There is a presumption that, at some point in the future, the need to use animals in research will come to an end. However, as long as such use remains necessary, public acceptance is conditional on the understanding that the minimum amount of pain will be caused to the minimum number of animals to deliver the maximum benefit for humans, other animals and the environment( Reference Clemence and Leaman 1 ). The 3Rs of Replacement, Reduction and Refinement therefore provide a rational basis upon which animal use may continue to carry public support.
The 3Rs were first promulgated by Russell & Burch in 1959( Reference Russell and Burch 2 ). However, the ethical background to our use, and potential abuse, of animals predates that by several centuries. In Europe, René Descartes (1596–1650) espoused what became known as the Cartesian philosophy – that only humans have minds, souls and consciousness. Descartes considered animals to be like machines and, as such, unable to feel pain. Vivisection was thus widely practised throughout Europe, entirely without anaesthesia.
However, this philosophy was challenged over the ensuing years, particularly by Jeremy Bentham (1748–1832) who, in 1789, made his oft quoted declaration that ‘the question is not can they reason, nor can they talk, but can they suffer?’ The assumption that animals are capable of suffering led to the requirement that the harms imposed on animals should be minimised and be balanced against the benefits which might be expected to accrue. This utilitarian doctrine – that an action is right in so far as it promotes happiness, and that the greatest happiness of the greatest number should be the guiding principle of conduct – remains the position today in many parts of the world where research using animals is effectively regulated.
Almost 100 years later, following a Royal Commission Report, Britain passed the first legislation in the world to regulate the use of animals in science – the Cruelty to Animals Act of 1876. It utilised a system of licensing and inspection administered by the UK Home Office. Records show many notable physiologists worked under this regulatory system including Ernest Starling who requested the use of dogs in 1898 to contribute to his understanding of the Starling Principle of fluid exchange in blood vessels. The Home Office’s Annual Report for 1922 also mentions Alexander Fleming whose experiments on dogs led to him introducing the term ‘lysozyme’ in a Royal Society paper that year.
In 1954, the Universities Federation for Animal Welfare (UFAW) appointed William Russell, a zoologist and classical scholar, and Rex Burch, a microbiologist, to carry out a systematic review of current laboratory techniques with regard to ethical aspects. Their general report in 1956 included a description of the 3Rs. This formed the nucleus of their book entitled ‘Principles of Humane Experimental Technique’ published by UFAW in 1959( Reference Russell and Burch 2 ), a remarkably prescient piece of work given the status of ethical thinking at that time.
For several decades, little attention was given to the concept of the 3Rs with most focus being given to ‘Alternatives’ which were considered to be only those which replaced the use of animals either entirely (absolute replacement) or which merely used tissues obtained from animals (relative replacement). In 1981 the Center for Alternatives in Animal Testing (CAAT) was set up at Johns Hopkins University in Baltimore, USA, primarily funded by the cosmetics industry which was coming under increasing pressure to find methods which replaced the use of animals to test the safety of their products. CAAT, however, recognised that, for progress in science to continue, the use of animals remained necessary and therefore options for reduction and refinement needed equally to be considered. In 1993, CAAT organised the First World Conference on the 3Rs in Baltimore, significantly including ‘Alternatives and Animal Use’ in the conference title and hence bringing the 3Rs into modern parlance.
Further World Conferences have since been held every 2–3 years in various parts of the world including Europe, Asia and North America. Arguably the greatest progress in embedding the 3Rs into modern science has been in Europe, particularly in the UK. In 2002, a House of Lords Select Committee reviewed the status of animal research in the UK and concluded that more needed to be done to ensure the 3Rs were being actively considered by scientists in designing their experiments( 3 ). The report called upon the Government to take action and, in 2004, the National Centre for the 3Rs (NC3Rs – www.nc3rs.org.uk) was launched with Government funding. The primary focus of the NC3Rs is to increase the uptake of the 3Rs amongst the scientific community, particularly in the UK although recognising the importance of international impact. It achieves this primarily through providing competitive funding to UK research scientists proposing projects based on the 3Rs. Its portfolio includes projects in all three areas, thus supporting the use of animals but in an ethical context. It also focuses both on fundamental research and on translating the results into practical application. In addition, its own scientists carry out reviews and publish reports and guidance in collaboration with relevant experts. Examples include its work with the pharmaceutical industry where it acts as a neutral party, receiving, analysing and publishing anonymised company data and thus enabling companies to bench-mark their performance in implementing the 3Rs, particularly in safety testing. The remarkable success of NC3Rs may be measured by the increase in the funding it has attracted over the 12 years of its existence (from £600 K in 2004 to over £10 million in 2016). Similar national centres have now been established in a number of other countries, particularly in Europe.
In 2010, the European Parliament passed Directive 2010/63 on the Protection of Animals used for Scientific Purposes( 4 ) which explicitly requires scientists to consider the 3Rs in all their work. The impact of the Directive has been to both raise and harmonise standards of animal welfare and use across Europe( Reference MacArthur Clark 5 ). Also in 2010, the UK Government published a commitment to ‘work to reduce the use of animals in scientific research’( 6 ) followed by a Delivery Plan which outlines how this commitment was to be achieved( 7 ).
Three priorities were identified in the Delivery Plan:
(1) to advance the 3Rs in the UK by putting them at the heart of science-led programmes;
(2) to influence the global uptake and adoption of 3Rs approaches; and
(3) to promote an understanding about the use of animals where no alternatives exist.
The subsequent Delivery Report( 8 ) demonstrates how political leadership at the highest level can support real impact in all areas of scientific endeavour, both in academia, industry and in government research.
Compared with the original definitions of Russell and Burch, a number of attempts have been made to redefine the terms replacement, reduction and refinement. Tannenbaum & Bennett( Reference Tannenbaum and Bennett 9 ) reviewed these and concluded that the original definitions have much to recommend them. However, the definitions alone do not recognise the remarkable advances in technology which have enabled scientists to address the needs of their target population (usually humans) in ways which were not possible even a few years ago. Table 1 therefore proposes a contemporary approach which has been developed by the NC3Rs for delivering each of the 3Rs. By considering the 3Rs in such dynamic terms, the contemporary approach becomes more relevant and appealing to scientists, demonstrating clearly how their ongoing efforts to apply the 3Rs might benefit their science rather than simply being an ethical hurdle to be overcome( Reference Blakemore, MacArthur Clark and Nevalainen 10 ). The application and potential impact of these approaches, particularly in research relevant to nutrition, digestion and metabolism in both health and disease, will be considered in this review paper.
Table 1 Comparing the conventional definitions of the 3Rs proposed by Russell & Burch( Reference Russell and Burch 2 ) with contemporary approaches to advancing scientific progress (courtesy National Centre for the 3Rs)

Replacement
Effective progress in replacement generally needs to be led by scientists who have an intimate understanding of their particular subject and thus can assess the suitability of specific replacement options. However, new technologies have introduced options which may have generic application. For example, non-mammalian systems such as insects and nematode worms are being used to study basic biology in conditions such as diabetes and obesity( Reference Ashrafi, Chang and Watts 11 ). The ability to generate complex cell cultures, including stem cell technologies, three-dimensional (3D) approaches and tissue engineering all contribute to better modelling of the digestive system in vitro. Monteiro-Riviere et al.( Reference Monteiro-Riviere, Ortega and Choi 12 ) used four canine cell types to study potential toxicity of ingredients commonly used in dog food, illustrating how an in vitro panel can be used for hazard assessment. 3D intestinal organoids (mini-guts) appear to faithfully replicate healthy gut physiology as well as helping us to understand host-parasite interactions( Reference Eichenberger, Talukder and Field 13 ) and diseases such as cystic fibrosis( Reference Dekkers, Wiegerinck and de Jonge 14 ). Furthermore, the power of CRISPR-Cas9 genome-editing technology makes it possible to introduce mutations into organoids derived from normal human intestinal epithelium to mimic conditions such as colorectal cancer( Reference Matano, Date and Shimokawa 15 ).
Replacement technologies often enable scientists to perform experiments which would not be possible in classical animal models. For example, recent advances in microfluidics have created the human ‘gut-on-a-chip’ technology which provides a microenvironment for mimicking the natural conditions of the human intestines in a small-scale, controllable, in vitro platform( Reference Kim and Ingber 16 ). This has enabled co-culture of intestinal cells with living microbes from the normal and abnormal microbiome for an extended period of time( Reference Kim, Li and Collins 17 ). More recently it has been shown that this may also provide a suitable in vitro model for enteric virus infection and investigating mechanisms of enterovirus pathogenesis, none of which would be feasible in animal models or static cell culture systems( Reference Villenave, Wales and Hamkins-Indik 18 ).
Advances in computational methods and mathematical modelling offer new options for large-scale human nutritional and metabolomics studies involving complex data sets( Reference Bijlsma, Bobeldijk and Verheij 19 ) including the scientist’s ‘treasure trove’ of genetic data offered by the UK Biobank study( 20 ). Such work might previously have involved in vivo animal studies generating results of lesser value than studying the target human species.
Gaining confidence in a replacement method may initially require increased use of animals to ensure scientific validity, but the increase can be justified by the ultimate reduction. For example, replacing the classic mouse bioassay for marine biotoxins in shellfish required scientists to identify the relevant toxins in suitable analytical systems such as HPLC and liquid chromatography-MS. This was followed by extensive validation studies to ensure human safety would not be placed at risk by adopting the non-animal methods. The result is a set of more accurate assays which completely replace a mouse model which involved paralysis and death. In the UK, by 2013 there was no reported use of mouse bioassays compared with over 8000 mice used in this very severe test in 2007( 7 ).
Reduction
For many years, it has been recognised that reducing to a minimum the number of animals used may not be best for ethical and welfare outcomes. First, too few animals used may lead to results which are statistically difficult or impossible to interpret accurately. Second, it is generally considered that the harm to each individual animal should be minimised, even if this means using larger numbers of animals. Therefore, a balance needs to be achieved between minimising total numbers while obtaining reliable results and avoiding greater harm than necessary to individuals.
The contemporary approach to Reduction therefore focuses more on experimental design and ensuring that results are reproducible wherever possible. Significant concern has been expressed in recent years about reproducibility with a review by Amgen able to confirm findings in only six out of fifty-three (11 %) ‘landmark’ pre-clinical cancer trials( Reference Begley and Ellis 21 ). A number of reasons are offered including inadequate cell lines and mouse models. However, a major contributor was the poor quality of experimental design and published data. The studies where the findings could be reproduced described close attention being given to controls, reagents, investigator bias and including the complete data set in the analysis. This confirmed earlier findings by Ioannidis( Reference Ioannidis 22 ) and Prinz et al.( Reference Prinz, Schlange and Asadullah 23 ). Further, a 2016 Nature survey showed that 90 % of the more than 1500 scientists surveyed considered lack of reproducibility to be a crisis of either significant or slight proportions( Reference Baker 24 ). Clearly this is an ethical challenge for the scientific community globally, and especially where animals have been used in those studies.
Two particular problems of experimental design were identified in a comparison of 1117 studies of multiple sclerosis utilising the standard experimental autoimmune encephalomyelitis model( Reference Vesterinen, Sena and ffrench-Constant 25 ). First, the authors identified the importance of randomisation. The effect size in non-randomised studies was 42 % but only 21 % in randomised studies. Second, the importance of blinding was highlighted. Non-blinded studies showed an effect size of 41 % compared with only 30 % in blinded studies. Both errors in experimental design led to a significant overestimate of treatment effect in the former studies, potentially influencing expensive decisions about clinical studies.
A review of published animal experiments in 2009 concluded that there were major discrepancies in the majority of papers considered( Reference Kilkenny, Parsons and Kadyszewski 26 ). This led to publication of the Animal Research: Reporting of In Vivo Experiments (ARRIVE) guidelines which provide a checklist of twenty items which are required to maximise the internal validity, reproducibility and context of a paper( Reference Kilkenny, Browne and Cuthill 27 ). These guidelines have now been endorsed by more than 1000 journals internationally as well as all the major UK research funders and many universities, learned societies and scientific organisations.
To further support scientists in both designing and reporting their experiments, NC3Rs has developed an online tool called the Experimental Design Assistant (EDA – https://eda.nc3rs.org.uk). The EDA tool consists of a web application and supporting website. Its use can help to ensure robust study design leading to reliable and reproducible research findings. It supports randomisation, blinding and sample size calculation and improves transparency by allowing colleagues and collaborators to discuss experimental plans.
Reduction may also be achieved by utilising new technologies which provide more quantifiable and objective data. For example, Wallis et al. used Quantitative Light-induced Fluorescence to measure dental plaque in dogs. The technique was reliable, objective and required about 60 % fewer animals than the traditional method( Reference Wallis, Gill and Colyer 28 ). Marshall-Jones et al showed similar results in cats( Reference Marshall-Jones, Wallis and Allsopp 29 ).
Refinement
There is an underlying principle that poor welfare is at odds with good science. Therefore, in addition to our ethical obligations to optimise welfare, the science-led rationale for Refinement is persuasive. Under the contemporary approach, Refinement includes new in vivo technologies which minimise the pain or distress experienced by animals as well as the range of methods which deliver enhancements in animal care, housing, handling and use.
Modern methods of non-invasive imaging allow for subtle changes to be monitored at time intervals without the need to kill animals for samples. Nevertheless, these methods often require animals to be repeatedly sedated or anaesthetised and it is important to consider the stress this may cause. This can be mitigated by training animals to willingly undergo non-invasive procedures whilst conscious. Wrigglesworth et al.( Reference Wrigglesworth, Bailey and Cloyer 30 ) used positive reinforcement training (PRT) techniques to assess meal progression through the gastrointestinal tract by fluoroscopic imaging without sedation or physical restraint of the trained dogs. Similarly, training dogs to be completely still during measurement of transepidermal water loss can give very accurate data without the need for sedation( Reference Watson, Fray and Clarke 31 ).
PRT techniques have been used in a range of other species to minimise stress by increasing the level of cooperation in carrying out routine procedures. Reviews of this approach in non-human primates( Reference Prescott and Buchanan-Smith 32 , Reference Schapiro, Bloomsmith and Laule 33 ) have concluded that using PRT techniques can significantly reduce the adverse impact of experimental, husbandry and veterinary procedures. Laule( Reference Laule 34 ) provides a comprehensive review of PRT for a wide range of laboratory species.
Advances in bioanalytical techniques have opened up the potential to use smaller sample volumes (micro-samples) of blood, plasma or serum. It is now possible to assess circulating levels of drugs or other chemicals, and to measure blood biochemistry parameters, from samples as small as 5–20 µl. Particularly for small laboratory rodents, this enables multiple samples to be taken from the same animal over time thus avoiding the need to use greater group sizes and kill animals. In addition to reducing numbers needed, micro-sampling provides a refinement because it is quicker and less stressful. For example, warming of mice to dilate tail veins can be reduced or avoided.
Anaesthesia and analgesia can be used as part of invasive experimental protocols to minimise pain or distress experienced by animals. All personnel should be trained to recognise signs of pain and distress in the relevant species. The development of ‘grimace scales’ for a number of commonly used species offers a reliable and rapid means of pain assessment alongside other indicators. For example, the mouse grimace scale has been shown to be accurate and reliable, requiring only a short amount of training for the observer( Reference Langford, Bailey and Chanda 35 , Reference Miller and Leach 36 ). Posters illustrating grimace scales for mice, rats and rabbits are available from NC3Rs (www.nc3rs.org.uk) as well as online interactive guidance in recognising and preventing pain suffering and distress in laboratory animals.
Handling of animals for routine husbandry and minor procedures can also be a source of profound stress leading to increased background variation. For example, studies have shown( Reference Hurst and West 37 , Reference Gouveia and Hurst 38 ) that the traditional method of picking up mice by the tail induces aversion and high anxiety whereas use of open hand or tunnels, regardless of prior familiarity, leads to the animals voluntarily approaching with low anxiety.
Importantly, Refinement also includes methods of improving the quality of housing and care of animals through, for example, environmental enrichment. It has been suggested that environmental enrichment may increase inter-animal variability within a study leading to increased animal numbers and less conclusive experimental outcomes. However, Garner( Reference Garner 39 ) argued that barren housing, which prevents animals being able to control and modify their environment, leads to levels of stress which impact many physiological parameters. For example, providing nesting material to small rodents allows them to create a microenvironment closer to their homoeostatic temperature than the typical animal facility environment of 20–22°C. Abnormally stressed animals, in which homoeostatic adaptation is failing, make poor experimental models including in studies related to nutrition and metabolism. Stressed animals often develop abnormal repetitive behaviours reflecting underlying abnormalities of brain function and correlated with a wide range of behavioural changes which affect experimental outcomes. Hence, both validity and reliability of data are impacted as well as reproducibility of results between laboratories.
A wide range of methods and devices to enrich animals’ environments have been reported, many of which are relatively inexpensive and may sometimes save significant cost. Even larger species such as dogs and cats can be provided with enriched environments at relatively small additional cost. Desforges et al.( Reference Desforges, Moesta and Farnworth 40 ) described a simple adaptation of commercially available stand-alone shelving for indoor housed cats being used in nutritional studies. They showed that this not only enabled the cats to exploit the unused vertical space but also reduced agonistic interactions. A number of others have shown that the use of enrichment reduces stress and abnormal behaviours including in rats, rabbits and dogs as well as reducing aggression in cats( Reference Belz, Kennell and Czambel 41 – Reference Damasceno and Genaro 44 ).
All these methods of reducing potential stress by training animal subjects and optimising care, housing and husbandry practices are just as relevant to nutritional studies as to any other field of research. Furthermore, there is good evidence that the suitability of diets for experimental animals is a key factor in minimising stress. This applies not only to the nutritional content but also the presentation of the food (dry, moist, powdered, fresh), frequency of feeding (ad lib or periodic), variety of foodstuffs (particularly for larger species such as non-human primates) and overall palatability. Sometimes the results are striking – for example Goodwin( Reference Goodwin 45 ) showed that incorporating a live ciliate protozoa in the diet of zebrafish fry significantly increased survival rates and reduced tail abnormalities and sex bias. Hence nutritional expertise is relevant to refinement in all fields of research involving animals.
Ethical review and the 3Rs
A core feature of regulating the use of animals in research in most countries is a requirement for ethical review of research proposals. In 2010, the World Organisation for Animal Health (the OIE) recommended that a system of animal use oversight should be implemented when formulating regulatory requirements in each country. This included ethical review as part of the oversight framework for OIE Members to follow( 46 ). Ethical review involves consideration of the validity and justification for using animals including a harm-benefit analysis which considers the potential harms for animals and the likely benefits of the use. The 3Rs provide a key tool in minimising the harms in this ethical analysis( Reference Griffin, MacArthur Clark and Zurlo 47 ).
It is thus critically important that scientists become aware of all the options to apply the 3Rs relevant to their field. This requires new approaches and new incentives in which NC3Rs has played an innovative and very effective role. For example, a 2010 call for research proposals using the 3Rs to support innovative and multidisciplinary approaches to study asthma has led to improved scientific and clinical understanding of this condition as well as reduced reliance on the use of animals. A more recent call for new approaches to ageing research is likely to have similar impact. Likewise, the focused CRACK IT programme brings together scientists from academia and industry both to define problems and to apply new technologies in delivering solutions.
Although decisions about the appropriateness of a particular 3Rs measure might be taken by the scientist responsible for the research, it is important that other professionals, including veterinarians and animal care staff, are included in the consideration and able to contribute their particular skills and knowledge. This is particularly relevant to refinement options where the broader professional understanding may bring original suggestions to the consideration.
However little attention has been given to the potential burden on scientists of complying with the requirements of a regulatory oversight system( Reference Blakemore, MacArthur Clark and Nevalainen 10 ). Taken to extreme, the regulations may become so onerous that they prohibit important scientific progress without any perceivable improvement in animal welfare. This is critical since, in general, society places a high priority on benefiting from the medical and other scientific advances which can be realised through high-quality research. Nevertheless, it is equally true that the public seeks assurances that animals do not suffer unnecessarily in order to deliver those advances (Fig. 1).
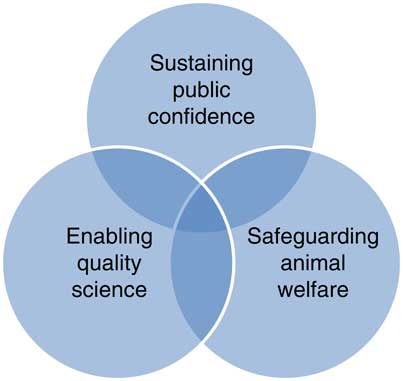
Fig. 1 In ethical review, achieving the right balance between enabling high-quality science and safeguarding the welfare of the animals sustains public confidence in the oversight system.
There is thus an imperative on all who are involved in regulating the use of animals in research, whether members of local ethical review committees or officials acting on behalf of national governments, to ensure efficient and effective consideration of research proposals and to not place in the way of science bureaucratic or pointless obstacles which have no welfare benefit. ‘Paralysis by Analysis’ is a well-recognised problem in which over-analysis of a situation results in a decision never being taken, in effect paralysing the outcome.
Conclusions
Promoting the 3Rs in research delivers not only ethical benefits associated with avoidance of animal use and improved animal welfare, but also has great potential to deliver scientific benefits.
Many Replacement technologies achieve greater consistency and accuracy, give rapid results and are generally less expensive than using animals. Furthermore, many of these studies are simply not possible in animal models, either because of limitations of low-throughput in animals or because tissues relevant to the target human species are essential. Applying robust criteria to experimental design to ensure reproducibility alongside Reduction also results in better scientific outcomes. And thoroughly applying the principles of Refinement ensures stress as a scientific variable is minimised.
Hence, the 3Rs provide opportunities for high standards of animal welfare alongside better science, faster science, and more cost-effective science. Furthermore, using Replacement methods has the added advantage of avoiding the burden of regulatory oversight necessary to ensure animal use is justified. Nevertheless, where animal use is proposed, it is important to ensure that unnecessary bureaucracy is avoided in delivering prompt, fair and justifiable oversight decisions.
A balance is demanded between the needs of the science and the needs of the animals and it is this balance which supports and sustains the public’s confidence in ethical review processes and the regulatory oversight of the use of animals in science. Tinkering with this balance, either excessively in favour of science or excessively in favour of animal welfare, will lead to loss of public confidence and erosion of the conditional permission society grants to enable animal research to continue for as long as it is necessary.
Acknowledgements
This review paper is based upon a presentation made at the Waltham International Nutritional Science Symposium 2016 for which the author received financial support from the Waltham Centre for Pet Nutrition.
The author declares that there are no conflicts of interest.