Physiological stress, such as exercise, leads to oxidative stress that is associated with reactive oxygen species (ROS) and free radicals(Reference Bloomer, Goldfarb and Wideman1). Oxidative stress occurs as the cellular generation of pro-oxidants exceeds the physiological capacity to eliminate them, which involves the endogenous antioxidant mechanism as well as exogenous antioxidants obtained via diet(Reference Suzuki2). Evidence suggests that nutritional supplements containing antioxidants including glutathione, vitamins E, A and C, proteins and taurine are safe for human consumption (rare side effects) and can have a positive influence on exercise performance and the reduction of exercise-related oxidative stress(Reference Asadi, Arazi and Suzuki3,Reference Bobeuf, Labonte and Dionne4,Reference Calder5,Reference Arazi, Hosseini and Asadi6,Reference Lee, Lin and Lin7,Reference Takahashi, Suzuki and Kim8,Reference Thirupathi, Pinho and Baker9,Reference Gonzalez and Trexler10,Reference Gonzalez, Church and Townsend11) . Thus, the assessment of dietary compounds with antioxidant properties is a vital line of research for the development of different nutritional interventions to help diminish ROS production and oxidative stress after exercise.
l-Citrulline (l-Cit) is a non-protein and non-essential amino acid with antioxidant properties(Reference Allerton, Proctor and Stephens12) that is well known as the precursor of l-arginine in the urea cycle(Reference Stanelle, McLaughlin and Crouse13). Dietary l-Cit enters the kidneys, vascular endothelial cells and other tissues which leads to a rise in plasma and tissue l-arginine levels. l-Arginine is the substrate for endothelial production of nitric oxide (NO), a strong vasodilator(Reference Stanelle, McLaughlin and Crouse13,Reference Papadia, Osowska and Cynober14,Reference Figueroa, Wong and Jaime15) . Animal research and tentative human evidence also show l-Cit as an anabolic pharmaconutrient that has a beneficial impact on protein synthesis; it is thought that its contribution is mediated by the mechanistic target of rapamycin pathway(Reference Stanelle, McLaughlin and Crouse13,Reference Papadia, Osowska and Cynober14,Reference Rhim, Kim and Park16,Reference Da Silva, Jacinto and De Andrade17) . Recently, Gonzalez et al. (Reference Gonzalez and Trexler10) indicated an increase in athletic performance and recovery with a decrease in muscle damage following l-Cit supplementation. Moreover, Stanelle et al. (Reference Stanelle, McLaughlin and Crouse13) reported that l-Cit supplementation might lead to a slight improvement in the cycling performance of trained athletes. It was also shown that, due to exercise-induced muscle damage, l-Cit supplementation can decrease serum creatine kinase concentrations along with increasing perceived recovery(Reference Stanelle, McLaughlin and Crouse13,Reference Terasawa and Nakada18) . According to prior research, l-Cit supplementation reduces concentrations of lactate and muscle pain 24 h after exercise(Reference Stanelle, McLaughlin and Crouse13,Reference Rhim, Kim and Park16) , which might be related to its antioxidant effects. Furthermore, it has been previously reported that l-Cit supplementation efficiently increases NO metabolites(Reference Ochiai, Hayashi and Morita19,Reference Wong, Alvarez-Alvarado and Jaime20) . However, limited studies have found whether acute ingestion of l-Cit alters the response of antioxidant markers after a single bout of exercise(Reference Sureda, Cordova and Ferrer21). Therefore, the purpose of this study was to evaluate the effect of 12 g l-Cit supplementation on antioxidant markers after acute high-intensity interval exercise in young men. We hypothesised that acute l-Cit supplementation would enhance our primary outcome of blood antioxidant markers and secondary outcome of NO metabolites after a session of high-intensity interval exercise.
Experimental methods
Participants
Nine trained young men (see participant’s characteristics in Table 1) from the University of Guilan were recruited for this study. The participants were familiar with resistance and endurance exercises and trained 5 times/week (≥90 min/session) for at least 6 months prior to the present study. Inclusion criteria were (1) healthy and free of any injury; (2) no history of surgery and musculoskeletal disorders for 2 years prior to the study and (3) no history of ergogenic aids, supplements and drug/medication use for at least 6 months before the study. All participants were made aware of the research procedures and gave written consent ahead of data collection. This study was authorised by the ethical review board and the university research council (IR.GUMS. RES1397/428), registered at the Iranian Registry of Clinical Trials (IRCT20210323050758N1) and conducted in the Exercise Physiology Laboratory of the University of Guilan in accordance with the Declaration of Helsinki II.
Table 1. Participant’s characteristics
(Mean values and standard deviations, n 9)

Study design
This study was performed using a random sampling, double-blind, crossover and placebo (PL)-controlled design. Overall, participants visited the laboratory three times. Anthropometrics (height, body mass and body fat percentage) were assessed during an initial familiarisation visit for participants, which included a review of study procedures and exercise techniques. In the second visit (a week following the first visit), the participants were randomly allocated into either an acute l-Cit (12 g) or PL supplementation trials that were subsequently completed. After the second visit, there was a 1-week washout period before the third visit, in which participants performed the other trial (crossover approach, Fig. 1). Blood samples were obtained from the brachial vein at baseline (PRE, 15 min before exercise), immediately after exercise (IP), at 10 (10P) and 30 min (30P) post-exercise to assess serum concentrations of the antioxidant markers including superoxide dismutase (SOD), glutathione peroxidase (GPx), catalase (CAT) and NO. The participants were asked to refrain from any exercise for at least 48 h and to avoid caffeinated beverages at least 12 h prior to each visit. The study schematics are illustrated in Fig. 1.

Fig. 1. Study design. Supplementation of placebo or l-citrulline 1 h prior to blood sampling at pre, immediately post, 10 and 30 min post-exercise.
Procedures
Randomisation and blinding
Randomisation was carried out by utilising a random number table; for this, an independent coordinator, not otherwise involved in the study, created the allocation sequence assigning participants to the l-Cit or PL trials. The independent coordinator also provided and witnessed supplement consumption. Both the participants and research team members were blinded to the treatment allocation until the database was unlocked and data analysis was completed.
Anthropometric measures
Height was measured using a wall-mounted stadiometer with an accuracy of 0·1 cm (Seca 222). Body mass was measured using a scale (Camry, FB9003) with a precision of 0·1 kg. Body fat percentage was acquired from the skinfold thickness of three different points (i.e., pectoral, quadriceps and abdominal) on the right side of the body using a calliper (Lafayette calliper, model 01128). All measurements were calculated based on Jackson Pollack’s body fat percentage equations(Reference Wong, Nordvall and Walters-Edwards22).
l-Citrulline and placebo supplementations
One hour before exercise, the participants consumed either 12 g of l-Cit powder dissolved in 200 ml of water (l-Cit 1200 mg, NOW® Sports) or PL (12 g of maltodextrin) with the same appearance, taste, smell and colour. The selected dose was based on previous studies that showed increases in plasma l-Cit and NO metabolites levels without the negative effects of saturation(Reference Sureda, Cordova and Ferrer21,Reference Moinard, Nicolis and Neveux23) . We used a 1-week washout period between acute l-Cit and PL supplementations, which was based on a previous study(Reference Papadia, Osowska and Cynober14).
Acute high-intensity interval exercise
Before initiation of the exercise protocol, the participants performed a 10-min warm-up, which consisted of 5-min cycling (with the minimal workload, 50–60 % of maximum heart rate) and 5-min dynamic stretching (total body) exercise. Thereafter, participants performed twelve consecutive rounds of the two-hand kettlebell swing exercise including (30 s of exercise and 30 s of rest) using a 16-kg kettlebell (Wirecutter), a weight that has been shown to induce significant metabolic and cardiorespiratory stress and seems to be standardised in prior kettlebell exercise research(Reference Budnar, Duplanty and Hill24). The swing exercises were performed at a rate of 1-s eccentric/1-s concentric phase (15 swings per round) and were controlled by using a metronome(Reference Wong, Nordvall and Walters-Edwards22). The participants were verbally encouraged, and feedback was given on their performance by an experienced trainer.
Blood sampling and analysis
Blood samples (8 ml) were obtained from the brachial vein in a seated position and then evacuated in test venoject tubes. The blood was clotted at room temperature for 30 min and centrifuged at 1500 × g for 10 min. To further analyse, the serum layer was removed and frozen in various aliquots at −70°C. The serum concentrations of SOD, GPx, CAT and NO metabolites (NOx) (circulating levels of nitrite + nitrate) were measured by existing commercial ELISA kits (Zellbio GmbH Veltliner). The intra- and inter-assay CV for all blood measurements were <7 %.
Statistical analysis
All participants who completed the study were included in the data analysis. Statistical analysis was conducted using SPSS 24 for Windows (SPSS Inc.). Estimation of an appropriate sample size was conducted using the G*Power analysis software(Reference Faul, Erdfelder and Lang25). Our rationale for sample size was based on a previous study evaluating changes in blood NOx responses to high-intensity exercise after acute l-Cit supplementation in nine young men(Reference Terasawa and Nakada18,Reference Wiecek, Szymura and Maciejczyk26) . This study revealed an effect size of 0·4 for the increase in NOx after high-intensity exercise. A total sample size of nine participants was determined with an effect size of 0·4 and 80 % power at the predetermined level of α = 0·05. It was relevant to have sufficient power to detect changes in NOx as an enhanced NO bioavailability leads to increases in blood flow, which in turn eliminates metabolites such as H+ and free radicals from muscle tissue and may improve antioxidant production(Reference Allerton, Proctor and Stephens12). Data were expressed as mean values and standard deviation. The normality of data was examined and confirmed using the Shapiro–Wilk test. For analysis of SOD, CAT, GPx and NO data, repeated-measures ANOVA (2 × 4, group × time) was used (SPSS 21.0). Bonferroni post hoc test was used to change the different measurement steps after a significant F value. In each variable, for 1 % of the difference, the difference between the basic time and all subsequent time points was calculated. An independent t test was used between groups, and the standard trapezoidal method to the AUC was assessed for dependent variables. Significance levels were considered at P ≤ 0·05.
Results
Between 6 and 23 December 2018, we screened twelve trained young men. Of these, nine qualified for baseline evaluation and were subsequently randomised to either the l-Cit or PL trials. After randomisation, no participants dropped out of the study and no harm or unintended effects were reported. Data are presented for the nine participants who successfully completed our study protocol (Fig. 2). At baseline concentrations, no significant differences were observed in the dependent variables between the two conditions (i.e., SOD, GPx, CAT and NO) (P > 0·05).
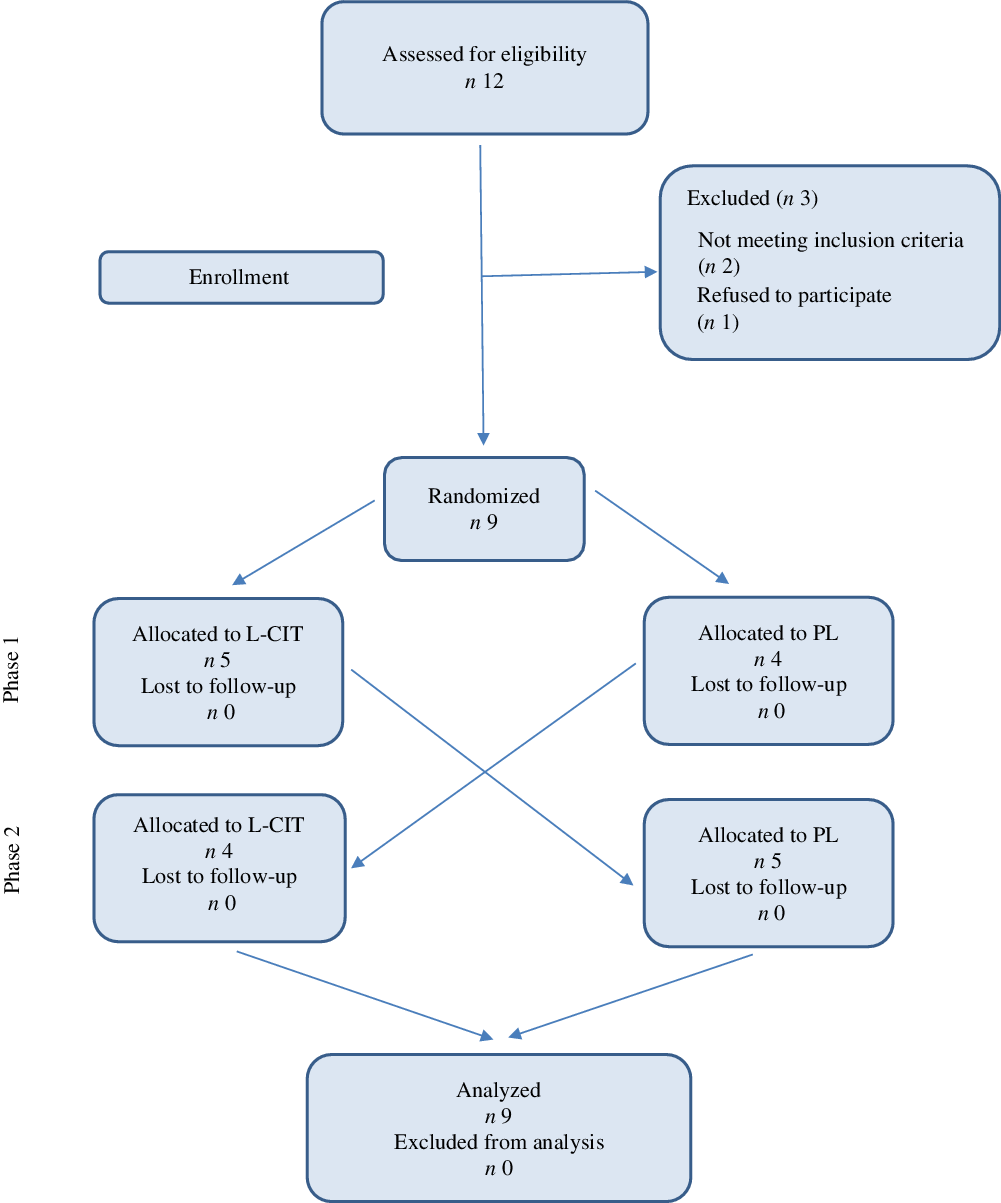
Fig. 2. Participants’ flow diagram.
There was no significant interaction for group × time for SOD (F = 1·02, P = 0·39). Only the l-Cit showed a significant increase in SOD from PRE to 10P (P = 0·01). The AUC analysis for SOD did not show a significant difference (P = 0·13) between the conditions. Due to the % change in SOD, the l-Cit showed more changes than PL group in PRE to 10P (P = 0·042, −29 %, 15·3 U/ml, 95 % CI (−0·284, 30·882)) (Fig. 3)).

Fig. 3. Changes in superoxide dismutase (SOD) levels after exercise. Values are mean values and standard deviations. l-Cit, l-Citrulline; PL, placebo. *Significant differences compared with PRE, **Significant differences compared with PL. PRE: before, IP: immediately after, 10P: 10 min after exercise, 30P: and 30 min after exercise.
No significant interaction was noted for group × time for GPx (F = 0·24, P = 0·86). The l-Cit group showed a slight (3 %, 95 % CI (−22·449, 28·989)) but significant increases in GPx at 10P compared with PRE (P = 0·048). The AUC analysis for GPx showed no noticeable difference (P = 0·58) between groups. Based on % change in GPx, the l-Cit group indicated higher changes than PL group in PRE to 10P (P = 0·05, Fig. 4).

Fig. 4. Changes in glutathione peroxidase (GPx) levels after exercise. Values are mean values and standard deviations. l-Cit, l-Citrulline; PL, placebo. *Significant differences compared with PRE, **Significant differences compared with PL. PRE: before, IP: immediately after, 10P: 10 min after exercise, 30P: and 30 min after exercise.
No significant interaction was seen for group × time for CAT (F = 0·24, P = 0·86). Both the l-Cit and PL conditions showed a significant increase in CAT at IP (P = 0·014) and did not show any significant difference (P = 0·69) between the conditions. Due to the % change in CAT, the PL revealed more changes than the l-Cit group in PRE to IP (P = 0·023, −33 %, 0·84 U/ml, 95 % CI (−0·107, 1·787)) and PRE to 10P (P = 0·036, −45 %, 1·1 U/ml, 95 % CI (−0·005, 2·205)). In contrast, the l-Cit showed more changes than PL group in IP to 30P (P = 0·041) and 10P to 30P (P = 0·039, Fig. 5).

Fig. 5. Changes in catalase (CAT) levels after exercise. Values are mean values and standard deviations. l-Cit, l-Citrulline; PL, placebo. *Significant differences compared with PRE, **Significant differences compared with PL. ***Significant differences compared with l-Cit PRE: before, IP: immediately after, 10P: 10 min after exercise, 30P: and 30 min after exercise.
There was no noticeable interaction for group × time for NO (F = 1·8, P = 0·17). The l-Cit group showed a small (−4 %, 0·44 Umol/l, 95 % CI (−0·553 to 1·433)) but significant increase in NO at IP compared with PRE (P = 0·05). The AUC analysis for NO showed a significant difference (P = 0·041) between the conditions. Based on the % change in NO, the l-Cit group revealed higher changes than PL group in PRE to IP (P = 0·013, −4·5 %, 0·48 Umol/l, 95 % CI (−0·625, 1·584)), PRE to 10P (P = 0·04, −4 %, 0·44 Umol/l, 95 % CI (−0·454, 1·334)) and PRE to 30P (P = 0·042, −4 %, 0·41 Umol/l, 95 % CI (−0·288, 1·108)) (Fig. 6).
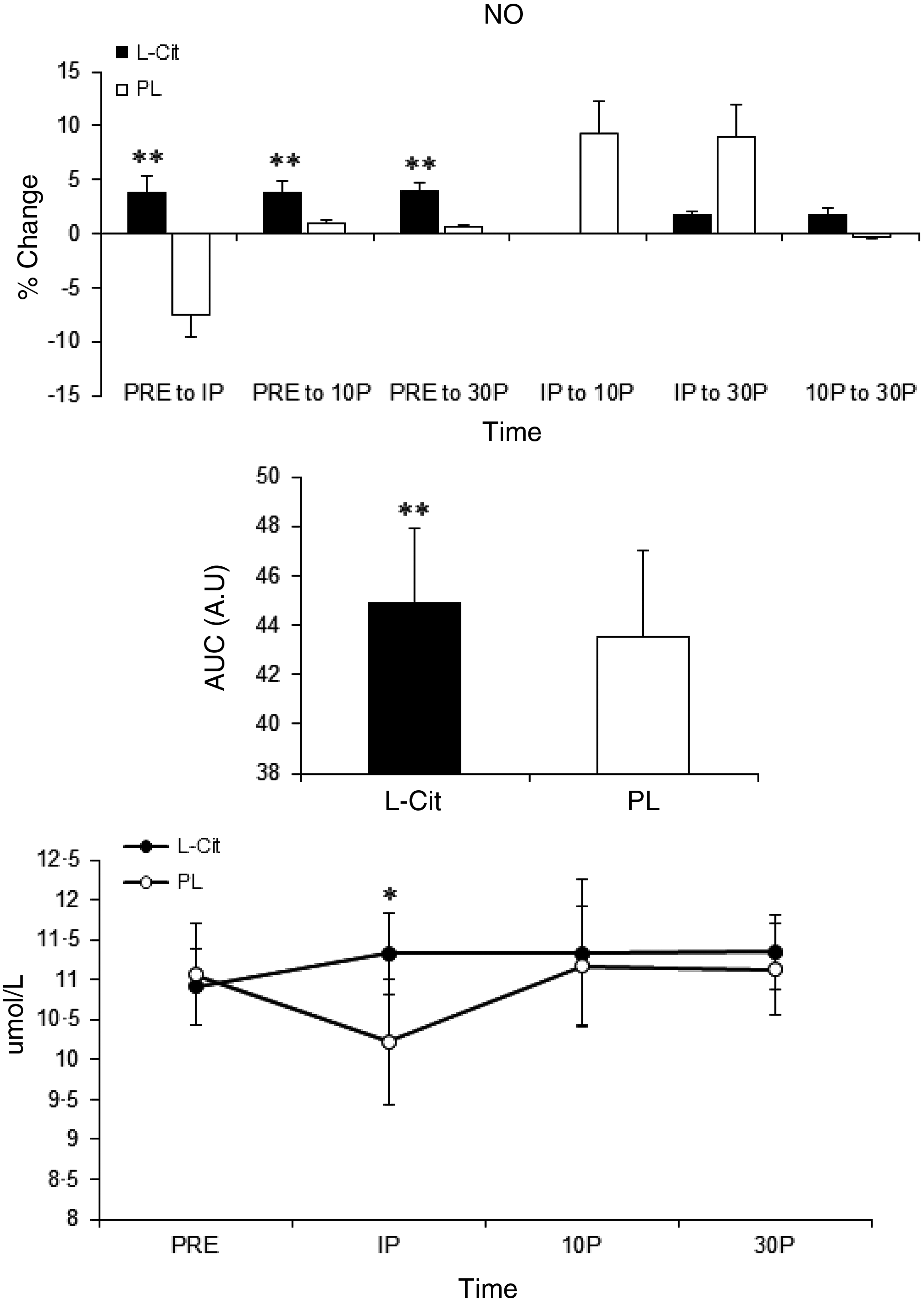
Fig. 6. Changes in nitrite + nitrate (NO) concentrations after exercise. Values are mean values and standard deviations. l-Cit, l-Citrulline; PL, placebo. *Significant differences compared with PRE, **Significant differences compared with PL. PRE: before, IP: immediately after, 10P: 10 min after exercise, 30P: and 30 min after exercise.
Discussion
The major findings of our study are that l-Cit supplementation induced significant changes to antioxidant markers in response to an acute bout of high-intensity interval exercise in young men. Our results were in line with our hypothesis that acute l-Cit supplementation (12 g) an hour prior to high-intensity interval exercise would significantly increase antioxidant markers compared with the PL condition. To the best of our knowledge, this is the first study to investigate the effects of l-Cit supplementation on antioxidant markers following acute high-intensity interval exercise in humans.
The effects of l-citrulline supplementation on the antioxidant and nitric oxide response to exercise
Higher concentrations of l-arginine are transported into the cell during physical activity to sustain adequate amounts of this amino acid and allow for its optimum coupling with inducible NO synthase. As a result of the ability to restrict NO activity in the absence of sufficient l-arginine, inducible NO synthase will primarily use O2 to form O2 .-, possibly resulting in oxidative stress. Although the fact of the antioxidant properties of l-Cit was first discovered in leaves of drought-tolerant wild watermelon plants(Reference Kawasaki, Miyake and Kohchi27), our current knowledge of the role of l-Cit and inducible NO synthase in the regulation of skeletal muscle antioxidant defence mechanisms is insufficient and further studies are needed(Reference Ham, Gleeson and Chee28). The primary aim of this research was to assess whether the l-Cit supplementation would improve the antioxidant markers and NOx concentrations in response to high-intensity interval exercise in young men. In the current study, the findings showed increased serum concentrations of SOD, GPx and CAT after exercise in the l-Cit trial. In line with our results, da Silva et al. (2014)(Reference da Silva, Tromm and Bom29) indicated that taurine supplementation increases SOD, GPx and CAT concentrations following eccentric exercise(Reference da Silva, Tromm and Bom29). In contrast, Lee et al. (2020)(Reference Lee, Pekas and Lee30) showed no changes in SOD and GPx concentrations following 4 weeks of high-intensity exercise combined with aspirin consumption. It seems that disparities in exercise and trial duration (acute v. longitudinal), as well as supplementation type, are probable reasons for these inconsistencies(Reference Lee, Pekas and Lee30). Antioxidant defensive systems are in control of scavenging ROS in order to avoid oxidative stress. The main antioxidant molecules in the antioxidant defence are SOD, GPx and CAT. SOD converts superoxide anions to less harmful hydrogen peroxide, which CAT and GPx then further eliminate. Antioxidative enzyme production is a response to ROS activities that commonly occurs in aerobic exercise(Reference Patlevič, Vašková and Švorc31,Reference Kawamura and Muraoka32) . l-Cit increases blood flow as well as enhances NO bioavailability, which in turn eliminates metabolites such as H+ and free radicals from muscle tissue and eventually improves antioxidative production(Reference Allerton, Proctor and Stephens12). Indeed, our findings suggest that l-Cit supplementation before exercise can be an effective antioxidant agent that enhances SOD, GPx and CAT through its antioxidant properties. In fact, l-Cit has been shown to have a protective effect against ROS and oxidative stress, which can be attributed to its antioxidant capacity(Reference Allerton, Proctor and Stephens12). However, this area is relatively unexplored, and further studies are needed to clarify the beneficial effects of l-Cit supplementation on antioxidant enzyme activity following physical exercise.
Our results indicate that NOx concentrations increased significantly in the l-Cit group after exercise. These findings are consistent with the hypothesis of the study as well as previous researchers who have reported significant improvements in NOx concentrations following l-Cit supplementation(Reference Schwedhelm, Maas and Freese33,Reference Bloomer34) . Also, our findings are in line with the results of studies by McKinley et al. (2015)(Reference McKinley-Barnard, Andre and Morita35) and Fu et al. (2013)(Reference Fu, Li and Jia36). However, in another study by Hickner et al. (2015)(Reference Hickner, Tanner and Evans37), l-Cit supplementation following a graded exercise test did not cause any significant increase in NOx concentrations. The discrepancy in the type of exercise protocol and supplemental dose is probably reasons for this difference because participants consumed 3-g l-Cit over 3 h or 9-g l-Cit over 24 h prior to treadmill testing to exhaustion(Reference Hickner, Tanner and Evans37). It has been shown that l-Cit supplementation leads to an increase in l-arginine concentrations both at rest and during exercise in humans(Reference Rhim, Kim and Park16,Reference Hickner, Tanner and Evans37) . l-Arginine is the main substrate for NO synthesis as a blood flow modulator(Reference Bloomer34). Indeed, it has been reported that l-Cit supplementation not only may lead to an indirect increase in NO synthesis but also blood NO concentrations which rises the blood flow of active muscles(Reference Bloomer34). Accordingly, l-Cit supplementation is not only considered a precursor to l-arginine but is also a viable alternative to increasing plasma l-arginine concentrations for greater NO generation(Reference Schwedhelm, Maas and Freese33), which is consistent with the findings of the present study. Additionally, l-Cit may play an important role in preventing the oxidation of LDL, which leads to an improved endothelial dysfunction(Reference Napoli, Ackah and De Nigris38,Reference Morita, Sakurada and Watanabe39) .
The effect of high-intensity interval exercise on antioxidant enzymes and nitric oxide metabolites
It is clear that during high-intensity training, free radicals and ROS are continually produced by cells as part of metabolic activity, while an antioxidant defence system can neutralise both components(Reference Powers and Lennon40,Reference Matsuo, Kaneko and Radak41) . SOD and GPx are both vital for vascular tone and normal risk to cells. Throughout the exercise, SOD and GPx act in the cell as a progressive resistance to ROS generation and oxidative stress as a primary antioxidant defence mechanism against superoxide radicals(Reference Powers and Lennon40).
In this study, SOD concentrations did not show any significant change in the PL trial. It seems that high-intensity interval exercise has no effect on SOD and GPx concentrations. Our results support previous research that found high-intensity exercise has no effect on SOD and GPx concentrations on its own(Reference Saritaş, Uyanik and Hamurcu42,Reference Miyazaki, Oh-Ishi and Ookawara43) . According to the studies by Saritas et al. (2011)(Reference Saritaş, Uyanik and Hamurcu42) and Miazaki et al. (2001)(Reference Miyazaki, Oh-Ishi and Ookawara43), SOD concentrations in humans did not improve after exhausting exercise. In contrast to our findings, Parker et al. (2018)(Reference Parker, Trewin and Levinger44) showed a significant decrease in SOD concentrations response to a high-intensity interval exercise protocol in healthy adults. This seems to be due to the disparity between protocol and population, in which eight untrained men and women completed the high-intensity interval exercise protocol, which consisted of five 4-min bouts, and SOD concentrations were assessed 3 h after the exercise(Reference Parker, Trewin and Levinger44). In our study, the redox state was evaluated up to 30 min following exercise, which was based on previous research that showed significant differences in antioxidant markers (such as SOD and GPx) at this post-exercise time point(Reference Wiecek, Szymura and Maciejczyk26). However, we may not have captured optimum plasma oxidative stress and antioxidant function as total antioxidant capacity has been reported to peak around 2 h post-exercise(Reference Michailidis, Jamurtas and Nikolaidis45). In addition, due to the participants’ fitness levels, it appears that the variation in outcomes is most likely because SOD activity tends to assess the impact of exercise on redox haemostasis, and trained men with higher basal SOD activity have a lower risk of exercise-induced oxidative stress than untrained men with lower basal SOD activity. Similarly, in our study, there were no major differences in GPx concentrations in the PL condition. Wiecek et al. (2018)(Reference Wiecek, Szymura and Maciejczyk26) found significant differences in GPx concentrations after high-intensity exercise, which is contrast to our findings. In the current study, it can be observed that there was a significant increase in CAT and NOx concentrations in the PL condition. In line with our study, Rowinski et al. (2013)(Reference Rowiński, Kozakiewicz and Kędziora-Kornatowska46) reported that performing physical activities has a positive effect on antioxidant enzyme (i.e., CAT) activity in older adults. Similarly, other researchers have reported increased serum CAT concentrations after exercise(Reference Alessio and Goldfarb47,Reference Servais, Couturier and Koubi48,Reference Poprzecki, Klapcinska and Sadowska-Krepa49) which supports our findings. On the other hand, Djordjevic et al. (2012)(Reference Djordjevic, Cubrilo and Barudzic50) showed a significant decrease in CAT and NOx concentrations after acute exercise in young athletes. This disparity is likely due to a different type of exercise protocol and various characteristics of participants (age, fitness level and health). It seems that athletes have a significantly higher basal concentrations of CAT and NOx which provide for proficient elimination of excess exercise-produced H2O2 and consequently decline their concentrations after exercise in this population(Reference Djordjevic, Cubrilo and Barudzic50).
Study strengths and limitations
A key strength of the present research is its randomised, double-blind, crossover and PL-controlled design. Another strength of this work is that, to our knowledge, few studies have assessed the effect of l-Cit on antioxidant responses to exercise. Our research is limited by relatively small sample size, although our results reached statistical significance. Additionally, we were unable to measure plasma l-Cit/l-arginine concentrations due to a limited budget, yet prior studies have shown an increase in plasma l-Cit/l-arginine concentrations with doses of ≥6 g(Reference Sureda, Cordova and Ferrer21,Reference Moinard, Nicolis and Neveux23) . Moreover, our participants were young, trained men, and consequently our findings may not apply to other cohorts with dissimilar age, sex and training status. Even though the antioxidant changes found in the l-Cit were statistically significant compared with the PL trial, these remained modest and consequently further investigations are needed to establish the clinical relevance of our findings. Another limitation of this investigation may be that we did not evaluate pro-oxidant markers (reactive oxygen species), exercise performance, recovery, or muscle fatigue, which would have strengthened our study design. However, the acute effects of l-Cit supplementation on exercise performance, recovery and muscle fatigue have been formerly described and highlighted in various review articles(Reference Gonzalez and Trexler10,Reference Figueroa, Wong and Jaime15,Reference Sureda and Pons51) , including a couple of meta-analyses(Reference Rhim, Kim and Park16,Reference Trexler, Persky and Ryan52) . Future research should aim at assessing the effects of acute l-Cit supplementation on post-exercise oxidation products.
Conclusion
In conclusion, l-Cit supplementation (12 g dose before exercise) can boost antioxidant markers and significantly increase NOx concentrations after a high-intensity interval exercise session in young men.
Acknowledgements
The authors are thankful to all participants who have volunteered in this study and also laboratory of Physiology department of Guilan University staff for technical analysis.
This research received no specific grant from any funding agency, commercial or not-for-profit sectors.
A. W. and J. M. formulated the research question and designed the study. K. V. performed data collection, analysed the evidence, interpreted the results and wrote the paper. J. M supervised the research process. Paper revisions were completed by A. W. and K. V. The final manuscript was read and accepted by all authors.
There are no conflicts of interest declared by the authors.