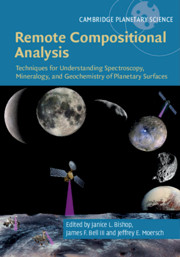
Book contents
- Remote Compositional Analysis
- Cambridge Planetary Science
- Remote Compositional Analysis
- Copyright page
- Contents
- Contributors
- Foreword
- Preface
- Acknowledgments
- Part I Theory of Remote Compositional Analysis Techniques and Laboratory Measurements
- 1 Electronic Spectra of Minerals in the Visible and Near-Infrared Regions
- 2 Theory of Reflectance and Emittance Spectroscopy of Geologic Materials in the Visible and Infrared Regions
- 3 Mid-infrared (Thermal) Emission and Reflectance Spectroscopy
- 4 Visible and Near-Infrared Reflectance Spectroscopy
- 5 Spectroscopy of Ices, Volatiles, and Organics in the Visible and Infrared Regions
- 6 Raman Spectroscopy
- 7 Mössbauer Spectroscopy
- 8 Laser-Induced Breakdown Spectroscopy
- 9 Neutron, Gamma-Ray, and X-Ray Spectroscopy
- 10 Radar Remote Sensing
- Part II Terrestrial Field and Airborne Applications
- Part III Analysis Methods
- Part IV Applications to Planetary Surfaces
- Index
- References
1 - Electronic Spectra of Minerals in the Visible and Near-Infrared Regions
from Part I - Theory of Remote Compositional Analysis Techniques and Laboratory Measurements
Published online by Cambridge University Press: 15 November 2019
- Remote Compositional Analysis
- Cambridge Planetary Science
- Remote Compositional Analysis
- Copyright page
- Contents
- Contributors
- Foreword
- Preface
- Acknowledgments
- Part I Theory of Remote Compositional Analysis Techniques and Laboratory Measurements
- 1 Electronic Spectra of Minerals in the Visible and Near-Infrared Regions
- 2 Theory of Reflectance and Emittance Spectroscopy of Geologic Materials in the Visible and Infrared Regions
- 3 Mid-infrared (Thermal) Emission and Reflectance Spectroscopy
- 4 Visible and Near-Infrared Reflectance Spectroscopy
- 5 Spectroscopy of Ices, Volatiles, and Organics in the Visible and Infrared Regions
- 6 Raman Spectroscopy
- 7 Mössbauer Spectroscopy
- 8 Laser-Induced Breakdown Spectroscopy
- 9 Neutron, Gamma-Ray, and X-Ray Spectroscopy
- 10 Radar Remote Sensing
- Part II Terrestrial Field and Airborne Applications
- Part III Analysis Methods
- Part IV Applications to Planetary Surfaces
- Index
- References
Summary
A variety of features in the visible and near-infrared regions that are observed in remote sensing applications are the result of electronic transitions, typically involving cations of transition metals, most commonly Fe and Ti, or the molecular species S. The position and intensity of these features are sensitive not only to the particular cation, but also to its oxidation state, the particular phase in which it occurs, the geometric structure of the site that it occupies, and interactions between and among neighboring cations. Often these features are diagnostic for the host mineral.
- Type
- Chapter
- Information
- Remote Compositional AnalysisTechniques for Understanding Spectroscopy, Mineralogy, and Geochemistry of Planetary Surfaces, pp. 3 - 20Publisher: Cambridge University PressPrint publication year: 2019
References
- 4
- Cited by