Many in vitro animal and human studies have shown that water-soluble β-glucan is one of the main bioactive components responsible for a number of the putative health benefits attributed to oat products, as highlighted in the articles in this Supplement of the British Journal of Nutrition. The biological behaviour of this polysaccharide is of particular importance in relation to the well-documented effects of oat bran in reducing postprandial glycaemia, insulinaemia and fasting concentrations of the plasma cholesterol (usually the LDL-cholesterol fraction).
The basic chemical structure of oat β-glucan was elucidated as early as the 1960s( Reference Parrish, Perlin and Reese 1 ) and many aspects of its physiochemical properties have also been known for some time( Reference Doublier and Wood 2 ). However, the critical role of the physico-chemical properties of oat β-glucan, notably solution rheology, in determining the physiological effects of oat-containing foods has been more difficult to establish, although understanding of this has improved significantly in recent years. As with other types of water-soluble dietary fibre, such as guar gum (a galactomannan-rich leguminous seed flour), oat β-glucan may confer its physiological influence by the combination of a number of mechanisms. The mechanisms involved in modifying digestion kinetics include, but are not limited to( Reference Judd, Ellis and Soumyanath 3 – Reference Lia, Hallmans and Sandberg 6 ), increasing digesta viscosity in the lumen of the gastrointestinal tract (GIT), interacting with starch and other nutrients, interfering with the activity of gut enzymes (e.g. pancreatic α-amylase) and increasing bile salt excretion. For instance, the effects of oat β-glucan and similar forms of fibre on postprandial glycaemia are strongly related to their capacity to reduce the rate of digestion of macronutrients such as starch( Reference Judd, Ellis and Soumyanath 3 , Reference Kim and White 7 ).
The aim of the present paper is to review the physical and chemical properties of oat β-glucan that have been suggested as potentially influencing gut function and metabolism, a topic covered by other papers in this special issue. The present paper provides some mechanistic insight of the nutritional properties of oat products, with a specific focus on the capacity of oat β-glucan to lower blood-glucose and cholesterol concentrations in human subjects.
Molecular structure and conformation
Chemical structure
Oat β-glucan is a linear polymer of d-glucose bonded by β-(1 → 4) and β-(1 → 3) glucosidic linkages. About 90 % of the glucose units are arranged in blocks of β-(1 → 3)-linked cellotriosyl and cellotetraosyl units, with the rest being the longer cellulosic segments (Fig. 1). The ratio of trisaccharides–tetrasaccharides in oat β-glucan is typically 2:1, which is distinctively different from that found in barley (3:1) and wheat (4:1) β-glucans( Reference Cui, Wood and Blackwell 8 , Reference Cui, Wood and Nishinari 9 ). This structural variation leads to differences in some of the physical properties of these β-glucans, which will be discussed in the following sections. A small variation in the trisaccharide–tetrasaccharide ratio has been reported for oat β-glucan, which may arise from several factors including differences between species( Reference Miller, Wood and Pietrzak 10 ), growing conditions, and extraction and analytical methods. A number of reports have suggested the presence of amino-acid residues( Reference Autio, Myllymäki and Suortti 11 , Reference Vårum and Smidsrød 12 ) and inner C-6 carbon-bound phosphomonoesters( Reference Ghotra, Vasanthan and Wettasinghe 13 ) in the oat β-glucan molecule. However, these additional structural features have not always been observed.

Fig. 1 Chemical structure of oat β-glucan. G represents a glucose unit and the numbers indicate the linkage sites; the ratio of m/n is approximately 2.
Molecular weight and conformation
The molecular weight (MW) and conformation of oat β-glucan are important because they can largely determine many other physical properties, including the solubility, solution viscosity and viscoelasticity and gelation properties. For instance, high MW oat β-glucan does not form a gel, but gels can be produced from samples with reduced MW under certain conditions( Reference Lazaridou and Biliaderis 14 , Reference Lazaridou, Biliaderis and Izydorczyk 15 ). All the studies so far have indicated that oat and other cereal β-glucans adopt overall an extended random coil conformation in aqueous solution( Reference Vårum, Martinsen and Smidsrød 16 – Reference Roubroeks, Mastromauro and Andersson 18 ). Oat β-glucan, like all other natural polysaccharides, has a MW distribution that is polydisperse. This means that, instead of one unique value (as in proteins), a wide MW distribution exists in each polymer sample. Therefore, depending on the method used for the measurement, various MW averages are obtained to describe a polymeric material. The weight-average MW and number-average MW (M w and M n) are the most frequently encountered values for β-glucans, which are often measured, for example, by static light-scattering and osmotic pressure measurements, respectively( Reference Vårum and Smidsrød 12 , Reference Vårum, Smidsrod and Brant 19 , Reference Li, Wang and Cui 20 ). High-performance size exclusion chromatography has been extensively used in recent years for characterisation of β-glucan. When connected with multi-detectors (using reflective index, light scattering and viscometry), the high-performance size exclusion chromatography method can provide a range of molecular parameters in addition to the MW distribution and average MW( Reference Wang, Wood and Cui 21 ). The method of specific dye binding of calcofluor to cereal β-glucans offers a unique way of determining the MW of β-glucan by high-performance size exclusion chromatography. The post-column detection of calcofluor binding to β-glucan makes it possible to measure β-glucan in the presence of other polysaccharides that do not bind this fluorescent stain( Reference Wood 22 , Reference Rimsten, Stenberg and Andersson 23 ). This is especially useful in making comparisons between different food products and extracts containing β-glucan, because purification of β-glucan is not necessary before the measurement.
Factors that affect the molecular weight
The MW of β-glucan in common oat grain (Avena sativa L.), which is the main commercial species, is influenced by the variety and growing conditions( Reference Andersson and Börjesdotter 24 , Reference Ajithkumar, Andersson and Åman 25 ). It has not been possible to directly measure the MW of β-glucans in the cell-wall matrix of oat grain. The MW of β-glucan in groats or flour is subject to changes caused by endogenous hydrolytic enzymes, and various processing and storage factors. Because endo-β-glucanases are present in oat seeds( Reference Wood, Siddiqui and Paton 26 ), inactivation of these enzymes (such as by IR heating, steaming or boiling in aqueous ethanol) is considered essential to obtain high MW β-glucan extracts from the oat-grain cell walls( Reference Wood and Webster 27 , Reference Doehlert, Zhang and Moore 28 ). The reported MW of β-glucan is also therefore subject to significant variations, as a result of differences in the conditions used for extraction and purification of the polysaccharide. The MW obtained for purified oat β-glucans through a well-designed process to minimise molecular depolymerisation is in the range of 2 × 106 to 3 × 106Da. It is known that the MW of β-glucan can be decreased as a result of food preparation such as bread-making( Reference Andersson, Armö and Grangeon 29 ), baking of muffins or cookies( Reference Beer, Wood and Weisz 30 , Reference Kerckhoffs, Hornstra and Mensink 31 ), and extensive extrusion( Reference Tosh, Brummer and Miller 32 ). Åman et al. ( Reference Åman, Rimsten and Andersson 33 ) reported that the MW of β-glucan was preserved in rolled oats, oat bran and different types of oat-bran concentrate, porridge and pancakes, whereas the degradation of β-glucan was observed in bread, pasteurised apple juice, pasta and teacake (see Decker et al. ( Reference Decker, Rose and Stewart 34 ) in this Supplement for more examples).
Solubility and extractability
Content and location in oat grain
The β-glucan contents in oat groats vary considerably with cultivars and growing conditions. It has been shown that a 2- to 3-fold variation in the β-glucan content exists among oat cultivars( Reference Miller, Wood and Pietrzak 10 , Reference Ajithkumar, Andersson and Åman 25 , Reference Peterson, Wesenberg and Burrup 35 ). A number of surveys from different parts of the world revealed that the β-glucan contents were in the range of 1·8–5·5 % of the total dry weight of the oat groat, and frequently in the range of 4·5–5·5 %( Reference Miller, Wood and Pietrzak 10 , Reference Saastamoinen, Hietaniemi and Pihlava 36 , Reference Dvončová, Havrlentová and Hlinková 37 ). Higher β-glucan contents of up to 7 % have also been reported for some oat varieties( Reference Decker, Rose and Stewart 34 ).
Oat β-glucan is primarily located in the cell walls of the starchy endosperm (Fig. 2). The endosperm cell walls of oats( Reference Miller, Fulcher, Webster and Wood 38 ) have a layered structure. In this layered structure, there is a relatively thin outer layer, consisting of mainly water-insoluble polysaccharides such as cellulose, glucomannan and arabinoxylan, and a thick inner layer of water-soluble polysaccharide, most of which consist of the mixed linkage β-glucan plus a small amount of soluble arabinoxylan. The cell wall of the subaleurone layer is usually much thicker than that of the inner endosperm( Reference Wood and Fulcher 39 ) and is rich in β-glucan. A relatively thin layer of β-glucan is also found in the inner layer of the aleurone cell walls. This inner layer is surrounded by a thick insoluble outer layer, which in turn makes the β-glucan less readily soluble compared with the endosperm β-glucan( Reference Miller, Fulcher, Webster and Wood 38 , Reference Wood and Fulcher 39 ).

Fig. 2 β-Glucan in the cell wall of oat stained with calcofluor. a, aleurone; s, subaleurone; e, endosperm. (Courtesy of S. Shea Miller, Agriculture and Agri-Food Canada.)
Solubility and its determination
In the literature, the term ‘solubility’ for a polysaccharide does not represent the true thermodynamic equilibrium solubility. Rather, it is used to refer to the property of a polysaccharide in a solid form or contained in a solid food matrix to disperse in a liquid medium (often water) and form a homogeneous dispersion under specified conditions. It is sometimes used interchangeably with the word ‘extractability’ of the polysaccharides. Solubility is usually expressed by the percentage of the dissolved fraction relative to the total amount of the polysaccharide in the original solid matrix under specific conditions (such as temperature).
The solubility of β-glucan in water has been tested recently under different conditions of processing and laboratory measurements( Reference Johansson, Tuomainen and Anttila 40 ). The choice of the method depends on the objective of the investigation. Clearly, it is only meaningful to compare the solubility of different β-glucans, and indeed other polysaccharide preparations, under the same conditions. In order to establish reliable methods for the preparation of β-glucan concentrates with maximum yields and minimal depolymerisation, several studies have been carried out to compare extractability of β-glucan under various conditions. Different extraction schemes have been developed based on these studies for laboratory or commercial production of β-glucan, and the methods have been reviewed previously( Reference Wood 41 , Reference Stevenson, Webster and Wood 42 ). The extractability of β-glucan was found to be strongly dependent on many factors, such as temperature, pH and the presence of digestive enzymes. When the health benefits of β-glucan as a soluble fibre are of primary interest, the solubility properties of β-glucan under conditions close to the gut environment are highly relevant. Because animal and human trials are expensive and time-consuming, having an in vitro method as a pre-screening tool to evaluate the properties of β-glucan in oat products is very useful.
There are two main types of in vitro methods used to evaluate the solubility of β-glucan in foods. One of these is designed to simulate physiological conditions of digestion in the upper GIT( Reference Beer, Wood and Weisz 30 , Reference Lebet, Arrigoni and Amado 43 ) of human subjects. For a typical example of such a procedure, see the online supplementary materials. This method involves incubating test foods with a series of digestive enzymes at human body temperature (37°C) and appropriate pH levels for defined periods to simulate the acidic and neutral conditions in the stomach and small intestine, respectively. The solubilised β-glucan in the supernatant can then be quantitatively measured by the method of Jørgensen & Aastrup( Reference Jørgensen and Aastrup 44 ), and the total β-glucan content in the same sample can be measured by the method of McCleary & Glennie-Holmes( Reference McCleary and Glennie-Holmes 45 ). A similar procedure has been developed by directly measuring the viscosity developed during the simulated digestion process. This allows the contribution of solubility and MW of β-glucan to the viscosity to be accounted for simultaneously( Reference Gamel, Abdel-Aal and Wood 46 ). Another procedure, which adapts essentially the method of Asp et al. ( Reference Asp, Johansson and Hallmer 47 ), was developed initially for the isolation of water-soluble NSP( Reference Johansson, Tuomainen and Anttila 40 ). This method involves a de-starching process during which the samples are treated at boiling temperature to allow starch gelatinisation. The starch and protein are then hydrolysed by various enzymes, and β-glucan is precipitated from the supernatants by alcohols. A similar approach to this is the use of hot-water extraction at 100°C with only a heat-stable α-amylase and 0·28 mg/ml CaCl2 added( Reference Rimsten, Stenberg and Andersson 23 , Reference Ajithkumar, Andersson and Åman 25 , Reference Åman, Rimsten and Andersson 33 ). Few direct comparisons have been made among the results from these methods. Because it is known that increasing extraction temperature increases the solubility of the polymer( Reference Johansson, Tuomainen and Anttila 40 ), the amount of solubilised β-glucan is likely to be lower using in vitro physiological extraction than that obtained using the hot-water extraction method( Reference Beer, Wood and Weisz 30 ). Further work is required, therefore, to investigate the precise relationship between in vitro measurements of β-glucan solubility and polysaccharide dissolution in vivo.
Factors that influence solubility
Sources
As mentioned earlier, most β-glucan in the oat groat is located in the inner layer of the cell wall that is enclosed by an insoluble cellulosic and hemicellulosic outer layer. It is generally less water soluble than extracted β-glucan material. Because the cell walls in the inner endosperm are thinner than in the aleurone and subaleurone layers, it appears that β-glucan in the milled whole groat is more easily extracted than that in oat bran( Reference Beer, Wood and Weisz 30 , Reference Miller, Fulcher, Webster and Wood 38 ). For example, Beer et al. ( Reference Beer, Wood and Weisz 30 ) has found that the proportion of soluble β-glucan extracted from oat brans and rolled oats, by hot-water extraction in conjunction with a heat-stable α-amylase treatment, was 30–65 and 70 %, respectively (Table 1). Under physiological conditions, the percentage of extractable β-glucan was also noticeably higher in rolled oats than in bran samples.
Table 1 The β-glucan content and the percentage and molecular weight of extracted β-glucan from oat bran and rolled oats using hot water (90°C for 2 h with Termamyl) or physiological in vitro digestion method( Reference Beer, Wood and Weisz 30 )
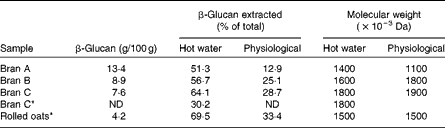
ND, not determined.
* Samples cooked before extraction.
Processing and food preparation
The solubility of β-glucan from oat bran varies largely because of different processing conditions in addition to varietal differences( Reference Mälkki, Autio and Hanninen 48 ). Using an in vitro digestion model to simulate human digestion, Beer et al. ( Reference Beer, Wood and Weisz 30 ) found that only 12–33 % of total β-glucan in bran and rolled oats was solubilised, whereas Tosh et al. ( Reference Tosh, Brummer and Miller 32 ) reported a slightly higher solubility value of 39 % in a commercial oat bran. When the oat bran was incorporated into an extruded cereal, the solubility of β-glucan increased markedly from 39 to 67 % in breakfast cereals prepared under mild extrusion conditions. Further increases in the extrusion temperature and mechanical disruption resulted in a complete solubilisation of β-glucan, although the process was accompanied by a >10-fold decrease in MW. Microscopy observations clearly showed that extrusion disrupted the cell-wall structure from which β-glucan labelled by calcofluor was released and dispersed into the food matrix (Fig. 3). Zhang et al. ( Reference Zhang, Bai and Zhang 49 ) also reported that extrusion improved the solubility of β-glucan in oat bran. Johansson et al. ( Reference Johansson, Tuomainen and Anttila 40 ) have shown that the baking of wheat bread with oat bran and subsequent drying of the bread decrease the amount of β-glucan solubilised, whereas the cooking of oat flakes as porridge increases it. Also, fermentation of oat bran by rye sourdough was shown to increase the solubility of oat β-glucan( Reference Johansson, Tuomainen and Anttila 40 , Reference Degutyte-Fomins, Sontag-Strohm and Salovaara 50 ).

Fig. 3 Cryosections of test cereals, stained with calcofluor and mounted in acid fuchsin, showing that extrusion disrupted the cell wall structure, during which β-glucan (blue stain) was released and dispersed into the food matrix. (a) Premix (control); (b) after moderate extrusion and (c) after extensive extrusion. AL, aleurone cells; SAL, subaleurone cell walls. (Adapted from Tosh et al. ( Reference Tosh, Brummer and Miller 32 ), with permission).
The presence of endogenous enzymes, mainly β-glucanase, can have both detrimental and beneficial effects, the former being a depolymerisation of β-glucan and the latter being an increase in polymer solubility( Reference Zhang, Doehlert and Moore 51 ). In a study by Tosh et al. ( Reference Tosh, Brummer and Wolever 52 ), β-glucanase at different concentrations was added to the wheat dough containing oat bran to produce muffins containing different MW β-glucans. A decrease in MW of β-glucan from 2 200 000 to 400 000 Da led to an increase in the solubility of β-glucan in oat-bran muffins from 44 to 57 %. This phenomenon is in agreement with dissolution studies showing that the hydrolysis of a similar water-soluble polysaccharide, such as guar galactomannan, significantly increased solubilisation of the polymer( Reference Wang, Ellis and Ross-Murphy 53 ). However, when the MW was reduced further to 120 000 Da, the solubility of β-glucan decreased to as low as 26 %. The authors suggested that the subsequent diminution of β-glucan solubility at very low MW (120 000 Da) was due to a stronger self-association of the depolymerised β-glucan, thus leading to the formation of insoluble aggregates. It is also worth speculating that the interaction of β-glucan with other food components, especially proteins via the Maillard reaction, might have contributed to the low solubility. Lower MW polysaccharides seem to possess an increased reactivity towards proteins( Reference Wong, Day and Augustin 54 ) and other components found in foods( Reference Lazaridou, Biliaderis and Izydorczyk 15 , Reference Tosh, Wood and Wang 55 ).
Storage
Storage conditions have been shown to alter the solubility of β-glucan in oat-containing products. Beer et al. ( Reference Beer, Wood and Weisz 30 ) noticed that frozen storage reduced the amount of extractable (soluble) β-glucan in oat muffins and the degree of reduction depended on the other ingredients in the formulation. After 8 weeks of storage, there was up to a 50 % decrease in the extractable β-glucan in the muffins. A more recent study demonstrated that taking fresh muffins through a number of freeze–thaw cycles, each consisting of 14 h at − 18°C and 10 h at room temperature, progressively reduced β-glucan solubility in the muffins. After four cycles of freeze–thaw treatment, the extractable β-glucan was reduced by over 50 %( Reference Lan-Pidhainy, Brummer and Tosh 56 ). The authors ascribed the loss of solubility of β-glucan during frozen storage to the reorganisation of β-glucan chains due to intermolecular interactions (mostly stabilised by hydrogen bonding), which are likely to lead to increased ordered structure( Reference Lazaridou and Biliaderis 14 ).
Isolation and purification
The extent and rate of dissolution of isolated and purified β-glucan powders has not been well investigated, in contrast to materials such as galactomannan-rich guar-gum powders( Reference Wang, Ellis and Ross-Murphy 53 , Reference Wang, Ellis and Ross-Murphy 57 , Reference Wang, Ellis and Ross-Murphy 58 ). Panahi et al. ( Reference Panahi, Ezatagha and Temelli 59 ) showed that an oat β-glucan concentrate (60 % of β-glucan on dry weight basis) prepared by an alcohol-based enzymic technique had better solubility and higher MW than the product produced by a conventional aqueous extraction process. It has long been known that the solubility of isolated β-glucan is influenced by the drying method. Freeze-dried oat and wheat β-glucans are sometimes difficult to dissolve in water( Reference Cui, Wood and Nishinari 9 ); the replacement of water molecules with isopropanol before drying (the so-called solvent exchange process) is critical to ensure good solubility of β-glucan isolates. The effect of MW on the solubility of β-glucan isolates therefore also depends on the way in which the isolate is prepared. In general, the ease of solubilisation of a polymer decreases as MW increases. This behaviour was demonstrated clearly with guar galactomannan powders where an inverse relationship between the dissolution rate and MW was observed( Reference Wang, Ellis and Ross-Murphy 53 ). Recently, Kim & White( Reference Kim and White 7 ) reported that the solubility of a β-glucan isolate decreased with an increase in MW in a starch suspension after in vitro digestion. This is not always the case with oat β-glucan isolates, however. When the solvent exchange stage before drying is omitted, low-MW β-glucan molecules can potentially form stronger polymer–polymer interactions than the high-MW molecules do, which may then lead to a low solubility.
Rheological properties in aqueous medium
Viscoelastic properties of β-glucan solution
A freshly prepared and fully hydrated oat β-glucan solution behaves as a typical neutral random-coil polysaccharide solution. The viscosity of a polysaccharide solution depends on the structure, MW and polymer concentration, as well as conditions of measurement, such as shear rate and temperature. The shear rate dependence of viscosity for oat β-glucan has been extensively studied( Reference Doublier and Wood 2 , Reference Ren, Ellis and Ross-Murphy 60 ). In terms of conditions in the GIT, shear rate is related to the degree of mixing of fluid (digesta) caused by peristalsis, although flow patterns in the gut are obviously much more complex. Fig. 4 shows the steady shear-flow profiles for solutions of a high MW (2 × 106Da) oat β-glucan at different concentrations( Reference Ren, Ellis and Ross-Murphy 60 ). At low β-glucan concentrations (approximately lower than the overlap concentration c*), the solution viscosity does not change with respect to shear rate, a form of behaviour that is typical of a Newtonian fluid. At high concentrations, however, shear-thinning behaviour is observed, meaning that the viscosity decreases with an increasing shear rate. Because of the shear-rate dependence of viscosity, the solution viscosities of different samples have to be compared at the same shear rate, most usefully the value at ‘zero’ shear rate is preferred. In heterogeneous systems containing a polysaccharide gum and insoluble particulates, which are common in food suspensions or ingested food mixed with digestive fluids (i.e. digesta) in the gut, more complex rheological behaviour is seen. For instance, if there is a high fractional volume of insoluble particulates present, the suspensions exhibit shear-thinning even at low shear rates (i.e. loss of the Newtonian plateau) and also substantial increases in viscosity are observed( Reference Rayment, Ross-Murphy and Ellis 61 , Reference Rayment, Ross-Murphy and Ellis 62 ). For calculation of the zero-shear viscosity from the flow profiles, see online supplementary material. As seen in the literature, most researchers, in an effort to simplify the method and obtain reproducible data, remove the particulate material by centrifugation before taking viscosity readings, because the presence of insoluble material can seriously interfere with measurements. Moreover, a single measurement of viscosity is often performed at an arbitrary shear rate (see Table 2). This is because the shear rates that occur in the intestinal lumen of human subjects and experimental animals following a meal containing a polysaccharide gum are not known and may vary at different regions of the gut and at different postprandial times. Also, zero shear viscosity is not always readily obtainable due to instrumental limitations. Caution is therefore needed when comparing viscosity data from different laboratories.

Fig. 4 Apparent viscosity (η) v. shear rate (
$$ \gamma \bullet $$
) for different concentrations of oat β-glucan solutions. (Re-printed, with permission, from Ren et al.
(
Reference Ren, Ellis and Ross-Murphy
60
)).
Table 2 Examples of viscosity (η) measured at specific shear rates (
$$ \gamma \bullet $$
) and temperature (temp.) of oat-containing meals tested in human studies, which have demonstrated positive postprandial blood-glucose lowering (PGL) and cholesterol-lowering (CL) effects

M p, peak molecular weight; M w, weight average molecular weight; values with *indicate Mw only; ND, not determined.
Solution viscosity is affected by the polymer concentration (c) and MW in a power-law relationship: η~(c[η]) n . The intrinsic viscosity, [η], is a measure of hydrodynamic volume of the individual molecule in solution and is related to MW by, for instance, the Mark–Houwink relationship ([η] = k MWα, where k and α are constants). Therefore, the parameter c[η] represents the space occupancy of the polymer molecules in the solution by taking into account both concentration and MW factors. Fig. 5 is a double logarithmic plot of ηsp,0 (the specific viscosity at zero shear rate) v. the parameter cη for oat β-glucan solutions. This plot consists of two linear regions connected by a continuous transition region starting at c[η] approximately 1. The concentration at this point is designated c*, which corresponds to the concentration at which individual β-glucan molecules begin to overlap. For example, for two β-glucans of MW 1 million Da and 0·1 million Da, the c* values are approximately 0·1 and 0·5 %, respectively. At c< c*, the solution is classified as being in a dilute regime and the relationship ηsp,0~c 1·1 holds; whereas at c>c*, the solution is classified as being in a semi-dilute regime and ηsp,0~c 4·4. It is well known that the viscosity of β-glucan solution changes more markedly with concentration in the semi-dilute solution than it does in dilute solution. The zero-shear viscosity at the critical concentration is approximately 1 mPa·s (Fig. 5). In most clinical trials that showed positive blood-glucose and cholesterol-lowering effects, the viscosity (as estimated in vitro in the shear rate range 10–30 s− 1) is considerably higher than this value (Table 2), although the in vivo viscosity may be different from that measured in vitro ( Reference Wood, Braaten and Scott 63 ). According to Wood( Reference Wood 41 ), the (in vitro viscosity) dose–response for the glycaemic response of β-glucan is in the range of 20–2000 mPa·s (at shear rate 30 s− 1). When the viscosity is lower than 20 mPa·s, no glucose-lowering effect is observed, whereas when the viscosity is higher than 2000 mPa·s, no further reductions in blood glucose are seen. In other words, the metabolic response appears to reach a plateau above 2000 mPa·s. This highlights the importance that changes in MW have on the viscosity of β-glucan products, which in turn determines their physiological activity.

Fig. 5 Log ηsp,0 (the specific viscosity at zero shear rate) v. log c[η] for oat β-glucan. ●: data of Doublier & Wood( Reference Doublier and Wood 2 ); Δ: data from Ren et al. ( Reference Ren, Ellis and Ross-Murphy 60 ). (Reprinted, with permission, from Ren et al. ( Reference Ren, Ellis and Ross-Murphy 60 )).
It must be emphasised that in vitro viscosities of polysaccharides might not accurately reflect the viscosities of these polymers when present in the GIT. The level of viscosity required to achieve a specific physiological effect has not been carefully reviewed and systematically studied. There are no data available on the direct measurements of luminal viscosity of oatmeals. However, studies by Marciani et al. ( Reference Marciani, Manoj and Hills 64 , Reference Marciani, Gowland and Spiller 65 ) on locust bean gum, a source of water-soluble galactomannan with rheological properties similar to high MW oat β-glucan, provides some useful comparative information. In these studies, the gastric-content samples were obtained after ingestion of meals containing different doses of locust bean gum using nasogastric intubation and aspiration, and the viscosity was measured in vitro by a viscometer. This group also used an echo-planar MRI technique to measure the in vivo gastric lumen viscosity, which allowed viscosity to be measured non-invasively( Reference Marciani, Manoj and Hills 64 ). A good correlation was obtained between the rheological data from in vitro viscometry and echo-planar MRI. Table 2 lists the viscosity of test meals that have demonstrated positive physiological functions. Taking these data into consideration, along with the recommended minimum dose of oat β-glucan used for achieving significant physiological effects, the viscosity of the luminal digesta seems to fall within the lower end range of a semi-dilute regime (see Fig. 5).
From the analysis of data from Lan-Pidhainy et al. ( Reference Lan-Pidhainy, Brummer and Tosh 56 ), it was found that the viscosity was related to concentration exponentially by an exponent of 1·51, although the soluble β-glucan concentration was well within the range of the dilute regime. This is due, at least in part, to the existence of particulate materials, which are known to raise the viscosity of a solution/suspension as explained by Rayment et al. ( Reference Rayment, Ross-Murphy and Ellis 61 ). It is well documented that cereal β-glucan tends to form macromolecular aggregates in aqueous solutions( Reference Li, Wang and Cui 20 , Reference Wang, Wood and Cui 21 , Reference Tosh, Wood and Wang 66 ). Moreover, the low-MW oat β-glucan aggregates more easily than their high-MW counterparts( Reference Cui, Wood and Blackwell 8 , Reference Lazaridou and Biliaderis 14 , Reference Lazaridou, Biliaderis and Izydorczyk 15 ). Low-MW β-glucan solutions have been shown to have a ‘yield stress flow’ behaviour at very low shear rates giving higher than expected apparent viscosity values, which is attributed to the presence of β-glucan aggregates (Fig. 4)( Reference Doublier and Wood 2 , Reference Ren, Ellis and Ross-Murphy 60 ).
Impact on the blood-glucose and cholesterol-lowering properties
The mechanisms by which oat β-glucan and other soluble fibres elicit their physiological effects on carbohydrate and lipid metabolism are discussed in the article on oats and CVD risk markers by Thies et al. ( Reference Thies, Masson and Boffetta 67 ) in this Supplement. Although several mechanisms have been proposed, the ability of the soluble polysaccharide to increase the viscosity of the gut digesta, and thus to delay nutrient absorption from the gut, is believed to be a key factor( Reference Judd, Ellis and Soumyanath 3 , Reference Jenkins, Wolever and Leeds 68 ). Wood et al. ( Reference Wood, Braaten and Scott 63 ) first demonstrated an inverse linear relationship between peak postprandial blood glucose and insulin increments and log (viscosity) in healthy subjects who consumed glucose drinks containing extracted and purified β-glucan samples of different doses and MW values. A number of studies also reported that high-viscosity β-glucan drinks significantly attenuated postprandial glycaemia, but low-viscosity β-glucan showed no such effect( Reference Panahi, Ezatagha and Temelli 59 ). A similar relationship was reported when β-glucan was incorporated into a food matrix such as baked goods and breakfast cereals( Reference Brummer, Duss and Wolever 69 , Reference Tappy, Gügolz and Würsch 70 ). Recently, an inverse linear relationship between glycaemic responses and in vitro viscosity of extracted β-glucan, as measured under simulated physiological conditions, was clearly demonstrated in healthy subjects consuming bread( Reference Östman, Rossi and Larsson 71 ), muffins( Reference Tosh, Brummer and Wolever 52 , Reference Regand, Tosh and Wolever 72 ) and granolas( Reference Regand, Chowdhury and Tosh 73 ) containing oat or barley β-glucans.
In a recent human intervention trial using extruded breakfast cereal containing oat β-glucan of various MW values, Wolever et al. ( Reference Wolever, Tosh and Gibbs 74 ) demonstrated an inverse relationship between serum LDL-cholesterol and log (viscosity), as measured by in vitro extraction under physiological conditions. These studies provide convincing evidence that the ability of β-glucan and other viscous polysaccharides to increase the digesta viscosity in the GIT is a key factor in determining their blood-glucose and cholesterol-lowering effects. In addition to this rheological mechanism, direct interference of soluble fibre with the biochemical degradation of starch( Reference Regand, Chowdhury and Tosh 73 , Reference Slaughter, Ellis and Jackson 75 , Reference Brennan, Blake and Ellis 76 ) and bile-acid binding( Reference Kim and White 5 ) have been reported to contribute to these beneficial effects on metabolism.
Although numerous human trials have been carried out to test oat β-glucans from various sources and present in different forms of foods, only some of these studies showed reductions in blood-cholesterol and -glucose concentrations( Reference Keogh, Cooper and Mulvey 77 – Reference Tosh 79 ). This discrepancy can be attributed to a range of factors, apart from the β-glucan dosage used, that may potentially affect the amount and MW of solubilised β-glucan in the GIT by influencing the alimentary viscosity. Regand et al. ( Reference Regand, Tosh and Wolever 72 ) reported that depolymerisation of β-glucan in bread and pasta reduced its efficacy in attenuating the peak blood-glucose response in healthy human subjects. The same group also found that an extruded oat-based breakfast cereal given at a dose of 3 g of oat β-glucan/d with a high (2·2 × 106Da) or medium (0·5 × 106Da) MW lowered LDL-cholesterol similarly (5 %), but the efficacy was reduced by 50 % when MW was reduced to 0·2 × 106Da( Reference Wolever, Tosh and Gibbs 74 ). Kerckhoffs et al. ( Reference Kerckhoffs, Hornstra and Mensink 31 ) also demonstrated that the cholesterol-lowering effect of β-glucan from oat bran administered in orange juice diminished when the same β-glucan is incorporated into bread and cookies. Depolymerisation of β-glucan and/or reduced solubility in food may account for the attenuation in efficacy reported for the latter products. Moreover, it has been shown that the reduced solubility induced by freeze–thaw cycling( Reference Lan-Pidhainy, Brummer and Tosh 56 ) or gelation( Reference Kwong, Wolever and Brummer 80 ) decreased the efficacy of β-glucan in lowering postprandial glycaemic responses in human subjects. These studies provide compelling evidence that the physico-chemical properties of oat β-glucan play a crucial role in determining its physiological functions. Thus, reduced solubility and/or MW of β-glucan might be responsible for some of the published studies that failed to show positive metabolic effects of oat products.
Conclusions and future research
Based on the results of physiological and human studies to date, there is strong evidence to show that oat β-glucan lowers total and LDL-cholesterol and attenuates postprandial glycaemia and insulinaemia when it is ingested in sufficiently high doses and at a suitably high MW. The physico-chemical properties of oat β-glucan, namely MW and solubility, should be considered when assessing the physiological properties. Oat β-glucan concentrates or isolates have shown similar beneficial effects to oat bran and rolled oats, provided that detrimental processing conditions are avoided.
The current recommended intake of ≥ 3 g/d β-glucan by US Food and Drug Administration does not ensure a significant decrease in blood cholesterol, because the quantity of β-glucan ingested does not solely determine the physiological efficacy. It is the amount and MW of β-glucan that become solubilised in the GIT that determines, to a large extent, the blood-glucose and cholesterol-lowering properties. Clear inverse linear relationships have been demonstrated between the in vitro log (viscosity) of drink or extracts from solid foods and postprandial blood-glucose or fasting blood-cholesterol concentrations. It appears that there is a range of viscosity values over which β-glucan exhibits such biological activities, although the minimum and maximum values for this range need to be defined. There is little doubt that in vitro evaluation can be effectively used to screen oat products in order to increase the likelihood of success in achieving beneficial biological effects in human subjects. How the viscosity of drinks or extracts measured in vitro corresponds to the in vivo viscosity developed in the GIT is not straightforward. A combination of factors, including the dilution effects of digestive fluids, enzymic degradation of β-glucan and interaction of this polymer with other food components, is likely to influence the in vivo viscosity. Direct measurements of viscosity in the human gut have not yet been carried out on ingested oat products, as has been done with other polysaccharides such as the leguminous galactomannans (locust bean gum)( Reference Marciani, Manoj and Hills 64 , Reference Marciani, Gowland and Spiller 65 ). Accordingly, the minimum in vivo viscosity that is required to achieve desirable metabolic effects is currently unknown.
The development of inexpensive and palatable oat-containing products, which are still physiologically effective, is needed in the future. To facilitate this, it is necessary to establish a standardised protocol to characterise β-glucan products, which provides sufficient physico-chemical data to allow useful comparisons and interpretations to be made of the results obtained in various human studies.
Supplementary material
To view supplementary material for this article, please visit http://dx.doi.org/10.1017/S0007114514002256
Acknowledgements
The authors thank Shea Miller at Agriculture and Agri-Food Canada (AAFC) for providing Fig. 2 and Peter Butterworth (Kings College London), Simon Ross-Murphy (University of Nottingham), and Steve Cui and Susan Tosh of AAFC for helpful feedback on various drafts of the present paper. The authors dedicate this review to the late Peter Wood, a close colleague and friend of both the authors, for his huge research contribution to the structure, properties and nutritional effects of oat β-glucan. Q. W. received an honorarium from Quaker Oats Company (a subsidiary of PepsiCo, Inc.) for attending the workshop in May 2012 to discuss the content of the supplement. Research in the laboratory of P. R. E. was funded by the Biotechnology and Biological Sciences Research Council, UK.
Author contributions: Q. W. drafted the paper and P. R. E. contributed to writing and editing the paper.
This paper was published as part of a supplement to British Journal of Nutrition, publication of which was supported by an unrestricted educational grant from Quaker Oats Co. (a subsidiary of PepsiCo Inc.). The papers included in this supplement were invited by the Guest Editor and have undergone the standard journal formal review process. They may be cited.
The Guest Editor to this supplement is Roger Clemens. The Guest Editor declares no conflict of interest.