Introduction
Antimicrobial-resistant (AMR) bacteria are a growing issue of global concern, threatening our capacity to treat common infections, prolonging the duration of hospitalisations and increasing treatment costs and mortality among infected patients [Reference Prestinaci, Pezzotti and Pantosti1, Reference Murray2]. Antimicrobial use (AMU) in the community, healthcare and agriculture sectors are thought to be the principle drivers of increases in antimicrobial resistance (AMR) [Reference Holmes3]. The recruitment of resistance genes in the environmental resistome, genetic mutations and inter or intra-species exchange of antimicrobial resistance genes (ARGs) between bacteria are also of recognised importance [Reference Von Wintersdorff4].
The Codex Guidelines for Risk Analysis of Foodborne AMR [5] provide a useful and transparent way to assess the risk of AMR arising through the food chain. One component of the risk analyses pathway is a risk profile; suggested elements for inclusion are described in detail in the Codex Guidelines [5]. A foodborne AMR risk profile presents the current state of scientific information on identified AMR food safety issues to inform risk managers prior to decision-making. A risk profile incorporates various elements for consideration including information on antimicrobial-resistant (AMR) microorganism(s) and/or determinant(s). One of the challenges with conducting risk profiles, is providing pertinent information in a timely manner. A scoping review (ScR) is an ideal synthesis tool to use when the aim is to identify and map available evidence on a given topic [Reference Arksey and O'Malley6, Reference Anderson7]. Several suggested elements within a risk profile lend themselves to ScRs while other elements need only succinct targeted pieces of information to address risk managers questions. In this case, we were interested in describing the findings and range of research related to the distribution, frequency and concentrations of extended-spectrum β-lactamase (ESBL)- producing bacteria in salmon and shrimp.
In terms of the AMR hazard, in 2017, the World Health Organization (WHO) published a list of antibiotic-resistant ‘priority pathogens’ that pose the greatest threat to human health [Reference Tacconelli8]. Priority 1 is considered ‘critical’ and includes extended-spectrum β-lactamase-producing Enterobacteriaceae (ESBL-E) [Reference Tacconelli8]. These bacteria can cause severe infections and have become resistant to many antimicrobials, including the best available treatments for multi-drug resistant bacteria [Reference Tacconelli8]. ESBL-E are a group of organisms which demonstrate resistance to 3rd generation cephalosporins such as cefuroxime, cefotaxime, ceftriaxone, ceftazidime, cefepime and cefpirome [Reference Bradford9, Reference Livermore10]. They are also able to hydrolyse a wide range of β-lactams, including numerous penicillins and monobactams. ESBLs are mainly encoded on plasmids but may also be present in the chromosome [Reference Bradford9]. ESBLs consist of class A β-lactamases including TEM/SHV derivatives and CTX-M enzymes (e.g., CTX-M-1, CTX-M-15 and CTX-M-55, CTX-M-2, CTX-M-8, CTX-M-9 and CTX-M-25) [Reference Castanheira, Simner and Bradford11]. There are 183 and 178 variants of TEM and SHV enzymes, respectively; however, not all of them are ESBLs [Reference Bush and Bradford12].
Resistance to 3rd generation cephalosporins is alarming, as they are considered high priority antimicrobials by the WHO, of ‘very high importance’ in human medicine according to Health Canada's categorisation, and are used as ‘last resort’ treatments in some multidrug-resistant infections [13, 14]. Resistance to 3rd generation cephalosporins can correlate with the presence of ESBL genes (bla TEM, bla SHV and bla CTX−M) [Reference Maina15]. Of particular note, bacteria containing ESBL genes may exhibit high levels of cross-resistance to various human and veterinary cephalosporin formulations (ceftriaxone, ceftazidime, cofotaxime, ceftiofur) [Reference Li16].
Enterobacteriaceae encompass several important human pathogens including Klebsiella pneumonia, Escherichia coli, Enterobacter spp., Serratia spp., Proteus spp., Providencia spp and Morganella spp, which have been reported in agriculture and aquatic animal production systems [Reference Economou and Gousia17, Reference Koonse18]. Of particular relevance, their ubiquitous presence and propensity for genetic exchange make them potential reservoirs of AMR genes [Reference Rozwandowicz19].
In Canada, there has been a recent discovery of Enterobacteriaceae that possess and express carbapenemase and β-lactamase–conferring genes in isolates from imported retail seafood [Reference Janecko20]. Salmon and shrimp are of interest because they are the most commonly consumed seafood products in Canada [21]. Most salmon and shrimp products consumed in Canada are farmed (aquacultured). Atlantic salmon (Salmo salar) is the main farmed salmon species, is primarily produced domestically, and its production is subject to strict regulations on site selection, quality control and AMU [22, 23]. The main farmed shrimp species worldwide is the white legged shrimp (Litopenaeus vannamei) [Reference Liao and Chien24]. Shrimp are mostly grown and imported to Canada from Central America and Southeastern Asia [23], where several classes of antimicrobials are used and regulations concerning use and enforcement are different from those in Canada [Reference Rico25].
The objective of this study was to conduct a ScR of the current state of knowledge on the distribution, frequency and concentrations of ESBL-E in salmon and shrimp. Information from this ScR will contribute to a larger risk profile of ESBL-producing bacteria from seafood following the Codex guidelines. To our knowledge, this is the only ScR of ESBL-E in these popular seafood commodities. A secondary objective was to identify areas where more research is needed and support evidence-informed decision-making concerning the need for ongoing surveillance of AMR in seafood in Canada.
Methods
This ScR was designed to answer the following question: ‘What is the distribution, frequency and/or concentrations of extended-spectrum β-lactamase (ESBL)- producing Enterobacteriaceae in shrimp and salmon?’. At the time this review was initiated (2019), the bacterial scientific order was labelled as ‘Enterobacteriaceae’, however, in 2020 a taxonomy change was implemented to make the change to ‘Enterobacterales’ [Reference Adeolu26]. For consistency between the a priori study protocol and results we have decided to use the terminology ‘Enterobacteriaceae’ throughout this review. A ScR protocol was created a priori to ensure reproducible, transparent and consistent results. The protocol includes a rationale for the project, search algorithms, abstract screening form and data characterisation form. The protocol can be found in the Supplementary Material. Creation of the protocol and execution of the review was completed by a team of individuals with multi-disciplinary expertise in epidemiology, public health, knowledge synthesis, risk assessment, aquaculture and veterinary medicine. This ScR followed the Preferred Reporting Items for Systematic review and Meta-Analyses extension for Scoping Reviews (PRISMA-ScR) guidelines [Reference Tricco27].
Search strategy
The full electronic search strategy can be found in the ScR protocol (Supplemental Material). In brief, the search strategy included general AMR terms (e.g., ‘Drug Resistance’), ESBL specific terms (e.g., ‘ESBL’ or ‘beta-lactams’), population terms (e.g., ‘salmon’ or ‘shrimp’ or ‘seafood’) and bacteria terms (e.g., ‘E. coli’ or ‘Salmonella’ or ‘Enterobacteriaceae’) combined into search strings/algorithms. There was no limit on publication date.
The initial search was conducted in November 2019 and an update was conducted in February 2021. These searches were conducted in five bibliographic databases: MEDLINE® (1946 to current), AGRICOLA™ (1970 to current), EMBASE® (1974 to current), CAB Abstracts (1973 to current) and Food Science and Technology Abstracts (1969 to current). The electronic search was verified by hand searching the reference lists of three literature reviews focused or partially focused on AMR in salmon or shrimp [Reference Thornber28–Reference Miller and Harbottle30]. Spot checks were also done in reference lists of other relevant literature as the authors were reviewing.
A search was also conducted in Google Scholar using the algorithm: (antibiotic resistance) and (ESBL) and (Enterobacteriaceae) and (salmon or shrimp). To limit the amount of irrelevant articles in our search, only articles that mentioned AMR were considered for inclusion.
Relevance screening and inclusion criteria
A relevance screening form was developed a priori. Abstracts, titles and keywords were screened for relevance using the following question: Does this citation describe primary research on: presence of ESBLs in Enterobacteriaceae of salmon and shrimp? Two reviewers screened titles and abstracts for relevance and any conflicts were resolved by a third reviewer.
Study characterisation and extraction
The study characterisation form was developed a priori and can be found in the ScR protocol (Supplemental Material). The study characterisation form aimed to classify and characterise the research on ESBL-E from salmon and shrimp. The form first reconfirmed the relevance of the publication prior to extracting important characteristics of the study. This included the following study details: language (due to limited translation resources, articles published in languages other than English or French were excluded), location, study date, document type, focus of paper (i.e., relevant to ESBLs in the food chain), food commodity, if AMR data were extractable for each food commodity, Enterobacteriaceae investigated, and outcomes. Two reviewers characterised full-text articles and any conflicts were resolved by a third reviewer.
Scoping review management, data charting and analysis
The search strategy results were exported and de-duplicated in Mendeley (Mendeley Ltd, 2021). These articles were then exported to Rayyan, a web-based systematic review software, where relevance screening (keyword and abstract only) was conducted [Reference Ouzzani31]. Full text of articles deemed relevant were then procured. Data extraction and analysis was conducted in DistillerSR® (Evidence Partners Inc., Ottawa, Canada).
Descriptive tabulation of all pertinent information that aids in the characterisation and illustration of the available knowledge of the Distribution, Frequency and Concentration of ESBL-producing Enterobacteriaceae of salmon and shrimp were conducted in MS Excel (Microsoft Office, 2016). Meta-analysis or other statistical analyses were not conducted due to the scoping nature of this study and the heterogeneity in study data/methods.
Results
After a rigorous literature search and deduplication process, 4913 abstracts and titles were screened for relevance from which 457 full-text articles were reviewed. A total of 16 research articles on ESBL-E in salmon or shrimp were characterised in this ScR (Fig. 1. PRISMA Diagram). Exclusions were due to non-relevance to the research topic (n = 348), non-primary research (n = 18), language (n = 49) and inability to procure full-text article (n = 10). Six articles explicitly looked for ESBL-E (either phenotypically or genotypically) and none were identified, and therefore no outcomes were available for extraction. These were retained, however, for any future analysis or systematic review that would be completed on this topic (S2, Supplementary Material). Furthermore, 10 studies were excluded because they did not report AMR data separately for each food commodity (S3, Supplementary Material).

Fig. 1. PRISMA flow diagram for scoping review of the distribution and frequency of extended-spectrum β-lactamase (ESBL)-producing Enterobacteriaceae in shrimp and salmon.
Retained articles were published between 2014 and 2020 (Fig. 2. Histogram of Publication Dates). The time between isolate collection and publication ranged from 1 to 4 years. Studies were conducted in India (n = 3), Thailand (n = 2), Vietnam (n = 3), Myanmar (n = 1), Denmark (n = 1), Germany (n = 1), Algeria (n = 1), Portugal (n = 1), Spain (n = 1), Brazil (n = 1) and the United States (n = 1). Studies either reported the prevalence or frequency of ESBL-producing bacteria; none reported extractable concentrations. Studies were primary-research articles, cross-sectional in design and utilised a convenience sampling approach. No surveillance reports monitoring the occurrence of ESBL-E in these food commodities were identified in the ScR; however, it should be noted that grey literature was only investigated through a Google search in this review and not an intensive search of government/other grey literature sources.
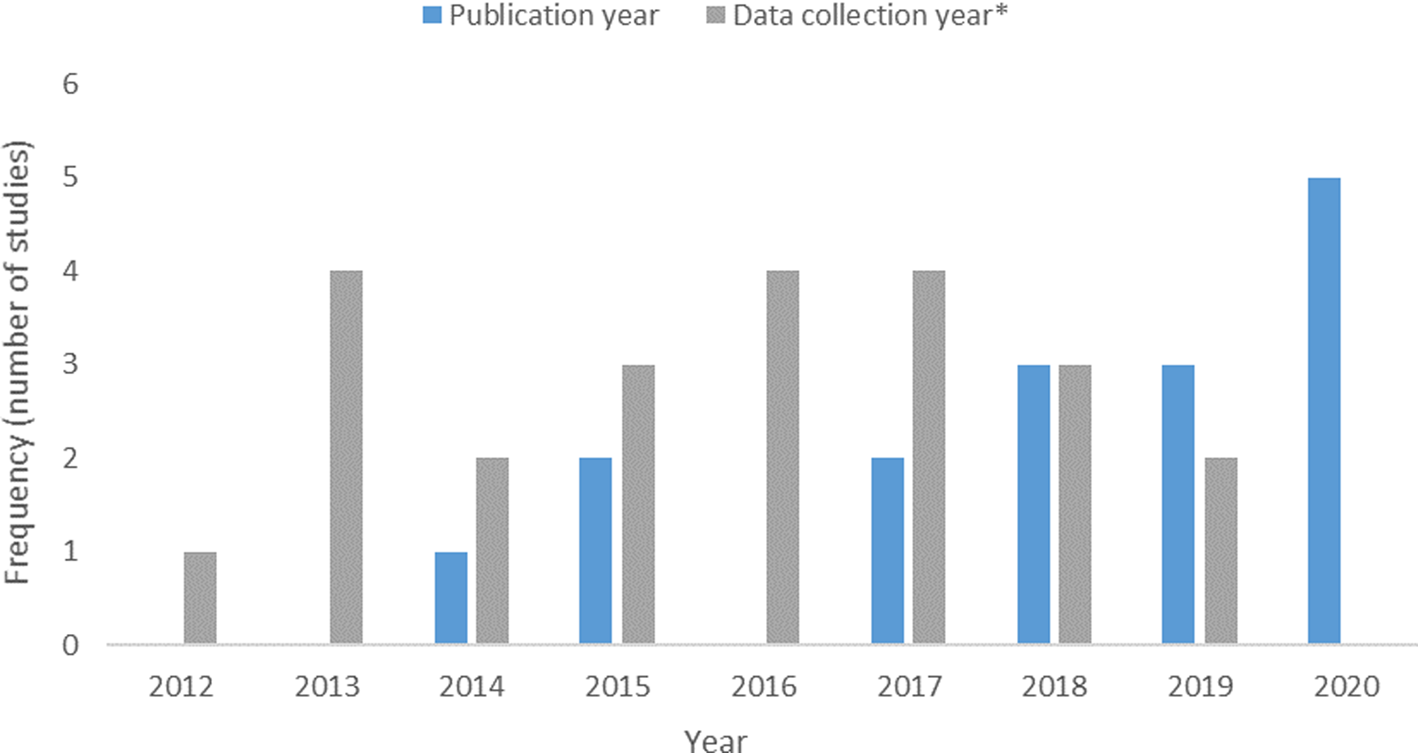
Fig. 2. Histogram of the years of data collection and publication year for studies included in the scoping review of the distribution and frequency of extended-spectrum β-lactamase (ESBL)-producing Enterobacteriaceae in shrimp and salmon. Each year of data during a multi-year collection period is recorded as one value under frequency of data collection*.
The majority of included studies (n = 14) identified ESBL-E in shrimp/prawns while only 2 studies identified ESBL-E in salmon (Table 1). All of the samples were collected at the retail/market level and studies reported collection of fresh/raw samples (n = 7), frozen samples (raw or precooked, n = 2) and samples of unknown status (n = 5).
Table 1. Details of studies that reported the presence of extended-spectrum β-lactamase (ESBL)-producing Enterobacteriaceae in salmon and shrimp
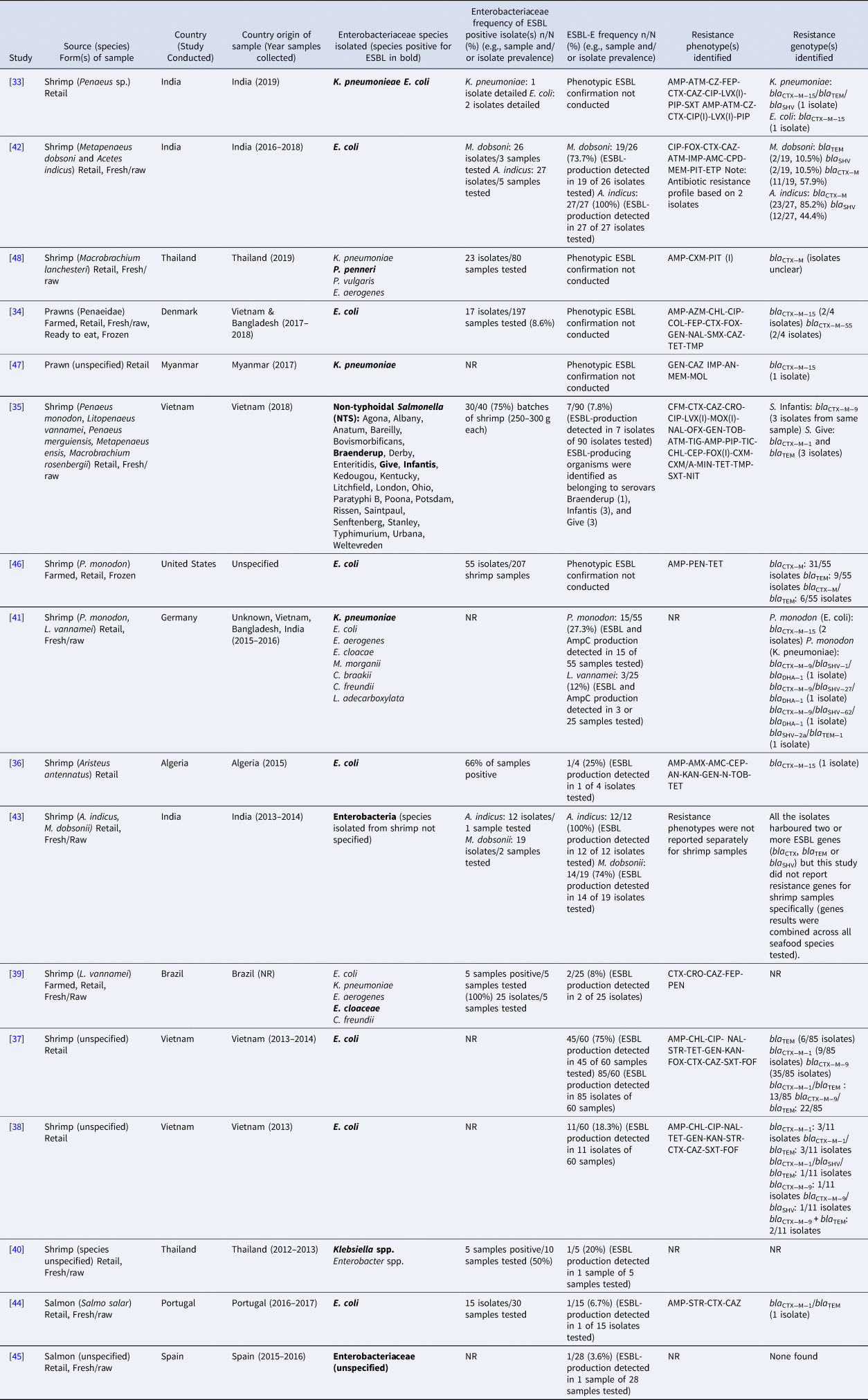
AMC, Amoxicillin-clavulanic acid; AMG, Aminoglycosides; AMP, Ampicillin; AMX, Amoxicillin; AN, Amikacin; APL, Amphenicol; ATM, Aztreonam; AZM, Azithromycin; BAC, Bacitracin; CAZ, Ceftazidime; CEC cefaclor; CEP, Cephalothin; CFM, Cefixime; CFP, Cefoperazone; CHL, Chloramphenicol; CIP, Ciprofloxacin; CLA, Clavulanic acid; CLI, Clindamycin; COL, Colistin; CPD, Cefpodoxime; CPM, carbapenem; CRO, Ceftriaxone; CTX, Cefotaxime; CXM, Cefuroxime; CXM/A, Cefuroxime Axetil; CZ, Cefazolin; DOX, Doxycycline; EN, Enrofloxacin; ERY, Erythromycin; ETP, Ertapenem; FEP, Cefepime; FFC, Florfenicol; FIS, Sulfisoxazole; FLQ, Fluoroquinolones; FOF, Fosfomycins; FOX, Cefoxitin; GEN, Gentamicin; IMP, Imipenem; KAN, Kanamycin; LVX, levofloxacin; LZD, linezolid; MAC, Macrolides; MEM, Meropenem; MEZ, Mezlocillin; MIN, Minocycline; MOL, Moxalactam; MOX, Moxifloxacin; N, Neomycin; NAL, Nalidixic acid; NIT, Nitrofurantoin; NOR, norfloxacin; NOV, Novobiocin; OFX, ofloxacin; OTC, Oxytetracycline; PEN, Penicillin; PIP, Piperacillin; PIT, Piperacillin/Tazobactam; PMB, polymyxin B; RIF, Rifampicin; SAM, Ampicillin/sulbactam; SMX, Sulfamethoxazole; SPC, Spectinomycin; SSS, Sulfisoxazole; STR, Streptomycin; SXT, Trimethoprim-sulfamethoxazole; TEL, Telithromycin; TET, Tetracycline; TIC, Ticarcillin; TIG, Tigecycline; TIO, Ceftiofur; TOB, Tobramycin; TMP, Trimethoprim; TZB, Tazobactam; VAN, Vancomycin; NR, Not reported; I, Intermediate Resistance.
Phenotypic results
Among the included studies, 15/16 used selective media and/or antimicrobial susceptibility testing results to identify potential ESBL phenotypes. Double disk diffusion/double-disk synergism was conducted in 11 studies to confirm ESBL production [Reference Jarlier32]. When specified, CLSI (CLSI guideline (M100). Wayne, PA: Clinical and Laboratory Standards Institute) cut-points were used for susceptibility classification in 9 studies and EUCAST (European Committee on Antimicrobial Susceptibility Testing. Breakpoint tables for interpretation of minimum inhibitory concentrations and zone diameters. http://www.eucast.org/clinical_breakpoints/) were used in 2 studies. While not all studies may have been designed to look for resistance to multiple antimicrobial classes, 6 studies reported isolates resistant to >2 antimicrobial classes [Reference Sivaraman33–Reference Le38] and 4 reported resistance to >4 antimicrobial classes [Reference Ellis-Iversen34, Reference Yen35, Reference Le37, Reference Le38].
Phenotypic detection of ESBL-E included: Enterobacter cloaceae in shrimp (L. vannamei) from Brazil [Reference de Almeida39], Klebsiella spp. in shrimp from Thailand [Reference Boonyasiri40], K. pneumoniae and E. coli in shrimp (Penaeus monodon and L. vannamei) from Vietnam, Bangladesh and India [Reference Vu41], E. coli in shrimp (Aristeus antennatus) from Algeria [Reference Dib36], E. coli in shrimp from Vietnam [Reference Le37, Reference Le38], E. coli in shrimp (Metapenaeus dobsoni and Acetes indicus) from India [Reference Singh, Nayak and Kumar42], non-typhoidal Salmonella in shrimp from Vietnam [Reference Yen35], unspecified Enterobacteria in shrimp (M. dobsoni and A. indicus) from India [Reference Singh43], E. coli in salmon (S. salar) from Portugal [Reference Silva44] and unspecified Enterobacteriaceae in salmon from Spain [Reference Vitas45].
Genotypic results
Several studies reported on single- and multi-ESBL genes in isolates derived from shrimp and salmon samples, identified via polymerase chain reaction and whole genome sequencing. However, they did not always report the specific gene type for the identified bla TEM, bla SHV and bla CTX−M genes. Because not all bla TEM and bla SHV genes encode ESBLs, a decision was made to only include unspecified bla TEM/bla SHV genes when another ESBL gene like bla CTX−M was identified in the study or phenotypic prevalence of ESBL production was detected in the study.
Three studies detected ESBL-E genotypes in imported seafood products (Table 1). ESBL genes bla CTX−M−15 and bla CTX−M−55 were detected in E. coli isolated from prawns purchased in Denmark that were imported from Vietnam and Bangladesh [Reference Ellis-Iversen34]. Single- and multi-ESBL genes were detected in shrimp purchased in Germany that were imported from Vietnam, Bangladesh and India including E. coli with bla CTX−M−15 and K. pneumoniae isolates with bla CTX−M−9/bla SHV−1/bla DHA−1, bla CTX−M−9/bla SHV−27/bla DHA−1, bla CTX−M−9/bla SHV−62/bla DHA−1 and bla SHV−2a/bla TEM−1 [Reference Vu41]. Single- and multi- ESBL genes were also detected in E. coli isolated in imported shrimp (origin not specified) in the United States including bla CTX−M, bla TEM and bla CTX−M/bla TEM [Reference Nawaz46].
The majority of ESBL genes detected in shrimp were in Enterobacteriaceae isolated from samples collected in Vietnam (Table 1). Single- and multi-ESBL genes were detected in E. coli isolates and included bla TEM, bla CTX−M−1, bla CTX−M−9, bla CTX−M−1/bla TEM, bla CTX−M−9/bla TEM, bla CTX−M−9/bla SHV, bla CTX−M−1/bla SHV/bla TEM [Reference Le37, Reference Le38]. Also detected were bla CTX−M−9 and bla CTX−M−1/bla TEM in Salmonella Infantis and S. Give isolated from shrimp in Vietnam, respectively [Reference Yen35].
In India, ESBL genes detected in shrimp included bla TEM, bla SHV and bla CTX−M from E. coli [Reference Singh, Nayak and Kumar42] and bla CTX−M−15 and bla CTX−M−15/bla TEM/bla SHV from E. coli and K. pneumoniae, respectively [Reference Sivaraman33] (Table 1).
Other genes identified included bla CTX−M−15 from E. coli isolated in shrimp from Algeria [Reference Dib36], bla CTX−M−15 from K. pneumoniae isolated in shrimp from Myanmar [Reference Sugawara47] and bla CTX−M from P. penneri in shrimp from Thailand [Reference Thongkao and Sudjaroen48]. The only study that identified an ESBL gene from salmon detected bla CTX−M−1/bla TEM in an E. coli isolate in a sample from Portugal [Reference Silva44]. The bacterial species and genes identified are summarised in Table 2.
Table 2. Synthesis table of ESBL genes found in Enterobacteriaceae from salmon and shrimp

Discussion
Our ScR found that E. coli, K. pneumoniae, and other Enterobacteriaceae producing ESBLs have been isolated in retail shrimp products originating in the Asian region. Only three studies reported ESBL-E in imported food products, two in Europe and one in the United States. This ScR found that there is a lack of published Canadian data on ESBL-E in salmon and shrimp. Research and surveillance activities regarding ESBL-E in Canadian retail seafood have been undertaken; the results of these studies are in the process of being analysed and disseminated (Personal communication with Janecko et al. [Reference Janecko20]). Publication of this work will help address the data gap with AMR/ARGs in retail seafood in Canada. However, other important ARGs have been identified. One excluded Canadian study identified carbapenem-resistant Enterobacteriaceae in 8 of 1328 seafood samples investigated as part of a targeted retail surveillance project by the Public Health Agency of Canada [Reference Janecko20]. Of 928 shrimp samples, 2 imported from Vietnam contained E. cloacae harbouring blaIMI − 1, and 1 from Bangladesh contained E. aerogenes harbouring blaIMI-2 [Reference Janecko20]. While no ESBL-E was identified in shrimp, 2 clam samples from Vietnam contained E. cloacae harbouring bla NDM−1, bla TEM and bla OXA−1 and were phenotypically resistant to ampicillin, cefoxitin and amoxicillin/clavulanic acid [Reference Janecko20]. In another study, Janecko et al. found that imported shrimp were the source of over half (65%, 37/57) of all ESBL-E isolates identified in imported and domestic seafood products purchased at retail in Canada (Personal communication with Janecko et al. [Reference Janecko20]). ESBLs were harboured by E. coli, K. pneumoniae and Enterobacter sp., with bla CTX−M genes (bla CTX−M−15, bla CTX−M−27 and bla CTX−M−55) being the most common ESBL determinant in shrimp and seafood (Personal communication with Janecko et al.). Together, these findings indicate that contaminated retail seafood products in Canada may be a source of human exposure to AMR-organisms.
Across the published studies, there was a general and consistent lack of reporting of study methodology and results of interest to our ScR, precluding the ability to conduct a systematic review and/or meta-analysis on this specific study question. Some heterogeneity may be attributable to different study locations, sampling methods and test methods used for measuring bacterial prevalence and AMR. Some studies reported overall sample prevalence while others reported isolate prevalence; making direct comparisons and synthesis difficult. The reporting of the raw quantitative data concerning sample types and isolates as well as the genetic sequences obtained from PCR or whole genome sequencing analysis should be encouraged. Provided as supplemental information, it would enable inter-study comparison, even if the results as published are not evaluated using the same methodology. Additionally, the use of standard laboratory procedures for bacterial isolation where possible, and international standards for antimicrobial susceptibility testing or epidemiological cut-off values to evaluate antimicrobial susceptibility would permit the comparison of study results derived from different sample types or regions. Further limitations of the studies evaluated here included small sample sizes and convenience sampling instead of random sampling, which would have provided better representativeness of the information across the shrimp and salmon populations. We considered whether the taxonomy change which resulted in a new scientific order, ‘Enterobacterales’ in 2020 could be a limitation affecting our results; the only impact being that some species we had considered under the umbrella of Enterobacteriaceae moved to their own families (i.e., Morganella and Providencia are now under the Morganellaceae family and Yersinia and Serratia are now under the Yersiniaceae family) [Reference Adeolu26]. These all would have been captured by our study.
Our ScR has some limitations related to resourcing information on an emerging topic. Figure 2 demonstrates an increasing tendency in the number articles evaluating ESBL in seafood from 2012–2020. If the excluded publications presented in S2 and S3 in the Supplementary Materials had been included in this graphic, this tendency would be accentuated. This may speak to the increased interest in AMR research by Universities, Governments and International organisations [Reference Luz49, 50]. The top six countries exporting seafood to Canada are the US, China, Vietnam, Chile, Thailand and India respectively [51]. Among the 16 studies cited in this paper, India, Vietnam, Thailand and the US are present with 3, 3, 2 and 1 publications respectively. Additionally the US study examines shrimp imported into the United States, so the results would not be indicative of the local AMR environment/situation. The top two exporters of seafood to Canada, the US and China, were absent. Further, the US and Chile are the top exporters of salmon to Canada, however, no studies examining AMR in salmon were identified in this ScR [51]. Even if consideration were given to the 6 articles excluded for negative results and the 10 for which the reporting did not sufficiently stratify the seafood types, it would encompass 3, 4 and 1 additional articles attributed to the source countries of China, Vietnam and Chile respectively. The lack of proportional representativity of studies concerning countries exporting seafood to Canada belies an important data gap. The ScR and risk profile approach are time and resource demanding. We acknowledge that further relevant research on this topic has been published since the search strategy for this review was executed. After conducting this review we identified 3 studies that are worth mentioning here for further context, discussion and to further supplement the findings. Because these studies were not identified in a systematic manner we are unable to say that they represent the totality of relevant research published on this topic since our search was executed. One study from India reported that out of 77 shrimp samples, 2 tested positive for ESBL-E. coli and 4 for ESBL-K. pneumonia [Reference Sivaraman52]. The genes bla CTX−M−1 and bla CTX−M−9 were each identified in 6 E. coli isolates from shrimp; bla TEM and bla SHV genes were isolated from 10 K. penumoniae isolates [Reference Sivaraman52]. A study from Vietnam identified ESBL-E. coli in 19.6% (22/112) of retail shrimp examined [Reference Le53]. Finally, a study from the United States found bla CTX−M in 42% and bla SHV genes in 6% of domestic wild caught imported shrimp; no ESBL genes were found in farmed shrimp imported from Ecuador [Reference Sharma54]. However, pragmatism and timeliness are needed for making synthesis studies of ESBL-E available for decision-making; hence, this ScR provides useful and relevant information needed for current decision-making.
While the studies may not have been originally designed to detect resistance to multiple classes of antimicrobials, this ScR identified 6 studies reported isolates resistant to >2 antimicrobial classes [Reference Sivaraman33–Reference Le38] and 4 reported resistance to >4 antimicrobial classes [Reference Ellis-Iversen34, Reference Yen35, Reference Le37, Reference Le38]. The use of β-lactam antimicrobials or other non-β-lactam antimicrobials such as aminoglycosides or (fluoro)quinolones administered via water or in-feed medications has the potential to select or enrich multidrug-resistant bacterial species carrying relevant co-resistance genetic determinants [Reference Cabello29, Reference Heuer55] ESBL producers often display multidrug resistance against several important antimicrobial groups due to the co-presence of ESBL genes and other AMR genes in the same plasmid [Reference Vu41, Reference Liu56–Reference Sapugahawatte58]. Cross-resistance and co-resistance can facilitate the selection and enrichment of multidrug resistant bacteria. Many other factors are also known to motivate the emergence of AMR in aquaculture including high stocking densities, elevated stress and infections, chemical additives, nutrient-rich environments and release of human waste into the environment [Reference Thornber28].
In terms of current regulations in the aquaculture industry, cephalosporins are not authorised for use in aquaculture operations in the European Union or North America [59–61]. However, their use has been reported in Asia including 1st generation cephalosporins (cephalexin and cefradine) in Vietnam and China and cefotaxime, a 3rd generation cephalosporin, detected in Chinese aquaculture operations [Reference Rico25, Reference Pham62–Reference Song64]. In Canada, there are a small number of non-β-lactam antimicrobials authorised for use in aquaculture including erythromycin, florfenicol, ormetoprim, oxytetracycline and sulphonamides [65]. Regulatory structure and enforcement may differ among aquaculture producing countries, as well as the antimicrobial classes used. There remains a lack of regulatory structure and enforcement in several countries known to export seafood to Canada [Reference Cabello29]. Canada has established risk management regulations such as Safe Food for Canadians Regulations which includes a preventative control plan for seafood importations [66]. Although this system can help maintain low levels of antimicrobial residues in relation to imported aquacultured products, it does not include an evaluation of AMR. Moving forward, surveillance using modern molecular techniques such as PCR and whole genome sequencing would be useful in detecting AMR genes of concern in imported seafood products. These are just brief considerations; a more comprehensive evaluation of aquaculture operations and regulations will be conducted as part of the risk profile.
Together, the findings presented in this review indicate that seafood products from countries with a history of exporting to Canada have been identified to harbour ESBL-producing Enterobacteriaceae. Salmon and shrimp would be appropriate initial targets for surveillance of seafood AMR in Canada, as they are the two most commonly consumed seafood products in Canada and would provide a snapshot of both domestic and imported seafood product for the distribution, frequency and concentrations of this AMR hazard(s) in the food chain. Genetic analysis of bacteria is of utmost importance in the risk analysis process. Understanding the interconnection between bacterial species, AMR genotypes and mobile genetic elements (i.e., plasmids) will be vital to the understanding of AMR selection and dissemination, particularly under a ‘One Health’ lens. Our intention is that the available literature described herein will support decision-making on this issue as a component of a larger risk profile.
Supplementary material
The supplementary material for this article can be found at https://doi.org/10.1017/S0950268822001819.
Acknowledgements
We would like to acknowledge the Public Health Agency of Canada library for help with article procurement.
Financial support
This study was funded by the Government of Canada as part of the Genomics Research and Development Initiative on Antimicrobial Resistance (GRDI-AMR).
Conflict of interest
None.
Data availability statement
Materials needed to replicate the findings of the article are available as Supplementary Materials.