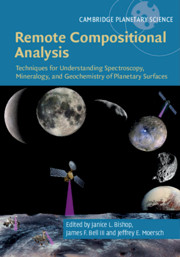
Book contents
- Remote Compositional Analysis
- Cambridge Planetary Science
- Remote Compositional Analysis
- Copyright page
- Contents
- Contributors
- Foreword
- Preface
- Acknowledgments
- Part I Theory of Remote Compositional Analysis Techniques and Laboratory Measurements
- Part II Terrestrial Field and Airborne Applications
- Part III Analysis Methods
- Part IV Applications to Planetary Surfaces
- Index
- References
Part I - Theory of Remote Compositional Analysis Techniques and Laboratory Measurements
Published online by Cambridge University Press: 15 November 2019
- Remote Compositional Analysis
- Cambridge Planetary Science
- Remote Compositional Analysis
- Copyright page
- Contents
- Contributors
- Foreword
- Preface
- Acknowledgments
- Part I Theory of Remote Compositional Analysis Techniques and Laboratory Measurements
- Part II Terrestrial Field and Airborne Applications
- Part III Analysis Methods
- Part IV Applications to Planetary Surfaces
- Index
- References
Keywords
- Type
- Chapter
- Information
- Remote Compositional AnalysisTechniques for Understanding Spectroscopy, Mineralogy, and Geochemistry of Planetary Surfaces, pp. 1 - 258Publisher: Cambridge University PressPrint publication year: 2019