Epidemiological studies have suggested that there is an inverse correlation between the consumption of polyphenol-rich foods and the prevention of CVD(Reference Hertog, Kromhout and Aravanis1, Reference Lampe2). Moderate red wine intake has also been associated with a reduced coronary artery disease mortality(Reference Gronbaek, Becker and Johansen3, Reference Klatsky, Friedman and Armstrong4), which may be due to its ability to improve endothelial function(Reference Szmitko and Verma5), induce an acute increase in endothelium-dependent flow-mediated dilatation(Reference Agewall, Wright and Doughty6, Reference Hashimoto, Kim and Eto7) and inhibit endothelin-1 synthesis(Reference Fitzpatrick, Hirschfield and Coffey8, Reference Corder, Douthwaite and Lees9). These effects of red wine have been linked to both its alcohol content and to its high content of polyphenols, in particular flavonoids, hydroxycinnamates and phenolic acids(Reference Scalbert and Williamson10). Following consumption, nanomolar quantities of flavonoids and other polyphenols enter the circulation(Reference Scalbert and Williamson10, Reference Spencer, Abd El Mohsen and Minihane11) where they may act to improve NO bioavailability and/or inhibit endothelin-1(Reference Loke, Hodgson and Proudfoot12, Reference Stoclet, Chataigneau and Ndiaye13). In support of this, white wine, which has significantly lower concentration of polyphenols, induces significant reduced vascular effects(Reference Shimada, Watanabe and Hosoda14, Reference van Velden, Mansvelt and Fourie15), although its cardioprotective effects have been reported(Reference Cui, Tosaki and Cordis16, Reference Dudley, Lekli and Mukherjee17).
In contrast to white wine, Champagne wine is relatively rich in polyphenols such as hydroxybenzoic acids, hydroxycinnamic acids (and their tartaric derivative esters), flavonoids, phenolic alcohols and phenolic aldehydes(Reference Chamkha, Cathala and Cheynier18). The increased levels of phenolics in Champagne wine derive predominantly from the two red grapes, Pinot Noir and Pinot Meunier, which are used in its production along with the white grape Chardonnay(Reference Constant19). Moderate Champagne wine consumption has been shown to exert a number of effects in vivo, effecting peripheral serotonin and dopamine release(Reference Boyer, Bancel and Fabbro Perray20) and increasing plasma vitamin A concentration(Reference Cartron, Fouret and Carbonneau21). Champagne wine polyphenols have also be shown to protect cells against injury induced by peroxynitrite(Reference Vauzour, Vafeiadou and Corona22), a physiologically relevant oxidising species which has been implicated in vascular wall pathology(Reference van der Loo, Labugger and Skepper23, Reference Xu, Ying and Jiang24). However, thus far, there have been no studies investigating its consumption and changes in endothelial function and thus cardiovascular risk.
In the present study, we have performed a randomised, single-blind, controlled, cross-over design study in order to assess whether acute, moderate Champagne wine consumption is capable of modulating endothelial function in healthy human volunteers. We show that consumption of Champagne wine induces acute changes in endothelium-independent vasodilatation but not endothelium-dependent vasodilatation. These effects were accompanied by a change in the level of matrix metalloproteinase-9 (MMP-9), reductions in plasma levels of oxidants and with an increased urinary excretion of a number of phenolic metabolites. Together, our data suggest that moderate Champagne wine consumption may help to improve cardiovascular risk via its effects on the vasculature and that that these effects may be mediated by circulating Champagne wine-derived polyphenols.
Material and methods
Materials
Phenolic standards (caffeic acid, ferulic acid, homovanillyl alcohol, gallic acid, vanillic acid, p-hydrobenzoic acid, (+)-catechin, ( − )-epicatechin, hydroxytyrosol, vanillin, hydroxyhippuric acid, p-coumaric acid, protocatechuic acid, sinapic acid, hydroferulic acid, 3,4-dihydroxyphenylacetic acid, tryptophol, resveratrol, quercetin, hippuric acid) and type H-1 β-glucuronidase from Helix pomatia (EC 3.2.1.31) were all obtained from Sigma (Poole, Dorset, UK). Caftaric acid was obtained from Apin Chemicals (Abingdon, Oxon, UK), homovanillic acid was obtained from Lancaster Synthesis Ltd (Heysham, Lancs, UK) and tyrosol and isoferulic acid were purchased from Extrasynthese (Lyon, France). Solvents were all of HPLC grade and were purchased from Fisher Scientific (Loughborough, Leics, UK). Ethyl acetate was purified by distillation on a Raschig column before use. The K2EDTA, serum separation tubes and heparin vacutainer tubes were obtained from BD Vacutainer (Oxford, Oxon, UK). Wallace Y-can cannulas were from 3S Healthcare (Enfield, London, UK).
Subjects
Healthy male and female subjects (n 15), aged between 20 and 65 years (mean age 39·5 (sem 4·3) years) with a BMI of 18·9–28·4 kg/m2 (mean BMI 23·6 (sem 0·7) kg/m2), were recruited from the University of Reading and surrounding area. Individuals with diabetes mellitus, any form of liver or gastrointestinal disorder, high blood pressure (>150/90 mm/Hg), anaemia, gall bladder problems, present illness, or those taking dietary supplements, consuming caffeine or aspirin, vigorous exercise (more than three times × 20 min per week), or alcohol consumption more than 120 g (women) and 168 g (men) per week were excluded from the study, along with pregnant or lactating females. Subjects were healthy, based on a medical questionnaire, and had normal concentrations of liver enzymes (aspartate aminotransferase, alanine aminotransferase and γ-glutamyl transferase), normal Hb, packed cell volume and leucocyte counts and an absence of glucose and protein in urine.
Study design
The study was conducted according to the guidelines laid down in the Declaration of Helsinki and all procedures involving human subjects were approved by the University of Reading Research Ethics Committee (ref. 07/16). The study was also registered with the National Institutes of Health (NIH) randomised trial records held on the NIH ClinicalTrials.gov website (ref. NCT00937313) and with the Current Controlled Trials website (ref. ISRCTN38867650). Written informed consent was obtained from all subjects before the study started. Subjects refrained from consuming high-polyphenol foods for 48 h before the start of the study and for 32 h post-initiation. In particular, the following foods and beverages were excluded from volunteer diets: cocoa-containing products, coffee, tea and wine. The study was designed as a single-blind, randomised, cross-over intervention trial, where volunteers were asked to consume either 375 ml of Champagne wine (Chardonnay, Pinot Noir and Pinot Meunier; 12 % alcohol) or a control matched for alcohol content, fruit sugars and acids (Table 1). The Champagne wine used in the study contained no vitamin C. Subjects were assessed for anthropometric measurements and provided a urine sample before baseline laser Doppler imaging with iontophoresis (LDI) measurements (detailed below). Subjects were then cannulated in the antecubital vein and one baseline blood sample was collected. Subjects were then randomly assigned to either the Champagne wine or control group and asked to consume the beverage within a 10 min period. Following a standardised breakfast, blood samples were collected at 15, 30, 45, 60, 120, 180, 240, 300, 360 and 480 min post-consumption and pooled urine samples were collected over 3 × 8 h periods. A standardised breakfast and lunch were consumed at 15 and 200 min post-beverage. LDI measurements were carried out at 120, 240, 360 and 480 min. Subjects also provided 24 h and 32 h blood and urine samples. Following a washout period of 28 d, volunteers returned to the unit to complete the second arm of the study where the procedure above was repeated.
Table 1 Composition of Champagne wine and placebo
(Mean values and standard deviations)
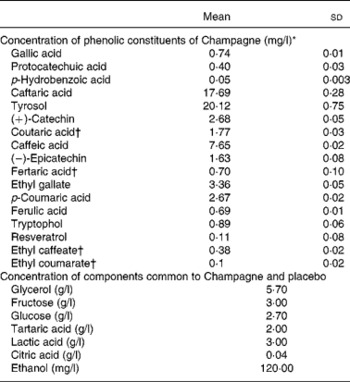
* Calculated from three independent injections.
† Calculated using the equivalent of the corresponding cinnamic acid derivative.
Laser Doppler imaging with iontophoresis
In all cases, subjects were rested in the supine position for 30 min in a temperature-controlled environment (22–24°C) before LDI determination. Peripheral microvascular function was assessed using a validated technique which quantifies the vasodilator responses to 1 % acetylcholine (endothelium-dependent vasodilatation) and 1 % sodium nitroprusside (endothelial-independent vasodilatation), delivered transdermally using iontophoresis. This non-invasive in vivo method provides a robust surrogate marker of vascular function, as described previously(Reference Armah, Jackson and Doman25, Reference Ferrell, Ramsay and Brooks26). For the vascular reactivity measurements, participants were requested to lie in a semi-recumbent position with their right arm supported. A temperature probe and iontophoresis chambers were attached to the volar aspect of the forearm and freshly prepared solutions of acetylcholine chloride (2·5 ml; 1 % (w/v) in 0·5 % (w/v) NaCl solution; Sigma Aldrich, Poole, Dorset, UK) and sodium nitroprusside (2·5 ml; 1 % (w/v) in 0·5 % (w/v) NaCl solution; Sigma Aldrich) were introduced into the anodal and cathodal chambers respectively. Following a basal measurement of skin perfusion, an incremental current was delivered progressively in 5 μA steps (5, 10, 15 and 20 μA) to yield a total charge (current × time) of 8000 coulombs during a 20 min measurement. A series of fifteen scans was performed as the current increased from 0 to 20 μA (with a further five scans performed following current termination) and skin perfusion, or erythrocyte flux, was measured using a laser Doppler imager (Moor Instruments Ltd, Axminster, Devon, UK). In all cases the within-day and between-day CV were less than 10 %, as previously reported(Reference Ramsay, Ferrell and Greer27).
Matrix metalloproteinase and tissue inhibitor of metalloproteinase analysis
MMP and tissue inhibitor of metalloproteinase (TIMP) were analysed by gelatine zymography and reverse zymography, respectively, as previously described(Reference Buache, Garnotel and Aubert28). Briefly, for the detection of gelatinases in serum, SDS-PAGE was performed on gels containing 0·1 % gelatine and 9 % polyacrylamide. Samples (dilution 1/50), previously mixed with loading buffer (2 % SDS and 0·1 % bromophenol blue), were electrophoresed under non-reducing conditions. After electrophoresis, gels were washed in 2 % Triton X-100 and immersed in buffer containing 50 mm-2-amino-2-hydroxymethyl-propane-1,3-diol (Tris)-HCl (pH 7·6), 200 mm-NaCl and 10 mm-CaCl2 for 18 h at 37°C. The gels were stained with 0·5 % Coomassie blue G-250 in acetic acid–methanol–water (1:4:5, by vol.) and de-stained in acetic acid–methanol–water (1:2:7, by vol.). The gelatinolytic activities appeared as clear bands against the blue background of gelatine. For the detection of TIMP in serum, SDS-PAGE was performed on gels containing 0·1 % gelatine, 12 % polyacrylamide and pro-MMP-2 (20 ng/ml). Samples (dilution 1/50), previously mixed with loading buffer (2 % SDS and 0·1 % bromophenol blue), were electrophoresed under non-reducing conditions. After electrophoresis, gels were washed in 2 % Triton X-100 and immersed in buffer containing 50 mm-Tris-HCl (pH 7·6), 200 mm-NaCl and 10 mm-CaCl2 for 18 h at 37°C. The gels were stained with 0·5 % Coomassie blue G-250 in acetic acid–methanol–water (1:4:5, by vol.) and de-stained in acetic acid–methanol–water (1:2:7, by vol.). Reverse zymography revealed inhibitory activity, which appeared as blue zones against a clear background, demonstrating inhibition of gelatine lysis in the gels. MMP-2, MMP-9, TIMP-1 and TIMP-2 concentrations in serum were quantified with human commercial ELISA kits (Amersham Biosciences) following the manufacturer's instructions. Each sample was assayed in duplicate, and the values were within the linear portion of the standard curve.
HPLC analyses
Analysis of Champagne wine extracts for phenolic content were performed as previously described(Reference Vauzour, Vafeiadou and Corona22). Determination of the excreted urinary metabolites was carried out as follows: untreated urine samples were filtered through a 0·45 μm membrane before HPLC analysis. For β-glucuronidase treatment, urine samples (1 ml) were acidified with 1·2 m-acetic acid (50 μl) and mixed with β-glucuronidase (230 mg/ml in 0·2 m-sodium acetate; pH 5) under Ar for 45 min at 37°C. After addition of 1 m-HCl (40 μl), samples were extracted twice with ethyl acetate and centrifuged for 15 min at 5000 g. The combined organic layers were evaporated under N2, re-dissolved by vortexing in 25 % aqueous methanol (200 μl), filtered (polytetrafluoroethylene (PTFE) membrane, 0·45 μm; Millipore, Chandlers Ford, Hants, UK) and injected (50 μl) onto an Agilent 1100 Series HPLC linked to a diode array detector. Sample separation was achieved using a C18 Nova Pak® column (250 × 4·6 mm internal diameter; 5 μm particle size), fitted with a guard column C18 NovaPak® (Waters Ltd, Elstree, Herts, UK). The mobile phase consisted of: A, aqueous methanol (5 %)+5 m-HCl (0·1 %) and B, acetonitrile–methanol (1:1)+5 m-HCl (0·1 %) and was pumped through the column at 0·7 ml/min. Samples (50 μl) were injected and separated using the following gradient system (min/% B): 0/5, 5/5, 40/50, 55/100, 59·9/100, 60/5 and the eluant was monitored by photodiode array detection at 254, 280, 320 and 370 nm, with spectra of products obtained over the 220–600 nm range. All data were analysed using ChemStation® software (Agilent Technologies, Inc., Santa Clara, CA, USA). Components were identified according to retention time, UV or visible spectra and spiking with commercially relevant standards when available.
Biochemical analysis
The blood samples collected in lithium–heparin tubes were spun (1700 g; 10 min; 4°C) immediately after collection. Samples were also collected in serum separation tubes (SST) and allowed to stand for 30 min before centrifugation (1300 g; 10 min; 21°C). All samples were sampled and frozen at − 80°C until analysis. All biochemical parameters were assayed on an ILAB 600 chemistry analyser (Instrumentation Laboratory, Warrington, Cheshire, UK) using enzyme-based colorimetric tests supplied by Instrumentation Laboratory. The following parameters were determined in all samples: total cholesterol, LDL-cholesterol, HDL-cholesterol, glucose, TAG, uric acid, total bilirubin, albumin, C-reactive protein, aspartate aminotransferase, alanine aminotransferase and γ-glutamyl transferase. Plasma total antioxidant capacity (TAC) was measured by using the TAC kit provided by Medicon SA (Gerakas, Greece) as reported previously(Reference Kampa, Nistikaki and Tsaousis29). This assay is based on the competition of a parallel reaction, where the peroxyl-radical donor 2,2-azobis-(2-amidinopropane) dihydrochloride (ABAP) bleaches the carotenoid crocin. Antioxidants present in the sample then inhibit the bleaching by trapping formed radicals. The assay was performed at 37°C in the following steps: 2 μl of sample, calibrator or control were mixed with 250 μl of crocin reagent (R1) and incubated for 160 s. Subsequently, 125 μl ABAP (R2) were added and the decrease in absorbance at 450 nm was measured 256 s later. Values of TAC were expressed as mmol/l of Trolox and corrected TAC values were calculated from TAC after subtraction of the interactions due to endogenous uric acid, bilirubin and albumin accounting for 0·11, 0·11 and 0·01 mmol/mg of the antioxidant capacity, respectively(Reference Kampa, Nistikaki and Tsaousis29, Reference Malliaraki, Mpliamplias and Kampa30). Endothelin-1 was determined using ELISA kits obtained from R&D Systems (Abingdon, Oxon, UK). Total NO levels were assessed by using the Total NO/Nitrite/Nitrate assay kit (ref. KGE001) obtained from R&D Systems. Total NO (NO2− /NO3− ) in serum/plasma ranged between 10 and 97 μmol/l (mean 37 μmol/l; n 25). Before analysis, samples were deproteinised by using 10 000 Da molecular-weight cut-off filters (R&D Systems). Total oxidative capacity (TOC) was determined by a rapid enzymic in vitro diagnostic assay (POX-ACT) obtained from Tatzber KEG (Höflein at the Danube, Austria)(Reference Tatzber, Griebenow and Wonisch31). This assay measures endogenous levels of peroxides, an indicator of either the production of pro-oxidative substances by the organism or an impaired uptake or consumption of antioxidants, using tetramethylbenzidine as the chromogen substrate. This assay is robust and provides intra- and inter-assay CV of 3·73 and 5·51 %, respectively.
Statistical analysis
Data were analysed using SPSS version 12.1 (SPSS, Inc., Chicago, IL, USA). Results are presented in the text and figures as mean values with their standard errors. Bonferroni tests for multiple comparisons and t tests were subsequently used to examine differences between individual treatments. All data were checked for normality and log-transformed where necessary before statistical analysis. Values of P < 0·05 were taken as significant.
Results
Assessment of vascular reactivity by laser Doppler imaging with iontophoresis
Consumption of both the Champagne wine (P = 0·030) and the control (P = 0·045) induced an increase in endothelium-dependent vascular reactivity at 4 h, as indicated by increases in skin erythrocyte flux in the presence of acetylcholine chloride (Fig. 1(a)). These increases in vascular reactivity returned to baseline by 8 h and there was no significant difference between the vascular effects induced by Champagne wine or the control at any time point. Endothelium-independent vasodilatation (skin erythrocyte flux following iontophoresis with sodium nitroprusside) was found to be significantly increased at 4 and 8 h Champagne wine consumption (P = 0·045 and P = 0·037, respectively) and a close to linear correlation was found between treatment and time (P = 0·057). In contrast, the alcohol-matched control did not induce endothelium-independent changes in vascular reactivity. Furthermore, there was a significantly greater degree of endothelium-independent vasodilatation observed following Champagne wine intervention relative to placebo intervention at 4, 6 and 8 h (P = 0·013, P = 0·034 and P = 0·031, respectively).

Fig. 1 Response of forearm skin erythrocyte flux v. baseline, following the iontophoresis of (a) acetylcholine or (b) sodium nitroprusside. (–■–), Champagne; (- -●- -), placebo. Values are means, with standard errors represented by vertical lines. The vasodilatation to sodium nitroprusside was significantly higher in the Champagne wine group than the placebo group (P < 0·05). * Mean value was significantly different from that at baseline (0 h) (P < 0·05). † Mean value was significantly different from that following placebo intake (P < 0·05).
Urinary excretion of polyphenols
The major phenolic derivatives indentified in urine were hippuric acid, protocatechuic acid, isoferulic acid, homovanillic acid, homovanillyl alcohol and 3,4-dihydroxyphenylacetic acid (Fig. 2). All of these compounds were present in baseline urine samples and all, with the exception of isoferulic acid, increased significantly over time following intervention with either Champagne wine or the alcohol control (Fig. 2). However, the mean total excretion of hippuric acid (Champagne wine, 159·30 (sem 29·3) mg; control, 100·70 (sem 20·8) mg; P < 0·001), protocatechuic acid (Champagne wine, 10·60 (sem 1·30) mg; control, 7·17 (sem 1·56) mg; P < 0·05) and isoferulic acid (Champagne wine, 2·04 (sem 0·84) mg; control, 0·24 (sem 0·39) mg; P < 0·001) were all significantly greater following the Champagne wine intervention compared with the control intervention. More specifically, hippuric acid excretion (Fig. 2(a)) was significantly higher at 8 h (P = 0·032) and 24 h (P = 0·036) and protocatechuic acid excretion (Fig. 2(b)) was greater at 24 h (P = 0·038) post-Champagne wine intervention compared with control. Isoferulic acid, which was present at very low concentration in baseline urine, increased significantly only following Champagne wine consumption and was significantly different from control at 24 h (P = 0·039) and 32 h (P = 0·011) post-intervention (Fig. 2(c)). Although there was a tendency for excretion to be increased following Champagne wine intervention, there were no significant differences in the mean total excretion of homovanillic acid (Champagne wine, 8·04 (sem 1·51) mg; control, 7·07 (sem 1·73) mg), homovanillyl alcohol (Champagne wine, 11·35 (sem 1·91) mg; control, 9·15 (sem 1·89)) or 3,4-dihydroxyphenylacetic acid (Champagne wine, 18·74 (sem 3·58) mg; control, 21·34 (sem 4·98) mg).

Fig. 2 Variation of the concentration of the urinary metabolites assessed by HPLC after Champagne wine (–■–) or placebo (- -●- -) consumption: (a) hippuric acid; (b) protocatechuic acid; (c) isoferulic acid; (d) homovanillic acid; (e) homovanillyl alcohol; (f) 3,4-dihydroxyphenylacetic acid (DOPAC). Values are means, with standard errors represented by vertical lines. † Mean value was significantly different from that following placebo intake (P < 0·05).
Biochemical markers
A significant increase in TAG concentrations was observed 6 h post-consumption of both Champagne wine and control, although there was no significant difference in the magnitude of change induced by the two interventions (Table 2). No significant changes in glucose, total cholesterol, HDL-cholesterol or LDL-cholesterol were observed post-Champagne wine or control consumption. All endothelial markers (endothelin-1, total NO∙/nitrite/nitrate) revealed values within a normal healthy range but did not change significantly following either intervention (Table 2). Liver enzyme levels (alanine aminotransferase, aspartate aminotransferase, γ-glutamyl transferase) did not show any statistical changes in response to either intervention. All biochemical markers were within the expected healthy range.
Table 2 Time course of biochemical parameters after either Champagne wine or placebo intake
(Mean values with their standard errors)

γGT, γ-glutamyl transferase; AST, aspartate aminotransferase; ALT, alanine aminotransferase, CRP, C-reactive protein, cTAC, corrected total antioxidant capacity.
Mean value was significantly different from that at baseline (0 h): * P < 0·05, *** P < 0·001.
Oxidative status and inflammatory markers
Intervention with both the control and Champagne wine led to increases in ‘total oxidant capacity’ (TOC) over the 6 h period immediately post-consumption, reflecting increases in endogenous peroxide production (P < 0·001; Fig. 3). In general, this increase in TOC was greater following the control intervention and was found to be significantly lower in the Champagne wine group at 6 h post-intervention (11 % reduction relative to placebo; P < 0·01) (Fig. 3). In contrast, there were no differences in ‘total antioxidant’ levels (corrected TAC) following either intervention (Table 2). Furthermore, there were no significant alterations in serum levels of C-reactive protein following either intervention.

Fig. 3 Variation of the total oxidative capacity over time after Champagne wine (–■–) or placebo (- -●- -) consumption. Values are means, with standard errors represented by vertical lines. Champagne wine demonstrated a significant decrease in the total oxidant capacity 6 h post-consumption when compared with placebo. †† Mean value was significantly different from that following placebo intake (P < 0·01).
Matrix metalloproteinase and tissue inhibitor of metalloproteinase levels
Gelatin–zymography analysis and ELISA revealed a stable concentration of MMP-2 at 1, 6 and 23 h post-intervention with Champagne wine and control (Fig. 4). In contrast, MMP-9 significantly decreased 1 h post-Champagne wine consumption (38·1 (sem 6·3) %; P < 0·05) but not following the control intervention (Fig. 4(a) and (d)). No significant modifications in the concentrations of TIMP-1 and TIMP-2 were observed (Fig. 4(b), (e) and (f)).

Fig. 4 Evaluation of matrix metalloproteinase (MMP) (a) and tissue inhibitor of metalloproteinase (TIMP) (b) by gel zymography. (a) Gelatin zymogram showing diminution of MMP-9 in blood 1 h after Champagne wine consumption. Human MMP-2 and MMP-9 were loaded as standards. (b) Reverse zymogram gel showing no significant differences after Champagne wine or placebo consumption. Evaluation of (c) MMP-2, (d) MMP-9, (e) TIMP-1 and (f) TIMP-2 concentrations by ELISA after Champagne wine () or placebo (□) consumption. Samples were studied in duplicate. Data are expressed as percentage of control (baseline; 30 min before Champagne wine or placebo intake). Values are means, with standard deviations represented by vertical lines. * Mean value was significantly different from that at baseline (30 min before Champagne wine or placebo intake) (P < 0·05).
Discussion
Many epidemiological studies have suggested that a daily and moderate consumption of red wine is associated with a lower incidence of CVD(Reference Klatsky, Armstrong and Friedman32–Reference St Leger, Cochrane and Moore34). In agreement with this, previous studies have indicated that red wine consumption significantly improves enthothelial function(Reference Fitzpatrick, Hirschfield and Coffey8) and that these effects can be, in part, attributed to its polyphenol content(Reference Dell’ Agli, Busciala and Bosisio35). Many of the effects of red wine are compatible with the action of wine-derived polyphenols on endothelium-derived NO∙ production, implying that NO∙ might be a mediator for their vascular actions(Reference Andriambeloson, Magnier and Haan-Archipoff36, Reference Flesch, Schwarz and Bohm37). Indeed, a rapid activation of endothelial NO synthase and endothelium-dependent vasodilatation has been reported for grape-derived polyphenols in vitro (Reference Anselm, Chataigneau and Ndiaye38) and a single dose of red wine has been shown to increase NO∙ production(Reference Matsuo, Nakamura and Takahashi39) and endothelium-dependent dilation(Reference Vlachopoulos, Tsekoura and Tsiamis40, Reference Bau, Bau and Naujorks41) in healthy volunteers. There is also evidence that white wine and Cava (sparkling white wine) may exert vascular actions. It has been suggested that such effects may result from the synergistic actions of polyphenols and other phenolic constituents on LDL oxidation and platelet function(Reference Natella, Nardini and Belelli42–Reference Pignatelli, Ghiselli and Buchetti44). In the present study, we show that Champagne wine consumption is capable of inducing acute vascular effects and in modifying levels of specific vascular active components.
The consumption of either Champagne wine or the alcohol control induced a rapid increase in endothelium-dependent vasodilatation, which returned to basal levels after 8 h. These observations are in agreement with previous studies which indicate that moderate alcohol is capable of inducing an acute increase in blood flow in an endothelium-dependent manner(Reference Agewall, Wright and Doughty6, Reference Hashimoto, Kim and Eto7, Reference Vlachopoulos, Tsekoura and Tsiamis40, Reference Bau, Bau and Naujorks41, Reference Tawakol, Omland and Creager45). However, we found that only the Champagne wine intervention was capable of significantly inducing an increase in endothelium-independent vasodilatation, which was maintained up to 8 h post-consumption. These data suggest that moderate Champagne wine consumption may enhance microvascular blood flow for a sustained period, through maintenance of local NO levels, in this case delivered via iontophoresis. Our data also suggest that this effect may be mediated by absorbed Champagne wine polyphenols, the metabolites of which (hippuric acid, isoferulic acid and protocatechuic acid) were detected in urine following Champagne wine ingestion. These metabolites are known to derive from the bacterial metabolism of caffeic acid and other hydroxycinnamates in the large intestine(Reference Rechner, Spencer and Kuhnle46). Whilst urinary hippuric acid may derive from aromatic amino acids, its increased excretion following Champagne wine ingestion indicates that absorption and metabolism of Champagne wine phenolic compounds, such as caffeic acid, had occurred post-consumption(Reference Rechner, Spencer and Kuhnle46). In support of this, the increased excretion of isoferulic acid after Champagne wine consumption is a specific biomarker of caffeic acid absorption, as this metabolites is only derived from the 4-methoxylation of caffeic acid by catechol-O-methyltransferase(Reference Mannisto and Kaakkola47) before or after its absorption(Reference Rechner, Spencer and Kuhnle46). Furthermore, the majority of urinary isoferulic acid has been shown to result from the cleavage of the caffeoyl quinic acid derivative of caffeic acid(Reference Scalbert and Williamson10, Reference Rechner, Kuhnle and Bremner48). Protocatechuic acid, which also increased in response to Champagne wine intervention, has previously been detected in human plasma following red wine consumption(Reference Caccetta, Croft and Beilin49) and has been identified as the urinary product of hydroxycinnamate ingestion from various food sources(Reference Choudhury, Srai and Debnam50, Reference Olthof, Hollman and Buijsman51).
The presence of such metabolites in urine between 6 and 32 h suggests that caffeic acid and other phenolic metabolites are absorbed into the circulation following Champagne wine consumption. These phenolic metabolites may affect vascular function by improving local NO bioavailability by two potential mechanisms. First, they may increase the local half-life of NO∙ via reaction with reactive oxygen species, such as superoxide(Reference Klotz and Sies52–Reference Radi, Peluffo and Alvarez54). In support of this, we observed a significant reduction in the ‘total oxidant capacity’ following Champagne wine intake, indicating that Champagne wine intervention leads to a reduction in plasma oxidant levels relative to control. Second, phenolic metabolites, such as those excreted post-Champagne wine consumption, may mimic NADPH oxidase inhibitors(Reference Halliwell55–Reference Steffen, Schewe and Sies57), such as apocynin (4′-hydroxy-3′-methoxyacetophenone), thereby reducing the cellular production of superoxide and increasing the half-life of NO∙, without any change in the rate of NO∙ synthesis(Reference Schewe, Steffen and Sies56). Indeed, previous studies have shown that the presence of an aromatic vicinal hydroxy-methoxy arrangement is highly effective in defining NADPH oxidase inhibition(Reference Schewe, Steffen and Sies56, Reference Steffen, Schewe and Sies57). Champagne wine phenolics such as tyrosol, hydroxytyrosol, ferulic acid and homovanillic alcohol have been shown to outcompete apocynin with respect to its inhibitory potency(Reference Steffen, Schewe and Sies57). The combined inhibition of NADPH oxidase and the scavenging of reactive oxygen species by phenolic metabolites would be expected to affect local NO∙ concentrations without influencing global endothelial NO production. Such effects may influence blood pressure, something which is supported by previous data showing that a low-molecular-weight fraction (1 kDa) of Champagne wine induces an anti-hypertensive effect in animals(Reference Perrot, Dukic, Charpentier, Lonvaud-Funel, Revel and Darriet58). Another study investigating the effect of olive oil phenolic components in rat aortic rings has demonstrated that caffeic acid is able to induce a vasorelaxant effect which persists in denuded aorta and in conjunction with NO synthase inhibitors such as NG-methyl-l-arginine (L-NMMA) or methylene blue(Reference Benkhalti, Legssyer and Gomez59). This suggests that the vascular effects induced by this phenolic may be mediated by the inhibition of Ca2+ channels and/or the blockage of the protein kinase C-mediated contractile mechanism, as has been observed for caffeic acid phenyl ester and sodium ferulate, respectively(Reference Chen, Ye and Li60, Reference Cicala, Morello and Iorio61). Further investigation is necessary to unravel the precise mechanism by which Champagne wine phenolics modulate endothelium-independent vasorelaxation in vivo.
The Champagne wine intervention also had a potentially beneficial effect on the vascular system in its ability to inhibit MMP-9. MMP and their specific tissue inhibitors (TIMP) play an important role in the physiological maintenance of the extracellular matrix and the pathogenesis of vascular disease(Reference Li, Feldman and Sun62, Reference Visse and Nagase63). For example, the over-expression of MMP-9 has been reported in atherosclerotic plaques(Reference Galis, Sukhova and Lark64) and has been linked with plaque rupture through its capacity to thin the protecting fibrous cap of the plaque(Reference Libby, Schoenbeck and Mach65, Reference Loftus, Naylor and Bell66). Individual red wine phenolics have been shown to inhibit gelatinase expression and/or activity(Reference Dell'Agli, Canavesi and Galli67). A transient inhibition of MMP-9 by Champagne wine phenolics could influence type IV collagen degradation and therefore improve basement membrane structural integrity. Although unlikely to have long-term implications on the vascular system, this acute inhibition of MMP-9 appears to be consistent with our other observations, as the ability of Champagne wine phenolics to reduce plasma oxidant formation would be expected to inhibit peroxynitrite formation, an oxidant known to activate MMP-9.
Its effects on endothelial-independent vascular reactivity may indicate that Champagne wine has the potential to improve ‘reactivity’ in the cutaneous microvasculature (arterioles, capillaries and venules). If so, it could reduce stiffening of the conduit arteries and a decline in arterial compliance, something which is observed with ageing, in hypertensive patients, in diabetics and those with cardio- and cerebrovascular disease(Reference Asmar, Pannier and Santoni68). Our findings may have wider significance in that attenuated cutaneous microvascular responses in heart transplant patients are paralleled by reduced responsiveness of coronary blood vessels(Reference Andreassen, Kvernebo and Jorgensen69). As such, our data suggest that moderate Champagne wine consumption may improve microvasculature blood flow and therefore vascular responsiveness generally. Further investigation will be necessary to determine whether acute, or indeed chronic, intake of Champagne has the potential to reduce CVD risk through its effects on microvascular responsiveness.
Acknowledgements
The authors wish to thank Ms Jan Luff, Ms Sofia Moran and Mr Anestis Dougkas for help with subject recruitment and study days. The authors are funded by the Biotechnology and Biological Sciences Research Council (BB/F008953/1; BB/E023185/1; BB/G005702/1).
J. P. E. S., D. V. and J. A. L. conceived of and designed the study. Whilst all authors played a role in data interpretation, the data were obtained as follows: E. J. H. and K. G. J, biochemical parameters; T. W. G. and E. J. H., LDI data; R. G., C. S. and P. G., MMP data. All authors were involved in manuscript preparation and read and approved the findings of the study. J. P. E. S. prepared the final manuscript.
The authors have no conflict of interest to disclose.