The role of the anatomist is to provide a system of description that distinguishes between the features of the various components of the body. In a perfect system of description, having defined a name for a given entity, the same name will not then be used to account for a different entity. Use of the same term to account for different entities, potentially, is the road to disaster. It is possible, nonetheless, that the term in question may have become so ingrained, due to usage over time, that those using the same term are unaware they are describing different entities. This is the current situation with the naming of the channels that provide the potential for shunting between the ventricles. In the settings of concordant or discordant ventriculo-arterial connections, such channels are called ventricular septal defects. They can be closed both to separate the systemic and pulmonary blood streams, and to restore septal integrity. When there is a double outlet ventriculo-arterial connection, in contrast, the channel in question, when directly adjacent to the space subtended between one or other of the arterial roots, not only provides the substrate for interventricular shunting but it is also the outlet for one or other of the ventricles. Hence, it cannot be closed during surgical repair but must be tunnelled to one or the other of the subarterial outlets so as to separate the blood streams and restore septal integrity. When considered in terms of their anatomical borders, nonetheless, the areas closed by the surgeon are comparable in these settings. Logic dictates that if the area closed by the surgeon is defined as the “ventricular septal defect” when the ventriculo-arterial connections are concordant or discordant, it should continue to be defined as the “ventricular septal defect” when there is double outlet ventriculo-arterial connection. When this logical principle was emphasised following an investigation of hearts with double outlet right ventricle, Reference Ebadi, Spicer, Backer, Fricker and Anderson1 it was argued that usage was based on the surgical approach, rather than the anatomy. Reference Walters2 It is the underlying anatomy itself, however, that underscores the appropriate surgical approach.
At present, therefore, for better or worse, the channel between the ventricles in these specific settings is usually considered to be the “ventricular septal defect.” To justify this approach, some might argue that it would be demeaning to suggest that, when correcting double outlet right ventricle with subarterial defects, the surgeon is unaware that the defect cannot be closed. Those using such logic would argue that the surgeon, although describing the space in question as a “ventricular septal defect,” is fully aware that the operative procedure does not close it. Others might suggest that the anatomical situation is sufficiently complex to exclude a simple explanation. We contest such suggestions. When note is taken of the building blocks of the ventricular outflow tracts, Reference Aiello, Spicer, Anderson, Brown and Mohun3 the anatomical difference between the two channels is obvious. We review here those differences, taking as our paradigm the variation in overriding of the aortic root in the setting of tetralogy of Fallot. When using this example, we show that, irrespective of the ventriculo-arterial connection, the area closed or baffled by the surgeon to separate the blood streams and restore the integrity of the ventricular septum can be compared in terms of its borders. We then compare the situation as found in tetralogy with those encountered in the perimembranous defects found in hearts with concordant or discordant ventriculo-arterial connections, and double outlet right ventricle when the channel between the ventricles is directly adjacent to the aortic root. We point out that, in all these situations, the area in question is an aorto-right ventricular communication.
The building blocks of the ventricular outflow tracts
During normal development of the heart, the muscular ventricular septum is produced concomitantly with ballooning of the ventricular apical components from the primary heart tube. Reference Hikspoors, Kruepunga, Mommen, Köhler, Anderson and Lamers4 When first recognisable as a ventricular septum, the entirety of the atrioventricular canal is supported by the developing morphologically left ventricle, while the developing right ventricle supports the entirety of the outflow tract. All the blood entering the developing left ventricle must pass through the primary embryonic interventricular communication so as to reach the developing right ventricle (Fig. 1a). So as to provide the biventricular circulations, the right ventricle must develop its inlet, and the developing left ventricle its outlet. This requires remodelling of the area providing shunting between the developing ventricles. Reference Anderson, Spicer, Mohun, Hikspoors and Lamers5 The area in question is the primary interventricular communication, The first step in its remodelling is achieved by expansion of the atrioventricular canal (Fig. 1b). Reference Lamers, Wessels and Verbeek6 Subsequent to such expansion, the outflow tract remains supported by the developing right ventricle. The persisting part of the channel between the ventricles is now the outlet for the developing left ventricle. It can be described as the secondary embryonic interventricular foramen (Fig. 2a). The final stage in conversion to the biventricular circulations is then achieved by fusion of the proximal parts of the cushions that separate the outflow tract into the aortic and pulmonary channels (Fig. 2b). This process creates a baffle in the basal part of the cavity of the right ventricle, producing a tunnel between the aortic root, still supported above the cavity of the right ventricle, and the cavity of the left ventricle. Subsequent to fusion of the cushions to produce the tunnel, however, communication still remains between the aortic root and the right ventricle (Fig. 3a). Subsequent to its closure, the space beneath the aortic root, initially belonging to the right ventricle, will become part of the left ventricle. The persisting aorto-right ventricular communication, therefore, can also be considered to represent the tertiary interventricular communication. Reference Anderson, Spicer, Mohun, Hikspoors and Lamers5 It is this persisting aorto-right ventricular communication that is closed by tubercles derived from the atrioventricular cushions to complete ventricular septation (Fig. 3b). The tubercles then remodel to become the membranous part of the ventricular septum.

Figure 1. The images show sections from episcopic datasets prepared from developing hearts. Panel A shows a section through the ventricular loop in a murine embryo sacrificed at embryonic day 10.5. Panel B shows a four-chamber section from a human embryo at Carnegie stage 14, which is at the end of the fifth week of development.

Figure 2. The panels are from an episcopic dataset prepared from murine embryos sacrificed at embryonic day 12.5 (panel A) and 13.5 (panel B). Panel A shows the proximal outflow cushions in the process of closure as seen from the right ventricle, while panel B shows a four-chamber section taken subsequent to their fusion.

Figure 3. Panel A is a four-chamber section from the same episcopic dataset as used to prepare Figure 2b. It is from a mouse embryo sacrificed at embryonic day 13.5. It shows the entrances to the aortic root from the right and left ventricles. Panel B is a histological section prepared from a human embryo at Carnegie stage 21, which is toward the end of the seventh week of development. It shows the tubercles of the atrioventricular cushions, which have closed the tertiary embryonic foramen, and which will remodel to become the membranous septum.
If development proceeds normally, the proximal outflow cushions fused to produce the tunnel within the right ventricle muscularise. Reference van den Hoff, Moorman and Ruijter7 They become the free-standing subpulmonary infundibulum, which together with the inner heart curvature of the right ventricle produces the supraventricular crest (Fig. 4a). The septal attachment of the cushions becomes sufficiently well incorporated into the crest of the apical muscular septum that it is not possible, in the normal heart, to recognise an outlet septum (Fig. 4b). Although an outlet septum does not exist in the normal heart (Fig. 5), should septation be incomplete, the leading edge of the muscularised cushions can be recognised as the muscular outlet septum, albeit still with their distal parts forming a free-standing infundibular sleeve. In such settings of deficient septation, the initial inner heart curvature then remains recognisable as a ventriculo-infundibular fold. It retains its position as a structure which interposes between the leaflets of an arterial and an atrioventricular valve. Reference Aiello, Spicer, Anderson, Brown and Mohun3 The building blocks can be identified as individual entities in genetically perturbed mice that retain the initial arrangement in which both arterial trunks are supported by the morphologically right ventricle. In most of these mice, the blocks are muscular (Fig. 6a, b). In some of the mice, however, the outflow cushions fail to myocardialise. The outlet septum, which does not exist in the normal heart (Fig. 5), is then seen as a fibrous entity (Fig. 6c). In the normal heart, furthermore, the part of the inner heart curvature, or ventriculo-infundibular fold, transferred to the left ventricle along with the aortic root remodels to become the area of mitral-to-aortic fibrous continuity. In the setting of deficient ventricular septation, the inner heart curve can again persist as the muscular ventriculo-infundibular fold (Fig. 6). It can also remodel to become a fibrous area of arterial-to atrioventricular valvar continuity. During early development, the ventricular walls themselves are largely made up of trabeculations. It is these trabeculations that coalesce to form the papillary muscles of the atrioventricular valves. In the right ventricle, they also form the septomarginal and septoparietal trabeculations (Fig. 2a). The trabeculations that coalesce to become the septomarginal trabeculation reinforce the site of attachment of the muscularised proximal cushions to the muscular septum itself.

Figure 4. The panels are from mouse embryos programmed to show the presence of myocardium (yellow areas in panel A) and the contributions made from the second heart field (yellow areas in panel B). Panel A shows the location of the myosin light chain protein, while panel B shows the site of the Islet-1 gene, which marks the right ventricle and the outflow myocardium.

Figure 5. The drawings show the changes that take place as the muscularised proximal cushions are converted to become the free-standing infundibular sleeve of the right ventricle subsequent to closure of the embryonic aorto-right ventricular communication. Panel A shows that arrangement whilst both arterial roots are supported above the cavity of the developing right ventricle. The channel between the ventricles is the secondary interventricular communication. It opens to the right ventricle between the limbs of the septomarginal trabeculation, shown by the yellow Y, which is formed by coalescence of the ventricular trabeculations. Panel B shows the arrangement subsequent to closure of the tertiary interventricular communication and formation of the membranous septum. The muscularised proximal cushions have been inserted into the muscular septum between the limbs of the septomarginal trabeculation.

Figure 6. The images are taken from episcopic datasets from biologically perturbed murine embryos. In the mouse shown in panels A and B, the mother was reared in abnormal concentrations of oxygen. There is a double outlet right ventricle with a subaortic defect. The images show how the building blocks of the right ventricle have retained their individuality. The dotted lines in panel B show the structures forming the area around which a partition would need to be placed to connect the aortic root with the left ventricle. The white dashed line is the parietal wall of the right ventricle. Panel C is from a mouse in which the Furin enzyme was perturbed. The proximal outflow cushions have failed to muscularise, leaving a fibrous outlet septum and double outlet right ventricle. The embryo shown in panels A and B was produced by Dr. Duncan Sparrow and is reproduced with his permission. The embryo shown in panel C was prepared by Dr. Tim Mohun and again is reproduced with his permission.
Divorce of the building blocks with deficient ventricular septation
In the normal heart, as already emphasised, the inner heart curvature and the muscularising proximal cushions unite to become the supraventricular crest. Reference Aiello, Spicer, Anderson, Brown and Mohun3 Part of the crest continues distally to become the posterior part of the free-standing infundibular sleeve. The crest attaches to the ventricular septum between the limbs of the septomarginal trabeculation, also known as the septal band (Fig. 5). There are no anatomical landmarks in the normal heart that permit any part of the right ventricular outflow tract to be identified as a muscular outlet septum. When the ventricular septum is deficient, however, it does become possible to recognise these building blocks as independent entities, as shown in the images obtained from episcopic datasets prepared from genetically perturbed murine embryos (Fig. 6). The building blocks can also be recognised in autopsy specimens. In hearts with perimembranous defects, for example, the subpulmonary infundibulum usually remains as a free-standing muscular sleeve. It inserts to the crest of the muscular septum, with the site of insertion, in the absence of malalignment, being reinforced by the limbs of the septomarginal trabeculation (Fig. 7). The leading edge of the sleeve, which in the normal heart has been incorporated within the supraventricular crest, can now be recognised in its own right as the muscular outlet septum. The cranial margin of the defect is the right ventricular part of the initial inner heart curvature. This supports the small part of the aortic root that overrides the crest of the muscular ventricular septum. It interposes between the leaflets of the aortic and tricuspid valves. The infero-posterior part of the defect is the area of fibrous continuity between the leaflets of the mitral and tricuspid valves, which we now recognise to be the defining feature of all perimembranous defects. Reference Tretter, Tran and Gray8 The defects, which extend from the subaortic outflow tract of the left ventricle, open to the right ventricle above the crest of the muscular ventricular septum, which in the absence of malalignment is reinforced by the caudal limb of the septomarginal trabeculation. The opening is posterior when assessed relative to the muscularised part of the outflow cushions now recognisable as the muscular outlet septum (Fig. 7).

Figure 7. The image shows the make-up of the boundaries of a perimembranous defect without malalignment of the outlet septum as seen from the right ventricular aspect. It is the fibrous continuity as marked between the leaflets of the mitral and tricuspid valves that makes the defect perimembranous. Note that the muscular outlet septum inserts between the limbs of the septomarginal trabeculation, with the septomarginal trabeculation reinforcing the crest of the muscular ventricular septum. The white stars with red borders show the septoparietal trabeculations. The colour coding of the components of the borders of the defect is used for subsequent images.
The situation is subtly different when a perimembranous defect is associated with malalignment of the outlet septum, although the margins of the defect to be closed to restore septal integrity remain comparable, albeit with differing dimensions when compared to the defect found in the absence of malalignment. In the presence of a malaligned outlet septum, the component of the infundibular sleeve derived from the muscularised cushions is attached cephalad to the anterior limb of the septomarginal trabeculation (Fig. 8). The cranial margin of the defect is then a more extensive area of the right ventriculo-infundibular fold. The postero-inferior margin of the defect remains the area of fibrous continuity between the leaflets of the mitral and tricuspid valves. The defect continues to open across the crest of the muscular septum from the space subtended beneath the aortic root to the right ventricle. With this variant, however, the opening is cradled between the limbs of the septomarginal trabeculation. When the subpulmonary outlet is not obstructed, the lesion with malalignment of the outlet septum is also known as the Eisenmenger defect. Reference Oppenheimer-Dekker, Gittenberger-de Groot, Bartelings, Wenink, Moene and Van der Harten9 Such malalignment defects opening to the right ventricular outlet can also have muscular postero-inferior rims.

Figure 8. The image shows the boundaries of a perimembranous outlet defect opening to the outlet of the right ventricle with malalignment of the outlet septum as seen from the right ventricular aspect. The white stars with red borders show septoparietal trabeculations.
The perimembranous defects can also be found with obstruction at the mouth of the subpulmonary infundibulum. This arrangement is now described as tetralogy of Fallot. Reference Bailliard and Anderson10 The hallmark of the lesion is the squeeze found at the mouth of the free-standing subpulmonary infundibular sleeve between the malaligned outlet septum and the septoparietal trabeculations (Fig. 9). The margins of the space existing between the right ventricle and the aortic root, however, when assessed on the basis of their anatomical make-up, remain directly comparable to those seen in the malalignment outlet perimembranous defect (Fig. 8). The cranial margin remains the right ventriculo-infundibular fold, interposing between the leaflets of the aortic and tricuspid valves. The anterior margin is the malaligned outlet septum, attached to the cranial limb of the septomarginal trabeculation. When the defect is perimembranous, its infero-posterior rim is the area of fibrous continuity between the leaflets of the mitral and tricuspid valves. The defect continues to open to the right ventricle between the limbs of the septomarginal trabeculation (Fig. 9). It used to be debated whether the difference between tetralogy of Fallot and double outlet right ventricle was the presence or absence of the subaortic infundibulum. Reference Walters, Mavroudis, Tchervenkov, Jacobs, Lacour-Gayet and Jacobs11 It is now recognised that there is no justification for this stance. In the first place, it abrogates the important “morphological method” established by Van Praagh and his colleagues. Reference Van Praagh, David, Wright and Van Praagh12 This concept rightly pointed out that one variable feature should not be defined on the basis of another feature that is itself variable. It is also now well established that a significant portion of hearts with double outlet right ventricle lack a subaortic infundibulum. Reference Ebadi, Spicer, Backer, Fricker and Anderson1 It is also the case that, when considering the overall spectrum of hearts having the phenotypic features of tetralogy of Fallot, the extent of aortic override can vary markedly, with some having concordant, but others having double outlet ventriculo-arterial connections (Fig. 10). Assessment of the channel between the ventricles in these settings is particularly pertinent in establishing the location of the “ventricular septal defect.”

Figure 9. The image shows the boundaries of a perimembranous outlet defect in the setting of tetralogy of Fallot as seen from the right ventricular aspect. The white stars with red borders are again showing the septoparietal trabeculations.

Figure 10. The drawings show how, irrespective of the degree of override of the aortic root in the setting of tetralogy of Fallot, when the space subtended beneath the aortic root is simplified so as to be represented by a triangle, the root has comparable right and left ventricular entrances.
Which area should we describe as the ventricular septal defect?
There is no right or wrong way of describing a “ventricular septal defect” when the defect itself is found at the margins of the muscular ventricular septum. Muscular defects are the true defects of the ventricular septum. Even these defects are not as straight forward as might be imagined. The muscular defect opening between the ventricular outlets is not a defect within the apical septum. Instead, it represents divorce between the apical septum and the muscularised proximal outflow cushions, with the muscular postero-inferior rim formed by fusion between the ventriculo-infundibular fold and the caudal limb of the septomarginal trabeculation, with the latter structure itself formed by coalescence of the embryonic ventricular trabeculations. The so-called “ventricular septal defect” in the setting of a common atrioventricular junction is also found at the margins of the apical muscular septum. This defect is the ventricular component of an atrioventricular septal defect. What, then, of the defects found in the setting of tetralogy of Fallot? Can all these channels properly be considered as “ventricular septal defects”? In this setting, there is an area of space subtended beneath the circumference of the overriding arterial root. Reference Anderson, Becker and Tynan13 The area of space is itself a complex three-dimensional entity. It can be simplified to a triangle when considered in terms of the arrangement seen in a long axial oblique view (Fig. 11). If considered during ventricular diastole, the area is delimited apically by the crest of the muscular septum, and distally by the closed leaflets of the arterial valve. Within the area in question, when simplified to a two-dimensional entity, any plane within it represents a “ventricular septal defect”. It is the entrances to the space subtended beneath the aortic root from the right and left ventricles that are of clinical relevance. The entrance to the space from the left ventricle is the outflow tract from the left ventricle. It is an aorto-left ventricular communication (Fig. 11). When considered in terms of the known steps of cardiac development, the aorto-left ventricular communication is the secondary interventricular foramen. The entrance to the space from the right ventricle is obviously an aorto-right ventricular communication. Such a space is also to be found in the perimembranous defects as encountered in hearts with concordant ventriculo-arterial connections (Fig. 12). In developmental terms, these aorto-right ventricular communications represent the tertiary interventricular foramen. Reference Anderson, Spicer, Mohun, Hikspoors and Lamers5 The right and left ventricular entrances to the aortic root, therefore, are both anatomically and developmentally discrete. The communication between the right ventricle and the space subtended beneath the aortic root remains comparable in all the variants of tetralogy, irrespective of the extent of aortic overriding (Fig. 10). It is also this communication between the right ventricle and the space beneath the aortic root that is named as the perimembranous ventricular septal defect when the ventriculo-arterial connections are concordant, with the aortic root being an integral part of the left ventricle in this setting (Fig. 12).
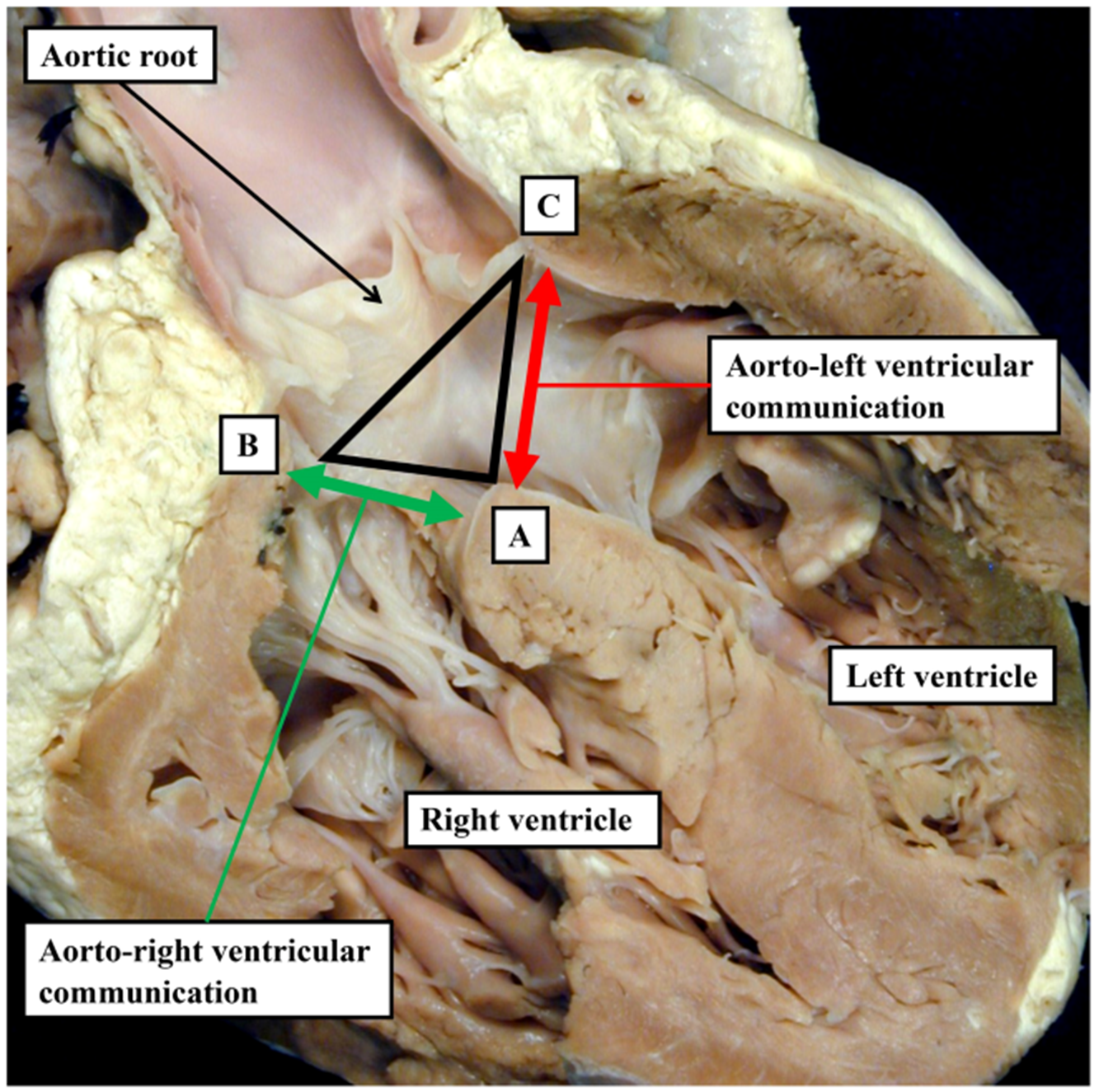
Figure 11. A specimen with tetralogy of Fallot has been sectioned along the long axis of the ventricular cone to show the overriding aortic root. The space subtended beneath the leaflets of the aortic valve has been simplified to a triangle. When the crest of the muscular ventricular septum is represented by point A, then the right ventricular entrance to the aortic root is line A–B, while the left ventricular entrance is line A–C. Comparable triangles are shown in Figures 12 and 15 for the regular perimembranous defect, and double outlet with subaortic defect, respectively.

Figure 12. A specimen with a perimembranous defect in a heart with concordant ventriculo-arterial connections has been sectioned in comparable fashion to the heart shown in Figure 11 with tetralogy of Fallot. The space beneath the aortic root, part of the cavity of the left ventricle in this setting, has been simplified to a triangle, as was the case in Figure 11. The line A–B is the communication between the cavity of the right ventricle and the space subtended beneath the aortic root. It is described as the perimembranous defect.
What about the situation in double outlet right ventricle with subaortic defect?
The arrangement to be found when both arterial trunks arise from the morphologically right ventricle, with the channel between the ventricles located in subaortic position, is comparable to the situation observed in the developing heart prior to the transfer of the aortic root to the left ventricle (Fig. 2). Such a heart is shown in Figure 13. As in the other hearts with deficient ventricular septation, there is divorce of the infundibular building blocks. In the heart shown in Figure 13, the ventriculo-infundibular fold, or inner heart curvature, has persisted beneath both arterial roots. Hence, the heart not only has double outlet right ventricle but also bilateral infundibulums, or conuses. Reference Walters2 The muscular outlet septum separates the arterial roots. As in the heart shown with tetralogy (Fig. 9), it is malaligned and inserted antero-cephalad relative to the septomarginal trabeculation, or septal band. The space between the limbs of the septal band is the outflow tract for the morphologically left ventricle. It is this area that, currently, is usually described as the “ventricular septal defect” when the aortic root is supported exclusively above the morphologically right ventricle, as shown in Figure 13. If described in terms of precise relationships, the area in question is the aorto-left ventricular communication. The area is of obvious clinical significance, since if restrictive, it will be the substrate for left ventricular outflow tract obstruction. It is not, however, the area around which the surgeon places the baffle so as to connect the aortic root with the cavity of the morphologically left ventricle, hence separating the blood streams and at the same time restoring septal integrity. The entirety of the margins of this area is of surgical significance since the sutures securing the baffle must be placed within those margins. Sections taken parallel to the ventricular septal surface (Fig 14A) do not demonstrate the full extent of the margins. Their full extent is seen only when the right ventricle itself is opened in clam-like fashion, as was the case in image shown in Figure 13. In this figure, the white dotted line shows the extensive area around which the surgeon would need to place a patch so as to baffle the aortic root into the left ventricle through the aorto-left ventricular communication. The area that is closed by the surgeon in this setting was initially a communication between the cavity of the right ventricle and the space subtended beneath the aortic root (Fig. 15). And, as can be seen from Figures 11 and 12, the comparable aorto-right ventricular communication in the setting of tetralogy and the perimembranous defect as found in hearts with concordant ventriculo-arterial connections is the one conventionally described as the “ventricular septal defect.” Its extent can now be demonstrated in the clinical setting when using computed tomography. In the example shown in Figure 14a, the channel shown is the aorto-left ventricular communication. This is the area revealed by the “en face” view of the ventricular septum. It is not the area that is closed by the surgeon during operative repair. This area is shown when the three-dimensional dataset is sectioned so as to show the arrangement at the base of the ventricular cone. This image (Fig. 14b) shows that, as in the specimens, when the defect opens adjacent to the aortic root, the area closed by the surgeon includes the crest of the muscular ventricular septum, the ventriculo-infundibular fold between the leaflets of the tricuspid and aortic valves, and the muscular outlet septum. Unlike the arrangements in tetralogy of Fallot and the regular perimembranous defects, it also includes part of the parietal wall of the right ventricle. The area closed would be subtly different, of course, if the defect in the setting of double outlet right ventricle opened in subpulmonary or doubly committed fashion. The concept would not be applicable in those examples of double outlet right ventricle with muscular defects in the apical septum. In all of the situations in which the concept is applicable, the complexity of the areas in question is not necessarily obvious when the arrangement is shown in two-dimensional fashion. The three-dimensional endocast image shown in Figure 16a, nonetheless, serves to show the difference between the area conventionally described as the “ventricular septal defect” in the setting of double outlet right ventricle when compared with the area that is filled by the surgeon during surgical repair when the defect itself is directly subaortic. Blood speckle-tracking echocardiography in the same patient highlights blood flow from both the channel from the interventricular communication to the space subtended beneath the aortic root, as well as the direct channel between the right ventricle and aortic root (Fig. 16b).
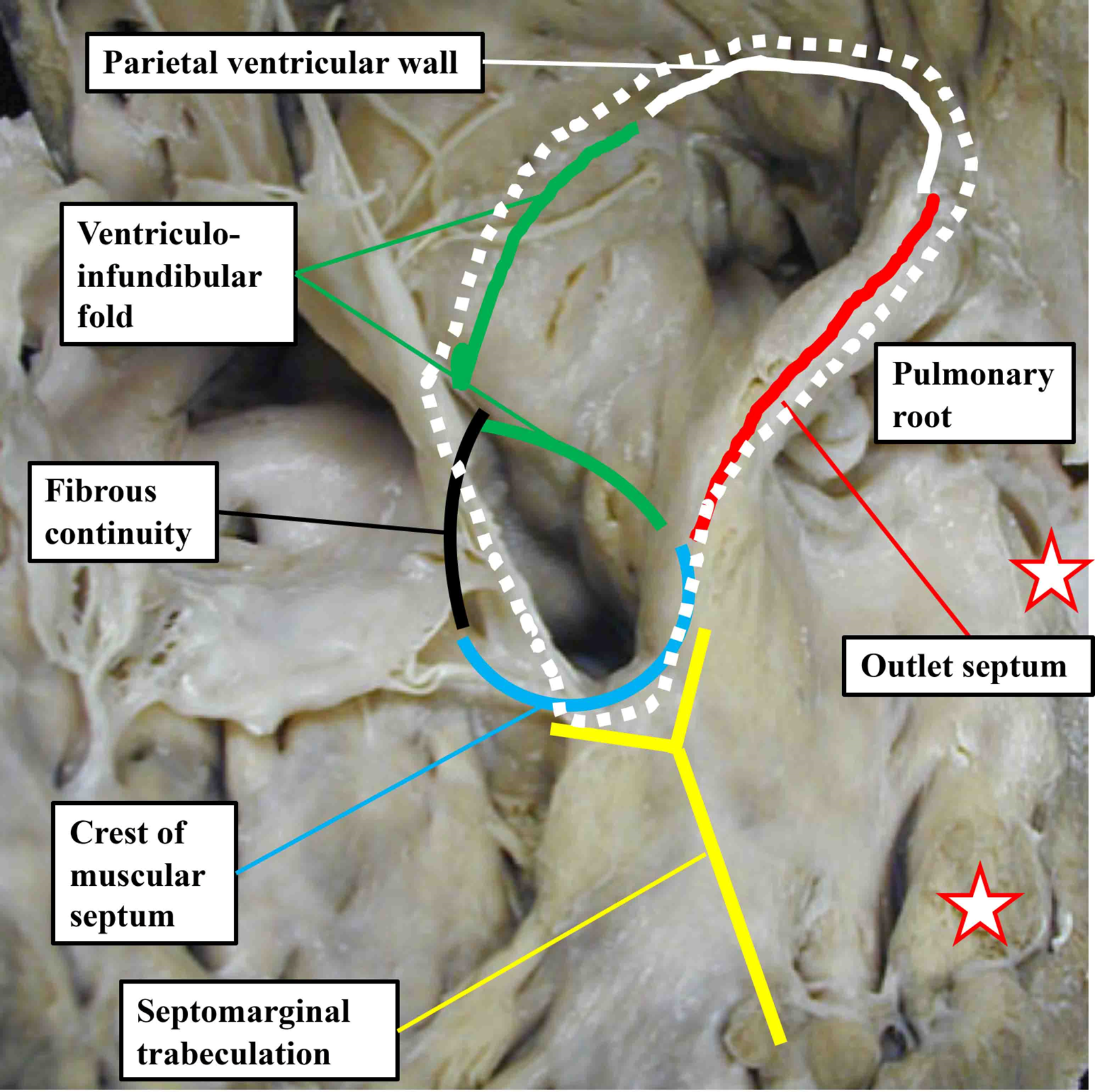
Figure 13. The specimen shown in this image has been prepared by opening the right ventricle in clam-like fashion. It shows the margins of the defect, which is the outflow tract for the left ventricle, and also, by the white dashed line, the area that will need to be closed by the surgeon so as to restore septal integrity. The arrangement can be compared with Figure 6B. The stars are coloured as in previous images. The area to be closed by the surgeon in this example will also include part of the parietal wall of the right ventricle.

Figure 14. The images are taken from a three-dimensional computed tomographic dataset prepared from a patient with double outlet right ventricle and subaortic defect. Panel A shows the “en face” view of the ventricular septum from the right ventricular aspect. The channel seen is the interventricular communication. This channel has to be tunnelled to the aortic root so as to restore septal integrity. The area around which sutures need to be placed to secure the partition to restore septal integrity can be seen only when assessing the base of the ventricular mass, as shown in Panel B. The parietal wall of the right ventricle is shown by the black dashed line. The other areas are coloured as for the previous figures.

Figure 15. A heart from a patient with double outlet right ventricle and subaortic defect has been sectioned in comparable fashion to the hearts shown in Figures 11 and 12. The space subtended beneath the aortic root has again been simplified to a triangle. The line A–C shows the outlet for the left ventricle. In most current settings, it is this line that is usually described as the “ventricular septal defect.” The line is obviously different from the line A–B, which was the area correctly described as the “ventricular septal defect” in the setting of the regular perimembranous lesion, and in tetralogy of Fallot.

Figure 16. The images are from the same patient as shown in Figure 14. Panel A shows an endocast computed tomographic reconstruction demonstrating the pathways between the left ventricle and right ventricle with the space subtended beneath the aortic root, with the pathways coloured red and green, respectively. An analogous two-dimensional echocardiographic plane in subcostal short axis with blood speckle tracking is demonstrated in Panel B, tracking blood flow from both the left ventricle- and right ventricle-aortic communications, respectively. The other areas are coloured as for the previous figures.
Comment
We hope we have shown that the space closed during normal cardiac development to complete ventricular septation, and separate the aortic and pulmonary blood streams, is an aorto-right ventricular communication. Subsequent to its closure, the space beneath the aortic root, initially part of the cavity of the right ventricle, is transformed to become the left ventricular outflow tract. When not closed during normal development, the channel is the area described as a perimembranous ventricular septal defect. It is a comparable space between the cavity of the right ventricle and the aortic root that is baffled by the surgeon to separate the blood streams when correcting the variant of double outlet right ventricle in which the interventricular communication is subaortic. But in the setting of double outlet right ventricle, it is the aorto-left ventricular communication that is usually described as the “ventricular septal defect.” It is a breach of linguistic practise for the same term to be used to account for both the aorto-right ventricular and the aorto-left ventricular communications. The channels could be differentiated by describing the hole found in double outlet right ventricle as the interventricular communication, rather than the ventricular septal defect. Reference Ebadi, Spicer, Backer, Fricker and Anderson1 This solution is appropriate in hemodynamic terms, since the channel is, indeed, the space permitting interventricular shunting. And, when the defect is subaortic, it is the aorto-right ventricular communication that is closed by the surgeon to separate the blood streams. As we have now shown, when simplified to a two-dimensional triangle, the area closed to separate the blood streams is comparable in terms of its borders to the perimembranous defects found with concordant ventriculo-arterial connections, the perimembranous defects found in tetralogy of Fallot, and the arrangement on double outlet right ventricle with subaortic defect (Compare Figs. 11, 12 and 15). Linguistic logic dictates that this area in all three lesions should be called the ventricular septal defect. But will this dissuade some investigators from continuing to account for the channel providing the outlet from the left ventricle in double outlet right ventricle also as the ventricular septal defect? Perhaps the better solution would be to distinguish the areas in question as the aorto-right ventricular and aorto-left ventricular communications? Both channels are of obvious clinical relevance. Understanding the differences between them might hasten the resolution of the dilemma of how best to describe them. We hope our review, at the least, has now demonstrated their anatomical differences.
Acknowledgements
We are indebted to Dr Tim Mohun, who prepared all the episcopic datasets used to prepare the figures showing the features of cardiac development whilst he was working at the Crick Institute in London. We also thank the staff of the Human Developmental Biology Resource at Newcastle University, who made the histological sections of the human embryos available for our use.
Financial support
This research received no specific grant from any funding agency, commercial, or not-for-profit sectors.
Competing interests
None.