Introduction
The forest tent caterpillar, Malacosoma disstria (Hübner) (Lepidoptera: Lasiocampidae), is a periodic defoliator of broadleaved trees throughout North America (Sippell Reference Sippell1962). Trembling aspen, Populus tremuloides Michaux (Salicaceae), and sugar maple, Acer saccharum Marshall (Sapindaceae), are the primary host tree species consumed by forest tent caterpillar larvae (Witter Reference Witter1979). In eastern Canada, outbreaks generally cycle regularly, with a periodicity of 9–13 years (Cooke et al. Reference Cooke, MacQuarrie and Lorenzetti2012). Curiously, outbreaks in the prairie provinces of western Canada are thought to have occurred less frequently and less synchronously (Hildahl and Reeks Reference Hildahl and Reeks1960; Cooke and Roland Reference Cooke and Roland2018). Recurring outbreaks are conjectured to be the result of a deterministic host–parasitoid interaction that is subject to stochastic meteorological perturbations (Hodson Reference Hodson1941, Reference Hodson1977; Witter and Kulman Reference Witter and Kulman1972; Roland et al. Reference Roland, Mackey and Cooke1998; Cooke and Roland Reference Cooke and Roland, J2003; Roland Reference Roland2005); however, the duration of a given outbreak cycle appears to be determined by several factors, including climatic conditions (Daniel and Myers Reference Daniel and Myers1995) and the influence of forest landscape structure on natural enemy communities (Roland and Taylor Reference Roland and Taylor1997).
Understanding the quasiperiodic nature of forest tent caterpillar population fluctuations is important because the probability of severe forest impacts rises sharply as defoliation during long-lasting outbreaks increases from two to three or more years of heavy defoliation (Churchill et al. Reference Churchill, John, Duncan and Hodson1964). Indeed, there have been some outbreaks so intense that hundreds of thousands of hectares of host trees have died in response to prolonged defoliation (Candau et al. Reference Candau, Abt and Keatley2002). To date, nobody has asked whether there is a pattern to these forest tent caterpillar–caused aspen decline events in Canada, although recent work in Minnesota, United States of America, suggests these decline episodes do not recur periodically in time, even though outbreaks do (Cooke et al. Reference Cooke, Sturtevant and Robert2022). This is the biggest paradox in boreal forest insect pest ecology: how supposedly cycling pest populations are able to produce aperiodic patterns of forest decline (Sturtevant et al. Reference Sturtevant, Cooke and James2023).
Sutton and Tardif (Reference Sutton and Tardif2009) provided a brief and informal overview of the history of forest tent caterpillar impacts from Manitoba to New Brunswick, Canada, focusing especially on tree-ring responses. In the present paper, I review the history of the insect’s outbreak occurrence across Canada from 1938 to 2001 and evaluate its role in large-scale hardwood host–forest decline events. I examine the occurrence of host tree mortality in response to forest tent caterpillar outbreaks and, in a companion paper (Cooke Reference Cooke2024), illustrate how rising host abundance could induce a slow negative feedback loop, whereby those rising host volumes are bound to be lost to herbivory, thus stabilising the forest–pest interaction in the long run. The interaction between a fast top–down-driven population cycle and a slow bottom–up-driven forest regeneration cycle is what Holling (Reference Holling1973, Reference Holling1992) hypothesised was driving the slow–fast dynamics of spruce budworm (Choristoneura fumiferana Clemens) (Lepidoptera: Tortricidae) outbreaks and what Raffa et al. (Reference Raffa, Aukema, Bentz, Carroll, Hicke, Turner and Romme2008) hypothesised was driving the “cross-scale” dynamics of mountain pine beetle (Dendroctonus ponderosae Hopkins) (Coleoptera: Curculionidae). Here, I ask whether the same conceptual model might be driving forest tent caterpillar–caused hardwood decline in boreal North America, as first hypothesised by Rose and Harmsen (Reference Rose and Harmsen1981).
Methods
This synthesis review was conducted in two parts, the first dealing with unpublished patterns of occurrence of defoliation by forest tent caterpillar and the second dealing with published plot-based estimates of defoliation impacts. The question I sought to answer was whether outbreaks were strictly periodic in their pattern occurrence across Canada and whether published reports of large-scale host tree mortality followed this pattern.
Analysis of Forest Insect and Disease Survey defoliation records across Canada
Each year, from 1938 to 1995, the Forest Insect and Disease Survey unit of the Government of Canada mapped areas of moderate-to-severe defoliation of trembling aspen in each province using fixed-wing aircraft. The identity of the defoliator was typically confirmed using a sparse and constantly varying network of spot ground checks. Under the federal programme, aerial survey methods were identical amongst provinces; however, these survey data and practices have never been formally audited for accuracy, despite the existence of both error and bias (Cooke Reference Cooke2001) – imprecisions that can undermine the quality of data analysis when these sketch maps are used as independent predictors in local-scale analyses (Cooke and Roland Reference Cooke and Roland2023). Caveats aside, confidence in these sketch survey records arises from the degree of broad concordance between defoliation and tree-ring records from Ontario, Canada (Cooke and Roland Reference Cooke and Roland2007), and Minnesota (Cooke et al. Reference Cooke, Sturtevant and Robert2022) and from the comparison between these neighbouring jurisdictions, which operate independently of each other. Despite the independence of operational surveys, the same outbreak patterns are observed over the 20th century on either side of the international border in Ontario–Minnesota: six major outbreak cycles in the interval from 1938 to 2001, the second and fifth of which were intense and extensive, and the others of which were less so.
After 1995, survey responsibility in Canada was transferred to the appropriate provincial authority, and survey methods began to change, especially after 2001, with the advent of modern digital technologies, including global positioning systems and recording tablets. These records, up to 2001, have previously been analysed for Québec (Cooke and Lorenzetti Reference Cooke and Lorenzetti2006), for Ontario and Québec combined (Cooke et al. Reference Cooke, MacQuarrie and Lorenzetti2012), and for Alberta (Cooke and Roland Reference Cooke and Roland2018), using formal pattern analysis methods that are objective and systematic; however, the full set of interprovincial data has never been collated and considered as a whole across Canada, from coast to coast.
In 2002, these data were assembled to create separate eastern and western Canadian databases, and in 2014, these were fused into a single database with national coverage annually for 1938–2001. Rasterised to a common resolution of approximately 58 km2 per cell (the resolution of archived spatial data in Québec being the limiting factor), these national-scale data were submitted to a hierarchical cluster analysis using R package hclust, with a stopping criterion of 12 clusters, as in the manner described for the combined Québec and Ontario analysis (Cooke et al. Reference Cooke, MacQuarrie and Lorenzetti2012), in order to determine the dominant modes of variability in space and time (Kaufman and Rousseeuw Reference Kaufman and Rousseeuw2009). The goal was to determine whether these patterns would be dominated by periodic behaviour or aperiodic behaviour and whether the putative difference between western and eastern Canada stood up to formal scrutiny. All computations were done using R, version 4.2.3 (R Core Team; https://cran.r-project.org/).
Historical summary of impact studies across Canada
Federal surveyors did not systematically map aspen mortality as part of the annual exercise of defoliation mapping from 1938 to 1995. Starting in 1996, different provinces attempted to map cumulative aspen mortality, because it was being observed in parts of Alberta and Ontario. The causes of this aspen mortality were investigated independently by different researchers in separate jurisdictions. For each region of Canada, I surveyed the literature for studies of trembling aspen and sugar maple mortality that would help determine the degree to which major decline episodes were geographically associated with mapped forest tent caterpillar outbreaks. Each study was reviewed for quantitative evidence of causation. I then plotted the locations of these study areas on a national map of forest tent caterpillar activity in space and time to determine whether mortality coincided with unusually intense, frequent, or long-lasting outbreaks.
Results
Patterns of forest tent caterpillar defoliation and host forest decline in Canada
Forest tent caterpillar exhibited a semiperiodic pattern of six outbreak cycles across a 1 716 684-km2 area over the period 1938–2001, although significant spatial variations in the intensity and spatial pattern occurred with each outbreak. In fact, the area experiencing robust periodicity comprised less than 10% of the total forest tent caterpillar–defoliated area. Decadal cycles were observed only in northwestern Ontario (cluster 12), and bidecadal cycles were observed only in northwestern Alberta (cluster 10; Supplementary material, Fig. S1). The other 10 clusters, comprising more than 90% of the forest tent caterpillar-defoliated area, exhibited aperiodic fluctuations that were dominated by a single “spike” of defoliation that occurred in a relatively narrow set of years in close association with one of the primary outbreak cycles. These anomalous spikes tended to load heavily onto just one or two focal regions in the country (Table 1; Supplementary material, Fig. S1). A time-series analyst would consider any series dominated by a single spike – a single sustained eruption pulse – “nonstationary” and thus not amenable to autocorrelation analysis, which assumes homogeneity of variances and covariances.
Table 1. Summary of forest tent caterpillar cluster patterns and association with host aspen or maple decline (see Supplementary material, Fig. S1, for source data).

* Each raster cell was ∼58 km2 in area.
† First letter(s) correspond to provincial subregion (N, north; C, central; S, south; E, east; W, west), followed by two-letter provincial code (AB, Alberta; SK, Saskatchewan; MB, Manitoba; ON, Ontario; QC, Québec).
‡ Indicates whether or not host forest decline was observed in the region defoliated by forest tent caterpillar. “MN” indicates published studies of aspen decline in Minnesota at the time but no such reports in Canada.
Three of these anomalous clusters (4, 10, and 6) were directly implicated in four geographically distinct published reports of hardwood decline associated with cycles IV and V, in the 1980s and 1990s, in Québec (Bauce and Allen Reference Bauce and Allen1991), Alberta (Brandt et al. Reference Brandt, Cerezke, Mallett, Volney and Weber2003), and Ontario (Gross Reference Gross1991; Candau et al. Reference Candau, Abt and Keatley2002). Five of the anomalous clusters were not associated with any hardwood decline in Canada but were temporally associated with aspen decline episodes in Minnesota that occurred as a result of extensions of cycles II (clusters 2, 11, and 9) and III (clusters 5 and 3) from Ontario into Minnesota (Churchill et al. Reference Churchill, John, Duncan and Hodson1964; Witter et al. Reference Witter, Mattson and Kulman1975). Two of the clusters (8 and 1) were associated with extensive defoliation that occurred in 1976 and 1977 (during cycle IV) and were not affiliated with any published reports of hardwood decline. One of the clusters (12) exhibited regular cycling in a focal area in northwestern Ontario and was not associated with decline. A final cluster (7) was associated with extensive defoliation in 1940, during cycle I, and was not clearly affiliated with any authoritative, quantitative published studies of hardwood decline. However, as section 2 of this paper makes clear, Ghent (Reference Ghent1958) and Hodson (Reference Hodson1941, Reference Hodson1977) provided accounts of some aspen decline in Ontario and Minnesota at the time. In summary, pulse eruptive anomalies in 8 of the 12 clusters (2, 3, 4, 5, 6, 9, 10, and 11) could be at least indirectly tied to some form of evidence of hardwood decline. This comprises more than 70% of the total forest tent caterpillar–defoliated area. The single spot where cycling is most robust (cluster 12) – that is, where outbreaks are most frequent – appears to have suffered no decline. On the surface, it would appear that trembling aspen may be adapted to tolerate two consecutive years of defoliation every decade.
Although forest tent caterpillar exhibited semiperiodic outbreaks across Canada over the period 1938–2001, the anomalously extensive eruptive pulses occurring during each outbreak clearly dominated the spatial pattern of intensity during each outbreak interval (Fig. 1A). Several hardwood decline events were found to occur in the 20th century (Fig. 1B) in the provinces of Québec (1980s; sugar maple), Alberta (1990s; aspen), and Ontario (2000s; aspen), and these were invariably associated with the anomalously extensive pulses identified as constituting 90% of the variation evidence in cluster analysis (Table 1).

Figure 1. Total number of years of moderate-to-severe defoliation by forest tent caterpillar across Canada, 1938–2001. The top frame maps the sum number of years of defoliation, as reflected in the time-series below. The bottom frame plots the sum number of cells experiencing defoliation across a given longitude, as reflected in the map above. Also indicated in each frame, as circles, are the locations, in space and time, of six published episodes of either aspen or maple decline. Four of these (during cycles IV, V, and VI) were located in Canada. Two (during cycles II and III) were located in Minnesota, United States of America, just south of the Canadian border (see Cooke et al. Reference Cooke, Sturtevant and Robert2022). The size of these circles is arbitrary, unlinked to the extent or intensity of host decline, which was not aerially mapped in these studies but based on plot-level point observations.
In every one of these six cases of host decline, the documented episode was associated with an unusually long-lasting forest tent caterpillar outbreak, where one cycle would run into the next, with little or no reprieve between the two cycles. These decline events started at lower latitudes and steadily progressed northwards over time. Meanwhile, the extent of decline events, from Churchill et al. (Reference Churchill, John, Duncan and Hodson1964) in Minnesota to Candau et al. (Reference Candau, Abt and Keatley2002) in northern Ontario, rose over time, from less than 10 000 ha in Minnesota to more than 1 000 000 ha when the impacts in the Abitibi region of northern Ontario (Candau et al. Reference Candau, Abt and Keatley2002) and northern Québec (Charbonneau et al. Reference Charbonneau, Lorenzetti, Doyon and Mauffette2012) are summed together.
Review of forest tent caterpillar impact studies in Canada
Ontario region
Ghent (Reference Ghent1958) documented a long-standing trend in aspen mortality in northwestern Ontario but suggested that decreased mortality actually occurred during a significant forest tent caterpillar outbreak in the late 1930s. He argued that that outbreak could not have caused the decline (92% cumulative aspen mortality by 1949) because the mortality trend was initiated in 1918. However, he failed to consider what role the outbreak of 1910–1915 (Baird Reference Baird1917) might have played in bringing about either direct mortality or indirect mortality resulting from water table rise (per Witter et al. (Reference Witter, Mattson and Kulman1975) and Charbonneau et al. (Reference Charbonneau, Lorenzetti, Doyon and Mauffette2012)). Indeed, through the 1910s outbreak, a sequence of four minor growth reductions occurred in this part of Ontario, with peak reductions occurring in the years 1907, 1910, 1915, and 1920 (Ghent Reference Ghent1952). This resulted in the same sort of high-frequency cycling pattern that precipitated subsequent declines in Minnesota (Churchill et al. Reference Churchill, John, Duncan and Hodson1964; Witter et al. Reference Witter, Mattson and Kulman1975), Ontario (Candau et al. Reference Candau, Abt and Keatley2002), and Québec (Charbonneau et al. Reference Charbonneau, Lorenzetti, Doyon and Mauffette2012). Is it possible that a sequence of defoliation events from 1907 to 1920 initiated a decline that was hard to detect at first, and that the 1930s event merely added to the damage that had accumulated to that point? Specifically, had there been no forest tent caterpillar outbreak in 1930, would mortality due to the 1907–1920 events have stabilised at 40% instead of rising to its observed level of 92%? This highly plausible hypothetical example shows how it can be challenging to determine clear cause and effect when a continuous stream of mild periodic disturbances has occurred, each with lagged effects. Did Ghent underestimate the killing power of the multiple-wave outbreaks? Itter et al. (Reference Itter, D’Orangeville, Dawson, Kneeshaw, Duchesne and Finley2019) developed an ecological model of tree-ring width response that emphasises the cumulative effects of multiple interacting environmental disturbances spanning up to 10 years of accumulated time. Consequently, the presence of long lags in response time could explain Ghent’s (Reference Ghent1958) failure to attribute the 1950s decline to forest tent caterpillar.
No further aspen decline was noted in Ontario until Candau et al. (Reference Candau, Abt and Keatley2002) reported more than 200 000 ha of dead aspen through the Hearst–Kapuskasing corridor in northeastern Ontario. Although Candau et al. (Reference Candau, Abt and Keatley2002) did not conduct a full spatial analysis of factors associated with the 2000s-era decline, they surmised that the probability of decline was significantly related to the duration of tent caterpillar outbreak (Fig. 2).
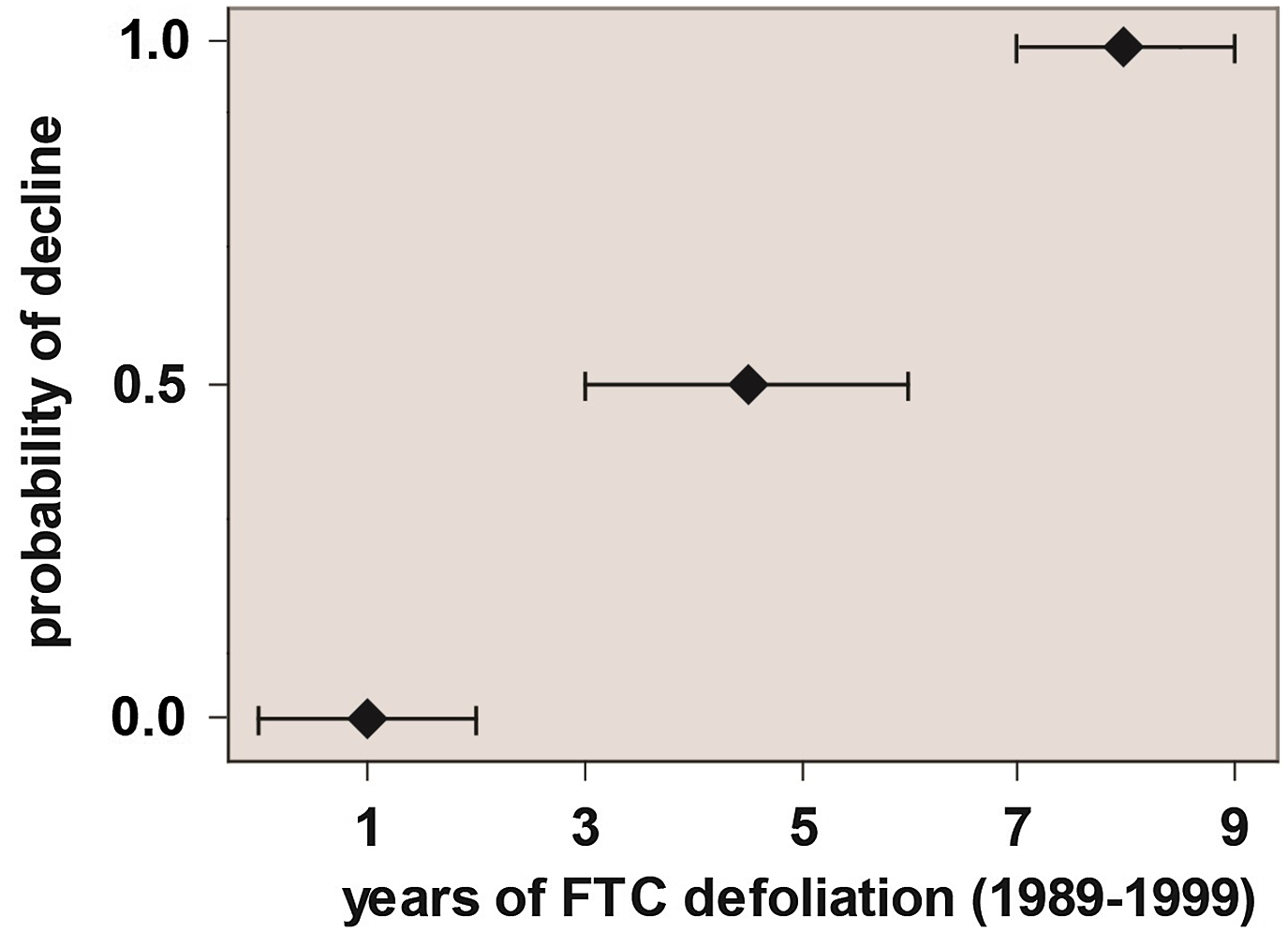
Figure 2. Probability of exhibiting overt signs of decline as a function of the number of years of defoliation during an anomalously long-lasting outbreak of forest tent caterpillar (based on data in Candau et al. Reference Candau, Abt and Keatley2002).
Man and Rice (Reference Man and Rice2010) followed Candau et al. (Reference Candau, Abt and Keatley2002) with a formal spatial analysis of the 2000s aspen decline using ground plot data. Man and Rice’s (Reference Man and Rice2010) table 2 states that each additional year of forest tent caterpillar defoliation yields an additional loss of 7% basal area. They identified the threshold between the presence and absence of large-scale decline as being between the third and fourth years of defoliation, which is consistent with Churchill et al. (Reference Churchill, John, Duncan and Hodson1964) and Candau et al. (Reference Candau, Abt and Keatley2002). This decline episode is the fifth indicated in Figure 1.
Gross (Reference Gross1991) attributed a decline of sugar maple in the early 1980s in Bruce County, Ontario, to forest tent caterpillar. In that study, regression models were developed showing that levels of dieback observed in 1978 and 1979 were most closely related to the starch content measured in sapwood in 1978, which was depressed as a result of forest tent caterpillar defoliation that had occurred through the period 1974–1978.
Québec region
Bauce and Allen (Reference Bauce and Allen1991) studied the decline of sugar maple in southeastern Québec that occurred in the early 1980s and surmised that the decline was partly a result of stand age but was accelerated by a combination of weather effects and defoliation by forest tent caterpillar. This decline episode is the third indicated in Figure 1. Consistent with Bauce and Allen (Reference Bauce and Allen1991), Payette et al. (Reference Payette, Fortin and Morneau1996), studying tree-ring patterns on healthy and declining sugar maple in the region, declared that “the double impact of insect infestations and drought likely explains the magnitude of the recent maple decline in this region.” In each case, forest tent caterpillar alone was found to be an insufficient single cause of decline because both healthy and declining trees carried a signal of significant periodic episodes of defoliation, especially in the 1910s, 1950s, and 1980s. This led to the view that the decline of sugar maple might be associated with process interactions involving xylem cavitation (from summer drought) and root breakage (from late spring thaw–freeze events), conditions that might be exacerbated by insect defoliation, which could affect either water relations, carbohydrate stores, or both.
Charbonneau et al. (Reference Charbonneau, Lorenzetti, Doyon and Mauffette2012), Moulinier et al. (Reference Moulinier, Lorenzetti and Bergeron2013), and Perrette et al. (Reference Perrette, Lorenzetti, Moulinier and Bergeron2014) studied a forest tent caterpillar outbreak in the Abitibi region of Québec in 1998–2000. Their results parallel those of Churchill et al. (Reference Churchill, John, Duncan and Hodson1964): a third year of defoliation resulted in a threefold increase in the long-term impact compared to just one to two years of defoliation (19.9% versus 6.6% stem mortality). This is an order of magnitude higher than the background levels of mortality in nominally undefoliated stands. This decline episode is the sixth indicated in Figure 1.
Not only did the impacts of the forest tent caterpillar outbreak cycle of 1998–2000 in Québec rise sharply with the number of years of defoliation but the mortality was initiated 10 years earlier, in 1991, during the previous outbreak cycle, V. The measurable impact of the second cycle, VI, was thus conditioned on the hidden effect of defoliation occurring much earlier, during cycle V. This is consistent with long response lags reported west of Québec (Itter et al. Reference Itter, D’Orangeville, Dawson, Kneeshaw, Duchesne and Finley2019), to be described in more detail later.
Prairies region
Studying 28 plots in Manitoba and Saskatchewan, Canada in 1951–1956, Hildahl and Reeks (Reference Hildahl and Reeks1960) stated unequivocally that forest tent caterpillar “defoliation causes no appreciable mortality.” Their data, however, indicate that levels of mortality double between the lowest and highest defoliation impact categories (4.9% versus 8.3% for small trees and 2.5% versus 4.2% for large trees). With 28 plots, these differences might have been declared statistically significant had a proper statistical analysis been conducted on the raw data.
Kulman (Reference Kulman1971) signalled his agreement with Hildahl and Reeks (Reference Hildahl and Reeks1960), even though their data indicate that tree mortality was weakly related to forest tent caterpillar incidence. A significant observation here is the pattern of forest tent caterpillar defoliation during the study period. A significant pattern of asynchronous behaviour amongst outbreak pulses occurred, the result of which is to give defoliated trees a significant reprieve between successive outbreaks. The lower impact of forest tent caterpillar on the prairies through the 1950s is not surprising because very rarely did outbreaks in this region last longer than three years.
Forty years later, studying forest tent caterpillar impacts in Alberta, Saskatchewan, and Manitoba, Brandt et al. (Reference Brandt, Cerezke, Mallett, Volney and Weber2003) used a linear model to show that each additional year of forest tent caterpillar defoliation should lead to an additional 3.2% aspen stem mortality. This decline episode is the fourth indicated in Figure 1. A nonlinear response model might perform better and would be consistent with the nonlinear responses revealed in the Ontario and Québec studies. A second limitation in Brandt et al.’s (Reference Brandt, Cerezke, Mallett, Volney and Weber2003) study is that their analysis did not focus on impacts as a consequence of a specific outbreak. Regardless, the conclusions are clear: forest tent caterpillar is capable of inducing large-scale decline when severe defoliation exceeds three years. The mortality response to defoliation is both nonlinear and lagged.
It is worth noting that there are instances in the Prairies region where large-scale aspen mortality has been reported in the driest parts of the southern margin of the boreal forest (Michaelian et al. Reference Michaelian, Hogg, Hall and Arsenault2011). These are places that had not been defoliated by insects for 20 years (clusters 4 and 7; Supplementary material, Fig. S1), suggesting the primary cause was moisture stress. It is therefore necessary to discuss the more recent quantitative science on the relative roles of these factors in western Canada.
Chen et al. (Reference Chen, Huang, Alam, Zhai, Dawson, Stadt and Comeau2017) demonstrated that drought has a significant impact on aspen basal area reduction in Alberta, in accordance with other studies (Hogg and Schwarz Reference Hogg and Schwarz1999; Brandt et al. Reference Brandt, Cerezke, Mallett, Volney and Weber2003). Chen et al. (Reference Chen, Huang, Dawson, Zhai, Stadt, Comeau and Whitehouse2018) revised the 2017 analysis to include forest tent caterpillar as a cofactor, reporting that the caterpillar does not contribute as much to basal area reductions as drought does. The follow-up study has several serious limitations, however – from its basic design of scattered (i.e., dispersed, not clustered) sample plot locations to the simplistic causal model to estimate insect impact. As Cooke and Roland (Reference Cooke and Roland2007) demonstrated, the national Forest Insect and Disease Survey data that they relied on are imprecise, with significant biases in space and time and in the density domain. Cooke et al. (Reference Cooke, Sturtevant and Robert2022) also illustrated, in Minnesota, that forest tent caterpillar population fluctuations that rise to 50% defoliation create a measurable impact on tree growth; however, these partial outbreaks are not detectable by fixed-wing aerial survey. Spatial autocorrelation in forest tent caterpillar population dynamics (Cooke and Roland Reference Cooke and Roland2000) also makes it nearly impossible to find a sharp contrast between sites that have been defoliated versus those that have not been defoliated. It is internally inconsistent logic to assume, in one instance, that pest impacts are synchronous enough that sparse sampling is sufficient and, in another instance, that impacts are asynchronous enough that good contrasts can readily be found between areas defoliated and undefoliated.
Finally, Chen et al. (Reference Chen, Huang, Dawson, Zhai, Stadt, Comeau and Whitehouse2018) discussed the potential positive influence of defoliation on forest growth without evidence, citing instead how such positive effects have been reported, unsurprisingly, in nonclonal systems. In contrast, Itter et al. (Reference Itter, D’Orangeville, Dawson, Kneeshaw, Duchesne and Finley2019) illustrated how the negative cumulative effects of such a hidden enemy on basal area increment may linger for as long as 10 years, suggesting the Chen et al. (Reference Chen, Huang, Dawson, Zhai, Stadt, Comeau and Whitehouse2018) causal model may be biased against detecting enduring defoliator impacts.
Discussion
Historical review of impact in a modern context
Kulman (Reference Kulman1971), in a first comprehensive review, cited six studies (Dils and Day Reference Dils and Day1950; Batzer Reference Batzer1955; Duncan and Hodson Reference Duncan and Hodson1958; Ghent Reference Ghent1958; Rose Reference Rose1958; Hildahl and Reeks Reference Hildahl and Reeks1960) that purported to show that forest tent caterpillar had no impact on tree mortality. However, Kulman (Reference Kulman1971) emphasised the later study by Churchill et al. (Reference Churchill, John, Duncan and Hodson1964) that shows that forest tent caterpillar incidence is strongly and positively correlated with inexplicable mortality arising from cause “unknown” a decade after the passage of the same forest tent caterpillar outbreak studied by Batzer (Reference Batzer1955) and by Duncan and Hodson (Reference Duncan and Hodson1958). Kulman (Reference Kulman1971) failed to note the nonlinear relationship with crown dieback reported by Batzer (Reference Batzer1955) and its close association with the pattern of whole-stem mortality reported by Churchill et al. (Reference Churchill, John, Duncan and Hodson1964), which is unfortunate because this is what explains the “unknown” mortality. In neither Batzer (Reference Batzer1955) nor Churchill et al. (Reference Churchill, John, Duncan and Hodson1964) were graphs or statistics used to determine whether the mortality response to defoliation might be nonlinear or lagged; in both cases, data tables of means were presented. The “unknown” mortality reported in Churchill et al. (Reference Churchill, John, Duncan and Hodson1964) is almost certainly caused by a nonlinear response to forest tent caterpillar defoliation. What is “unknown” are the cellular and molecular physiological details of how defoliation leads to death.
Kulman’s (Reference Kulman1971) citation of Dils and Day (Reference Dils and Day1950) was questionable because they reported on growth loss over the growing season and did not investigate longer-term effects such as cumulative mortality. Kulman’s (Reference Kulman1971) citations of Ghent (Reference Ghent1958) in Ontario and Hildahl and Reeks (Reference Hildahl and Reeks1960) in Manitoba and Saskatchewan as evidence that forest tent caterpillar does not kill aspen also need to be revisited because those studies’ results do not support this interpretation. What their data instead suggest are that (1) the defoliation needs to surpass a certain threshold before the damage is detectable and (2) it may take a few years postoutbreak before the evidence of impact is undeniable. I conclude that, in hindsight, Kulman (Reference Kulman1971) was wrong about the impact of forest tent caterpillar on aspen mortality. He underestimated the nonlinear and lagged response of trees to defoliation and therefore could not have foreseen the scale of decline that has been observed in more recent decades.
In Reference Hall and Moody1994, Hall and Moody, of the Canadian Forest Insect and Disease Surveys unit, reported that: “Forest tent caterpillar damages a wide variety of hardwoods – aspens, oaks, maples, birches, and elms. It causes substantial losses in growth, but little mortality” (Hall and Moody Reference Hall and Moody1994). Summarising forest tent caterpillar impacts through the period 1982–1987, Hall and Moody (Reference Hall and Moody1994) emphasised the negligible rates of mortality in every region of the country. Unfortunately, 1982–1987 is a period that occurs between cycles III and IV, where only Alberta was experiencing significant defoliation at the time (Hall and Moody Reference Hall and Moody1994, table 8; Supplementary material, Fig. S1, clusters 7 and 10). As we now know, the first signs of forest tent caterpillar–caused mortality were only just beginning at that time in Alberta (Brandt et al. Reference Brandt, Cerezke, Mallett, Volney and Weber2003) and they would not begin in Ontario and Québec until the end of cycle V, in about 1991–1993 (Man and Rice Reference Man and Rice2010; Moulinier et al. Reference Moulinier, Lorenzetti and Bergeron2013). The signs would not become a serious concern until the publication of Candau et al. (Reference Candau, Abt and Keatley2002). It is essential to read the optimistic 1994 report in this context. Like Kulman (Reference Kulman1971), Hall and Moody (Reference Hall and Moody1994) did not think that forest tent caterpillar might kill aspen over hundreds of thousands of hectares.
Lingering scepticism about the impact of forest tent caterpillar on its host trees can be found as recently as 2000. Thompson (Reference Thompson2000, p. 47) stated that [italics are the emphasis of the author of the current paper]:
Forest tent caterpillar appears to erupt on a 10- to 11-year cycle. Recent major infestations took place in Ontario in 1965, 1976, and 1987, but outbreaks are recorded as long ago as 1834 (Churcher and Howse 1989). Sugar maple, trembling aspen, and oaks are the preferred hosts of this insect. Trees are rarely killed, and new leaves are produced five to six weeks following defoliation. Loss of leaves may confer a competitive advantage to semi shade–tolerant species in the understory [sic], such as spruces and red maple; however, no Ontario studies of succession following outbreaks of forest tent caterpillar have been conducted, so the ecological effects on stand and landscape structure are not known. Roland (1993) suggests that the amount of edge is important to the success of outbreaks, and that logging in boreal forests may be exacerbating rates of infestation. Forest tent caterpillar is not a major force in landscape-level changes in forest species composition and structure in Ontario.
It may be true that large-scale host decline events have been “rare” historically, but it is also true that they have been occurring regularly in Ontario and beyond for all of recorded history, whenever outbreaks last longer than three years. As explained by Cooke et al. (Reference Cooke, Lorenzetti and Roland2009), this does not occur often in Ontario or Québec – perhaps only 5% of the area that is defoliated during an outbreak – but it does happen, and it happens regularly whenever two outbreak cycles occur in rapid succession and the foci are located near enough to one another; a zone of overlap is affected by both outbreak cycles. Although this seems to happen infrequently, it appears to precipitate a host decline every time it happens (Fig. 1). Whether this, in turn, has implications for forest succession towards nonhost cover types (conifers or shrubs) would require study in those conditions where it happens. If, during any given 10-year outbreak cycle, 5% of a forest experiences stand-replacing disturbance that leads to succession favouring nonhost species, then it might take only 20 cycles, or 200 years, for an entire forest to be disrupted by forest tent caterpillar. This throws into question the validity of Thompson’s (Reference Thompson2000) assertion that “forest tent caterpillar is not a major force in landscape-level changes in forest species composition and structure in Ontario.” Not only is forest tent caterpillar capable of killing trees over large areas, but strong support exists for a nonlinear response of aspen mortality to forest tent caterpillar defoliation, with the response strengthening significantly after a third and fourth year of defoliation at the stand and landscape scales. Volume losses may triple from approximately 10% to approximately 30% in a single year alone as one moves from two to three years of outbreak. I therefore contend that a lack of data exists on the long-term consequences of defoliation events lasting longer than three years. In light of the large-scale failure of aspen regeneration in northeastern Ontario following the decline of mature aspen associated with prolonged forest tent caterpillar outbreak (Man and Rice Reference Man and Rice2010), Thompson’s (Reference Thompson2000) remarks seem premature and, indeed, incorrect.
Thompson’s (Reference Thompson2000) remark aligns with Ghent (Reference Ghent1958); however, given what is now known, with full hindsight, to have occurred in the 1950s, 1980s, and 2000s, the most likely cause of the 1930s aspen decline in northwestern Ontario that Ghent (Reference Ghent1958) discussed was the forest tent caterpillar outbreak during the 1910s (Baird Reference Baird1917).
More recently, Schowalter (Reference Schowalter2017) wrote that “outbreaks can strip preferred trees of all foliage and cause substantial branch mortality and growth reduction but generally does not cause much tree mortality, at least not directly.” However, as early as 2002, it was clear that forest tent caterpillar was directly causing much mortality in northeastern Ontario. As the analysis here shows, these large-scale decline events extend back for as long as records have been kept (Table 1). Finally, it is worth noting that the decline events discussed in detail here in the interval 1938–2001 are complemented by additional observations of hardwood decline south of Canada. The two aspen decline events in Minnesota that occurred within this interval have already been discussed and are mapped in Figure 1. A more recent sugar maple decline also occurred beyond this interval, in New York and Vermont, United States of America, and was unambiguously attributed to an outbreak of forest tent caterpillar (cycle VI) in 2002–2007 (Wood et al. Reference Wood, Yanai, Allen and Wilmot2009).
The Border Lakes study
Robert et al. (Reference Robert, Sturtevant, Kneeshaw, James, Fortin and Wolter2020) studied cycling populations of forest tent caterpillar in the Minnesota–Ontario border region and showed that the amount of aspen in the landscape influenced the pest’s propensity for high-amplitude, synchronous cycling. Cooke et al. (Reference Cooke, Sturtevant and Robert2022) showed that high-amplitude cycling of outbreaks in Minnesota was most detrimental to aspen when it occurred in proximity to additional asynchronous pulses of defoliation, which happened in two different locations in the 1950s (Churchill et al. Reference Churchill, John, Duncan and Hodson1964) and 1960s (Witter et al. Reference Witter, Mattson and Kulman1975). These are the first and second decline episodes of the six indicated in Figure 1.
We do not know why outbreak cycles II and III in the 1950s and 1960s resulted in such anomalous durations, extents, and impacts in Minnesota. But what is clear now is that these questions need answers: Were the unexpected durations, extents, and impacts a result of anomalously warm weather favouring the defoliator and disfavouring its natural enemies? Were they a result of an overabundance of host trees? Or were both weather and host tree abundance both operating together? These questions and their answers are considered in a companion piece (Cooke Reference Cooke2024).
Cycles and anomalies revisited: reconciling periodic occurrence versus aperiodic impact
The record of forest tent caterpillar defoliation in Canada for the period 1938–2001 indicates that the sort of anomalies that produced unprecedented host forest declines in northeastern and north–central Minnesota did not stop recurring after outbreak cycles II and III: they continued on through cycles IV and V but in Québec, Alberta, and Ontario, not Minnesota. (Cycle VI, peaking in 2002–2004, was just starting and was not complete at the time these data were collated.) The fact that these anomalies spike so infrequently and do not recur periodically suggests that the cause may be affiliated with a slow process. One possible cause is climate warming, which might produce a latitudinal sequence of infrequent spike defoliation events as critical thermal thresholds are breached from south to north over time. A second possibility is forest conversion from precolonial conifers to postcolonial hardwoods via mechanised clearcutting. These possibilities are not mutually exclusive. Any theory of eruptive cycling must accommodate both possibilities and their joint action.
If these singular anomalies that dominate the historical record are so infrequent, why do they not disrupt the overall pattern of cycling evident in Figure 1? The answer is that the anomalies, though extensive – averaging 153 187 km2 in area – always coincide temporally with decadal cycle peaks of more modest intensity. Also, they invariably shift spatially from one part of the insect’s range during one cycle to a different area the next cycle so that they end up strengthening, rather than weakening, the aggregate pattern of cycling. Due to this skipping behaviour, the anomalies do not accumulate more strongly in any one part of the insect’s range; instead, they are distributed evenly across the country, just as the spikes are distributed evenly through time (Table 1).
The complexity in spatial patterning shown by forest tent caterpillar outbreaks is reminiscent of similar complexity by spruce budworm outbreaks, as discussed in Kneeshaw et al. (Reference Kneeshaw, Cooke, Work, Sturtevant, De Grandpré, Pureswaran and MacLean2015), who suggested that all of the basic patterns conceived of by Berryman (Reference Berryman1986) as applying to different systems are evidenced in the singular system. It is simply a matter of spatial and temporal scale whether Berryman’s “cyclic,” “eruptive,” “pulse,” “gradient,” or “sustained” patterns of occurrence and recurrence feature more prominently in the data under consideration. Forest tent caterpillar “cycles,” however, are locally amplified at times, in the form of “sustained” “eruptive” “pulses.” Spatially, these outbreaks take the form of a “gradient” (Fig. 1) that is shaped by climate and forest conditions (Cooke and Roland Reference Cooke and Roland2000, Reference Cooke and Roland2007). Roland (Reference Roland1993) showed there are places where these eruptive pulses are “sustained” for longer periods of time, and we see this also across Canada, as shown in Figure 1. Indeed, sustained eruptive pulses and deviation from synchronous cycling explain the occurrence of large-scale host hardwood decline in Canada.
Please see the present article’s companion review (Cooke Reference Cooke2024), which focuses on a model of the landscape-scale effect of bottom–up forces on a top–down-driven eruption cycle, for a discussion about what determines whether populations exhibit infrequent sustained eruptive pulses versus stable cycling.
I note that simple models of population cycling (e.g., Cobbold et al. Reference Cobbold, Lewis, Lutscher and Roland2005; Roland Reference Roland2005; Cooke et al. Reference Cooke, MacQuarrie and Lorenzetti2012) are incapable of addressing the only question that matters to foresters: when and where are major insect-caused forest decline events likely to occur? The issue that occupies the focus and energy of so much of academic theoretical ecology – the origins of synchronous population cycling – is therefore not relevant to economic forest entomology in Canada.
Conclusions
Over the period 1938–2001, forest tent caterpillar outbreaks in aggregate have generally recurred periodically across Canada but with considerable variation in the pattern of recurrence. The outbreaks occur somewhat synchronously nationwide, although outbreaks west of Manitoba have alternated between light and severe intensity. More importantly, during each cycle, there has always been one location, somewhere in Canada, where the outbreak cycle is so extensive and so long-lasting that it bleeds into the next cycle. These locations have often experienced two waves of outbreak in rapid succession, and this is where host forests (either trembling aspen or, in eastern Canada, sugar maple) are observed to decline nonlinearly in response to abnormally long-lasting outbreaks of three or more years instead of the normal one or two years. Notably, there have been no published reports of aspen decline in the only area where forest tent caterpillar cycles most stably: the Dryden district of northwestern Ontario. The most significant declines occur where the cycling dynamics are least stable. The independent impact studies done during these decline episodes consistently identify forest tent caterpillar as a primary cause of decline but always in association with abiotic stresses, such as drought. The different studies diverge in their assessments of the relative impacts of each agent, and this may be an unavoidable result of differing contexts. However, the studies agree that the mortality response to insect defoliation is both nonlinear and lagged. This means we might expect serious impacts only when critical response thresholds are breached, and that impacts need to be monitored long after the inciting outbreak is over.
How exactly host forest landscape structure regulates outbreak behaviour, including the amplitude and synchrony of cycling and the transition to travelling waves of eruption, is unclear, but both relationships clearly are relevant. To what degree climate warming is responsible for the northward shift in areas experiencing insect-caused hardwood decline is equally unclear. Do nonstationarities in host forest landscape structure and climate each contribute to disequilibrium cycling? The answers to these questions lie beyond the scope of the pattern analysis presented here; however, it is a subject that may be addressed through comprehensive analytical simulation modelling. In the companion paper (Cooke Reference Cooke2024) to this article, I show how forest tent caterpillar dynamics may be jointly regulated by a synergistic interaction between fast top–down and slow bottom–up forces, putting this species squarely in the class of Canadian forest insects that exhibit so-called “cross-scale” dynamics.
Supplementary material
To view supplementary material for this article, please visit https://doi.org/10.4039/tce.2024.4.
Acknowledgements
The Hovmoller Figure 1 was prepared with the assistance of Dr. Alex Chubaty, FOR-CAST Research & Analytics. Thanks are owed to Rory McIntosh, Saskatchewan Environment, for reviewing an early draft. Chris MacQuarrie and Amanda Roe, Canadian Forest Service, helped improve the structure of the manuscript. Thanks also to three anonymous reviewers for their helpful suggestions.