Book contents
- The Cambridge Handbook of Cognitive Development
- The Cambridge Handbook of Cognitive Development
- Copyright page
- Contents
- Figures
- Tables
- Contributors
- Introduction
- Part I Neurobiological Constraints and Laws of Cognitive Development
- Part II Fundamentals of Cognitive Development from Infancy to Adolescence and Young Adulthood
- Part III Education and School-Learning Domains
- Index
- Plate Section (PDF Only)
- References
Part I - Neurobiological Constraints and Laws of Cognitive Development
Published online by Cambridge University Press: 24 February 2022
- The Cambridge Handbook of Cognitive Development
- The Cambridge Handbook of Cognitive Development
- Copyright page
- Contents
- Figures
- Tables
- Contributors
- Introduction
- Part I Neurobiological Constraints and Laws of Cognitive Development
- Part II Fundamentals of Cognitive Development from Infancy to Adolescence and Young Adulthood
- Part III Education and School-Learning Domains
- Index
- Plate Section (PDF Only)
- References
Summary
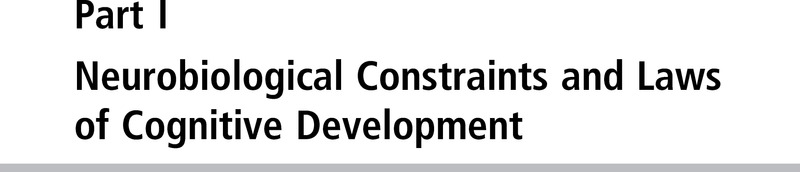
- Type
- Chapter
- Information
- The Cambridge Handbook of Cognitive Development , pp. 13 - 138Publisher: Cambridge University PressPrint publication year: 2022