Book contents
- Shrews, Chromosomes and Speciation
- Cambridge Studies in Morphology and Molecules: New Paradigms in Evolutionary Biology
- Shrews, Chromosomes and Speciation
- Copyright page
- Contents
- Contributors
- Preface
- 1 Milestones in Common Shrew Chromosomal Research
- 2 Introducing the Common Shrew
- 3 Morphology and Genetics of the Common Shrew: General Features
- 4 Phylogeography
- 5 Chromosomal Differentiation in the Common Shrew and Related Species
- 6 Phylogenetic Relationships of Chromosomal Races
- 7 Meiosis and Fertility Associated with Chromosomal Heterozygosity
- 8 Chromosomal Hybrid Zones
- 9 Gene Flow Between Chromosomal Races and Species
- 10 Geometric Morphometric Tests for Phenotypic Divergence Between Chromosomal Races
- 11 Is It Really the Chromosomes?
- 12 Further Divergence: the Role of Ecology and Behaviour
- 13 Climate, Diversification and Refugia in the Common Shrew: Evidence from the Fossil Record
- 14 Shrews, Chromosomes and Speciation
- Index
- References
7 - Meiosis and Fertility Associated with Chromosomal Heterozygosity
Published online by Cambridge University Press: 01 March 2019
- Shrews, Chromosomes and Speciation
- Cambridge Studies in Morphology and Molecules: New Paradigms in Evolutionary Biology
- Shrews, Chromosomes and Speciation
- Copyright page
- Contents
- Contributors
- Preface
- 1 Milestones in Common Shrew Chromosomal Research
- 2 Introducing the Common Shrew
- 3 Morphology and Genetics of the Common Shrew: General Features
- 4 Phylogeography
- 5 Chromosomal Differentiation in the Common Shrew and Related Species
- 6 Phylogenetic Relationships of Chromosomal Races
- 7 Meiosis and Fertility Associated with Chromosomal Heterozygosity
- 8 Chromosomal Hybrid Zones
- 9 Gene Flow Between Chromosomal Races and Species
- 10 Geometric Morphometric Tests for Phenotypic Divergence Between Chromosomal Races
- 11 Is It Really the Chromosomes?
- 12 Further Divergence: the Role of Ecology and Behaviour
- 13 Climate, Diversification and Refugia in the Common Shrew: Evidence from the Fossil Record
- 14 Shrews, Chromosomes and Speciation
- Index
- References
Summary
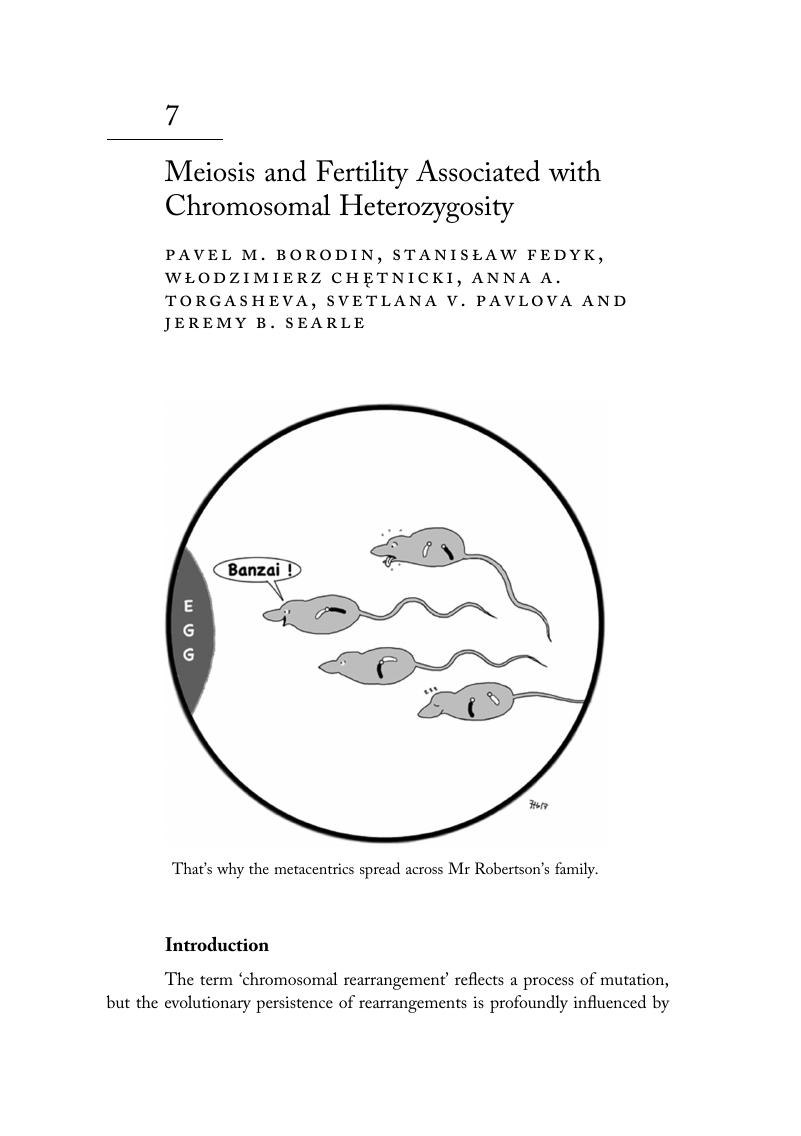
- Type
- Chapter
- Information
- Shrews, Chromosomes and Speciation , pp. 217 - 270Publisher: Cambridge University PressPrint publication year: 2019
References
- 6
- Cited by