampshire Downs (Robinson et al., Reference Robinson, Blyth, Clark, Comyn-Platt, Finch and Rudd2016), and spring-sown crops could have higher δ 13C values, indicating drier conditions during the growth period than autumn-sown barley, although caution is needed (Wallace et al., Reference Wallace, Jones, Charles, Fraser, Halstead and Heaton2013). This corresponds well with evidence for spelt-dominated assemblages, building support for the monocropping of spelt wheat in the late Roman period (Figure 2). In contrast, Dunkirt Barn pursued a different strategy of maintaining medium levels of manuring and appears to have returned to maslin cropping, evidenced by a mix of barley and spelt wheat recovered from a corn-drying oven (Campbell, Reference Campbell and Cunliffe2008: 71).
Overall, this analysis supports previous findings from Roman Britain that indicate a continuation of Iron Age crop husbandry systems but at an increased scale, both of the extent of land under cultivation and in the degree of processing (Campbell, Reference Campbell and Bird2016; Van der Veen, Reference Van der Veen, Millett, Revell and Moore2016; Lodwick, Reference Lodwick, Allen, Lodwick, Brindle, Fulford and Smith2017). Extensive cereal farming practices were based on those developed in the Iron Age, underpinned by the increasing circulation of iron for tools (Jones, Reference Jones, Gosden, Hamerow, de Jersey and Lock2007), the emergence of larger community units, and a move from uprooting to sickle harvesting which enabled sheep folding. Crucially, the integration of plant and animal husbandry was not an invention of the Roman imperial period in Italy but part of pre-existing Iron Age practices in temperate Europe. In this case study, these traditional practices fragmented after cereal production was pushed to a maximum in the late Roman period.
Socio-economic consequences of extensification
Market-orientated cereal production, as indicated through the batteries of grain-drying ovens at both Dunkirt Barn and Grateley villas, was achieved not through ‘intensification’, that is increased labour input per unit area, as postulated by Heinrich et al. (Reference Heinrich, Hansen and Erdkamp2021), but by extensification. This would support previous arguments that an increase in Roman cereal production was obtained through large agricultural units (Erdkamp, Reference Erdkamp, Erdkamp, Verboven and Zuiderhoek2015: 20). Whilst there is now a general picture of cereal extensification in central and southern Britain, the differing isotopic values of cereal grains between Dunkirt Barn and Grateley indicate that there were agricultural choices to be made by individual villa settlements. More activities indicating high social status are evidenced at Dunkirt Barn, a large winged corridor villa with a bath house, ornamental garden, and hypocaust-heated aisled barn (Cunliffe & Poole, Reference Cunliffe and Poole2008b), and perhaps fine dining, with domestic fowl and pig in the faunal assemblage (Hammon, Reference Hammon and Cunliffe2008: 88). In contrast, Grateley was a masonry villa, with buildings dedicated to beer and grain production (Cunliffe & Poole, Reference Cunliffe and Poole2008a), pursuing extensive cultivation to maximize cereal production.
Extensive farming requires the mobilization of large labour forces at harvest time (Halstead, Reference Halstead1995) and may rely on the coercive powers of the Roman state and/or the presence of sizeable villa estates. These annual cereal harvest rhythms have been studied at length by Shaw (Reference Shaw2015) in North Africa. On the Hampshire Downs, archaeobotanical and isotopic analysis make it possible to compare the impact of labour mobilization on rural communities. Both Grateley and Dunkirt Barn are situated within large enclosures of around 15 ha (Allen, Reference Allen, Smith, Allen, Brindle and Fulford2016: fig. 4.25). Whilst only a small area of each settlement has been excavated, we can infer that a sizeable workforce was needed, given the numerous grain-drying ovens and elite buildings which would have needed maintaining. Moreover, the aisled barns—long seen as spaces for agricultural labours and activities (Wallace, Reference Wallace2018)—may have also acted as assembly places (Cunliffe, Reference Cunliffe2009: 195). Furthermore, villas are often characterized as estate centres, able to draw on a range of local labour (Gerrard, Reference Gerrard2013: 93). Although the notion of villa estates has been strongly critiqued (Millett, Reference Millett, Millett, Revell and Moore2016), the extensive farming systems evidenced here mean that agricultural labour beyond that available in the villa buildings would have been needed at harvest time.
This workforce may have been free or enslaved people resident in the aisled barns or tenants including residents of nearby farmsteads that are only known through survey work (Cunliffe, Reference Cunliffe2009), or perhaps urban populations in Venta Belgarum (Winchester), as suggested by Taylor (Reference Taylor2013b: 417). Seasonal co-working at harvest time would have been significant for the lived identities of these agricultural labours (Taylor, Reference Taylor2013a). The social realities for these communities may have entailed seasonal agricultural labour with particularly hectic periods at harvest-time in late summer. Osteoarchaeological analysis of rural communities in Roman Britain has highlighted their poor health. The joints of adults show degeneration and stress, including joint injuries on upper and lower limbs from agricultural labour, rib fractures, and spinal trauma, linked to agricultural labour (Rohnbogner, Reference Rohnbogner, Smith, Allen, Brindle, Fulford, Lodwick and Rohnbogner2018: 340–42, 338, 345). The agricultural labour necessitated by extensive farming practices would hence have affected both the health and seasonal social patterns of farmworkers.
Agricultural sustainability
I end this discussion by turning to the environmental consequences of cereal extensification. Roman agricultural systems on calcareous landscapes have been studied in detail in only a few regions. Calcareous downlands are not present in Italy, and hence do not feature in the writings of the Roman agronomists. In eastern France, calcareous landscapes are found in the Champagne, where agriculture focused on barley (Lepetz & Zech-Matterne, Reference Lepetz, Zech-Matterne and Reddé2018: 352), whilst in Britain, calcareous soils in the south, north-east, and east of the country were ‘intensively’ exploited (Smith & Fulford, Reference Smith, Fulford, Smith, Allen, Brindle and Fulford2016: 391).
The farming system in the Danebury environs has been considered fairly resilient, with labour distributed across the production of both animal (meat, milk, and wool) and cereal products (Campbell, Reference Campbell and Cunliffe2008: 100). The favourable environment, with mild winters, a long growing season, and light soils allowed farmers to survive bad years, as observed in recent periods (Afton, Reference Afton1996). In particular, loam soils overlying chalk are considered to be resilient for cereal production (Campbell, Reference Campbell and Cunliffe2008: 68). However, the picture of extensification through time provided here by isotope and weed ecology analyses differs from that shown by previous studies (Campbell, Reference Campbell and Cunliffe2008; Hammon, Reference Hammon and Cunliffe2008) in that it suggests a broad continuity from the Iron Age to the Roman period in crop and animal husbandry.
In parallel with the shift to specialized villa production strategies, the cultivation of different landscapes emerged. A move to clay soils during the Roman period is supported by the increase in frequency of Anthemis cotula, a decline in calcareous grassland taxa (Figure 4), and higher crop δ 13C values, suggesting a focus on dryer clay soils. This targeting of heavy clay soils may have been facilitated by developments in tillage technology and made necessary by an exhaustion of calcareous soils (Cunliffe, Reference Campbell and Cunliffe2008: 19, 165). Barley is considered to be more suitable for cultivation on calcareous soils as it copes well with dryer, less fertile conditions compared to wheat (Zohary et al., Reference Zohary, Hopf and Weiss2012: 52). Roman rural settlements in the broader calcareous region have been observed as having higher proportions of barley (Allen & Lodwick, Reference Allen, Lodwick, Allen, Lodwick, Brindle, Fulford and Smith2017), yet there was a focus on spelt cultivation at some settlements, including Grateley, which may have ultimately led to unsustainable farming systems. Limited crop repertoires are widely understood not to withstand environmental change (Reed & Ryan, Reference Reed and Ryan2019). Furthermore, the extensive cultivation of spelt wheat would have been reliant on the mobilization of a large labour force. With the disappearance of spelt wheat being exploited centrally in the fifth century ad, and the fragmentation of society at that time, a large-scale extensive system would become unsustainable. Indeed, by the early medieval period, spelt wheat had gone from being the predominant crop in central-southern Britain to a trace occurrence (McKerracher, Reference McKerracher2018).
Conclusion
This study presents the first multi-site investigation of macroscopic plant remains, functional weed ecology, and crop stable isotope values in the Iron Age to Roman period. A picture of crop homogeneity and extensification emerges, with late Roman agricultural practice characterized by the cultivation of drier clay soils with extensive agricultural practices, and the use of agricultural labour focused on harvest time and large-scale crop processing. Whilst continuity over the longue durée of evidence from plants and animals has previously been interpreted as evidence for stability (Cunliffe, Reference Cunliffe2009: 173), this study has shown that further extensification, and perhaps monocropping, took place in the late Roman period, albeit with different villas pursuing slightly different agricultural strategies. The environmental implications of this overall strategy are that it was an unsustainable system. With the fragmentation of the Roman Empire in the West, this system based upon homogenous crop choice and extensive farming practices ceased to operate. In addition to highlighting such economic issues, this study also uses a multi-stranded approach to bring to the fore labour relation and highlight the consequences for third- and fourth-century agricultural villa estates.
Whilst this study characterizes the Middle Iron Age, Late Iron Age, and late Roman practices on the chalk downlands, the key period of transition in the later first and second century ad remains unexplored due to a lack of suitable material for analysis. Further work is required to disentangle the pre-existing Iron Age agricultural trajectories from those influenced by the Roman Empire to establish the nature of the shift towards large-scale cereal processing.
Supplementary Material
To view supplementary material for this article, please visit https://doi.org/10.1017/eaa.2022.47.
Acknowledgements
This work was supported by All Souls College, University of Oxford. Access to archives was granted by Hampshire Cultural Trust and Martin Jones, University of Cambridge. I am grateful to Elizabeth Stroud and Peter Ditchfield for assistance with isotopic analysis and to Amy Bogaard for assistance with FIBs analysis. All errors remain the author's own.
The EJA editors would like to thank Angela Trentacoste for her assistance with this article.