11.1 Introduction
Engineering advances have been opening new possibilities for sharing electric energy. Technological and social innovations in the electric energy sector may allow consumers to become more actively engaged in producing and managing the generation, distribution, and use of their electricity, which could shift the locus of organizational decision making and control away from traditional utilities. These innovations also have potential to diversify and restructure who is included and excluded from energy sector benefits (Stephens, Reference Stephens2020).
The transition toward a “sharing economy” in the transportation and lodging sectors, and in other emerging sharing economy systems, can be understood as a process of separating rights of use from the other rights of ownership for goods that had previously not been as easily divisible in this manner. For example, app-based short-term lease platforms have disrupted the lodging sector by enabling owners to create new value by more easily assigning the rights of use of their dwellings, and consumers now have new options of affordable unique rentals as an alternative to owning a vacation home. In the current traditional deregulated energy system, ownership of energy generation infrastructure is centralized along with allocation of rights of use. Generally, consumers did not own energy assets and had little power to consume the type (for example, traditional vs clean and renewable) or price (for example, fixed rate, time-of-use) of energy that aligned best with their economic and social motives. Sharing economy ideas combined with new technology promise to decentralize energy generation thus increasing ownership and opening broader markets (for example, demand response, community generation, and resilient microgrids).
Sharing economy innovations in the electric grid, including community solar and energy blockchain systems, are expanding the role of assets owned by consumers. These innovations are transitioning the role of generation and management from large corporations and utilities to consumers. This restructuring has potential to democratize energy systems depending on how policy and regulations guide the development of a more distributed renewable-based society (Stephens, Reference Stephens2019). As households and businesses become prosumers, they are producing and sharing surplus energy with the grid and other users, creating what some are referring to as “locavolts” linked in microgrid systems to enhance electric energy resilience and to leverage local financing. Prosumers who can “share” their electricity may also be empowered to change the rules that have governed their relationships with utilities for the past century.
At the same time, technological innovations related to renewable electric energy generation, distribution, and demand-side management may enable new types of energy sharing with the potential to disrupt and transform the current electrical energy industry. In the same way that Uber and Airbnb have disrupted existing transport and hospitality industries, we suggest that the electricity energy sector may also be trending toward large-scale system reconstruction by way of innovations for energy sharing. In recent years, one outcome of these energy sharing innovations has been the rise of a new group of energy platform operators (for example, thermostat companies and other third parties) acting as “energy services providers” that position themselves as the electricity sharing economy platforms facing consumers. Many have identified the “death spiral of the utilities’ business model” in which the traditional fee for energy delivery that utilities charge to customers fails to provide adequate revenue from prosumers who use the grid only for short periods of peak power (Felder and Athawale, Reference Felder and Athawale2014). These periods of peak power, for example, in the early evening when the solar resource diminishes and residential demand increases, drive infrastructure costs. Energy platform operators may enter the power sector as new market players that aggregate small amounts of distributed energy resources (DERs) provided by each user into megawatts that can be traded in the traditional regional energy, capacity, and ancillary services markets.
Future energy systems are likely to integrate a regionally appropriate mix of renewable electricity generation that is dispatched, stored, and distributed through platforms that enable sharing at multiple scales, from milliseconds to decades (Stephens, Reference Stephens2019). The potential for sharing in electricity systems is expanding as energy systems around the world are transitioning from centralized large fossil fuel power plants to distributed renewable-based power generation at multiple locations (Stephens, Reference Stephens2020). Such flexibility in time scale and locations is critical, as the success or failure of any organization or individual operating in the sharing economy is determined by its ability to effectively manage an elastic match between resource supply and demand. Sharing in future electric systems has the potential to disrupt relationships governing utilities, energy consumers, and distributed electricity generation at the individual and household levels, at the community and organizational levels, and at the regional, state, national and even international levels.
This chapter reviews the range of institutional models for electricity generation and distribution; considers how electrical energy systems are undergoing a transformation toward more distributed, renewable-based configurations; and explores the multiple evolving mechanisms for energy to be shared among generators, distributors, and consumers. We present a typology of sharing economies in electric energy systems and identify the technological and sociopolitical potential for sharing electrical energy as systems evolve. The chapter also explores policy considerations that flow from such large-scale transformations in the energy sector. The chapter concludes with opportunities for future research and questions about the implications of energy sharing for energy justice.
11.2 Background on the Electric System Structure, Business Models, and Regulatory Frameworks
Over time, a wide range of institutional models, shaped by both technological and sociopolitical factors, have emerged for provisioning energy for households and industry (Stephens et al., Reference Stephens, Wilson and Peterson2015). Regulatory frameworks have evolved in parallel with transformations of technology and business models. It is important to understand how these transformations evolved over the last century, and the sociotechnical factors that led to them, to understand the disruptive potential of sharing economy innovations in the electric power sector.
Traditional utilities are structured as regulated monopoly providers of electrical power and grid services for a specific geographic area (Kiesling, Reference Kiesling2014). The role of utilities is to deliver power to satisfy consumer demand, which may require the utility to generate, transmit, store, and/or purchase power and regulate its frequency and/or voltage. Early on, utilities faced steep barriers to market entry, because power plants and transmission lines were very expensive. Consequently, when household electricity was introduced in the United States in the early 1880s, it was available only in larger cities for wealthy homeowners and almost 90 percent of rural homes were without electricity in the first decades of the twentieth century (Velaga et al., Reference Velaga, Prabakar, Singh, Sen and Kroposki2019). Over time, growth in household energy demand drove down the average cost of delivered power. This combination of economies of scale and high barriers to entry led to the three dominant utility business models that operate in the United States today: (1) state-operated not-for-profit consumer-owned electric cooperatives, (2) local publicly run or managed utilities (POUs), and (3) investor-owned utilities (IOUs). Although there are relatively few investor-owned utilities, they tend to be very large, serving over 75 percent of utility customers nationwide.
Across the world, electric power markets operate in a variety of forms that lie on a spectrum from monopolization to democratization. The evolution of energy systems and markets around the world has been very heterogeneous. In some countries, deregulated markets were established through the restructuring of some of the existing utilities, with the intent to disrupt energy generation monopolies by increasing competition. In deregulated electric grids, electric energy, capacity, and reserves are each traded on a wholesale market, which must meet customer demands while satisfying any constraints of the transmission system (for example, line limits). Finally, in energy choice systems, energy users have the opportunity to select among multiple energy providers based on rates and energy generation options (see Figure 11.1).

Figure 11.1 Three different systems for electricity generation, transmission, and distribution.
In the twenty-first century there have been considerable advancements in technologies for household electricity generation with renewables. These affordable distributed generation technologies are beginning to challenge the stability of the centralized electricity system (McKenna, Reference McKenna2018). There is a growing embrace of distributed energy resources enabled by new information and communications technologies such as blockchain technology, which enables trusted peer-to-peer distribution; and internet-of-things (IOT) devices, which enable real-time response to changes in supply and demand. These technological changes have precipitated the rapid growth of sharing economy businesses (Hamari et al., Reference Hamari, Sjoklint and Ukkonen2016). A primary argument in favor of the sharing economy model is that collaborative effort among participating actors leads to more efficient, sustainable, and resilient outcomes than centralized decision making (for example, by utilities).
Continued global population growth and resource-intensive consumption practices have increased energy demands, contributing to the global climate crisis. In response, a growing number of consumers are beginning to look for opportunities to participate in alternatives to the current electric energy systems that promise improved efficiency, reduced fossil fuel emissions, and more local control over energy system decision making. There is tremendous disruptive potential associated with sharing economy innovations that will enable a broader group of actors to produce, transmit, store, and consume electric power with each other.
11.3 Taxonomy of Electric Energy Sharing
In this section we explore key trends in sharing of electric energy, looking at forms of sharing that have emerged based on market structures and services being provided.
At face value, electric energy may appear to be a perfectly fungible commodity that can be produced by a variety of methods, transmitted across nations, and consumed and billed through meters. In reality, electric energy markets face unique challenges compared to most other sharing economies, in that the product being traded is not discrete (compared with a ride between two points provided by a ride-hailing service or two nights in short-term rental), cannot be easily stored (generation must match consumption at every instant in time, unless expensive batteries or other storage systems are utilized), and transferring the commodity requires specialized infrastructure (for example, wires and transformers) that have limited capacity (for example, circuit breakers on distribution lines can trip if a large load overheats a transformer).
To facilitate the discussion of the sharing economy in the electricity sector, it will be helpful to classify various types of “sharing” by the market structure: Top-down, in the form of a vertically integrated utility, or bottom-up, where individuals govern the market forces. Additionally, it is important to identify what is being bought and sold in different arrangements of energy sharing: Energy (for example, kilowatt-hours), ancillary services (that is, voltage and frequency control, generator dispatch, operating reserves), storage, and/or transmission.
Figure 11.2 represents a framework and taxonomy of sharing economy approaches in the electric grid along two dimensions – market structure and services provided. The services encompassed by a sharing economy may only include energy, or all of the services required to operate an electricity system (including energy, voltage and frequency control, and asset management). Along the other dimension, the prices and availability of services may depend on the market structure.
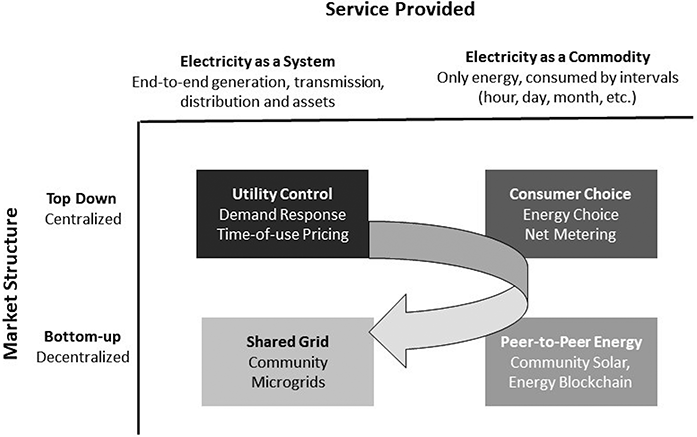
Figure 11.2 Taxonomy of sharing economy approaches in the electric grid.
In traditional utility-control approaches to energy sharing, consumers do not generate electricity; instead, they provide value through their flexibility to change demand. This is realized through demand response programs that ask users to modify behavior, or automatically adjust electric loads, for example, by turning off large energy loads such as air conditioners during times when the grid might overload. Similarly, time-of-use pricing enables users to shift their energy use to the times when low-cost, sometimes renewable, energy is available and can be easily transmitted to the consumer. However, such demand-side management approaches have limited capabilities since electricity demand is relatively inelastic to price.
Recent advances in regulatory structures and technology allow consumer choice in which consumers can choose which wholesale provider they buy their power from or generate power themselves. They then sell the excess energy (calculated over hourly, daily, or monthly periods) back to the grid through the wholesale power agreement of their distribution utility. Energy choice legislation in the form of the Energy Policy Act of 1992 enabled consumers to choose who they purchased their energy from, even specifying that only renewable energy be provided. As far back as 1983, consumers with the ability to produce electricity, primarily through rooftop solar, could share any excess, and receive the market value of that energy through net metering.
More recently, peer-to-peer energy sharing uses decentralized ledgers (energy blockchains, such as LO3 Energy) that track energy consumption, storage, and generation through a digital platform and enable users to choose who produces or consumes energy, and when, how and for how much the electricity is produced or consumed. The challenge in the future of the electric energy sharing economy is how to share all aspects of operations, safety, and management of the electric energy system.
Community microgrids promise to realize this future through breakthroughs in automation, machine learning, and digital platforms with both digital and physical aspects that enable sharing of distribution systems, or a fully shared grid. Community microgrids rely heavily not just on shared generation, including large amounts of renewables, but also on shared services through distributed energy resources (DERs). These DERs may include energy storage in parked electric vehicles, smart thermostats that run only when energy is available, and smart solar inverters that can help stabilize voltage and frequency on the shared wires. This sharing of the responsibility to balance supply and demand reduces costly peaks and transmission costs and creates a more resilient electric energy supply that can continue operating even when the larger grid suffers a blackout (Poudel & Dubey Reference Poudel and Dubey2018).
The following sections describe in more detail these four approaches to sharing economies in electric energy systems, moving clockwise around Figure 11.2 from the status quo utility control to future shared grids.
11.4 Utility Control – Demand Response and Time-Of-Use Pricing
Utility control describes top-down (centralized) management of grid services and reserves. In this arrangement, utilities are responsible for all grid services, including managing capacity of transmission lines and ancillary services that may include energy storage or controlling the readiness of power plants to begin producing energy to match demand.
Demand response innovations give some responsibility to energy consumers to help manage the electric grid. For example, during a time of high energy demand that might historically have led to a temporary partial shut off the grid (brownout), consumers can instead respond to signals from the energy provider and suspend operation of nonessential appliances, such as air conditioning units or pool pumps. This enables energy service to be maintained for essential functions and reduces total peak electrical load, reducing the need for costly increases in electric grid capacity. Such demand response (DR) may be fully automated with smart appliances (such as Google Nest) that adjust operation schedules based on signals from the utility or can simply take the form of utilities sending customers texts or other communications to encourage reducing energy use at specific times to avoid overloading the grid (for example, “Shave the Peak!”).
Time-of-use pricing involves changing the electricity rate based on current demand, more closely reflecting real-time energy prices on the wholesale market. For example, customers might be charged a much higher rate to use energy during times of the day when aggregate demand is at its peak. This time-of-use pricing incentivizes consumers to adjust their energy consumption for more efficient operation of the grid. Time-of-use pricing enables users to shift their energy use to the times when low-cost, sometimes renewable, energy is available and can be easily transmitted to the consumer.
Since electricity has become an integral and necessary part of modern life, electricity demand is largely inelastic, therefore limiting the impact of pricing policies on demand (Lee and Chiu, Reference Lee and Chiu2011). Furthermore, price elasticity is nonlinear and asymmetric, complicating the efficient implementation of such dynamic pricing approaches (Haas and Schipper, 1998).
11.4.1 Role of the Users
In these examples of utility control energy sharing, innovations enable consumers to share the value of their flexibility to change aggregate demand in real time. Both demand response and time-of-use pricing can work automatically or by active participation of consumers. Consumers can potentially benefit from sharing by avoiding situations where electrical power would otherwise be interrupted and by reducing their electrical energy bill by adjusting their behavior based on signals from the utility service provider. These utility control sharing mechanisms can be a considerable benefit to customers who have the flexibility and information access necessary to vary their energy usage in response to market signals. However, since the average household spends only 2 percent of its income on electric energy, and various consumption-specific social and behavioral factors make household-level flexibility difficult, the elasticity of energy consumption is low (Drehobl and Ross, Reference Drehobl and Ross2016; Wilson and Dowlatabadi, Reference Wilson and Dowlatabadi2007). Further, as low-income households may spend up to 30 percent of their income on electric energy, demand response and time-of-use pricing may place an inequitable burden on the already disadvantaged if they lack technology or flexibility necessary to adjust energy usage in response to market signals (Drehobl and Ross, Reference Drehobl and Ross2016).
11.4.2 Role of the Platform
For utility control of energy sharing, the utility, whether a private company, consumer-owned cooperative, or state-owned public company, functions as the platform for coordinating all sharing activities. Alternatively, demand response providers may be third-party commercial entities that aggregate the load flexibility of their customers into bids for the wholesale market. This motivates these third parties to help customers with strategies or technology to adjust their electricity consumption in response to market signals.
11.4.3 Notable Examples
Most US utilities offer both commercial and industrial customers options for centralized energy sharing in the form of demand response. Each of the nation’s independent system operators/regional transmission organizations (ISO/RTOs) sponsor demand response programs (Department of Energy [DOE], 2019). The Federal Energy Management Program (FEMP) helps federal agencies and other organizations identify opportunities for energy project incentives and demand response programs, execute these opportunities, and fully capture their benefits (DOE, 2019). Most utilities offer time-of-use pricing options as well: These program structures include simple time-of-use rates, where prices change at set times through a 24-hour cycle to reflect afternoon peak electricity use, overnight off-peak hours, and the shoulder periods in the morning and evening hours. Other variations of time-variable pricing include real-time pricing in which customers’ rates reflect the wholesale electricity market or the utility’s cost of production; day-ahead hourly pricing, where the utility sets prices to reflect the cost of acquisition or production for the coming day; and block-and-index pricing, in which the customer can lock in set energy prices for part of their energy use and pay current market price for additional usage.
11.5 Consumer Choice – Energy Choice and Net Metering
In the context of top-down (centralized) management of energy, without the complexity of real-time control of the grid, various forms of consumer choice energy sharing are possible on hourly, daily, or monthly timescales. These are distinct from what we have described in the previous section in that they engage the consumer in financing distributed energy resources (DERs), either on-site or remotely. DERs are decentralized because they rely on energy sources other than the utility to provide energy, and such sources can and often do include consumers themselves. DERs are not an innovation whose primary purpose is to manage constraints on the electrical grid. Instead, their primary benefits are to allow prosumers to buy energy from their preferred source (for example, renewables), reduce their bills through efficiency upgrades, and/or sell excess renewable energy they generate back to the grid at favorable prices.
Energy choice programs enable consumers to choose who they buy their energy from. For example, they might pay a premium for renewable electricity from wind turbines in another state. While this choice doesn’t directly affect the “source of electrons” transmitted to the household user, the arrangement directly finances clean energy production. In these wholesale markets, consumers are buying from a corporate producer of energy, such as a large wind farm aggregator or financial entity specializing in trading energy.
As far back as 1983, consumers with the ability to produce electricity, primarily through rooftop solar panels, could share any excess, and receive the market value of that energy through net metering. In net metering, consumers have the opportunity to produce energy locally and use their energy first, selling any excess back to grid, typically averaged over the day or the month. Sometimes energy sales back to the grid are managed on a yearly basis, and very rarely on an hourly basis. These systems give consumers the option to choose renewable energy for their own consumption and reduce dependence on the utility provider, thus leading a transition toward renewable energy generation. Electricity consumers who generate their own energy on-site, sell a portion of that energy back to the grid, and store energy or otherwise control their load, are called prosumers. Figure 11.3 depicts a generalized scheme for how energy choice and net metering systems interface with traditional utility businesses.

Figure 11.3 How energy choice and net metering systems interface with traditional utility businesses.
11.5.1 Role of the Users
The act of producing energy locally and sharing it back to the grid transforms “energy consumers” to “energy prosumers.” A growing body of research explores prosumers’ behavior and interactions with the electrical energy system. A survey of prosumer perceptions found that prosumers are motivated to collaborate with utilities to contribute to the societal goal of creating more renewable energy (Silva et al., Reference Silva, Karnouskos and Ilic2012). Information sharing and communication innovations are key to the successful management of energy sharing in prosumer engagement systems (Zafar et al., Reference Zafar, Mahmood, Razzaq, Ali, Naeem and Shehzad2018). However, since prosumer systems that require the individual to own an asset (for example, rooftop solar) are in general limited to those individuals that own their home, renters and other low-income citizens find it difficult to benefit from prosumer engagement, aside from energy choice.
11.5.2 Role of the Platform
In the case of the prosumer systems based on energy choice markets and net metering, traditional utility companies maintain all physical hardware and assets of the energy distribution system and real-time controls, while prosumers begin increasing their share of ownership or investment in energy generation assets. Energy choice and net metering programs do not specifically address the challenge of managing the electrical load on the grid to match supply with consumer demand. A growing body of research focuses on the problem of peak shaving, that is, reducing the maximum points of electricity demand. An index termed the sharing contribution rate (SCR) quantifies users’ contributions to energy sharing and peak shaving (Wang et al., Reference Wang, Zhong, Wu, Du, Xia and Kang2019).
11.5.3 Notable Examples
Globally, a broad array of programs for energy choice and net metering are emerging. In the United States, over a dozen states have deregulated electricity markets, meaning that electricity providers can compete to sell energy to consumers. One example of a platform that provides individual energy consumers with the opportunity to invest in solar energy projects is Mosaic, a solar lending platform. Traditionally, only individuals with sufficient starting capital could invest in household solar panels. However, new technologies have improved the efficiency and lowered the cost of manufacturing, installing, and permitting solar energy. Solar lending companies enable consumers to become prosumers by financing their initial solar panel installation, and then collecting back the loans over time with interest as these prosumers sell energy back to the utility. New platforms have emerged to connect investors with borrowers seeking financing for solar power projects.
11.6 Peer-to-Peer – Community Solar and Blockchain
Recent advances in regulatory structures and technology have enabled community solar programs in which solar panels or other DERs are installed on shared land, while dividends for the energy produced are paid out to the community. Even more recently, energy blockchains have emerged, functioning as decentralized ledgers that track energy consumption, storage, and generation through a digital platform and enable energy consumers and producers to choose when, how, by whom and at what price their electricity is purchased and sold.
Community solar and blockchain-based energy sharing are examples of peer-to-peer electricity sharing that integrates decentralized management of energy systems and does not directly address the technical considerations associated with real-time control of the electrical grid. In these systems, transmission lines and distribution infrastructure are still managed by the traditional utility. Because there are substantial costs to operating the grid, such as controlling voltage and managing storage, electricity consumers in these types of peer-to-peer arrangements continue to pay utilities for use of their infrastructure (but not for the generation of electricity).
11.6.1 Role of the Users
When prosumers and consumers trade self-produced energy in a peer-to-peer manner, they can both profit, and this could provide incentives for different kinds of investments. Previous research indicates that compared to traditional peer-to-grid models of energy sharing, peer-to-peer electricity sharing may confer economic benefits to consumers and environmental benefits in the form of carbon footprint reduction (Secchi and Barchi, Reference Secchi and Barchi2019).
11.6.2 Role of the Platform
In peer-to-peer sharing, the utility still manages (although, may not own) the physical assets to satisfy real-time needs. Recent research explores the potential for blockchain-based microgrid energy markets that do not require central intermediaries to facilitate transactions between producers and consumers, thus reducing costs associated with these traditionally labor-intensive markets.
11.6.3 Notable Examples
Examples of peer-to-peer sharing include community choice aggregation programs, as well as more sophisticated electricity trading platforms. One example of an electricity trading platform is Vandebron, a startup in the Netherlands that allows people to buy energy from independent producers (Vandebron, 2020). The mission of this company is to connect people who have surplus renewable energy with energy consumers who are not producing their own electricity. Utilities are not involved in the transaction. Functioning as an “Airbnb for electricity,” this platform allows energy consumers to search for producers. For example, a consumer could share their information on the platform to arrange for the purchase of their power from a farmer in a neighboring community who has wind turbines or solar panels in their fields. Such business models may be easier to implement in deregulated energy markets, such as the Netherlands, in contrast to other countries and many states in the United States (Schiller, Reference Schiller2014).
While the case has been made that blockchains are an effective technology to decentralize the energy system, to date there are only limited examples of blockchain-based, local, peer-to-peer energy sharing operating in practice (Mengelkamp et al., Reference Mengelkamp, Gärttner, Rock, Kessler, Orsini and Weinhardt2018; Noor et al., Reference Noor, Yang, Guo, van Dam and Wang2018). The Brooklyn Microgrid project (BMG) is one such example (Brooklyn Microgrid, Reference Microgrid2020). Note that this example differs from true shared grid systems described in detail later, because while consumers are able to trade and share energy with fellow consumers in the BMG project, the energy distribution infrastructure is owned and managed by a centralized utility. The network connects prosumers and consumers by enabling people to buy and sell locally generated renewable energy through a Brooklyn Microgrid mobile app, which gathers and records energy data for users. Through blockchain technology, BMG developed Exergy, a data platform that creates localized energy marketplaces for transacting energy across existing grid infrastructure. The BMG also acts as an educational and community engagement initiative: For example, the organization facilitates workshops in partnership with public schools in New York City to teach community members about the technology behind electric microgrid systems and encourage broader participation in energy sharing.
11.7 Shared Grid – Community Microgrids
When shared ownership of electricity generation infrastructure is combined with shared ownership of distribution and energy management infrastructure, these systems can be classified as a shared grid. Shared grid systems are characterized by decentralized management of real-time energy generation and advanced grid controls, made possible by breakthroughs in automation and machine learning. They promise improved physical reliability and resilience to cyber threats, opportunities to improve energy system sustainability, reduce reliance on fossil fuels, and improved economic efficiency. These innovations help scale and reduce the costs associated with the hundreds of people required to run a regional grid, to a handful of contractors to operate a microgrid.
Community microgrids rely heavily not just on shared generation or renewable energy, but also on shared grid management services provided by distributed energy resources (DERs). These DERs may include energy storage in a parked electric vehicle, smart thermostats that run only when energy is available, and smart solar inverters that can help stabilize voltage and frequency on the shared wires. Thus, DERs represent a pathway for sharing the responsibility to balance supply and demand among all participants in the system. When integrated into a community microgrid system, these technologies have the potential to reduce costly peaks in energy demand as well as transmission costs. Many researchers also argue that community microgrids are a viable solution for more resilient local electric energy systems that can continue operating even in instances when the larger national or regional electric grid suffers a blackout (Jiménez-Estévez et al., Reference Jiménez-Estévez, Navarro-Espinosa, Palma-Behnke, Lanuzza and Velázquez2017; Marnay et al., Reference Marnay, Aki, Hirose, Kwasinski, Ogura and Shinji2015; Wu et al., Reference Wu, Ortmeyer and Li2016).
11.7.1 Role of the Users
In a shared grid system, all users contribute to the cost of grid management. There are various ways that such cost sharing might be structured in real-world shared grid systems. For example, third-party platforms, decentralized algorithms, and/or self-governed user decisions can determine how the resources are managed.
11.7.2 Role of the Platform
In shared grid systems, the role of a traditional utility significantly diminishes. When energy generation, assets, and management of the grid are controlled by third-party platforms and decentralized algorithms that integrate a system of DERs, electrical energy systems can potentially function without any involvement of a centralized utility. A market of third-party platforms may even operate simultaneously in the same way that Uber and Lyft both operate in the same city. This future vision for shared grid systems requires, however, new technologies for information sharing between users in the system. For example, the algorithms needed to control millions of household-scale renewable energy generators and the load at millions of homes is very different than the algorithms that control load and generation in the traditional centralized generation system.
In addition to developing information sharing technologies to facilitate operation of shared grid systems, some scholars regard resource optimization technologies to be critical for continued innovation of smart grids. For example, Zafar et al. (Reference Zafar, Mahmood, Razzaq, Ali, Naeem and Shehzad2018) discuss linear and nonlinear optimization programming in the context of prosumer-based energy management and sharing. Other researchers present the optimization technique in a two-stage approach. For instance, Cui et al. (Reference Cui, Wang, Xiao and Liu2019) argue that the bi-level optimization problem could be transformed into a single-level mix integer linear programming problem through proper linearization techniques. In the second stage, an online optimization model is proposed for each prosumer to make the energy schedule according to the latest system situation and prediction error (Cui et al., Reference Cui, Wang, Xiao and Liu2019). Alternatively, Long et al. (Reference Long, Wu, Zhou and Jenkins2018) present a nonlinear programming optimization in the first stage with a rule-based control to update the control set-points through real-time measurement in the second stage.
11.7.3 Notable Examples: Community Microgrids
To date, most examples of shared energy generation and grid management can be found in institutional microgrids. The best examples of shared grid systems can be found at US military bases, where there are systems in place for coordinated management to govern when and how system users produce and consume energy (Hossain et al., Reference Hossain, Kabalci, Bayindir and Perez2014; Prehoda et al., Reference Prehoda, Schelly and Pearce2017). Shared grid examples also exist in hospitals and corporate landscapes. For example, East Boston has a microgrid network made up of institutions that share renewable electricity generation and backup generators among the buildings (Sheehan, Reference Sheehan2015).
Looking ahead toward the possibility of more shared grid systems being implemented throughout the US and globally, there is considerable potential for increased production of renewable energy and efficient management of supply and demand enabled by DERs and algorithms for real-time grid management. However, while these innovations suggest potential benefits of the sharing economy for the electric energy sector, there are important access and equity considerations to be addressed. In a future where corporations and wealthy neighborhoods shift toward community microgrids, less wealthy and powerful energy consumers could suffer negative consequences. If a subset of energy consumers invests in their own microgrid’s reliable distribution and generation infrastructure, they are no longer effectively investing in the reliability and efficiency of the whole electrical energy system. Such a trend in consumer behavior would effectively create islands of energy resilience in a sea of energy vulnerability.
11.8 Policy Considerations
In the future, in much the same way that sharing economy platforms such as Airbnb have simplified the process of contracting with an independent short-term lodging provider, electricity sharing has the potential to radically change how electricity consumers interact and behave. Indications of the future impacts can already be observed in the form of smart devices such as thermostats, operated by third-party platforms, that optimize their demand based upon real-time energy markets.
In earlier chapters, authors have discussed how the rise of the sharing economy in some sectors may increase consumption without improving sustainability. However, in the case of energy sharing, we anticipate a different trajectory that does not lead to increased consumption of fossil fuels. In part, this is because energy sharing is connected with a larger transformation toward renewable energy and a reduced carbon footprint.
The other benefits of utility control, consumer choice, and peer-to-peer energy system innovations primarily involve the reduced cost of energy generation and delivery. These reduced costs and impacts could theoretically result in an increase in energy consumption, but due to the price inelasticity mentioned earlier, we do not expect energy sharing to significantly impact energy consumption rates. That is, while reducing the price of lodging (for example, with the growth of Airbnb) may lead to more travel, energy consumption is unlikely to increase appreciably as the cost of energy goes down, particularly in developed economies (US Energy Information Administration, 2021). Any future increases in electricity consumption will instead likely be driven by increased adoption of electric vehicles and heat pumps as opposed to the specific influence of the sharing economy on energy. Finally, even with the rise of smart metering, demand response, and time-of-use pricing, there is relatively limited transparency in energy pricing. For example, the average consumer will most likely not be aware of the cost of running their dishwasher. This is due to the traditional monthly electricity billing cycle, temporally separating the saliency of the energy costs from the energy consumption occasions. New real-time IoT devices bring the potential to better synchronize consumption and costs; however, care must be taken to not overload users with too much information in real-time.
The potential societal benefits of a transition toward peer-to-peer and shared grid energy systems include improved efficiency, an increased share of renewable energy production, and more consumer engagement. In addition, these new mechanisms for local control and management provide individuals, households, and communities with more economic and political power as well as electrical power. Thus, increased energy sharing can be understood as a pathway toward realizing the goals of the energy democracy movement. Originating from trade union activism, energy democracy focuses on restructuring the political, economic, and social makeup of the energy system by transitioning to renewables while establishing democratic energy decision-making processes, equitable access to energy and energy-ownership for marginalized groups, and widely distributed and renewable energy resources (Sweeney Reference Sweeney2012; Burke and Stephens Reference Burke and Stephens2018).
However, while sharing economy innovations enable consumers to have more say in where and how their electricity is produced, there are possible negative consequences associated with a transition to a shared grid and associated “energy democratization” (Stephens et al Reference Stephens, Wilson and Peterson2015). Increased development of community microgrid systems could potentially lead to greater inequality in access to reliable and affordable electric energy. The traditional electric grid doesn’t just provide electric energy, it provides electric energy reliability. That reliability is critical to economic productivity and, in some cases, social wellbeing. If the transformation underway in the electric energy sector does in fact represent a death spiral of the utility business model, the result could be that only wealthy energy consumers could afford to have reliable community microgrids, and fewer resources would go toward maintaining the grid. This could lead to a decrease in energy reliability for consumers who do not have access or cannot afford to participate in microgrid systems. One possible solution would be to increase public investment in shared grids for communities who may not otherwise have access to emerging energy sharing systems. This may in practice resemble the move in recent decades toward public–private partnerships that support charter schools in low-income communities (Koirala et al., Reference Koirala, Koliou, Friege, Hakvoort and Herder2016).
Another way that energy sharing is tied to disruption and transformation in the electric energy sector is through altering the geographic distribution of energy generation sites (Stephens Reference Stephens2019). Historically, power generation occurred near coal reserves or at hydroelectric facilities. With increased local solar- and wind-powered electricity generation, preferred sites for power generation are changing and power lines built for transmission over long distances are no longer optimally placed, leading to increased potential for congestion. However, building new transmission lines is exceedingly expensive. There is a market opportunity to invest in reducing energy consumption demand. For example, it is cheaper to give away smart thermostats and selectively adjust them automatically during peak demand times than it is to build a new transmission line. This example points to just one of the ways that new technologies and changing social and economic priorities may transform the electrical energy sector.
11.9 Conclusions
As the pace of transformation in electrical energy generation and distribution systems continues to accelerate, the possibilities for innovative ways to “share” electricity are rapidly evolving. This chapter has reviewed this dynamic landscape and provided a taxonomy to characterize different modes of energy sharing. There is a transition underway away from legacy systems with high levels of utility control and toward new opportunities for prosumer engagement, peer-to-peer sharing, and ultimately in the direction of fully integrated sharing of all aspects of electricity generation and grid management. In this transition from utility control, to prosumer engagement, to peer-to-peer sharing, toward a decentralized market structure and integrated end-to-end management energy and assets, we recognize a possible end of the traditional utility business model. As third-party platforms for energy sharing and technologies enabling local renewable energy generation, storage, and supply and demand management systems emerge, we anticipate that the traditional integrated power supply and grid management roles of utilities may shrink across many regions.
In addition to a rise in prosumers, who are both energy users and energy generators, future energy sharing could also lead to a growing number of passive electricity consumers who are integrated into community microgrids. We anticipate that prosumers, currently primarily homeowners who own private renewable energy generation infrastructure, will increasingly be outnumbered by energy consumers whose energy purchasing decisions are regulated by smart devices. For example, a customer who wants to charge their electric vehicle at their home will have their default charging algorithm set to only charge when electricity is most readily available or cheapest.
Previous research on energy sharing has focused on technological innovation and prosumer behavior, including both empirical case studies and theoretical analyses. This research provides a valuable foundation for future studies. There is a need for more research on policy mechanisms to promote and regulate sharing in real-world energy systems. Barriers to redesigning the current energy system include current regulations and policies, public awareness and acceptance of new technologies, and established corporations’ resistance to change, which can lead to manipulation of markets and disinformation campaigns that question the legitimacy and reliability of alternative energy systems. There is also need for more social science research on how sharing could contribute to more inclusive energy systems including opening up opportunities for women, people of color, and indigenous people who have been historically excluded from energy sector jobs and economic empowerment through energy systems (Allen et al., Reference Allen, Lyons and Stephens2019).
Government and regulatory bodies have an important role to play in facilitating a transition to increased electrical energy sharing. Policy makers could, for example, establish incentive programs in the form of subsidies for new energy sharing enterprises entering the market, and punitive measures such as a carbon tax or pollution charges for fossil fuel-based energy systems. By enacting policy measures such as these and providing an environment that supports fair competition, government regulatory bodies can facilitate increased energy sharing systems that balance the goals of energy efficiency, sustainability, resilience, and equity.