The small size for gestational age (SGA) neonate has reduced bone mass(Reference Akcakus, Koklu and Kurtoglu1), even after adjustment for body size(Reference Petersen, Gotfredsen and Knudsen2), compared with those born appropriate size for gestational age (AGA). The SGA neonate also has low arachidonic acid (AA) and DHA status at birth(Reference Cetin, Giovannini and Alvino3). Data from a variety of animal models clearly indicate that dietary n-3 and n-6 long-chain PUFA positively influence bone mass and metabolism(Reference Watkins, Yong and Allen4–Reference Coetzer, Claassen and van Papendorp7). However, such intervention has never been applied to the SGA neonate and since they are born with lower long-chain PUFA status(Reference Cetin, Giovannini and Alvino3) it is possible that a higher amount of supplementation is required.
The objective of the present study was to test for such benefits of long-chain PUFA in the SGA piglet that is known to have low bone mass at birth(Reference Adams8) and to establish if a dose–response relationship exists based on severity of growth restriction.
Experimental methods
Animals and diets
Thirty male Cotswold piglets born at term with a birth weight of ≤ 1·2 kg (SGA defined as ≤ 2 sd below the mean birth weight of 1·6 (sd 0·2) kg within sixteen litters) were obtained at age 3 d from the University of Manitoba. Piglets were identified as low birth weight (LBW; n 18; weight 1·1–1·2 kg) and very low birth weight (VLBW; n 12; weight ≤ 1·0 kg). At age 5 d, they were randomised within weight categories to one of three dietary treatments for 15 d: (1) control (unsupplemented) formula; (2) formula supplemented with AA and DHA as 0·6:0·1 g/100 g dietary fat; (3) formula supplemented with AA and DHA as 1·2:0·2 g/100 g dietary fat. Diets were liquid formula (350 ml/kg per d) for growing piglets(9) as previously published(Reference Mollard, Kohut and Zhao10). Both the AA (ARASCO®; 43·03 % AA) and DHA (DHASCO®; 42·95 % DHA) were from Martek Biosciences Corp. (Columbia, MD, USA).
Measurements
Weight was measured daily at 09.00 hours in the fasting state using a digital scale (Mettler-Toledo Inc., Highstown, NJ, USA). On day 16 in the non-fed state, heparinised blood samples and urine samples (U-Bag; Hollister Inc., Libertyville, IL, USA) were taken at 09.00 hours. Plasma and erythrocyte fractions were stored at − 80°C under N2 gas and urine at − 20°C until analysis. Piglets were then anaesthetised using sodium pentobarbital (Somnotol; MTC Pharmaceuticals, Hamilton, Ontario, Canada; 30 mg/kg intraperitoneal) followed by overdose (180 mg/kg intracardiac). A section of tibial diaphysis (1·0 g) was excised for measurement of PGE2 as previously described(Reference Blanaru, Kohut and Fitzpatrick-Wong11).
Total lipids were extracted from plasma and erythrocytes (membranes and cytosol) followed by methylation and gas chromatograph analysis as previously described(Reference Blanaru, Kohut and Fitzpatrick-Wong11). Plasma osteocalcin was measured using RIA (Diasorin, Inc., Stillwater, MN, USA) and urine cross-linked N-telopeptide was measured using ELISA (Osteomark; Ostex International, Seattle, WA, USA) with values corrected to creatinine (Sigma 555; Sigma-Aldrich Ltd, Oakville, ON, Canada). Plasma insulin-like growth factor-I was measured by ELISA (R&D Systems, Minneapolis, MN, USA) and ex vivo PGE2 production in bone organ culture was also measured with an ELISA (R&D Systems).
Piglet carcasses were analysed for bone mineral content (BMC) of the whole body and lumbar spine from L1 to L4 vertebrae using dual-energy X-ray absorptiometry (QDR 11.2 4500A series; Hologic Inc., Waltham, MA, USA) using infant whole-body and low-density lumbar spine software(Reference Blanaru, Kohut and Fitzpatrick-Wong11). To obtain ex vivo femur dual-energy X-ray absorptiometry scans, the right femur was subsequently dissected from each piglet carcass, cleaned of adherent soft tissue, measured for weight and length, then BMC was measured in a 3 cm water-bath to simulate soft tissue and standardise positioning(Reference Brunton, Weiler and Atkinson12).
For the VLBW group, following the dual-energy X-ray absorptiometry scan, the tibia was removed for fatty acid analysis(Reference Watkins, Yong and Allen4) of the proximal metaphysis (trabecular) both above and below the growth plate, proximal epiphysis articular cartilage, diaphysis (cortical), marrow, periosteum and muscle. Samples were not available for LBW piglets.
All experimental procedures were according to our published methods(Reference Blanaru, Kohut and Fitzpatrick-Wong11) and conformed to guidelines of the Canadian Council of Animal Care(13) and approved by the University of Manitoba Animal Care Committee.
Statistical analysis
Results are expressed as mean values and standard deviations unless otherwise stated. The level of statistical significance was set at P < 0·05. A factorial model ANOVA (diet, weight category) and post hoc Bonferroni tests were conducted using SAS (version 9.1; SAS Institute, Inc., Cary, NC, USA). For differences among the tibia long-chain PUFA in the VLBW piglets, a one-factor ANOVA with Bonferroni post hoc testing was used.
Results
Piglets in both birth-weight categories gained weight during the study and all formula offered was consumed. Final body weight (5·3 (sd 0·6) v. 3·9 (sd 0·7) kg; P < 0·0001) and length (54·3 (sd 2·2) v. 49·4 (sd 2·6) cm; P < 0·001) were lower in VLBW piglets, but did not vary according to diet groups. No effect of diet was observed in whole-body and femur BMC, but values in VLBW piglets were 26 to 35 % lower than those of the LBW piglets (Table 1). For lumbar spine BMC, an interaction revealed that LBW piglets supplemented 0·6 % AA and 0·1 % DHA had higher values than control, but that VLBW piglets required 1·2 % AA and 0·2 % DHA to elevate lumbar spine BMC.
Table 1 Bone mass and biomarkers of bone metabolism and fatty acid (FA) status in response to 15 d of dietary intervention with arachidonic acid (AA) and DHA in piglets born at low birth weight (LBW) and very low birth weight (VLBW)
(Mean values and standard deviations)
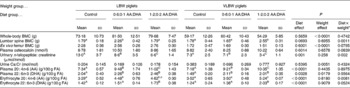
BMC, bone mineral content.
a,b,c For interaction effects, mean values within a row with unlike superscript letters were significantly different (P < 0·05).
A,B,C For the main effect of diet, mean values within a row with unlike superscript letters were significantly different (P < 0·05; post hoc testing).
* No post hoc testing required for main effect of the two weight groups. Sample size is six animals per group for LBW piglets and four animals per group for VLBW piglets.
Urinary cross-linked N-telopeptide was lower with the higher supplementation and the VLBW piglets had lower values compared with LBW piglets while osteocalcin was not affected by diet or weight category (Table 1). Urinary Ca excretion was not affected by diet, but was higher in the VBLW piglets compared with LBW piglets. There were no dietary, birth weight category or interaction effects for plasma insulin-like growth factor-1 (10·8 (sd 4·0) nmol/l; n 30) or release of PGE2 from bone (4·3 (sd 1·8) ng/g bone; n 30).
A main effect of diet was observed in a dose–response manner for plasma AA and DHA and a main effect of weight category showed higher DHA in LBW piglets (Table 1). Erythrocyte DHA was higher in the 1·2:0·2 % AA:DHA group compared with the other groups while for erythrocyte AA, an interaction revealed that the higher supplementation was required to elevate values in the VLBW group.
For tibia fatty acid analysis of VLBW piglets, the only significant effects observed were for muscle and trabecular bone compartments whereby the 1·2:0·2 % AA:DHA group had higher DHA compared with control (Fig. 1).
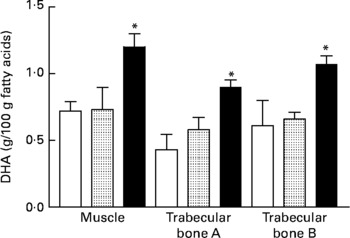
Fig. 1 DHA in tibial muscle and bone from very-low-birth-weight piglets fed a control diet (□; n 3) or a diet with additional arachidonic acid and DHA in a ratio of 0·6:0·2 (; n 3) or 1·2:0·2 (■; n 4) % of dietary fat. Trabecular bone A, trabecular bone above the growth plate; trabecular bone B, trabecular bone below the growth plate. Values are means, with standard errors represented by vertical bars. * Mean value was significantly different from that of the control group (P ≤ 0·03).
Discussion
The primary objective of the present study was to determine the skeletal response in growth-restricted piglets to dietary supplementation with AA and DHA at two different levels over 15 d in early neonatal life. The amount of long-chain PUFA required to enhance the BMC of the lumbar spine varied as a function of birth weight, with the VLBW piglets requiring twice the amount to elicit the response compared with LBW piglets. Since vertebral bone is predominantly trabecular, the data suggest that trabecular bone sites were more affected by dietary AA and DHA than more cortical bone sites, such as the femur, in the SGA neonate. Data from other animal studies indicate a heightened sensitivity of trabecular bone sites to dietary manipulation. A Ca-deficient diet fed to young rats was associated with decreased bone density in tibia trabecular bone, but not cortical bone, in as little as 24 h(Reference Seto, Aoki and Kasugai14), and in growing piglets leads to reduced trabecular bone volume and mineral apposition rate(Reference Eklou-Kalonji, Zerath and Colin15). Similarly, protein restriction (5 v. 20 % protein) in pregnancy to induce SGA rat pups reduces Ca of the trabecular-rich calvarium, but not the femur(Reference Gudehithlu and Ramakrishnan16). It is therefore conceivable that if trabecular bone was relatively more affected by an adverse intra-uterine environment than cortical bone, its responsiveness to subsequent nutritional repletion may be heightened. Indeed, the values for spine BMC and tibia trabecular bone DHA of the smallest piglets were elevated if fed the highest amount of supplement.
As reported previously in AGA piglets(Reference Blanaru, Kohut and Fitzpatrick-Wong11), supplementation of AA and DHA did not alter osteocalcin in our SGA piglets. Our values were lower relative to published values for AGA (16·2 nmol/l) piglets(Reference Blanaru, Kohut and Fitzpatrick-Wong11), signifying reduced bone formation in the SGA piglet. In contrast, bone resorption was suppressed by dietary AA and DHA below that typical of AGA piglets (10·7 μmol bone collagen equivalents/mmol creatinine)(Reference Blanaru, Kohut and Fitzpatrick-Wong11). These data corroborate with rodent studies of dietary n-6 and n-3 long-chain PUFA that caused enhanced bone mass and reduced bone resorption(Reference Claassen, Coetzer and Steinmann5, Reference Watkins, Li and Seifert17).
The prominent dose-dependent elevation of both AA and DHA in the piglets agrees with studies in the rat(Reference Danon, Heimberg and Oates18) and hamster(Reference Whelan, Surette and Hardardottir19). The higher requirement for AA by the VLBW piglets may reflect increased requirements for preformed AA in severely growth-restricted piglets to compensate for low stores, even if enzymic capacity to produce AA and DHA is normal(Reference McNeil, Finch and Page20). The fact that erythrocyte DHA, but not AA, was enriched equally by both supplements is a reasonable observation since AA is required for the growth of all tissues and DHA predominantly in the brain and retina.
In summary, the amount of long-chain PUFA supplementation needed to enhance bone mass was modulated by birth-weight classification, implying that severely growth-restricted mammals may benefit from additional long-chain PUFA supplementation. Further investigation in the SGA piglet model is necessary to delineate the underlying mechanisms and to clarify the long-term impact of dietary AA and DHA intervention on bone and other health outcomes.
Acknowledgements
This research was funded by a grant (H. W.) and scholarship (J. K.) from the Natural Sciences and Engineering Research Council of Canada. Each author contributed significantly to the present study. J. K. and H. W. designed and executed the study. B. W. measured bone fatty acids. All authors contributed to the interpretation of the data and writing of the report.
We have no conflict of interest.