The prevalence of obesity and overweight in the Western world is increasing and has become a major health threat( Reference Kopelman 1 , Reference Farooqi 2 ). Dietary intervention studies have shown a strong positive association between saturated fat intake, typical of Western-style diets, and the development of obesity and its co-morbidities( Reference Ponnampalam, Lewandowski and Nesaratnam 3 , Reference Erlanson-Albertsson, Montmayeur and le Coutre 4 ). Exocrine pancreatic enzymes, in general, and lipases, in particular, play an indispensable role in the digestion and, consequently, the absorption of dietary constituents, thus controlling the amount of nutrients and lipids entering the body( Reference Brannon 5 – Reference Kondo, Minegishi and Komine 7 ). Several studies have shown that exocrine pancreatic lipases are regulated by the type and amount of dietary fat consumed( Reference Brannon 5 – Reference Ricketts and Brannon 12 ). Furthermore, exposure to saturated fat has been shown to exert toxic effects on exocrine pancreatic cells, both in vitro ( Reference Landau, Alcaly and Forti 13 ) and in vivo ( Reference Thrower, Gorelick and Husain 14 ). Only a few studies have analysed pancreatic digestive enzymes during the development of diet-induced obesity (DIO); few studies have demonstrated exocrine function abnormalities in genetically obese models of laboratory animals( Reference Trimble, Bruzzone and Herberg 15 – Reference McLaughlin, Peikin and Baile 17 ), focused on short-term feeding regimens in young animals( Reference Sabb, Godfrey and Brannon 11 , Reference Wicker and Puigserver 18 ) and, by large, analysed only a fraction of the pancreatic digestive enzymes. The effect of high dietary fat intake on the time-dependent changes of exocrine pancreatic digestive enzyme levels during the development of DIO has not been studied.
Differences in the sensitivity to dietary fat have been demonstrated among mouse strains, particularly in strains that develop obesity after chronic consumption of high-fat (HF) diets. C57BL/6J (B6) mice have been found to develop severe obesity when fed a HF diet and thus classified as sensitive to DIO( Reference Kondo, Minegishi and Komine 7 ), mimicking the development of common obesity in humans.
In the present study, changes in the expression of exocrine pancreatic enzyme genes of C57BL/6J mice in response to either control low-fat diet or HF diet feeding were investigated.
Experimental methods
The institutional and national guidelines for the care and use of animals were followed and the experiment was approved by the Local Committee for Care and Use of Laboratory Animals of Wageningen University.
Animals and diets
The present study is part of the NuGO Proof of Principle Study package as described by Baccini et al. ( Reference Baccini, Bachmaier and Biggeri 19 ). Male C57BL/6J mice were obtained from Charles River (Maastricht, The Netherlands) at 3 weeks of age. The mice were housed in pairs and fed standard chow and given free access to water in the light- and temperature-controlled animal facility (12 h light–12 h dark and 20°C) of Wageningen University. At 12 weeks of age, all the mice were given a control diet as a run-in period diet for 4 weeks. After the run-in period, the mice were randomly divided into two groups and fed either a diet with 45 % energy (E%) derived from dietary fat or a control diet with 10 E% derived from dietary fat for additional 4 and 12 weeks (n 10 mice per diet and time group). The sample size was determined based on the minimum size required to provide at least 85 % power in the analysis of primary outcomes (α = 0·05 and β = 0·15). The sample size was ten mice per group. Diet composition has been published previously( Reference de Wit, Boekschoten and Bachmair 20 ), and it is summarised in Table 1. Palm oil was the main source of fat in the diets (containing 44 % 16 : 0 and 39 % 18 : 1). The only other variable component in the diets was the amount of maize starch( Reference de Wit, Boekschoten and Bachmair 20 ). Body weight and food intake were measured weekly. After 4 and 12 weeks of dietary intervention, the mice were fasted for 5 h and subsequently anaesthetised with a mixture of isoflurane (1·5 %), nitrous oxide (70 %) and oxygen (30 %). Blood samples were collected by orbital puncture before killing the mice. The mice were killed by cervical dislocation. For RNA and protein isolation, the pancreas was excised, divided into two parts and frozen immediately in liquid N2.
Table 1 Diet composition*

E%, percentage of energy.
* Based on the composition proposed by Research Diets, Inc.
Measurement of blood glucose, leptin and insulin levels
The blood levels of glucose were measured using an Accu-Chek glucose meter (Roche Diagnostics). The serum levels of leptin and insulin were measured using the mouse serum adipokine Lincoplex Kit (Linco Research, Nuclilab). The assays were conducted according to the manufacturers' protocol, and the samples were measured using the Luminex X100 system with the STarStation software (Applied Cytometry Systems). All individual samples were analysed in duplicate and the results were averaged when the difference between the two measurements was ≤ 5 %.
Isolation of RNA
Total RNA was extracted from the pancreas using TRIzol reagent (Invitrogen). RNA integrity was tested by agarose gel electrophoresis (1 %) with ethidium bromide (Mercury) staining. RNA was quantified using a NanoDrop ND-1000 spectrophotometer (NanoDrop Technologies). Total RNA (1 μg) was reverse transcribed using the Transcriptor High Fidelity cDNA Synthesis Kit (Roche).
Quantification of mRNA by real-time PCR
The transcript levels of amylase, chymotrypsinogen, trypsinogen, elastase 1 and elastase 2, pancreatic lipase (PL), pancreatic lipase-related protein 1 (PLRP1), pancreatic lipase-related protein 2 (PLRP2), pancreatic phospholipase A2 (PLA2) and cholesterol ester lipase (CEL) and of the housekeeping gene actin were determined by quantitative PCR using the STRATAGENE Mx3000 sequence detection system (STRATAGENE; Agilent Technologies). Gene-specific primers were designed using the Primer Express Software (Applied Biosystems). The quantitative PCR primer pairs were designed across exon(s) to avoid false-positive signals from potentially contaminating genomic DNA. Primer and complementary DNA concentrations were optimised (including melting curve analyses). The internal reference was ROX. Each 20 μl reaction mixture contained 2 μl (1–2 μg) first-strand complementary DNA, 10 μl PCR Master Mix (Applied Biosystems), and 300–700 nm of each of the forward and reverse primers (according to the optimisation of the primers). All reactions were carried out under the following conditions: pre-incubation at 50°C (2 min); denaturation at 95 °C (10 min); forty cycles of 95°C (15 s), followed by annealing and elongation at 60°C (1 min). Primer sequences are available upon request. Commercial software (Applied Biosystems) was used to calculate ΔΔCt (2− (Cttarget gene − Ctcontrol gene )) relative expression values of all the genes studied, normalised to that of the control.
Western blot analysis
Proteins were extracted using radioimmunoprecipitation assay lysis buffer (15 mm-Tris–HCl (1 %), Triton X-100 (0·1 %), SDS, 167 mm-NaCl and 0·5 % sodium deoxycholic acid), with a protease inhibitor cocktail (Caibiochem). Total protein (30 μg) was assayed. SDS–PAGE, protein transfer and Western blot analysis were carried out using standard laboratory techniques. The following primary antibodies were used: goat anti-pancreatic lipase and pancreatic lipase-related protein 2 (Santa Cruz Biotechnology) and mouse anti-β-actin (Millipore). The following secondary antibodies were used: bovine anti-goat (Santa Cruz Biotechnology) and goat anti-mouse (Millipore). Luminata Crescendo Western HRP Substrate chemiluminescence (Millipore) was performed. Densitometric analysis of the immunoblots was carried out using the ImageQuantTL software (GE Healthcare Life Sciences). All proteins were quantified relative to the loading control.
Statistical analysis
Data are reported as means with their standard errors. Differences between the mean values were tested for statistical significance using two-way ANOVA (diet × time). If a statistically significant (P <0·05) interaction between the factors diet and time was found, post hoc Bonferroni's method was used. Body weight over time was analysed using ANOVA with diet as a fixed factor and time as a repeated measure.
Results
Energy intake and body weight
The energy intake of C57Bl/6J mice fed the 45 E% diet for 4 and 12 weeks increased significantly (P< 0·05) compared with that of mice fed the 10 E% diet for 4 and 12 weeks (Fig. 1(a)). Food intake was not significantly different between the groups. Both time and diet had a significant (P< 0·05) effect on body weight gain (Fig. 1(b)).

Fig. 1 Average energy intake (a) and body weight gain (b) in C57BL/6J mice fed the control (10 % energy; ,
) and high-fat (45 % energy;
,
) diets for 4 and 12 weeks. Values are means (n 10 mice per diet and time group), with their standard errors represented by vertical bars. * Mean value was significantly different from that of the control diet-fed mice (P< 0·05).
Plasma parameters
The plasma levels of glucose and insulin were elevated in mice fed the 45 E% diet when compared with those in mice fed the 10 E% diet, and the differences reached significance (P< 0·05) at week 12 (Table 2). The levels of glucose at baseline (time 0) were already quite elevated. This was most probably due to the use of an anaesthetic (isoflurane), which induces blood glucose level elevation, as has been reported previously( Reference Desaulniers, Yagminas and Chu 21 , Reference Constantinides, Mean and Janssen 22 ). The levels of insulin and glucose following 45 E% diet feeding for 12 weeks indicated that the mice were developing hyperglycaemia or insulin sensitivity reduction, as fasting blood glucose and insulin levels were elevated when compared with those in mice fed the 10 E% diet. The plasma levels of leptin increased drastically and significantly (P< 0·05) in mice fed the 45 E% diet, starting at week 4 and at week 12 (Table 2).
Table 2 Plasma glucose, insulin and leptin levels in C57BL/6J mice fed the low-fat (LF, 10 % energy) and high-fat (HF, 45 % energy) diets for 4 and 12 weeks (Mean values with their standard errors; n 10 mice per diet and time group)
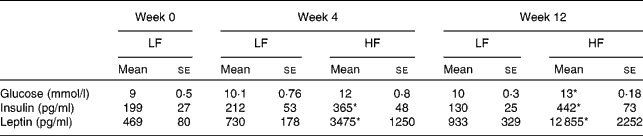
* Mean value was significantly different from that of the LF diet-fed mice (P< 0·05).
Exocrine pancreatic digestive enzyme transcript levels
The transcript levels of PL were significantly (P< 0·05) affected by time; that is, these levels were higher at 12 weeks than at all the other time points (Fig. 2(a)). The transcript levels of colipase were significantly (P< 0·05) affected by time and diet. The transcript levels of colipase were significantly (P< 0·05) elevated in the HF diet-fed mice than in the control diet-fed mice. The transcript levels of colipase at 4 and 12 weeks were significantly different from baseline levels at time 0 (Fig. 2(b)). The mRNA levels of PLRP1 were not detectable at any time point in mice fed either diet (data not shown). The transcript levels of PLRP2 were significantly (P< 0·05) affected by time (at 4 and 12 weeks compared with time 0). No significant effects of diet were found (Fig. 2(c)). The transcript levels of PLA2 were significantly (P< 0·05) affected by time (Fig. 2(d)). The transcript levels of CEL were not significantly affected by either time or diet (Fig. 2(e)). For all the lipolytic enzymes, no interaction between diet and time was found. The expression levels of chymotrypsinogen, trypsinogen, and elastase 1 and elastase 2 were not affected by either time or diet (data not shown). The transcript levels of elastase 1 and elastase 2 exhibited a modest increase as a function of time. The transcript levels of amylase exhibited a tendency to increase in the high-carbohydrate control diet-fed mice; however, the effect of time or interaction did not reach statistical significance (data not shown).

Fig. 2 Pancreatic lipase (PL) (a), colipase (b), pancreatic lipase-related protein 2 (PLRP2) (c), phospholipase A2 (PLA2) (d) and cholesterol ester lipase (CEL) (e) transcript levels in C57BL/6J mice fed the control (10 % energy) and high-fat (45 % energy) diets for 4 and 12 weeks. Values are means (n 10 mice per diet and time group), with their standard errors represented by vertical bars. * Mean value was significantly different from that at week 0 (P< 0·05; ANOVA). For PL, PLRP2 and PLA2 transcript levels, there was a significant effect of time (P< 0·05). †Mean value was significantly different from that of the 4-week 10 E% fat group (P< 0·05; ANOVA). For colipase transcript levels, there was a significant effect of time and diet (P< 0·05). For all the lipolytic enzymes, there was no significant interaction between time and diet.
Exocrine pancreatic lipase and pancreatic lipase-related protein 2 protein levels
The protein levels of PL were significantly (P< 0·05) elevated in the HF diet-fed mice than in the control diet-fed mice at 4 and 12 weeks. No significant effects of time or interaction were found (Fig. 3(a)). The protein levels of PLRP2 were significantly (P< 0·05) elevated in the HF diet-fed mice than in the control diet-fed mice at 4 and 12 weeks. No significant effects of time or interaction were found (Fig. 3(b)).

Fig. 3 Pancreatic lipase (PL) (a) and pancreatic lipase-related protein 2 (PLRP2) (b) protein levels in C57BL/6J mice fed the control (10 % energy (E%)) and high-fat (45 E%) diets for 4 and 12 weeks. Values are means (n 5 mice per diet and time group), with their standard errors represented by vertical bars. * Mean value was significantly different from that of the control diet-fed mice (P< 0·05). A, 0-week 10 E% fat; B, 4-week 10 E% fat; C, 4-week 45 E% fat; D, 12-week 10 E% fat; E, 12-week 45 E% fat.
Discussion
Feeding a HF diet to obesity-prone C57BL/6J mice resulted in increased body weight, elevated leptin levels and an associated hyperglycaemic and hyperinsulinaemic state similar to other findings reported for this mouse strain( Reference Surwit, Wang and Petro 23 – Reference Ahrén, Simonsson and Scheurink 25 ). The blood levels of glucose at baseline were already quite elevated. This was most probably due to the use of an anaesthetic (isoflurane), which induces blood glucose level elevation, as has been reported previously( Reference Desaulniers, Yagminas and Chu 21 , Reference Constantinides, Mean and Janssen 22 ), possibly due to the retention of the anaesthetic in adipose tissue. In the present study, the consumption of a HF diet was found to alter the mRNA expression of exocrine pancreatic enzymes related to lipid digestion such as PL, PLRP2, PLA2 and CEL. Although all lipases exhibited some elevated expression during the 12 weeks of HF diet feeding, the pattern of responsiveness to the diet was different among these enzymes. Lipids are known as the strongest stimulants of pancreatic enzyme secretion( Reference Keller and Layer 6 ). However, most of the studies have investigated the effect of HF diets during short periods of dietary change( Reference Tsunoda, Ikemoto and Takahashi 24 ) or, more frequently, in physiological and regulatory studies mostly individual digestive enzymes have been analysed( Reference Keller and Layer 6 ). Scarce information exists concerning the response of all major digestive enzymes to DIO development as a result of HF diet consumption. It is well established that the expression of pancreatic digestive enzymes changes in response to their respective dietary nutrients( Reference Keller and Layer 6 ) through several mechanisms( Reference Erlanson-Albertsson, Montmayeur and le Coutre 4 – Reference Keller and Layer 6 , Reference Wicker and Puigserver 18 , Reference Wicker, Scheele and Puigserver 26 – Reference Little, Russo and Meyer 31 ). Furthermore, it has become evident that the products of enzyme hydrolysis, such as fatty acids, have an important role in the mediation of appropriate gastrointestinal digestibility and absorption mechanisms and contribute to the release of satiety hormones( Reference Little, Russo and Meyer 31 ). As each individual digestive enzyme appears to be regulated by diet composition and metabolic state independently of the others, we studied changes in the gene expression of all pancreatic enzymes with a focus on lipases.
The mRNA levels of PL did not exhibit significant alterations induced by the HF load. Previous studies have suggested that major changes in PL levels appear only beyond a threshold level corresponding to more than 45 % of total energy provided as fat( Reference Brannon 5 ). Therefore, our finding in C57BL/6J mice seems to be in line with this observation. Yet, a time-dependent increase in transcript levels was found in the control diet-fed mice, and compared with these levels, PL levels were decreased in the HF diet-fed mice at week 12, and this may be explained by the apparent insulin and leptin resistance known to modulate and impair exocrine pancreatic enzymes and PL, in particular( Reference Rippe, Berger and Mei 27 , Reference Dandona, Freedman and Foo 32 , Reference Elinson, Amichay and Birk 33 ), or by the detrimental effects of obesity on exocrine pancreatic cells( Reference Landau, Alcaly and Forti 13 ). The protein levels of PL responded to HF diet feeding; these levels were significantly elevated in the HF diet-fed mice than in the control diet-fed mice at 4 and 12 weeks. However, the protein levels of PL in the HF diet-fed mice were down-regulated at 12 weeks compared with those at 4 weeks. The only partially parallel response of PL transcript and protein levels indicates mainly post-translational regulation of PL. The transcript levels of colipase were elevated rapidly following HF diet feeding, starting at week 4. This elevation paralleled PL transcript level elevation, although PL transcript level elevation was not significant. Unlike the transcript levels of PL at 12 weeks, which were reduced, the transcript levels of colipase in the HF diet-fed mice remained high. The colipase:PL ratio is an important determinant of lipolytic activity and colipase is the rate-limiting factor( Reference Rippe, Berger and Mei 27 ). The non-parallel change observed in the present study could be explained as a compensation mechanism to maximise PL activity following 4 and 12 weeks of HF diet feeding. Interestingly, the levels of colipase were also high at 12 weeks in the control diet-fed mice. Previous studies have shown that colipase and PL are regulated independently( Reference Rippe, Berger and Mei 27 ). Furthermore, at week 12, mice were insulin insensitive. This condition has previously been shown, in accordance with the present results, to lead to an increase in colipase transcript levels( Reference Duan and Erlanson-Albertsson 34 ). Of note is the colipase:PL ratio, which was close to the functional ratio for lipid hydrolysis only in the HF diet-fed mice (0·45 (se 0·06) and 0·5 (se 0·1) for 4 and 12 weeks, respectively); however, this ratio was significantly below the optimal value in the control diet-fed mice (0·2 (se 0·04) and 0·35 (se 0·08) for 4 and 12 weeks, respectively). These results show the importance of this ratio for the physiological adaptation of the pancreas to dietary alternations.
The two related lipases PLRP1 and PLRP2, which share homology to PL (68 and 65 %, respectively( Reference Giller, Buchwald and Blum-Kaelin 35 )), exhibited different patterns of responsiveness. The expression of PLRP1 was not detectable at any time point in mice fed either diet. Lipase activity has not been detected yet for the PLRP1 protein. Although its structural properties suggest that PLRP1 may participate in dietary fat digestion, its physiological role is still unknown( Reference Lowe, Kaplan and Jackson-Grusby 36 ). Furthermore, the expression of PLRP1 as well as PLRP2 is high during early development and declines in adulthood to minimal levels( Reference Payne, Sims and Jennens 37 ). The transcript levels of PLRP2 were significantly elevated during the 12 weeks of HF diet feeding, but no difference was found between the groups; this is in agreement with a previous report( Reference Rippe, Berger and Mei 27 ) and suggests that in order that changes in transcript levels occur, if at all, there needs to be a specific threshold level of fat intake. The biological meaning of this needs further investigation and may involve a role of PLRP2 in the catalysis of TAG digestion in vivo only at extreme fat intake. Previous studies related to newborns, lacking significant PL, have shown that PLRP2 contributes substantially to lipid digestion. Thus, the elevation of PLRP2 levels at 4 and 12 weeks could compensate for the down-regulation of PL transcript levels at 12 weeks( Reference Xiao, Mukherjee and Ross 38 ). Notably, PLRP2 has broader substrate specificity than PL and is dependent on colipase( Reference Lowe, Kaplan and Jackson-Grusby 36 ). The protein levels of PLRP2 were significantly affected by diet, exhibiting a response to HF diet feeding at 4 and 12 weeks. This indicates that PLRP2 is regulated by a HF diet at the post-transcriptional level. To the best of our knowledge, this is the first study to analyse the responsiveness of PLRP2 to diet in a DIO model.
PLA2 is an abundant pancreatic lipolytic enzyme that hydrolyses mainly dietary phospholipids. Phospholipids are important for the formation of micelles and also play an indirect role in cholesterol absorption( Reference Huggins, Boileau and Hui 39 , Reference Richmond, Boileau and Zheng 40 ). The transcript levels of PLA2 did not respond to dietary change. As in the case of PL expression levels, consumption of 45 % of energy as dietary fat in a state of obesity down-regulated PLA2 expression levels to basal levels; however, this effect did not reach statistical significance. This reduction could indicate, as in the case of PL, an effect of insulin resistance or an impaired dysfunction of exocrine pancreatic cells. However, this effect should be studied further.
The major role of CEL in the digestive tract is generally hydrolysis of cholesteryl esters and retinyl esters before their absorption( Reference Howles, Carter and Hui 41 ). We found no significant changes in the expression of CEL due to increased dietary fat intake and during the development of obesity. As the HF diet used in the present study was essentially free of cholesterol, the response of CEL to dietary fat intake would not be relevant; PL and PLRP2, which are complementary lipolytic enzymes, exhibited elevated expression levels. Taken together, our data suggest that individual pancreatic lipolytic enzymes respond differently to the changes in dietary fat intake and obesity development.
Pancreatic proteases adapt to changes in dietary protein levels( Reference Brannon 5 , Reference Keller and Layer 6 ), but the two diets used in the present study had the same amount of protein and thus no specific change in expression was expected. Yet, the expression of protease transcripts exhibited a tendency to increase as mice became older and body weight increased, and this may be related to elevated food consumption. Pancreatic protease content and activity elevation has been reported previously( Reference Bruzzone, Trimble and Gjinovci 16 , Reference Schneeman, Inman and Stern 42 – Reference Heller, Chavez Zander and Neuhaeuser 44 ) in obese Zucker rats and could result from obesity co-morbidities and pancreas pathology. Although the present study analysed a different animal model, it could demonstrate that these changes are partly, but not entirely, significant at the synthesis (expression) level.
The transcript levels of amylase were elevated in the high-carbohydrate control diet-fed mice; however, this effect did not reach significance. Amylase is known to adapt to the level of carbohydrate intake( Reference Brannon 5 ). In the present study, elevated levels of fat in the diet at the expense of carbohydrates resulted in the reduction of energy derived from carbohydrate from 69 to 34 E%; thus, the changes in amylase transcript levels at week 12 reflect the high-carbohydrate load. Additionally, changes in insulin sensitivity are known to down-regulate amylase secretion and activity levels in diabetic rats( Reference Sabb, Godfrey and Brannon 11 , Reference Bruzzone, Trimble and Gjinovci 16 ). Thus, the low amylase levels in animals on HF diets may also arise from alterations caused by impaired insulin sensitivity in the DIO state.
In conclusion, we demonstrated that obesity caused by chronic consumption of a HF diet by C57BL/6J mice leads to alternations in the transcript levels of exocrine pancreatic enzymes. However, the main regulatory effect of key lipases occurs through the post-transcriptional regulation of the levels of proteins that respond to macronutrient alterations favouring dietary fat. The changes may mainly originate from dietary fat intake, but may also be part of the obesity-derived co-morbidities.
Acknowledgements
The present study was partly funded by NuGO, the European Nutrigenomics Organization. I. R.-A., M. V. B., M. M. and H. Daniel are the core members of NuGO.
The authors' contributions are as follows: R. Z. B., I. R.-A., M. V. B., M. M. and H. Daniel contributed to the overall research plan and study protocol development, project management and study oversight, statistical analysis and manuscript preparation; R. Z. B, M. V. B. and H. Danino carried out the study and diagnostics and data collection and analysis.
None of the authors has any conflicts of interest to declare.