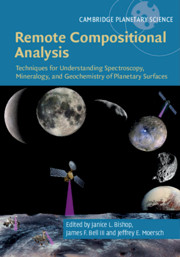
Book contents
- Remote Compositional Analysis
- Cambridge Planetary Science
- Remote Compositional Analysis
- Copyright page
- Contents
- Contributors
- Foreword
- Preface
- Acknowledgments
- Part I Theory of Remote Compositional Analysis Techniques and Laboratory Measurements
- Part II Terrestrial Field and Airborne Applications
- Part III Analysis Methods
- 13 Effects of Environmental Conditions on Spectral Measurements
- 14 Hyper- and Multispectral Visible and Near-Infrared Imaging Analysis
- 15 Thermal Infrared Spectral Modeling
- 16 Geochemical Interpretations Using Multiple Remote Datasets
- Part IV Applications to Planetary Surfaces
- Index
- References
16 - Geochemical Interpretations Using Multiple Remote Datasets
from Part III - Analysis Methods
Published online by Cambridge University Press: 15 November 2019
- Remote Compositional Analysis
- Cambridge Planetary Science
- Remote Compositional Analysis
- Copyright page
- Contents
- Contributors
- Foreword
- Preface
- Acknowledgments
- Part I Theory of Remote Compositional Analysis Techniques and Laboratory Measurements
- Part II Terrestrial Field and Airborne Applications
- Part III Analysis Methods
- 13 Effects of Environmental Conditions on Spectral Measurements
- 14 Hyper- and Multispectral Visible and Near-Infrared Imaging Analysis
- 15 Thermal Infrared Spectral Modeling
- 16 Geochemical Interpretations Using Multiple Remote Datasets
- Part IV Applications to Planetary Surfaces
- Index
- References
Summary
The advent of multiple orbital and in situ missions to planetary bodies beyond Earth has enabled characterization of extraterrestrial shallow crustal processes. We describe examples of interpreting geochemical, isotopic, and radar properties from multiple remote datasets, supplemented with in situ observations from rovers and landers, meteorites, and lunar samples. Given the availability of distinct data types and the relevance to bulk-silicate bodies in the Solar System, we present five case studies for the Moon and Mars. The first involves lunar magmatic processes in relation to TiO2 and radargram-derived physical properties. Next, O and Fe isotope variations relative to the Mg number provide insight into the degree of fractional crystallization in lunar lava flows. Physical mixing of endmembers and chemical weathering processes in Gusev crater soil on Mars are discussed. Effective use of the Chemical Index of Alteration (CIA) is also considered by comparing mineralogic observations across Mars with terrestrial references. Lastly, the nature of bulk soil hydration on Mars is described by assessing chemical variations with Principal Component Analysis (PCA). This chapter describes in situ analyses and mapping across local and regional scales. Data synthesis also involves contrasting depth scales from tens of microns to multiple kilometers.
Keywords
- Type
- Chapter
- Information
- Remote Compositional AnalysisTechniques for Understanding Spectroscopy, Mineralogy, and Geochemistry of Planetary Surfaces, pp. 337 - 348Publisher: Cambridge University PressPrint publication year: 2019