INTRODUCTION
In Canada, as in most industrialized countries, campylobacteriosis is the leading cause of acute bacterial gastroenteritis. Although the disease is generally self-limiting, it is associated with significant cost to society and severe consequences for some affected individuals [Reference Majowicz1, Reference Engberg2]. The epidemiology of the disease is complex, and many environmental sources and potential pathways of transmission have been described [Reference Skelly and Weinstein3]. Poultry seems of particular importance in the general epidemiology of campylobacteriosis, since they are often colonized and can shed the bacteria in very high numbers [Reference Park4–Reference Bull6]. Following the slaughtering process, contamination of poultry meat is frequent [7, Reference Geilhausen, Newell, Ketley and Feldman8], and handling or consumption of poultry meat has been associated with human infections in many case-control studies [Reference Engberg2]. However, according to recent human campylobacteriosis attribution estimates, poultry meat only accounts for about 40% of all human cases of campylobacteriosis [Reference Vellinga and Van Loock9–Reference Havelaar13]. The intestinal tract of wild birds, cattle and sheep is also frequently colonized by Campylobacter [Reference Skelly and Weinstein3], and these species are suspected of being involved in the dispersal of the bacterium in the environment following direct dropping or manure spreading [Reference Stanley and Jones14]. Many other animal species can carry the bacterium, including swine, pets and wild animals [Reference Skelly and Weinstein3]. Following its excretion by animals, Campylobacter is often found in aquatic environments, and may then act as a source of human contamination through drinking or recreational water [Reference Thomas15]. Domestic flies are also considered a likely vector of Campylobacter [Reference Wright16–Reference Ekdahl, Normann and Andersson18].
One of the key features in the distribution of campylobacteriosis cases is a marked seasonality and age-related variation in incidence rates, for which no consensual explanation exists [Reference Engberg2, Reference Nylen19, Reference Hall20]. One theory states that the environmental factors involved in the causal chain of campylobacteriosis are not contributing evenly over the various age groups and seasons. For example, flies as mechanical vectors of campylobacteriosis pose a risk to humans only during the warm periods of fly activity [Reference Ekdahl, Normann and Andersson18]. Similarly, children are believed to be more vulnerable than adults to environmental contamination, due to specific behaviours such as crawling and oral habits (e.g. biting, chewing objects) [Reference Hall20, Reference Cohen21]. For some of the previously identified risk factors for campylobacteriosis, such as ruminant density, different pathways of transmission could be involved, with some being clearly seasonally dependent (e.g. surface water contamination following manure spreading and pasture use), whereas others are not (e.g. raw milk consumption). A few case-control studies on campylobacteriosis have been performed by age strata, focusing on individual risk factors such as food consumption patterns [Reference Unicomb22–Reference Doorduyn25]. A better understanding of the age and seasonal variability regarding the association between campylobacteriosis incidence and environmental characteristics is likely to enhance our understanding of the pathways involved in the transmission.
The objective of this study was to estimate the association between environmental characteristics and incidence of campylobacteriosis in Quebec, Canada, considering age and season.
METHODS
A retrospective ecological study was conducted using reported cases of campylobacteriosis in the province of Quebec between 1996 and 2006, inclusively. The study area was defined as the province of Quebec, with the exclusion of unpopulated areas, non-organized territories, incompletely enumerated Indian reserves and settlements, and areas located in the Nunavik (northern regions). The populated area was defined as the region covered by census blocks in which at least one person was living according to the 2001 census of Statistics Canada (GeoSuite 2001, Statistics Canada, no. 92F0150XCB).
Data acquisition on campylobacteriosis cases
Following approval of the project by the research ethics board of the Montreal Health and Social Services Agency, laboratory-confirmed cases of Campylobacter infection reported in Quebec between 1996 and 2006 inclusively were obtained from health and social service regions (MADO database). Information extracted from cases included age, gender, occupation, likely place of infection (outside Quebec or not), Campylobacter spp. isolated, various dates related to the episode (onset, sampling, report), and indicators of place of residence including the six-digit postal code. For each case, the date of onset was estimated according to the available dates related to case report and with adjustment for an estimated 3 days of incubation period [Reference Engberg2].
Definition of age group and seasonal periods
Based on our knowledge about the epidemiology of campylobacteriosis and the observed age distribution of cases, the following four age groups were defined for the data analysis: ⩽4 years, 5–15 years, 16–34 years, and ⩾35 years. The number of reported cases in each of these age groups was 2402, 3026, 10 971 and 12 120, respectively.
Two seasonal periods were defined in order to capture the period of low incidence during cooler months and the period of higher incidence during warmer months. We used the terminology ‘winter seasonal period’ and ‘summer seasonal period’ to refer to these two periods for simplicity, even though they were not strictly defined according to the calendar seasons. The summer seasonal period was defined as days between 1 April and 15 October, inclusively; the remaining days formed the winter seasonal period. These cut-offs were defined in order to exclude the effect of manure spreading from the winter seasonal period and to accommodate any potential time lag effect (in Quebec, manure spreading is allowed by law between 1 April and 1 October [26]). Weekly incidence of campylobacteriosis was plotted in order to illustrate the incidence rates associated with each seasonal period, with trend illustrated by a smoothed curve calculated for each data point with local polynomial regression over 5-week periods.
Definition of exposure variables
Following a comprehensive literature review on campylobacteriosis epidemiology, including the development of a conceptual model of transmission and a search for availability of data in Quebec [Reference Arsenault27], a set of explanatory variables were selected and defined as follows. The ruminant density per km2 and the poultry density per km2 variables were described as the total number of ruminants (goats, sheep, dairy cattle or beef cattle) or poultry (hens, broilers, or turkeys) in farms within a municipality, divided by the populated area of the municipality in km2. Farms were excluded from calculation if they had only marginal production. These densities were calculated from data from the 1998, 2001, and 2004 censuses conducted by the Ministry of Agriculture, Food and Fisheries of Quebec, and then averaged. The slaughterhouse variable was defined as the presence within the municipality of ⩾1 slaughterhouses handling poultry, cattle, and/or pigs under governmental inspection. Information was based on the official website of the Canadian Food Inspection Agency for slaughterhouses under federal inspection or the Ministry of Agriculture, Food and Fisheries of Quebec for those under provincial inspection. The population density per km2 was defined as the average number of people living in an area divided by the populated area in km2 calculated from data from the 1996, 2001, and 2006 censuses of Statistics Canada. The temperature and precipitation climate variables were calculated as the average of the maximal and minimal daily temperatures (or of the daily precipitation) by seasonal period, based on data between 1996 and 2003 (interpolated data were not available after this period at the time of the study). Data derived from the interpolation of meteorological data collected in stations across Quebec were provided by the National Land and Water Information Service of Agriculture and Agri-Food Canada.
Regression analysis
The geographical unit of analysis chosen for this study was the municipality. For each municipality, the total number of reported cases of campylobacteriosis was calculated by seasonal period and age group, cumulated over the 11 years. Poisson regression was used for modelling the incidence rate [Reference Lawson, Browne and Vidal Rodeiro28]. In order to take into account that repeated observations were made for each municipality (eight observations were recorded per municipality considering four age groups and two seasonal periods), the municipality was added as a random effect. Local health regions were included as another hierarchical random effect to adjust for the expected spatial dependence in residuals. They were defined by the boundaries of Local Community Service Center districts (abbreviated as CLSC in Quebec), which are the smallest health-related geographical divisions used in Quebec. When a municipality was served by more than one CLSC, these local health regions were aggregated to form a single geographical unit coincident with the municipality. As a result, the dataset consisted of 8504 observations clustered within 1063 municipalities and 117 CLSC health regions. The Poisson model was set as follows:
casesobs,mun,hr~Poisson(πobs,mun,hr)
log(πobs,mun,hr)=log(populationobs,mun,hr)+Xβ+νhr+μmun,hr
νhr~N(0,σhr2)
μmun,hr~N(0,σmun,hr2)
variance(cases|πobs,mun,hr)=πobs,mun,hr,
where cases is the cumulated number of cases observed for the age group and seasonal period within the municipality, π is the intensity parameter of the Poisson distribution, population is the number of people at risk by age group within the municipality (used as an offset), X is the regression matrix modelling explanatory variables, β is the unknown vector of regression parameters, ν and μ are random effects, obs denotes the observed number of cases, mun indicates municipalities, and hr indicates local health regions.
The explanatory variables entered in the model were all categorized. The animal density variables were categorized as: absence of production, low to moderate density, and high density; the cut-off for high density was set as the 85th percentile of the density distribution. The ruminant density per km2 was thus classified as none (n=138), ⩽(n=721), and >20 (n=204), whereas the poultry density variable per km2 was set as none (n=750), ⩽250 (n=128), and >250 (n=185). The slaughterhouse variable was categorized into three groups: none (n=1016), large slaughterhouse(s) handling poultry only (n=6), and other types of slaughterhouse(s) (n=41). A large poultry slaughterhouse was defined as from one of the fourth largest poultry transformation industries in Quebec, slaughtering 90% of poultry and nearly 100% of turkeys [29]. The population density per km2 variable was categorized into three groups based on the 15th and 85th percentiles of its distribution: ⩽6 (n=206), 6–400 (n=681), and >400 (n=176). The climate variables were categorized into three groups based on the 15th and 85th percentiles of its distribution, by season (Table 1).
Table 1. Cut-offs used and distribution of municipalities for climate variables studied for their ecological association with incidence of reported cases of campylobacteriosis in Quebec, Canada, 1996–2006
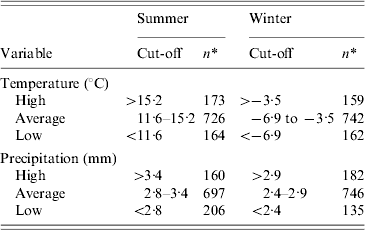
* Number of municipalities.
First, a full model was fitted to the data, with all exposure variables included as main effects. Additionally all one-way interactions with age group and season were included. The model was fitted with second-order penalized quasi-likelihood estimation, performed in MLwiN version 2.20 (University of Bristol, UK). A hierarchical backwards selection procedure was applied, using P>0·05 (i.e. Wald test at the α=5% significance level) as the rejection criterion. In the presence of a significant interaction with age groups, post-hoc tests were performed in order to evaluate between which pairs of age groups the interaction was significant (Wald test).
Incidence rate ratios with 95% confidence intervals (CI) were estimated to present the results; in presence of significant interactions with age or season, the incidence rate ratios were estimated for each level of the interacting variable(s). In order to explore the overall impact of variables on incidence, the average incidence rate of campylobacteriosis with 95% CI was estimated for each level of variable, adjusted for the average values of other variables included in the model, using functionalities implemented in MLwiN. Predicted incidences were then transformed in incidence per 100 000 person-years for interpretation. For each variable, pairwise comparisons in predicted incidences were then calculated in MLwiN to explore which levels were statistically different at α=0·05.
From the final model, standardized residuals at the municipality and health region levels were graphically evaluated for normality and presence of outliers. Spatial dependence in those two sets of standardized residuals was evaluated by calculating an empirical semivariogram in R version 2.11 (R Foundation, geoR package) with a 95% confidence band based on permutations. At the lowest level of the hierarchy, i.e. the observational level, the model fit was evaluated by estimating the extra-Poisson parameter.
RESULTS
Descriptive statistics
The age distribution and weekly distribution of campylobacteriosis incidence aggregated from the data spanning the 11 years of the study period are presented in Figures 1 and 2. The age distribution was bimodal, with a narrow peak in young children and a larger peak encompassing teenagers and young adults. The weekly distribution showed a strong seasonality, with a peak occurring around the first 3 weeks of August. The incidence rate at time of peak was about twice the incidence rate in winter.

Fig. 1. Distribution of incidence of reported cases of campylobacteriosis per 100 000 people by age in years, Quebec, 1996–2006. Vertical dashed lines indicate cut-off used for the definition of age groups used for testing ecological association with environmental variables.
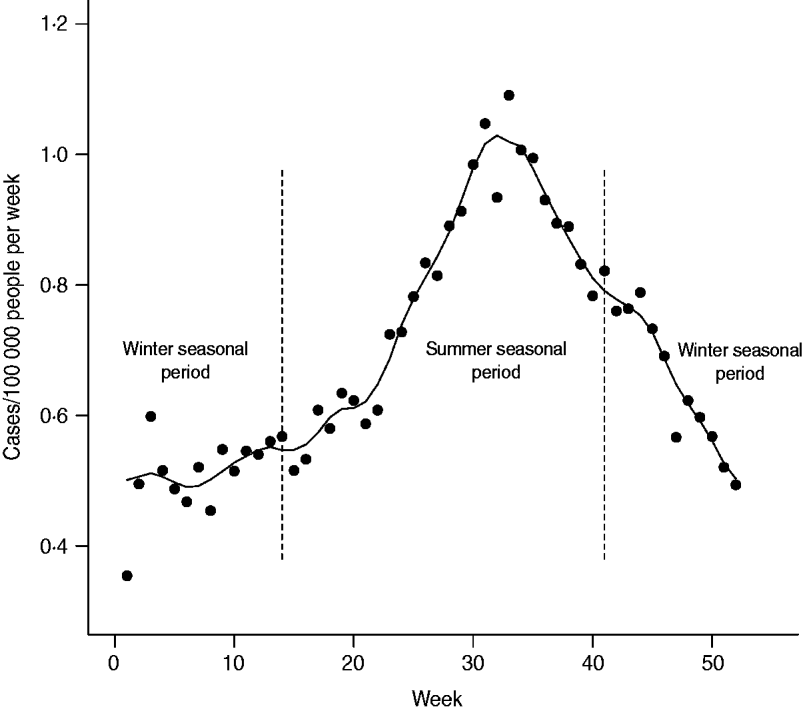
Fig. 2. Weekly average incidence of campylobacteriosis reported in Quebec over 11 years (1996–2006). Trend is illustrated by a smoothed curve and calculated by local polynomial regression over 5-week periods. Vertical dashed lines indicate cut-off used for the definition of seasonal periods (winter vs. summer) for testing ecological associations with environmental variables.
Regression analysis
During the model selection procedure, only the temperature variable was rejected from the model. In the final model, the age group variable presented highly significant interactions (all P<0·01, Wald test) with seasonal period, ruminant density, poultry density, population density and slaughterhouse. For the seasonal period variables, only the interactions with age group and population density were significant (P<0·01, Wald test) and thus kept in the model. The estimates of the random effects with standard error were 0·152 (0·027) for the local health regions and 0·147 (0·014) for the municipality. Standardized residuals at both municipality and health region levels were normally distributed based on normal probability plots and no evidence of spatial dependence or outliers was detected. The extra-Poisson parameter was estimated as 1·08, suggesting no departure from the Poisson distribution assumption.
The predicted mean and incidence rate ratio estimates from the final model are presented in Tables 2 and 3 for each variable and category. The variable ‘seasonal period’ had a strong and highly significant impact, with the predicted incidence of campylobacteriosis being 1·7–2·2 times higher in summer compared to winter. The effect of the seasonal period was greater for people aged 5–15 years compared to any other age group (all P<0·01, Wald test). The effect of age was also strong even after adjustment for other variables, with predicted incidences being three times higher in adults aged >35 years compared to children aged ⩽4 years (Table 2).
Table 2. Predicted mean of reported campylobacteriosis incidence per 100 000 people per year at the municipality level with 95% confidence intervals, according to environmental characteristics, Quebec, Canada, 1996–2006
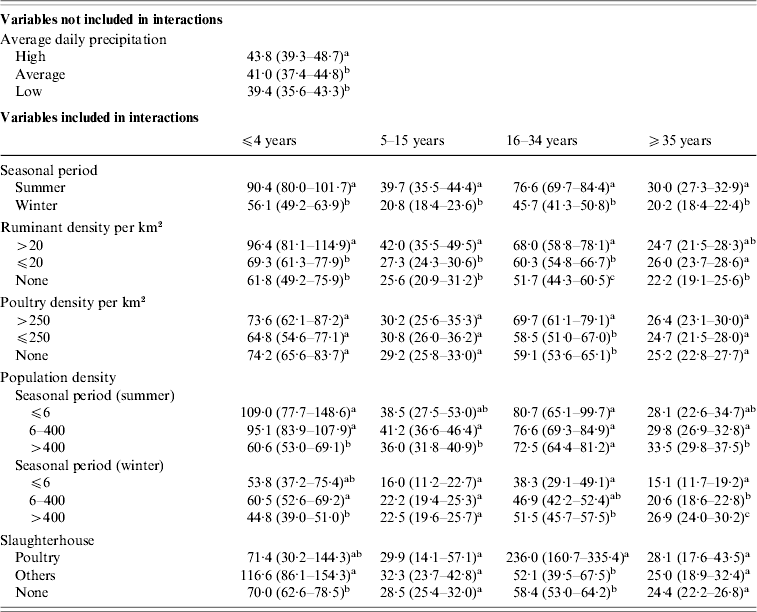
abc For each variable, difference in predicted incidence of campylobacteriosis between categories of variables with different superscripts are different from zero (by age group for variables included in interactions).
The variable ‘ruminant density’ was significantly associated with campylobacteriosis incidence for all age groups, with a consistently greater incidence in municipalities with ruminants compared to others (Table 3). The effect of high ruminant density was reduced as people became older, as illustrated in Figure 3. In general, differences in predicted incidences were small or not statistically different between municipalities with ruminant density ⩽20 compared to municipalities with no ruminant farms (Table 2). Larger differences ranging from 15 to 35 cases/100 000 person-years were observed between municipalities with ruminant density >20 compared to others, but only for children aged <16 years (Table 2).

Fig. 3. Mean predicted incidence of campylobacteriosis at municipality level according to ruminant density and age group in Quebec, 1996–2006. Different lower-case letters indicate statistically significant pairwise interaction between ruminant density and age groups (P<0·05, Wald test, 2 degrees of freedom).
Table 3. Incidence rate ratio estimates (95% confidence intervals) from a multi-level Poisson regression model predicting the incidence of reported cases of campylobacteriosis at municipal level in Quebec, Canada, 1996–2006Footnote *
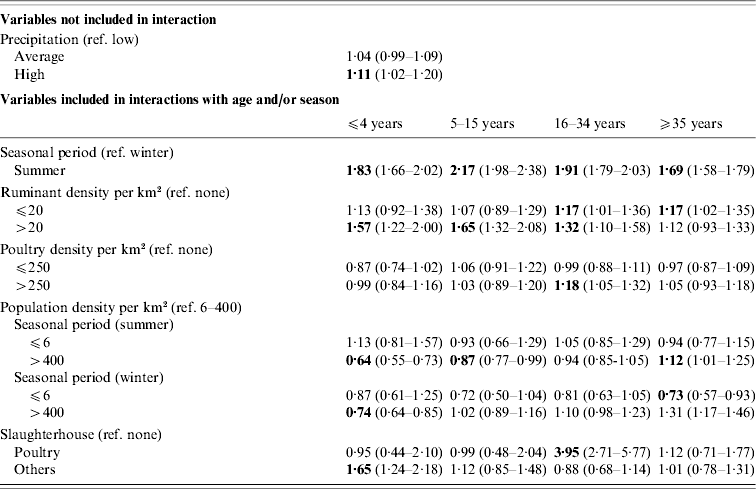
* Statistically significant estimates (P<0·05) are in bold.
For the ‘poultry density’ variable, an effect was detected in high-density municipalities, but this effect was restricted to the 16–34 years age group (Table 3). This was also reflected in the interactions, with the effect of poultry in the 16–34 years age group being different compared to every other age group (P<0·03, Wald test) as the only significant interactions. Among people aged 16–34 years, the differences in predicted incidence in municipalities with poultry density >250 compared to others was about of 11 cases/100 000 person-years (Table 2).
The ‘population density’ variable showed significant interactions with both the ‘age group’ and the ‘seasonal period’ variables (Table 3). We also explored the two-way interaction between population density, age, and seasonal period, but it was not found to be significant (P=0·49, Wald test). The overall effect of population density was different between each 2×2 combination of age group (all P<0·01, Wald test), with the exception of the 5–15 and 16–34 years age groups for which the effect of population density was not different (P=0·34). During the summer seasonal period, a high population density was a protective factor for children aged <16 years. This was also true during the winter period for children aged ⩽4 years only, whereas a low density was a protective factor in adults aged ⩾35 years. Adjusted for other covariates, the predicted incidence was particularly high in children aged ⩽4 years living in municipalities with population density <400 per km2, estimated to be 95–109 cases/100 000 person-years (Table 2).
The overall effect of slaughterhouses was significantly different between each pair of age groups (P<0·01, Wald test). The only exception was the 5–16 years age group compared to those aged ⩾35 years (P=0·42, Wald test); no effect of slaughterhouse was noted in these two age groups. For children aged ⩽4 years, the incidence rate was 1·65 times higher in municipalities with slaughterhouse(s) other than large ones for poultry compared to those without slaughterhouses (Table 3). The effect was different in people aged 16–34 years, for which the incidence rate was 3·95 times higher in municipalities with large poultry slaughterhouses. The predicted incidence for this age category within those municipalities was rather dramatic at 236 cases/100 000 person-years at risk, which was significantly higher than the predicted incidence associated with any other variable.
DISCUSSION
Ruminant density was associated with a higher incidence of campylobacteriosis, with a reduction in the strength of association as people grow older. The importance of ruminants as a source of human campylobacteriosis is supported by recent large population genetic studies conducted in the UK, where 18–39% of all human cases were attributed to cattle or sheep [Reference Wilson30, Reference Sheppard31]. Other genetic studies reported that the proportion of Campylobacter attributed to ruminant sources is larger in children from rural regions [Reference Strachan11, Reference Mullner32], which is consistent with our results. The infection of people by Campylobacter from ruminant sources could occur through the consumption of raw milk at the farm (note that the sale of raw milk for direct consumption is illegal in Quebec). Campylobacter can be found in raw milk [Reference Doyle and Roman33], and consumption of raw milk has often been identified as a risk factor for sporadic cases and a the source of infection in several epidemics [Reference Neimann34, Reference Greig and Ravel35]. Moreover, a source attribution study conducted in Quebec supports raw milk as a source of human infection [Reference Levesque36]. Regular raw milk consumption has been associated with elevated levels of specific serum antibodies against Campylobacter and with immunity to symptomatic infection, which could explain the higher strength of association seen in children [Reference Blaser, Sazie and Williams37]. Unfortunately, recent data on raw milk consumption in Quebec is not available to further investigate this hypothesis. Direct contact between cattle and children living on farms or children frequently visiting a farm could also be involved [Reference Doorduyn25, Reference Dilworth, Lior and Belliveau38]. Another potential pathway of transmission is through pets having access to the farming environment. In fact, dogs and cats are frequently colonized by the bacteria [Reference Hald39], and contact with pets has been reported as a risk factor for campylobacteriosis especially in young children [Reference Fullerton40].
Poultry density was associated with an increased incidence of campylobacteriosis, but only for adults aged 16–34 years and with no interaction with the seasonal period. This effect is more likely related to a professional exposure to the poultry industry, as supported by a case-control study [Reference Potter, Kaneene and Hall41] and a serological survey [Reference Price42]. Development of immunity within this particular population or a higher prevalence of poultry industry workers among younger people could explain the trend in reduction of the magnitude of the effect with age. Relative to the ruminant density, the effect of poultry density was relatively small. This could be driven by a lesser proportion of people exposed to poultry or its environment compared to ruminants.
The population density had different association with campylobacteriosis incidence according to seasonal period and age group, even after adjustment for other variables. Only in children aged ⩽4 years was an important increase in campylobacteriosis incidence rate observed as the population density decreased, and this association was limited to the summer seasonal period. This is in agreement with the higher ratio of childhood to adult infections in rural compared to urban areas observed in other studies [Reference Sibbald and Sharp43–Reference Green, Krause and Wylie45]. In young children, the inadvertent ingestion of soil following placing objects in mouth or consumption of soil present on hands or food items is maximal [Reference LaGoy46], supporting a transmission through environmental sources. These might includes summer exposure to wild-bird droppings or recreational water which are frequently positive for Campylobacter [Reference Skelly and Weinstein3]. In adults aged ⩾35 years, the association with population density was opposed to that observed for those aged <4 years and more pronounced during the winter, with higher incidence of campylobacteriosis associated with higher population densities. This observation may be explained by different food habits, social conditions, and access to travel for adults living in urban areas. According to an active surveillance programme implemented in the region of Waterloo in Canada, around 22% of reported cases of campylobacteriosis were acquired abroad, and travelling has been previously identified as an important risk factor for campylobacteriosis [Reference Ekdahl and Andersson47, Reference Ravel48].
The presence of a large poultry slaughterhouse in a municipality was associated with an increased incidence of campylobacteriosis, but only for the 16–34 years age group. This association strongly suggests a transmission restricted to occupational exposure, a factor independent of environmental conditions and seasons. One of the most likely routes of transmission for poultry workers is airborne, i.e. swallowing contaminated droplets [Reference Wilson49]. The absence of association in older people is suggestive of the development of immunity in long-term workers, as previously reported [Reference Cawthraw50]; older people could be less likely to work in slaughterhouses or might also work in less contaminated divisions within the slaughter plants. Interestingly, the proportion of cases reported as food industry workers in municipalities with large poultry slaughterhouses was 42% for the 16–34 years group and 22% for the ⩾35 years group, whereas this proportion was <3% in other municipalities. Finally, a higher incidence of campylobacteriosis was observed in children aged ⩽4 years in municipalities having slaughterhouses other than large poultry ones. The presence of other type of slaughterhouses, which includes many small plants, could be a surrogate for areas with many smaller or hobby farms that may represent a risk of transmission to young children through direct or indirect contacts. This is supported by our data, in which the number of farms per 1000 people (but not the poultry or ruminant density) was higher in municipalities with other types of slaughterhouses compared to ones with large poultry slaughterhouses (19·9 vs. 7·4 farms/1000 people, P=0·04, t test).
The incidence of campylobacteriosis was reduced in municipalities with low levels of total precipitation, with a similar effect for all age groups and seasonal periods. This is in accord with sensitivity of Campylobacter to desiccation, at least at the bacterial level [Reference Ullman and Kischkel51, Reference Sinton52]. To the best of our knowledge, only few studies have been published on the association between climatic factors and human campylobacteriosis incidence, but there has been some attention given to weather factors. In general, extreme precipitation events have been related to increased risk of waterborne outbreaks attributed to land wash-out by run-off water, which included but was not limited to campylobacteriosis [Reference Curriero53]. Other studies reported an association between the incidence of campylobacteriosis and weekly average temperature in the previous 6-week period, but no effect of rainfall [Reference Fleury54, Reference Tam55]. Therefore, our results suggest that weather and climatic factors are influencing campylobacteriosis incidence through different mechanisms.
In general, our results suggest that occupational or direct exposure to farm animals might explain a significant fraction in the variability of campylobacteriosis incidence in the municipalities of Quebec. The importance of direct exposure to animal pathways is supported by a recent source attribution study, in which 62% of the daily exposure of people to Campylobacter was attributed to direct contact with pets or farm animals [Reference Evers12]. A lower estimate for attribution of 19% was reported by a recent expert elicitation study [Reference Havelaar13]. In the surveillance database used in this study, there is a dedicated field for occupation. However, information was missing or unspecific for 94% of cases and there was no specific category for animal husbandry workers; thus, this information could not be used to explore this hypothesis. Similarly, the census data used only had broad categories for occupation, therefore it was not possible to explore the effect of those specific occupational exposures at the ecological level. Finally, a strong variation was noted in the adjusted incidence of campylobacteriosis across seasonal periods and age group, which suggests that further unmeasured factors are also involved. Interestingly, the predicted incidence and effect of the seasonal period was quite similar for patients aged ⩽4 years and 16–32 years, which might correspond to babies/toddlers and their parents, respectively, and suggests similar exposures.
Some methodological limitations should be noted for our study. First, our analysis was based on reported cases of campylobacteriosis, which represent a fraction of all cases occurring in the population [Reference Thomas56]. If the probability that a case will be reported depends on environmental characteristics, our results might be biased. However, it seems unlikely that people would have different consultation patterns or physicians would request stool samples differently across the regions or seasons, as Quebec relies on a public health system without any user fees. Similarly, we believe that the effect of environmental characteristics on the reporting rate would be the same for all age groups within a region. Indeed, any interactions between environmental characteristics and age group or season would remain valid. Another limit of our analysis is the potential dependence of results on the categories created for age groups and seasonal periods, which was not evaluated. The age groups were defined in order to present meaningful periods of life (i.e. infancy, school-age children, young adults with children at home, adults), characterized by different behaviours that could influence the risk of campylobacteriosis. Similarly, the seasonal periods were created based on differences in agricultural activities (i.e. manure spreading, pasture use) that could influence environmental contamination by Campylobacter, with adjustment for the estimated survival of the bacterium in soil following those activities [Reference Easton, Newell, Ketley and Feldman57]. Further, our study was based on aggregated data, and thus results could be influenced by the choice of the unit analysis; however, a previous analysis showed that using 11 years of aggregated data at the municipality level was a meaningful choice of unit in our context, and was associated to conservative associations with animal densities [Reference Arsenault27]. Finally, our study would have benefited from an analytical stratification by Campylobacter spp., considering that the importance of various reservoirs and risk factors differs between those species [Reference Doorduyn25], and restriction to domestically acquired cases. This was not done because more than 20% of reported cases had missing values for the species isolated and 96% for whether the case was related to out-of-province travel, and those missing numbers were not evenly distributed in space. For 93% of cases with non-missing values, Campylobacter jejuni was isolated, and so our results are probably driven by the specific epidemiology of this species.
CONCLUSION
This study strongly suggests that the environmental pathways involved in the transmission of Campylobacter to humans are dependent on age, with little seasonal variations. This indicates that different environmental factors should be targeted depending on the age stratum of the population, which should be considered in surveillance or research activities. Moreover, our results suggest that the link between animal density and campylobacteriosis is mostly through close contacts with the farming environment, which should be further investigated in order to design efficient and targeted disease control and prevention measures.
ACKNOWLEDGEMENTS
This work was made possible through doctoral research awards from the Canadian Institutes of Health Research and the Faculté de Médecine vétérinaire de l'Université de Montréal attributed to J. Arsenault. We are most grateful to the 18 provincial health units of Quebec, to the Ministère de l'Agriculture, des Pêcheries et de l'Alimentation du Québec, and to Statistics Canada for sharing their data.
DECLARATION OF INTEREST
None.