Conjugated linoleic acid (CLA) consists of a group of positional and geometrical isomers of octadecadienoic acid. Dietary CLA is usually a mixture of various CLA isomers and has received increasing attention during the last decades due to suggested health benefits in rodents, including reduced risk of cancer, atherosclerosis, obesity and diabetes, and stimulation of the immune system(Reference Wahle, Heys and Rotondo1). Ruminant milk and meat are the major natural sources of CLA, and the CLA content is stable under normal cooking and storage conditions(Reference Dhiman, Nam and Ure2). In milk and meat from ruminants, the most prevalent isomer of CLA is cis-9, trans-11-CLA followed by trans-7, cis-9-CLA, and only minor amounts of trans-10, cis-12-CLA are found(Reference Yurawecz, Roach and Sehat3, Reference Sehat, Kramer and Mossoba4). This is in contrast with synthetically prepared CLA isomer mixtures, which often contains mainly cis-9, trans-11-CLA and trans-10, cis-12-CLA(Reference Dhiman, Nam and Ure2). Recent evidence suggests that the health effects of CLA relate to the type of isomer(Reference Evans, Brown and McIntosh5–Reference Tricon, Burdge and Kew7), and especially the isomers cis-9, trans-11-CLA and trans-10, cis-12-CLA are believed to be responsible for the biological properties attributed to CLA mixtures, where trans-10, cis-12-CLA may play a role in the regulation of body weight and glucose homeostasis(Reference Park, Storkson and Albright8–Reference Henriksen, Teachey and Taylor11).
The ability of trans-10, cis-12-CLA to reduce adipose tissue mass has been linked to increased lipolysis and fatty acid oxidation(Reference Park, Albright and Storkson12, Reference Evans, Lin and Odle13), increased energy expenditure(Reference West, Delany and Camet14), decreased lipogenesis(Reference Brown, Halvorsen and Lea-Currie15) and reduced adipogenesis(Reference Kang, Liu and Albright16) as well as increased apoptosis(Reference Hargrave, Li and Meyer10). Enhanced energy expenditure in response to dietary CLA does not seem to be associated with changes in the expression of uncoupling protein (UCP)-1 in brown adipose tissue(Reference West, Blohm and Truett17), but increased expression of UCP-2 have been observed in white adipose tissue (WAT) of animals fed CLA(Reference Ryder, Portocarrero and Song9, Reference Tsuboyama-Kasaoka, Takahashi and Tanemura18). At the present time there are only a few studies on isomer-specific effects of CLA on UCP(Reference Rodriguez, Ribot and Palou6, Reference Choi, Jung and Park19).
It has been demonstrated that glucose tolerance is improved in animal models of insulin resistance such as obese Zucker rats(Reference Henriksen, Teachey and Taylor11) and Zucker diabetic fatty rats(Reference Ryder, Portocarrero and Song9) after trans-10, cis-12 feeding, but increased insulin resistance after dietary supplementation with this CLA isomer has been reported in normal and obese mice(Reference Tsuboyama-Kasaoka, Takahashi and Tanemura18, Reference Roche, Noone and Sewter20) and humans(Reference Riserus, Arner and Brismar21). The adipokines resistin and adiponectin have been studied due to their potential roles in regulating insulin resistance and glucose homeostasis also in obese subjects(Reference Steppan and Lazar22–Reference Li, Yu and Pan26). Adiponectin enhances fatty acid oxidation in muscle and inhibits hepatic gluconeogenesis, promoting reduced plasma levels of NEFA, TAG and glucose resulting in improved insulin sensitivity(Reference Berg, Combs and Du27–Reference Holst and Grimaldi29), whereas elevated plasma resistin levels may cause insulin resistance(Reference Steppan, Bailey and Bhat25).
The aim of the present study was to study the response to a specific CLA isomer (trans-10, cis-12-CLA) in WAT and liver by measuring fatty acid β-oxidation and synthesis, and to study the effect of this isomer on the expression of genes involved in the regulation of lipid metabolism and energy balance. In addition, we wanted to characterise the effects of trans-10, cis-12-CLA on glucose homeostasis and study whether these effects are related to the modulation of adiponectin and resistin gene expression. In the present study we used obese Zucker fa/fa rats, which have a null mutation of the leptin receptor gene and have been extensively studied as a model for obesity and diabetes in humans.
Materials and methods
Animals and diets
Male obese Zucker Crl:(ZUC)/FaBR (fa/fa) rats from Charles River Laboratories (Sulzfeld, Germany), aged 5 weeks, were divided into two experimental groups of six rats each with comparable mean body weight. The rats were housed individually in metabolism cages in a room maintained at a 12 h light–dark cycle (light from 07.00 to 19.00 hours) and a constant temperature of 20 ± 3°C and relative humidity of 65 ± 15 %. The rats were acclimatised under these conditions before the start of the experiment.
The rats were fed diets containing 20 % (by weight) casein sodium salt from bovine milk (C-8654; Sigma-Aldrich Norway AS, Oslo, Norway). To the diets were added 10 % (by weight) commercial soya oil (control group) or 9 % (by weight) commercial soya oil and 1 % (by weight) trans-10, cis-12-CLA (97·15 % non-esterified trans-10, cis-12-CLA, kindly provided by Natural ASA, Hovdebygda, Norway) (trans-10, cis-12-CLA group). To all diets were added 1 % of AIN-93VX Vitamin mixture (Dyets Inc., Bethlehem, PA, USA), 3 % of AIN-93G Mineral mixture (Dyets Inc.) and 0·2 % choline hydrogen tartrate (Merck, Darmstadt, Germany). Table 1 gives an overview of the experimental diets. The rats were fed these diets for 10 d. All rats had free access to tap water and feed (ad libitum).
Table 1 Composition of the experimental diets (g/kg diet)

CLA, conjugated linoleic acid.
* Fatty acid composition of the soya oil (mean of two measurements, deviation less than 3 %) (g/100 g fat): 18 : 2n-6, 55·9; 18 : 1n-9, 21·4; 16 : 0, 11·4; 18 : 3n-3, 5·8; 18 : 0, 3·3; 18 : 1n-7, 1·6.
† Trans-10, cis-12-CLA contained 97·15 % non-esterified trans-10, cis-12-CLA (Natural ASA, Hovdebygda, Norway).
‡ Casein (g/kg diet): fat, 9·8; ash, 31. The casein protein contained 91·9 % crude protein.
§ AIN-93VX; Dyets Inc., Bethlehem, PA, USA.
∥ AIN-93G; Dyets Inc., Bethlehem, PA, USA.
¶ Choline hydrogen tartrate (Merck, Darmstadt, Germany).
At the end of the feeding period, under non-fasting conditions, the rats were anaesthetised with a 1:1 mixture of Hypnorm™ (fentanyl citrate (0·315 mg/ml) and fluanisone (10 mg/ml); Janssen Pharmaceutica, Beerse, Belgium) and Dormicum® (midazolam (5 mg/ml); F. Hoffmann-La Roche AG, Basel, Switzerland) injected subcutaneously. Blood was drawn directly from the heart using a syringe containing heparin. Epididymal WAT (eWAT), visceral retroperitoneal WAT (rWAT) and subcutaneous inguinal WAT (iWAT) and liver were dissected out and weighed.
Plasma, WAT depots and a small piece of liver were frozen in liquid N2 and stored at − 85°C until analysis. The rest of the liver was kept on ice for subcellular fractionation and further analysis later the same day. The protocol was approved by the Norwegian State Board of Biological Experiments with Living Animals.
In vitro assays
Homogenisation and subcellular fractionation of the livers were performed as previously described(Reference Berge, Flatmark and Osmundsen30). Rat livers were homogenised individually in ice-cold sucrose solution (0·25 m-sucrose in 10 mm-HEPES buffer (pH 7·4) and 1 mm-EDTA) using a Potter-Elvehjem homogeniser. The procedures were performed at 0–4°C. The fractions were either used immediately for measurements of mitochondrial β-oxidation and carnitine palmitoyltransferase (CPT)-I activities, or stored at − 85°C for subsequent analysis. Protein was measured with the BioRad protein assay kit (BioRad, Richmond, CA, USA) using bovine serum albumin as the standard. The enzymic activities were analysed using two identical parallels for each sample, using the assays described below.
Palmitoyl-CoA (without or with 12 μm-malonyl-CoA added before the start of the reaction) and palmitoyl-l-carnitine oxidation were measured in the mitochondrial fraction as acid-soluble products(Reference Willumsen, Hexeberg and Skorve31). The assay medium contained 12 mm-HEPES buffer (pH 7·3), 11 mm-MgC12, 12 mm-dithiothreitol, 5·6 mm-ADP, 0·2 mm-NAD+, 0·6 mm-EDTA, 60 mm-KCl, antimycin A (1 pg/ml) and 1·0–1·2 mg protein from the mitochondrial fraction. Palmitoyl-l-carnitine oxidation was measured with 80 μm-[1-14C]palmitoyl-l-carnitine and the palmitoyl-CoA oxidation was measured with 40 pm-palmitoyl-CoA supplemented with 1 mm-l-carnitine. Cyanide-sensitive fatty acid oxidation was measured in the presence of 1 mm-KCN. After incubation for 120–240 s at 30°C, oxidation was stopped by the addition of 25 μl fatty acid-free bovine serum albumin (100 mg/ml) followed by 150 μl 1·5 m-KOH and 500 μl 4 m-HClO4. After centrifugation, the protein- and fatty acid-free supernatant fraction was assayed for radioactivity using a WinSpectral™ 1414 Liquid Scintillator Counter spectrometer (PerkinElmer (formerly Wallac), Waltham, MA, USA).
CPT-I and CPT-II activities were measured in the mitochondrial fraction(Reference Madsen, Froyland and Dyroy32). The assay for CPT-I contained 20 mm-HEPES, 70 mm-KCl, 5 mm-KCN, 100 μm-palmitoyl-CoA, 10 mg bovine serum albumin/ml and 180 μg hepatic tissue protein/ml, and pH was adjusted to 7·5. The reaction was started by adding 200 μm-[methyl-14C]-l-carnitine (200 cpm/nmol). When included, malonyl-CoA was added before the start of the reaction. Assay conditions for CPT-II were identical, except that bovine serum albumin was omitted and 0·01 % Triton X-100 was included. The radioactivity was determined using the WinSpectral™ 1414 Liquid Scintillator Counter spectrometer (Wallac).
Malonyl-CoA decarboxylase activity was measured in the liver post-nuclear fraction(Reference Alam and Saggerson33). A reaction mixture containing 0·1 m-2-amino-2-hydroxymethyl-propane-1,3-diol (Tris)-HCl buffer (pH 8·0), 0·5 mm-dithiothreitol, 10 mm-l-malate, 0·5 mm-NAD+, 40 μm-rotenone and 1·4 μg malate dehydrogenase (1·7 units) was pre-incubated for 7 min at 37°C after which 10 μg citrate synthase was added. After 1 min the reaction was initiated by the addition of 0·3 mm-malonyl-CoA and about 35 μg or 170 μg frozen/thawed mitochondrial or cytosolic fraction protein respectively before citrate synthase was assayed using a Varian 2300 spectrophotometer (Varian Inc., Palo Alto, CA, USA).
Fatty acyl-CoA oxidase activity was measured in the peroxisomal fraction by the coupled assay described by Small et al. (Reference Small, Burdett and Connock34). The spectrophotometric assay of acyl-CoA oxidase was based on the determination of H2O2 production, which was coupled to the oxidation of leuco-dichlorofluorescein in a reaction catalysed by exogenous peroxidase. The production of H2O2 was measured by monitoring the increase in dichlorofluorescein absorbance in the presence of palmitoyl-CoA using a Varian 2300 spectrophotometer.
3-Hydroxy-3-methylglutaryl (HMG)-CoA synthase was measured spectrophotometrically in the mitochondrial fraction(Reference Clinkenbeard, Reed and Mooney35) in a reaction mixture containing 20 pmol Tris-HCl buffer (pH 8·0), 20 nmol EDTA, 40 nmol [l- or 2-14C]acetyl-CoA (2 × 106 cpm/μmol), 10 nmol acetoacetyl-CoA, and from 0·1 to 1·0 milliunits of HMG-CoA synthase. The reaction was initiated by the addition of [14C]acetyl-CoA to the reaction mixture. Samples were transferred to glass vials containing 0·1 ml of 6 m-HCl, and the acidified sample was dried. Water and liquid scintillator were added and non-volatile 14C activity (as [14C]HMG-CoA plus 3-[14C]methylglutaconyl-CoA) was determined using the WinSpectral™ 1414 Liquid Scintillator Counter spectrometer (Wallac).
Acetyl-CoA carboxylase activity was determined in the cytosolic fraction by measuring the amount of NaH14CO3 incorporated into malonyl-CoA(Reference Tanabe, Nakanishi and Hashimoto36). The pre-incubated enzyme was added to an assay mixture containing 50 mm-Tris-HCl buffer (pH 7·5), 10 mm-potassium citrate, 10 mm-MgCl2, 3·75 mm-glutathione, 0·75 mg bovine serum albumin per ml, 3·75 mm-ATP, 0·125 mm-acetyl-CoA and 12·5 mm-NaH14CO3. After incubation for 10 min at 37°C, the reaction was terminated with 0·2 ml 5 m-HCl. After centrifugation for 10 min at 1500 g, a sample of the supernatant fraction was dried in a counting vial, and OptiPhase HiSafe 3 (Perkin Elmer) scintillator solution was added before the radioactivity was determined using the WinSpectral™ 1414 Liquid Scintillator Counter spectrometer (Wallac).
Fatty acid synthase activity was measured in the cytosolic fraction(Reference Roncari37) and modified according to Skorve et al. (Reference Skorve, al-Shurbaji and Asiedu38). The assay mix contained 100 μmol potassium phosphate, 3 μmol Na2EDTA, 19 nmol acetyl-CoA and 40 nmol [14C]acetyl-CoA. The reaction was started by adding 300 μm-NADPH and pre-incubated enzyme fraction (100 μg proteins) and run for 6 min at 37°C. The reaction was stopped by adding 30 μl 60 % perchloric acid, and the mixture was diluted with ethanol. Fatty acids were extracted with petroleum ether and the residues were dissolved in pentane. Samples were counted by using the WinSpectral™ 1414 Liquid Scintillator Counter spectrometer (Wallac).
Glycerol-3-phosphate acyl transferase activity was assayed at 30°C in the mitochondrial fraction(Reference Bates and Saggerson39), in an assay mix containing 120 mm-KCl, 50 mm-Tris-HCl buffer (pH 7·4), l-[U-14C]glycerol 3-phosphate, 65 μm-palmitoyl CoA, 0·7 mm-dithiothreitol, l-glycerol 3-phosphate and 6 mg bovine serum albumin. The reaction was initiated with 0·1 ml of mitochondrial fraction and performed for 3 min. The reaction was terminated with 2 ml water-saturated butanol and radioactive incorporation into butanol-soluble products before counting at the WinSpectral™ 1414 Liquid Scintillator Counter spectrometer (Wallac).
Malonyl-co-enzyme-A
The malonyl-CoA concentration in liver was measured by reversed-phase HPLC(Reference Wergedahl, Liaset and Gudbrandsen40). In brief, frozen liver was homogenised in ice-cold 1·4 m-HClO4 and 2 mm-d-dithiothreitol to obtain 10 % (w/v) homogenate, and centrifuged at 12 000 g for 1 min. Then 122 μl ice-cold 3 m-K2CO3 with 0·5 m-triethanolamine was added to 500 μl of the supernatant fraction. After 10 min on ice, the solution was centrifuged at 12 000 g for 1 min at 4°C. Then 40 μl of the supernatant fraction was injected onto the HPLC column.
Lipids and fatty acid composition
Plasma and liver lipids were measured enzymically on the Technicon Axon system (Miles, Tarrytown, NY, USA) using the following kits: TAG kit (Bayer, Tarrytown, NY, USA), total cholesterol (Bayer), NEFA (NEFA C; Wako Chemicals, Dalton, OH, USA) and phospholipids (PAP 150; bioMérieux, Lyon, France). The hepatic lipids were extracted using a mixture of chloroform and methanol(Reference Bligh and Dyer41), and the samples were evaporated under N2 and re-dissolved in isopropanol before analysis.
The lipids were extracted from the liver samples using chloroform and methanol(Reference Bligh and Dyer41). The samples were added 0·2 m-KOH in ethanol and incubated at 40°C for 90 min in order to obtain NEFA. Water was added to the samples before they were washed twice with hexane. To the samples was added 6 m-HCl, and the fatty acids were extracted by hexane and transferred to new tubes. After evaporation, 14 % BF3–methanol was added, and the samples were allowed to react for 30 min at room temperature to obtain methyl esters before the mixture was neutralised with 2 % KHCO3. The methyl esters were extracted by hexane before they were analysed on a GC8000 Top gas chromatograph (Thermo Fisher (formerly Carlo Erba Instruments), Milan, Italy), equipped with a flame ionisation detector and a capillary column coated with a highly polar SP2340 phase (Supelco Inc., Bellefonte, PA, USA). The methyl esters were identified by comparison with known standards (Larodan Fine Chemicals, Malmø, Sweden). Quantification of the fatty acids was performed using the Chrom-Card A/D 1·0 chromatography station (Thermo Fisher) by comparison with an internal standard (heneicosanoic acid).
Glucose in plasma
Plasma glucose was measured enzymically on the Technicon Axon system (Miles, Tarrytown, NY, USA) using the Gluco-quant kit (Roche, Mannheim, Germany).
Oil red O staining of liver
In order to study neutral fat deposits, 10 μm frozen sections of the livers were cut on a Leitz cryostat 1720 (Leitz, Wetzlar, Germany) and collected on uncoated glass slides. The sections were fixed in 10 % formaldehyde solution diluted in PBS at room temperature for 10 min, then rinsed three times in distilled water. The sections were stained with oil red O (Sigma, St Louis, MO, USA) for 10 min at room temperature, then rinsed three times in distilled water and dipped in 60 % ethyl alcohol to remove excess stain. The sections were then rinsed three times in distilled water, counter-stained with haematoxylin for 10 min, rinsed three times in distilled water and sealed with cover slips.
Microphotographs were taken with a Leica DM6000B light microscope with digital camera (Leica Microsystems, Heerbrugg, Switzerland). The area of liver for each measurement was 1 180 000 μm2. To analyse the whole liver lobule we chose one image with the central vein in the centre, and another image was centred on the portal tract for each rat. The quantification of the lipid droplets was performed using the analySIS soft imaging system to measure the area of positive oil red O staining. Images were coded for blinding.
Plasma leptin, adiponectin and insulin
Leptin, adiponectin and insulin in plasma were quantified by competitive RIA (Linco Research Inc., St Charles, MO, USA).
RNA isolation
Total RNA was isolated from 200–300 mg eWAT, iWAT and rWAT or 50 mg liver using TRIzol reagent (Life Technologies, Norway) and according to the manufacturer's instructions.
Reverse transcriptase PCR analysis
To quantify the mRNA levels of CPT-I in liver as well as liver isoform L-CPT-I and adiponectin in different WAT depots, a semi-quantitative RT-PCR assay was developed, using the housekeeping gene β-actin as an internal control(Reference Ribot, Felipe and Bonet42). In brief, 0·5 μg total RNA was denatured at 65°C for 10 min and reversely transcribed in the presence of 50 pmol of random primers, using MuLV RT (Perkin-Elmer, Madrid, Spain) at 42°C for 30 min in a Perkin-Elmer 9700 Thermal Cycler. After the reaction, the RT medium was added to a PCR mix containing Taq DNA polymerase (Promega, Lyon, France) and an appropriate amount of specific pairs of primers. The reaction mixture was first heated to 95°C for 2 min to denature the cDNA. This was followed by twenty-two to twenty-eight cycles of denaturation at 95°C for 15 s, annealing at 56–58°C for 15 s and extension at 72°C for 30 s, with an additional extension at 72°C for 7 min after the last cycle. Sequences of the sense and antisense oligonucleotides were as follows: 5′-GCT CGC ACA TTA CAA GGA CAT-3′ and 5′-TGG ACA CCA CAT AGA GGC AG-3′ for L-CPT-I; 5′-GCT CAG GAT GCT ACT GTT G-3′ and 5′-TCT CAC CCT TAG GAC CAA G-3′ for adiponectin; 5′-ACG GGC ATT GTG ATG GAC TC-3′ and 5′-GTG GTG GTG AAG CTG TAG CC-3′ for β-actin. These primers were derived from the sequences of the corresponding genes and cDNA. Amplification products had the expected sizes of 268, 241 and 160 bp for CPT-I, adiponectin and β-actin, respectively. The PCR products were separated in 2 % agarose gel (MS-8; Pronadisa, Madrid, Spain) in 0·5 × Tris-borate EDTA buffer, stained with ethidium bromide and visualised by an image recording system (Gelprinter, TDI, Madrid, Spain). The densities of the target bands were then quantified using an image processing and analysing program (ID Image Analysis, Kodak, Rochester, NY, USA).
Northern blot analysis
Total RNA (25 μg) from liver or WAT were denatured with formamide–formaldehyde, resolved by agarose gel electrophoresis, transferred to a Hybond nylon membrane and fixed with UV light(Reference Jacobsson, Stadler and Glotzer43). UCP2 mRNA, leptin mRNA, resistin mRNA and 18S rRNA as internal control, were analysed sequentially on the same membrane, in the above-mentioned order, by a chemiluminescence procedure based on the use of synthetic mouse-specific antisense oligonucleotide probes end-labelled with digoxigenin, essentially as in the protocols provided by Roche (Barcelona, Spain). The technique was first described by Trayhurn et al. in 1994(Reference Trayhurn, Duncan and Nestor44). In short, fixed membranes were pre-hybridised at 42°C for 1 h in DIG-Easy Hyb™, and then hybridised with the corresponding oligonucleotide probe in DIG-Easy Hyb™ at 42°C overnight. The following probes were used: UCP2, 5′-GGC AGA GTT CAT GTA TCT CGT CTT GAC CAC-3′; leptin, 5′-GGT CTG AGG CAG GGA GCA GCT CTT GGA GAA GGC-3′; resistin, 5′-TCC CAC GAG CCA CAG GCA GAG CCA CAG GAG CAGC-3′; 18S rRNA, 5′-CGC CTG CTG CCT TCC TTG GAT GTG GTA GCC G-3′. The membranes were blocked and incubated first with an anti-digoxigenin-alkaline phosphatase conjugate and then with the chemiluminescent substrate CDP-Star™. Finally, membranes were exposed to Hyperfilm ECL™ (Amersham, Barcelona, Spain). Bands in films were analysed by scanner photodensitometry and quantified using the ID Image Analysis software (Kodak). Stripping in between analysis was performed by exposing the membranes to boiling 0·1 % SDS.
Statistical analysis
All data in tables and figures are presented as mean values with their standard errors. The body weights of the rats fed CLA or control diets were compared using a non-parametric test (Mann–Whitney rank). All other results from trans-10, cis-12-CLA-fed rats and rats fed the control diet were compared pair-wise using a two-sample variance Student's t test (two-tailed distribution), with level of statistical significance set at P < 0·05.
Results
Growth, liver weight and white adipose tissue depot weights
The body weight before the experiment started (day 0) was similar between the rats receiving the 1 % trans-10, cis-12-CLA-supplemented diet or the control diet (127 (sem 14) and 116 (sem 10) g, respectively) (Fig. 1(a)). No significant differences in the body weights were seen between the feeding groups after 10 d (Fig. 1(a)). No significant effects were seen on the relative weight of liver or eWAT, rWAT and iWAT depots (Table 2). Also, no significant changes were seen in the absolute weights of these WAT depots (data not shown).
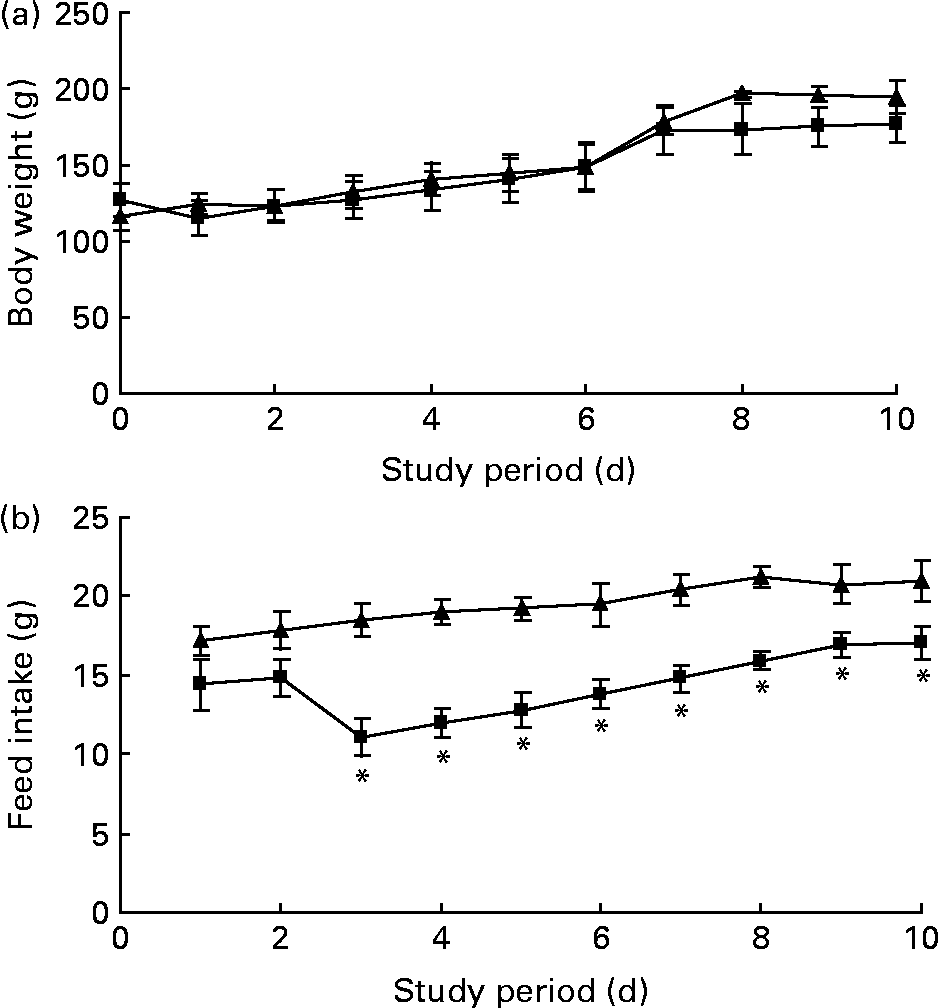
Fig. 1 Body weight (a) and feed intake (b) curves for obese Zucker rats fed a control diet (–▲–) or a diet containing 1 % trans-10, cis-12-conjugated linoleic acid (–■–) for 10 d. Values are means, with standard errors represented by vertical bars (n 6). * Mean value was significantly different from that of the control group (P < 0·05).
Table 2 Liver weight and weight of epididymal white adipose tissue (eWAT), inguinal white adipose tissue (iWAT) and retroperitoneal white adipose tissue (rWAT) in obese Zucker fa/fa rats after 10 d feeding control or trans-10, cis-12-conjugated linoleic acid (CLA)*
(Mean values with their standard errors for six rats per group)

* Liver and WAT weights are expressed as a percentage of the body weight (hepatic index and adiposity).
Feed intake was similar in rats fed the trans-10, cis-12-CLA or control diets for the first 2 d of the experiment, but feed intake was markedly lower for the rest of the experiment for the trans-10, cis-12 CLA-fed rats (Fig. 1(b)).
Liver lipids and hepatic fatty acid metabolism
To investigate whether trans-10, cis-12-CLA could affect hepatic lipid metabolism after only 10 d feeding, we stained the liver pieces from all rats with oil red O and measured the content of neutral lipids. To analyse the whole liver lobule we chose one image with the portal tract in the centre (Fig. 2), and another centred on the central vein (data not shown). The lipid droplets in livers from rats fed trans-10, cis-12-CLA were smaller as compared with control livers, and the total content of neutral lipids was markedly lower (Fig. 2). The hepatic TAG level was markedly decreased after administration of trans-10, cis-12-CLA (Fig. 2), but no changes were observed for the levels of cholesterol, phospholipids and NEFA (data not shown).

Fig. 2 Microphotographs of livers with a portal field in the centre stained with oil red O from obese Zucker rats fed a control diet (a) or a diet containing 1 % trans-10, cis-12-conjugated linoleic acid (CLA) for 10 d (b), showing one representative picture from each rat. Original magnification × 50. The mean relative lipid amount (from staining) for the trans-10, cis-12-CLA group (9·3 (sem 0·7)) was significantly different from that of the control group (26·8 (sem 2·8) (P < 0·02)). The mean liver TAG content for the trans-10, cis-12-CLA group (237 (sem 19) μmol/g liver) was significantly different from that of the control group (320 (sem 23) μmol/g liver) (P < 0·02).
To gain insight to the mechanism by which trans-10, cis-12-CLA decreased the level of hepatic TAG, we measured the capacity of mitochondrial and peroxisomal β-oxidation as well as lipogenesis and TAG biosynthesis in the liver. Mitochondrial β-oxidation, measured by using palmitoyl-CoA or palmitoyl-l-carnitine as substrates, was markedly higher in the liver from rats fed trans-10, cis-12-CLA (Figs. 3(a) and (b), respectively). This was accompanied by increased activities of CPT-I (Fig. 3(c)), the rate-limiting enzyme involved in the mitochondrial uptake of fatty acids, and of CPT-II (Fig. 3(d)). The expression of CPT-I mRNA was also increased in liver after trans-10, cis-12-CLA feeding (Fig. 3(e)). In addition, hepatic activity of mitochondrial HMG-CoA synthase, the rate-limiting enzyme in ketogenesis, was increased in rats fed trans-10, cis-12-CLA (Fig. 3(f)).

Fig. 3 The activity of mitochondrial β-oxidation using palmitoyl-CoA (a) or palmitoyl-l-carnitine (b) as substrate, the mitochondrial activities of carnitine palmitoyltransferase (CPT)-I (c) and CPT-II (d), the mRNA level of liver CPT-I (L-CPT-I) (e), the mitochondrial activity of 3-hydroxy-3-methylglutaryl (HMG)-CoA synthase (f), the malonyl-CoA sensitivity of palmitoyl-CoA oxidation (g), the malonyl-CoA concentration (h), the activity of malonyl-CoA decarboxylase (i) and the peroxisomal activity of fatty acyl CoA oxidase (j) in livers of obese Zucker rats fed a control diet or a diet containing 1 % trans-10, cis-12-conjugated linoleic acid for 10 d. Expression levels of CPT-I mRNA were analysed by RT-PCR and normalised to β-actin mRNA levels after densitometric analysis and expressed as a percentage of the control value. Values are means, with standard errors represented by vertical bars (n 6). * Mean value was significantly different from that of the control group (P < 0·05).
The sensitivity of palmitoyl-CoA oxidation towards malonyl-CoA was decreased after trans-10, cis-12-CLA feeding (Fig. 3(g)). In addition, the hepatic malonyl-CoA level was increased after trans-10, cis-12-CLA feeding (Fig. 3(h)), concomitant with a reduced activity of malonyl-CoA decarboxylase in liver (Fig. 3(i)).
Peroxisomal fatty acid oxidation in liver seemed also to be enhanced by trans-10, cis-12-CLA, as the hepatic activity of peroxisomal fatty acyl-CoA oxidase was increased more than twice in these rats (Fig. 3(j)).
Hepatic lipogenesis and TAG biosynthesis seemed to be unaffected by trans-10, cis-12-CLA feeding, as no changes were seen in mitochondrial and cytosolic acetyl-CoA carboxylase activity, in cytosolic fatty acid synthase activity, and in mitochondrial glycerol-3-phosphate acyl transferase in liver (data not shown).
Hepatic fatty acid composition
Analysis of the liver lipids revealed that trans-10, cis-12-CLA was recovered in rats fed trans-10, cis-12-CLA, and comprised 0·45 % of the fatty acids in the liver, whereas trans-10, cis-12-CLA was not found in livers from the control rats (Table 3).
Table 3 Selected fatty acids (wt%) in livers from obese Zucker fa/fa rats after 10 d administration of control feed or 1 % trans-10, cis-12-conjugated linoleic acid (CLA)
(Mean values with their standard errors for six rats per group)

ND, not detected.
*Mean value was significantly different from that of the control group (P < 0·05; Student's t test).
† 20 : 4n-6:18 : 2n-6 ratio.
‡ (20 : 5n-3+22 : 6n-3):18 : 3n-3 ratio.
The total amount of SFA was not altered after trans-10, cis-12-CLA feeding, although the amount of 18 : 0 was nearly doubled (Table 3). The total amount of MUFA was significantly decreased in rats fed trans-10, cis-12-CLA, due to decreased amounts of the Δ9 desaturated fatty acids 16 : 1n-7 and 18 : 1n-9, thereby resulting in decreased 16 : 1n-7:16 : 0 and 18 : 1n-9:18 : 0 ratios (Table 3). The hepatic total level of PUFAs was increased after administration of trans-10, cis-12-CLA, mainly due to an increase in n-3 PUFA, but the levels of 20 : 4n-6, 22 : 4n-6 and 22 : 5n-6 were also increased (Table 3). The n-3 PUFA:n-6 PUFA, 20 : 4n-6:18 : 2n-6 and (20 : 5n-3+22 : 6n-3):18 : 3n-3 ratios in the liver were increased after trans-10, cis-12-CLA feeding (Table 3).
Uncoupling protein-2 and l-carnitine-palmitoyltransferase-I mRNA levels in white adipose tissue depots
To investigate whether trans-10, cis-12-CLA could affect mitochondrial fatty acid β-oxidation and uncoupling in adipose tissue, the mRNA levels of the liver isoform of CPT-I (L-CPT-I) and UCP-2 in different WAT depots were measured. After trans-10, cis-12-CLA feeding, the mRNA level of UCP-2 was increased in eWAT and iWAT when compared with controls, but no change was seen in rWAT (Fig. 4). However, the L-CPT-I mRNA level in eWAT and iWAT was unaffected by trans-10, cis-12-CLA feeding, whereas the gene expression of L-CPT-I was increased in rWAT (Fig. 4).

Fig. 4 The mRNA levels of uncoupling protein (UCP)-2 and liver carnitine palmitoyltransferase (L-CPT)-I in epididymal white adipose tissue (eWAT) (a), inguinal white adipose tissue (iWAT) (b) and retroperitoneal white adipose tissue (rWAT) (c) from obese Zucker rats fed a control diet (□) or a diet containing 1 % trans-10, cis-12-conjugated linoleic acid (■) for 10 d. Expression levels of the specific mRNA were analysed by Northern blotting (UCP-2) or RT-PCR (L-CPT-I) and normalised to 18S rRNA (Northern blotting) levels or β-actin mRNA (RT-PCR) levels after densitometric analysis and expressed as a percentage of the control value. Values are means, with standard errors represented by vertical bars (n 6). * Mean value was significantly different from that of the control group (P < 0·05).
White adipose tissue mRNA and plasma levels of adipokines
Although dietary trans-10, cis-12-CLA did not significantly affect the adipose tissue weights in the present experiment, it was of interest to evaluate whether the gene expressions of adipokines in WAT were affected, and whether the circulating amounts of cytokines were affected. Trans-10, cis-12-CLA increased the resistin mRNA levels in all white adipose depots studied (Fig. 5). In contrast, significantly lower mRNA levels of adiponectin were found in iWAT and rWAT, but not in eWAT, after trans-10, cis-12-CLA feeding (Fig. 5). Interestingly, trans-10, cis-12-CLA supplementation significantly reduced leptin mRNA levels in all WAT depots studied (Fig. 5), although no changes in leptin and adiponectin plasma concentrations were observed after trans-10, cis-12-CLA feeding (Table 4).

Fig. 5 The mRNA levels of resistin, adiponectin and leptin in epididymal white adipose tissue (eWAT) (a), inguinal white adipose tissue (iWAT) (b) and retroperitoneal white adipose tissue (rWAT) (c) from obese Zucker rats fed a control diet (□) or a diet containing 1 % trans-10, cis-12-conjugated linoleic acid (■) for 10 d. Expression levels of the specific mRNA were analysed by Northern blotting (resistin and leptin) or RT-PCR (adiponectin) and normalised to 18S rRNA (Northern blotting) or β-actin mRNA (RT-PCR) after densitometric analysis and finally expressed as a percentage of the control value. Values are means, with standard errors represented by vertical bars (n 6). * Mean value was significantly different from that of the control group (P < 0·05).
Table 4 Leptin, adiponectin, glucose, insulin, C-peptide and lipids in plasma from obese Zucker fa/fa rats after 10 d administration of control feed or 1 % trans-10, cis-12-conjugated linoleic acid (CLA)
(Mean values with their standard errors for six rats per group)

*Mean value was significantly different from that of the control group (P < 0·05; Student's t test).
Plasma levels of lipids, glucose, insulin and C-peptide
The plasma TAG level was markedly higher in rats fed trans-10, cis-12-CLA when compared with controls, whereas no significant differences were observed in plasma levels of NEFA, cholesterol and phospholipids (Table 4). Administration of trans-10, cis-12-CLA did not significantly change the plasma levels of glucose, insulin and C-peptide (Table 4).
Discussion
The liver has been reported to be an important target for the effects of CLA on lipid metabolism. However, several published studies provide inconsistent results when comparing the effects of feeding different CLA isomers to different animal species. In an effort to penetrate these issues further, we examined the metabolic effects on liver and different adipose depots after feeding trans-10, cis-12-CLA since this isomer has been postulated to be the main mediator of CLA effects on body weight, adiposity and insulin resistance(Reference Park, Storkson and Albright8–Reference Henriksen, Teachey and Taylor11). The data obtained indicated an inverse regulation by this isomer on hepatic and plasma TAG levels, and also an inverse regulation of adiponectin and resistin gene expression in several WAT depots.
In the present study, we found that trans-10, cis-12-CLA feeding reduced the hepatic TAG content. This finding is in contrast to several published studies showing that trans-10, cis-12-CLA feeding increases the hepatic TAG content in rats(Reference Sanders, Teachey and Ptock45) and mice(Reference Clement, Poirier and Niot46–Reference Warren, Simon and Bartolini49). However, studies in rat models of obesity and insulin resistance have repeatedly demonstrated that feeding a CLA mixture containing mainly cis-9, trans-11- and trans-10, cis-12-CLA markedly reduces the TAG content in the liver of such rats(Reference Rahman, Huda and Uddin50–Reference Noto, Zahradka and Yurkova53). It has been debated whether trans-10, cis-12 feeding affects lipid metabolism, and although very few studies are performed using the pure trans-10, cis-12-CLA isomer, it has been reported that this CLA isomer increases the activities of CPT-I and CPT-II in mouse liver(Reference Degrace, Demizieux and Gresti54), suggesting that fatty acid oxidation is increased. This is in line with the significant increase in mitochondrial β-oxidation, as well as increased activities of both CPT-I and CPT-II in liver and the increased hepatic mRNA level of CPT-I observed in the present study. The increased mitochondrial β-oxidation after trans-10, cis-12-CLA feeding was accompanied by an enhanced hepatic amount of malonyl-CoA, probably caused by the decreased activity of malonyl-CoA decarboxylase. Malonyl-CoA is a strong inhibitor of CPT-I and it seems that the increased mitochondrial β-oxidation observed in the present experiment could be a consequence of a lower sensitivity towards malonyl-CoA, since palmitoyl-CoA oxidation was less affected by malonyl-CoA inhibition after trans-10, cis-12-CLA feeding as compared with controls. Ketogenesis also appears to be increased, as the mitochondrial activity of HMG-CoA synthase was increased by trans-10, cis-12-CLA. In addition, the increased hepatic peroxisomal acyl-CoA oxidase activity suggested a higher capacity for oxidation of fatty acids in liver peroxisomes after trans-10, cis-12-CLA feeding. The increased hepatic fatty acid oxidation may be mediated via PPARα, since trans-10, cis-12-CLA is an activator of PPARα(Reference Moya-Camarena, Vanden Heuvel and Blanchard55, Reference Belury, Moya-Camarena and Lu56), although the relative liver weight was unchanged in the present experiment. However, the unchanged activities of the mitochondrial and cytosolic acetyl-CoA carboxylase activity, the cytosolic fatty acid synthase activity, and the mitochondrial glycerol-3-phosphate acyl transferase in the liver after trans-10, cis-12-CLA feeding implied that the reduced hepatic TAG content was not caused by decreased biosynthesis of fatty acids or TAG. Thus, dietary trans-10, cis-12-CLA seems to improve fatty liver in obese Zucker fa/fa rats by increasing the hepatic capacity for fatty acid β-oxidation.
The reduced 18 : 1n-9:18 : 0 and 16 : 1n-7:16 : 0 ratios in liver after trans-10, cis-12-CLA feeding suggested that the activity of stearoyl-CoA desaturase was decreased. This is in accordance with studies in liver microsomes from mice(Reference Park, Storkson and Ntambi57) and rats(Reference Bretillon, Chardigny and Gregoire58) showing that trans-10, cis-12-CLA inhibits the stearoyl-CoA desaturase activity. Studies in stearoyl-CoA desaturase-deficient mice show that they have a lower hepatic TAG content compared with wild-type mice, despite normal activity of glycerol phosphate acyltransferase(Reference Miyazaki, Kim and Gray-Keller59), an enzyme taking part in TAG synthesis. This is in line with the finding that glycerol phosphate acyltransferase activity was unchanged by trans-10, cis-12-CLA in the present study. In addition, since the activity of stearoyl-CoA desaturase is correlated with the secretion of TAG from hepatocytes(Reference Legrand, Catheline and Fichot60), trans-10, cis-12-CLA may improve fatty liver by reducing the stearoyl-CoA desaturase activity as proposed by Sebedio et al. (Reference Sebedio, Angioni and Chardigny61). Thus, a reduction in the stearoyl-CoA desaturase activity could, at least partially, explain the reduced hepatic content of TAG from rats fed trans-10, cis-12-CLA.
Feeding rats trans-10, cis-12-CLA also affected hepatic PUFA, as the n-3:n-6, 20 : 4n-6:18 : 2n-6 and (20 : 5n-3+20 : 6n-3):18 : 3n-3 ratios were increased. These are important findings since it has been shown that these ratios are reduced in the liver of patients with hepatic steatosis(Reference Videla, Rodrigo and Araya62). The increased product:precursor ratio of the n-3 and n-6 PUFA cascades suggests that the hepatic activities of Δ5 desaturase, Δ6 desaturase and elongase may have been enhanced by trans-10, cis-12-CLA feeding. The increased product:precursor ratios and the increased n-3:n-6 ratio favour β-oxidation over the synthesis of fatty acids and TAG, since long-chain PUFA, especially the n-3 PUFA, are activators of PPARα(Reference Clarke63), and thus suggest that trans-10, cis-12-CLA may increase the hepatic β-oxidation via activation of PPARα.
The decreased hepatic TAG level in the trans-10, cis-12-CLA group could be attributed to increased fatty acid oxidation in the liver. However, trans-10, cis-12-CLA feeding increased the plasma TAG level when compared with controls, in spite of the reduced TAG content in liver. These results suggest that there may be an imbalance between the hepatic TAG production and secretion, and the peripheral clearance by lipoprotein lipase. It has been reported that trans-10, cis-12-CLA inhibits lipoprotein lipase activity in cultured 3T3-L1 adipocytes(Reference Lin, Kreeft and Schuurbiers64) and in mouse adipose tissue(Reference Xu, Storkson and Kim65). Thus, the clearance of plasma lipoproteins could be reduced in Zucker fa/fa rats in response to trans-10, cis-12-CLA feeding. In contrast to TAG, the plasma levels of NEFA, cholesterol and phospholipids in rats fed trans-10, cis-12-CLA were not statistically different from the control group. Hence, no hypolipidaemic effect was observed in our experiment in response to trans-10, cis-12-CLA, in agreement with some(Reference Roche, Noone and Sewter20) but in contrast to other(Reference Henriksen, Teachey and Taylor11) reports, which reflects the controversy that exists concerning the specific effects of trans-10, cis-12-CLA.
We observed increased UCP-2 mRNA levels in response to trans-10, cis-12-CLA in some, but not all WAT depots. Although the precise role of UCP-2 in the regulation of energy expenditure is still unclear, our and others' findings(Reference Ryder, Portocarrero and Song9, Reference Tsuboyama-Kasaoka, Takahashi and Tanemura18, Reference Roche, Noone and Sewter20) suggest that increased UCP-2 levels may be one of the mechanisms by which trans-10, cis-12-CLA could reduce adiposity and body weight. We found a significant increase in L-CPT-I mRNA levels in rWAT, possibly mediated via PPARα(Reference Moya-Camarena, Vanden Heuvel and Blanchard55, Reference Belury, Moya-Camarena and Lu56), but not in eWAT and iWAT. The UCP-2 mRNA level was increased in eWAT and iWAT, but was not affected in rWAT after trans-10, cis-12-CLA feeding, which may suggest that UCP-2 and CPT-I are regulatory coupled in the control of fatty acid oxidation. The enhanced potential for fatty acid oxidation in these adipose depots was not accompanied with a decrease in WAT mass, and a longer period of dietary supplementation with this isomer may be required to obtain a statistically significant decrease in adipose tissue mass.
In the present study, trans-10, cis-12-CLA feeding reduced the leptin mRNA levels in all white adipose depots studied, especially in visceral depots (eWAT and rWAT), confirming previous results obtained in brown adipose tissue(Reference Rodriguez, Ribot and Palou6). The reduced leptin mRNA levels were not a consequence of reduced adiposity, which was not statistically significant in our experiment, but could be due to a direct effect of trans-10, cis-12-CLA on gene expression, as suggested by Yamasaki et al. (Reference Yamasaki, Ikeda and Oji66). However, since trans-10, cis-12-CLA is demonstrated to bind and activate PPARγ(Reference Belury, Moya-Camarena and Lu56), and PPARγ activators are demonstrated to reduce the gene expression of leptin in rodents(Reference De Vos, Lefebvre and Miller67), the reduced leptin mRNA level in WAT may be due to the stimulation of PPARγ. Despite the reduction of leptin mRNA levels in adipose tissues, no significant change was found in plasma leptin levels in trans-10, cis-12-CLA-fed rats when compared with controls.
It has been reported that a mixture of CLA isomers improves glucose tolerance and insulin resistance in rodents(Reference Ryder, Portocarrero and Song9, Reference Nagao, Inoue and Wang68), but this has been difficult to reproduce in human studies(Reference Riserus, Arner and Brismar21). Elevated plasma resistin levels may cause insulin resistance(Reference Steppan, Bailey and Bhat25), whereas an increased adiponectin level promotes fatty acid oxidation in muscle and inhibits hepatic gluconeogenesis, resulting in reduced plasma concentrations of NEFA, TAG and glucose(Reference Berg, Combs and Du27–Reference Holst and Grimaldi29). Our data showed no effect of trans-10, cis-12-CLA on plasma concentrations of glucose and insulin, but an increased resistin mRNA level was found in all WAT depots studied whereas the adiponectin mRNA level was reduced in the same depots except for eWAT. This effect on adiponectin is consistent with the reduced adiponectin mRNA level found in rWAT from mice in response to trans-10, cis-12-CLA feeding(Reference Warren, Simon and Bartolini49). Nevertheless, plasma adiponectin levels were not significantly affected in our study, which can be due to the short period of supplementation in the present experiment (10 d). Studies in mice reveal that a marked reduction in the circulating levels of adiponectin and leptin could precede and induce a peripheral insulin resistance and hyperinsulinaemia after supplementation with trans-10, cis-12-CLA(Reference Poirier, Rouault and Clement69). It has been proposed that CLA-induced insulin resistance could be due, at least in part, to leptin depletion, because leptin infusion in CLA-fed mice may restore leptin and insulin plasma levels(Reference Tsuboyama-Kasaoka, Takahashi and Tanemura18). Thus, although we did not measure insulin sensitivity directly, our data on the expression of adipokines after feeding trans-10, cis-12-CLA in WAT are consistent with impaired insulin sensitivity reported by others(Reference Tsuboyama-Kasaoka, Takahashi and Tanemura18, Reference Roche, Noone and Sewter20, Reference Riserus, Arner and Brismar21).
Feed intake was lower in rats fed the diet containing 1 % trans-10, cis-12-CLA, but the reported metabolic effects in the present experiment are probably not solely due to the lower intake. Since the feed intake was similar for the trans-10, cis-12-CLA group and the control group during the first 2 d of the experiment, trans-10, cis-12-CLA probably did not affect feed taste but might instead induce an effect on appetite or energy requirement after a few days. Food deprivation may increase hepatic β-oxidation(Reference Boyd, Albright and Foster70) by reducing the malonyl-CoA concentration(Reference McGarry71) and inhibit fatty acid synthesis(Reference Boyd, Albright and Foster70). In the present experiment, however, trans-10, cis-12-CLA feeding enhanced the level of malonyl-CoA and increased β-oxidation in the liver, the latter probably due to a reduced malonyl-CoA sensitivity of CPT-I. In addition, the activities of rate-limiting enzymes involved in fatty acid synthesis were not affected by trans-10, cis-12-CLA. However, due to the reduced feed intake among rats fed trans-10, cis-12-CLA it is not possible to distinguish the effects of reduced energy supply and metabolic changes due to the intake of trans-10, cis-12-CLA. Thus, some of the effects of CLA feeding might be due to low energy intake among the rats fed trans-10, cis-12-CLA as compared with the controls. However, no difference was seen in body weight between the trans-10, cis-12-CLA-fed rats and the control rats. In addition, no differences were seen at the level of adipose tissue depot mass (adiposity) between the groups, corroborating with the unchanged plasma levels of NEFA. A lack of effect of CLA in reducing adiposity was previously reported in obese male Zucker rats fed a CLA mixture for 5 weeks(Reference Sisk, Hausman and Martin72), although Henriksen et al. found a decreased body weight after 21 d treatment of obese female Zucker rats with trans-10, cis-12-CLA(Reference Henriksen, Teachey and Taylor11).
Little is known about the effect of the trans-10, cis-12 isomer of CLA on O2 consumption, and CO2 and heat production in obese Zucker rats. However, studies on female Sprague–Dawley rats fed a mixture of CLA isomers (mainly cis-9, trans-11- and trans-10, cis-12-CLA) for 7 d showed no effect on feed intake, growth, O2 consumption, CO2 or heat production(Reference Azain, Hausman and Sisk73). A study with mice fed a mixture of cis-9, trans-11- and trans-10, cis-12-CLA, on the other hand, showed a reduced energy intake and growth rate, and an increased metabolic rate and decreased night-time respiratory quotient (RQ), with no overall effect on RQ measured over 24 h(Reference West, Delany and Camet14). The effect of the trans-10, cis-12 isomer of CLA on the RQ deserves further investigation to learn more about the relationship between energy intake and the effects on lipid metabolism after feeding this isomer.
In summary, the present results provide new evidence suggesting that dietary supplementation with trans-10, cis-12-CLA may change the expression of key genes involved in lipid metabolism in adipose tissues as well as key enzymes of fatty acid oxidation in the liver of Zucker fa/fa rats, resulting in an increased catabolism of lipids. The liver TAG content was reduced without improving overall adiposity, but rather by increasing fatty acid oxidation and reducing the malonyl-CoA sensitivity of CPT-I. Moreover, our data suggest that this CLA isomer modulates the expression of resistin and adiponectin inversely in adipose tissues. The reduced level of leptin mRNA and the increased level of resistin mRNA in WAT also suggest that insulin sensitivity was impaired after trans-10, cis-12-CLA feeding. Hence, the present results suggest that trans-10, cis-12-CLA may have some beneficial effects on lipid metabolism but possibly reduces insulin sensitivity. This aspect raises the importance of more detailed studies to evaluate the effects of trans-10, cis-12-CLA and the safety of using CLA for weight management.
Acknowledgements
The present study was supported by the Spanish Government (SAF 2001-4859E, BFI 2000-0988-C06 and FIS 01-1379), by the European Union (QLK1-2001-00183) and by the Norwegian Research Council.
E. R. was the recipient of a doctoral fellowship from the Spanish Government (Ministerio de Educación, Cultura y Deportes).
O. A. G., E. R., H. W., J. S., A. P. and R. K. B. designed the study. O. A. G., E. R. and H. W. performed the animal experiment, and analysed and interpreted the data. S. M. performed the oil red O staining of the livers and J. E. R. performed the analysis of the plasma leptin, adiponectin and insulin levels. A. P. and R. K. B. supervised the experiment, obtained funding and provided administrative, technical, and material support. O. A. G. and E. R. wrote the draft of the manuscript; all authors critically reviewed the manuscript. O. A. G. and E. R contributed equally to the present study.
We declare that there are no conflicts of interest regarding this paper.