Implications
Lactation in dairy ruminants is challenged by perturbations that can either be induced on purpose, such as by modifying management practices, or occur involuntarily, when adverse environmental constraints arise. These challenges can have immediate effects on milk yield and could be followed or not by carryover effects. A series of studies have investigated the cellular mechanisms involved in the process of adaptation of the mammary tissue to these challenges. An improved understanding of these mechanisms may help predict irreversible effects on the mammary tissue.
Introduction
During a female mammal’s life, the mammary gland undergoes many changes in size, structure, composition and activity. In dairy ruminants, females are subjected to successive reproductive cycles, and the mammary tissue adapts as it goes through the gestation, lactation and involution phases. During a normal lactation cycle, milk production follows a lactation curve (a strong increase up to a peak and then a gradual decrease) resulting from modulations of both the activity and the number of mammary secretory cells (Capuco et al., Reference Capuco, Wood, Baldwin, McLeod and Paape2001). Meanwhile, the mammary gland responds to perturbations that the animal must cope with. This organ is characterized by its capacity to adapt in response to changes to a wide variety of conditions. This adaptation capacity is referred to mammary tissue plasticity. The mammary tissue plasticity is a component of the robustness of the animal that can be defined as the ability of an animal to adapt to environmental constraints (Friggens et al., Reference Friggens, Sauvant and Martin2010).
Several transient external perturbations can challenge the lactating mammary gland. The word ‘challenge’ is used in order to conceptualize the action of an external perturbation that exercises a constraint to the ‘mammary tissue’ system and can stimulate or impair lactation. In sciences other than biology, scientists have questioned how a system can adapt to changing conditions (Sauvant and Martin, Reference Sauvant and Martin2010). The concepts developed in economics can be transposed to conceptualize how a biological system can adapt to challenges (Sauvant and Martin, Reference Littlejohn, Walker, Ward, Lehnert, Snell, Verkerk, Spelman, Clark and Davis2010). When an animal is subjected to a challenge, the mammary tissue sets up an adaptive response that involves biological processes, and the production of milk can be affected. The mammary tissue is thus a deformable system, characterized by its resistance and its resilience (Figure 1). Resistance could be defined as its ability to tolerate a disruption induced by a challenge and can be illustrated by the difference in milk yield before and during the application of the challenge. Resilience could be defined as the ability of a system to return to its initial state when a challenge is finished. The resilience of the mammary tissue can thus be characterized by the carryover effects on milk yield (Figure 1). If no carryover effect is observed (the system goes back to its initial state), then the mammary tissue shows elastic properties. In contrast, if some carryover effects are observed (milk yield remains different from before the challenge), then the mammary tissue shows flexible properties. Our assumption is that understanding the cellular mechanisms underlying the adaptation of the mammary tissue during the application of challenges could make it possible to understand why there are carryover effects on milk yield or not. The objective of this review is, therefore, to contribute to a better understanding of the cellular and molecular mechanisms involved in mammary tissue plasticity. We will limit the review to the mammary tissue and, particularly, to the mammary epithelial cells (MECs) to simplify the system, even though it is obvious that the challenges also lead to wide physiological modifications at the whole-body level (effects on blood flow, nutrient and hormone concentrations etc.). In this review, we will first describe the indicators of the potential mechanisms involved in mammary tissue plasticity. We will, then, describe how these mechanisms could be affected during the application of diverse challenges. And, finally, we will address the question of the carryover effects when the challenge is finished.

Figure 1 Schematic representation of the effect of a challenge (represented here with a negative effect on milk yield) on mammary tissue plasticity during lactation in ruminants as indicated by the variation in milk yield. The grey box indicates the phase when the challenge is applied, and t 0 corresponds to the time zero of the application of the challenge. The adaptation of the mammary tissue when animals are challenged during lactation can be characterized by an initial resistance phase followed by a resilience phase (Adapted from Sauvant and Martin, Reference Littlejohn, Walker, Ward, Lehnert, Snell, Verkerk, Spelman, Clark and Davis2010).
What are the indicators of the potential molecular and cellular mechanisms underlying mammary tissue plasticity?
Indicators of the secretory activity of the MECs
One of the main cellular mechanisms that may contribute to mammary tissue plasticity is the changes in the secretory activity of the MEC. The secretory activity of MEC can be analyzed in terms of enzyme activity and target-gene expression and using global transcriptomic or proteomic approaches. MECs express messenger RNA (mRNA) coding for milk-specific proteins and proteins necessary for the synthesis of milk constituents (enzymes, transporters, receptors, intra-cellular messengers etc.). Microarray and KEGG pathway analyses were used to provide a picture of the transcriptomic adaptations in the mammary tissue between the end of pregnancy and the onset of lactation, showing an overall induction of the metabolism categories, with the ‘lipid metabolism,’ ‘glycan biosynthesis and metabolism’ and ‘carbohydrate metabolism’ pathways being the most impacted (Bionaz et al., Reference Bionaz, Periasamy, Rodriguez-Zas, Everts, Lewin, Hurley and Loor2012). Another way to study the secretory activity of MEC is to target genes of interest. The main target genes studied are the ones coding for milk components such as milk proteins like casein αs1 type 1 (CSN1S1), αs1 type 2 (CSN1S2), β- (CSN2), or κ (CSN3) or α-lactalbumin (LALBA). This last gene is particularly interesting because α-lactalbumin is the co-factor of the enzyme galactosyltransferase (GT), which is involved in the last step of lactose synthesis. Since lactose is the main osmotic agent in most mammalian species, it has a prominent role in the determination of the volume of milk produced. Changes in mammary cell activity can also be shown through the activity of several key mammary enzymes, such as GT, acetyl-CoA carboxylase (ACC) and fatty acid synthase (FASN); the last two being involved in the synthesis of milk fat. It has been shown that the increase in milk production at the onset of lactation partly results from an increase in the activity of ACC, FASN and GT (Knight and Peaker, Reference Knight and Peaker1984). In addition, the proteome of milk or mammary tissue can also reveal changes in secretory cell activity.
Indicators of epigenetic modifications that may impact secretory cell activity
Epigenetic changes may participate in regulating mammary tissue plasticity. Indeed, epigenetic regulations may be partly responsible for the molecular regulation of milk constituent production. Studies in rodents or lagomorphs have shown that a combination of epigenetic events (chromatin opening, chromatin loop formation, histone acetylation and DNA methylation), on a global scale or targeted to specific genes, occurs during the development of the mammary gland and alters gene expression (Rijnkels et al., Reference Rijnkels, Kabotyanski, Montazer-Torbati, Hue Beauvais, Vassetzky, Rosen and Devinoy2010). In cattle, DNA is hypomethylated in the 5′ region of the αs1 casein gene when this gene is expressed (Platenburg et al., Reference Platenburg, Vollebregt, Karatzas, Kootwijk, De Boer and Strijker1996). It has been shown recently that the stage of mammary development modulates the degree of DNA methylation in the distal regulatory region upstream of this gene. This area of DNA contains three CpG islands near the binding sites of signal transducer and activator of transcription 5 (STAT5), a transcription factor involved in signal transduction of the hormone prolactin (Vanselow et al., Reference Vanselow, Yang, Herrmann, Zerbe, Schuberth, Petzl, Tomek and Seyfert2006). In the mammary gland, these CpG sites are less methylated (<30%) during lactation than during puberty, gestation or involution (between 35% and 60%), while being hypermethylated in the liver (>65%; Vanselow et al., Reference Vanselow, Yang, Herrmann, Zerbe, Schuberth, Petzl, Tomek and Seyfert2006; Singh et al., Reference Singh, Molenaar, Swanson, Gudex, Arias, Erdman and Stelwagen2012; Nguyen et al., Reference Nguyen, Boutinaud, Pétridou, Gabory, Pannetier, Chat, Bouet, Jouneau, Jaffrezic, Laloë, Klopp, Brun, Kress, Jammes, Charlier and Devinoy2014). The high level of DNA methylation at these three CpG sites during mammary involution was associated with a low expression of the CSN1S1 gene (Singh et al., Reference Singh, Molenaar, Swanson, Gudex, Arias, Erdman and Stelwagen2012). Epigenetic changes, such as DNA methylation, may occur during lactation and may result in long-term modulation of gene expression, thus affecting cell activity for a long period.
Indicators of the number of MECs
Another cellular mechanism that may contribute to mammary tissue plasticity is changes in the number of MEC in the mammary tissue. This number results from changes in the rates of cell proliferation and cell loss. The loss of MEC in the mammary tissue has been first considered to be due to cell death. However, MEC can be lost from the mammary tissue through exfoliation of MEC from the mammary epithelium into milk during lactation (Herve et al., Reference Herve, Quesnel, Lollivier and Boutinaud2016). The number of MEC in the goat mammary gland increases slightly during early lactation up until the peak of lactation (Knight and Peaker, Reference Knight and Peaker1984). After this, the gradual decrease in milk yield is associated with a decrease in MEC number in the mammary gland, due to a greater cell apoptosis rate than cell proliferation rate (Knight and Peaker, Reference Knight and Peaker1984; Capuco et al., Reference Capuco, Wood, Baldwin, McLeod and Paape2001) and a gradual increase in the MEC exfoliation rate (Herve et al., Reference Herve, Quesnel, Lollivier and Boutinaud2016). Standard indicators of variations in MEC number in the mammary tissue correspond to histological analyses that allow the rates of cell proliferation or cell death to be measured (Capuco et al., Reference Capuco, Wood, Baldwin, McLeod and Paape2001). Moreover, DNA concentration or overall DNA content in the mammary tissue (Capuco et al., Reference Boutinaud, Galio, Lollivier, Finot, Wiart, Esquerre and Devinoy2001) and the expression of genes involved in cell proliferation such as ATP-binding cassette super-family G member 2 (ABCG2), CCAAT enhancer binding protein delta (CEBPD), clusterin (CLU) and integrin subunit beta 6 (ITGB6) and cell death such as Bcl-2-modifying factor (BMF), insulin-like growth factor binding protein 5 (IGFBP5), Cathepsin B (CTSB), B-cell lymphoma 2 (BCL2) and BCL2 associated X (BAX) and caspases can also be indicators of variations in the number of MEC in the mammary gland (Boutinaud et al., Reference Boutinaud, Galio, Lollivier, Finot, Wiart, Esquerre and Devinoy2013).
Mammary stem cells may impact the number of MECs in the mammary tissue
The ability of the mammary gland to undergo many cycles of cell proliferation and tissue involution through successive gestations and lactations highlights the existence of resident cells able to regenerate the entire epithelial tissue. As in other somatic tissues, stem cells are believed to exist during the entire lifecycle of the mammary gland, thus representing a key mechanism in the plasticity of this organ. The mammary stem cells (MaSC) are required to generate the various cell populations in different lineages (epithelial and myoepithelial) during pregnancy and might sustain cell turnover to replace cells during lactation. Although research on MaSC has focused mainly on the murine and human models, pioneer studies in bovine identified a population of lightly stained cells with high proliferative capacity within the mammary parenchyma of the heifer mammary gland that may be the putative MaSC (Ellis and Capuco, Reference Ellis and Capuco2002). More recently, when flow cytometry was used to define the MaSC by the co-expression of cell surface proteins CD49f and CD24, it was found that the proportion of MaSC was stable during a cow lactation cycle (Perruchot et al., Reference Perruchot, Arévalo-Turrubiarte, Dufreneix, Finot, Lollivier, Chanat, Mayeur and Dessauge2016) but was markedly decreased at drying-off (Finot et al., Reference Finot, Chanat and Dessauge2018). These studies agree with the notion that the MaSC and their progeny may play a key role in mammary tissue regeneration. The regulation of the number of MaSC and their involvement in mammary tissue plasticity during cow lactation remain to be clarified.
What are the adaptation processes of the mammary tissue when lactation is challenged?
The challenges, including changes in milking frequency and level of feed supply and hormonal and heath manipulations, have been shown to modulate milk yield in association with changes in the mammary tissue functioning. To identify the mechanisms involved in the plasticity of the ruminant mammary gland, we analyzed mammary tissue responses to challenges that were experimentally applied during lactation.
Effect of challenges on secretory cell activity
The adaptation of the mammary tissue to milking frequency has been widely studied (Table 1). The decrease in milk yield observed after a reduction in milking frequency from twice daily (2×) to once daily (1×) was accompanied by a reduction of ACC and FASN enzyme activity (Farr et al., Reference Farr, Stelwagen, Kerr, Davis and Eichler1995). Conversely, the increase in milk yield in response to a high milking frequency per day (3× or 4×) was associated with an increase of mammary enzyme activity in the first days after the milking frequency was modified (Wilde et al., Reference Wilde, Henderson, Knight, Blatchford, Faulkner and Vernon1987; Travers and Barber, Reference Travers and Barber1993). After several weeks of 3× milking, FASN, GT and ACC activities were no longer different than during 2× milking (Wilde et al., Reference Wilde, Henderson, Knight, Blatchford, Faulkner and Vernon1987; Nørgaard et al., Reference Nørgaard, Sørensen, Sørensen, Andersen and Sejrsen2005), whereas greater glucose-6-phosphate dehydrogenase, lactate dehydrogenase and hexokinase activities were reported (Wilde et al., Reference Wilde, Henderson, Knight, Blatchford, Faulkner and Vernon1987). Regardless of whether a target-gene or transcriptomic approach was used (Table 1), most studies showed an increase in the expression of the genes involved in the synthesis of milk when milking frequency was increased and, accordingly, a decrease when milking frequency was reduced.
Table 1 Effects of the modification of milking frequency on indicators of secretory cell activity in the mammary tissue of dairy cows and goats)
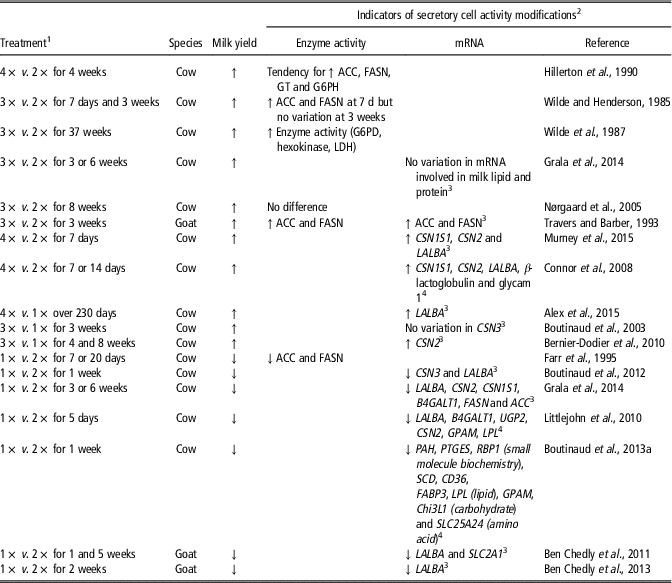
1 1×, 2×, 3× or 4× for milking one, two, three or four times daily, respectively.
2 Abbreviations are listed in the Supplementary material (S1).
3 Target-gene approach was taken.
4 Transcriptomic approach was taken.
The effect of feeding level manipulation on mammary secretory activity has not been as extensively investigated (Table 2). Feed or energy restriction has been found to induce a loss of milk yield. One study showed that the reduction of milk yield with energy restriction in mid-lactation dairy cows was associated with a tendency for lower GT enzyme activity (Nørgaard et al., Reference Nørgaard, Sørensen, Sørensen, Andersen and Sejrsen2005). Moderate feed restriction (30% and 20% less than the control diet) increased milk protein transcripts in early lactation (Sigl et al., Reference Sigl, Meyer and Wiedemann2014) but did not affect them in a later stage of lactation (Boutinaud et al., Reference Boutinaud, Ben Chedly, Delamaire and Guinard-Flament2008; Herve et al., Reference Herve, Quesnel, Veron, Portanguen, Gross, Bruckmaier and Boutinaud2018). In contrast, a severe feed restriction in early lactation that reduced milk production by 38% was accompanied by decreases in LALBA and CSN3 gene expression (Dessauge et al., Reference Dessauge, Lollivier, Ponchon, Bruckmaier, Finot, Wiart, Cutullic, Disenhaus, Barbey and Boutinaud2011), with no effect on CSN1S1 mRNA level. In another study, a severe feed restriction (40% less than the control diet) in mid-lactation dairy cows reduced the expression of genes involved in lipid metabolism (Abdelatty et al., Reference Abdelatty, Iwaniuk, Garcia, Moyes, Teter, Delmonte, Kadegowda, Tony, Mohamad and Erdman2017). Thus, the effect of feed restriction on secretory cell activity likely depends on the severity of the challenge and the timing of its application.
Table 2 Effects of the modification of feeding level on indicators of secretory cell activity in the mammary tissue of dairy cows

1 Abbreviations are listed in the Supplementary material S1.
2 FR, feed restriction.
Mastitis is also a challenge that has a temporary negative effect on milk production and may, therefore, affect secretory cell activity (Table 3). Lipopolysaccharide-induced mastitis downregulated LALBA gene expression but not CSN1S1 gene expression in bovine mammary tissue (Gross et al., Reference Gross, van Dorland, Wellnitz and Bruckmaier2015). A downregulation of genes involved in lipid synthesis was also reported after challenges with lipopolysaccharide (Gross et al., Reference Gross, van Dorland, Wellnitz and Bruckmaier2015) or Streptococcus uberis (Moyes et al., Reference Moyes, Drackley, Morin, Bionaz, Rodriguez-Zas, Everts, Lewin and Loor2009). In addition, a clear reduction in the content of milk proteins involved in the synthesis of milk (casein, α-lactalbumin, GT and β-lactoglobulin) was observed by means of proteomic approaches (Table 3). These results suggest that mammary tissue inflammation affects the activity of the MEC.
Table 3 Effects of inflammation induced by intramammary infection or infusion of LPSFootnote 2 on indicators of secretory cell activity in the mammary tissue of dairy ruminant
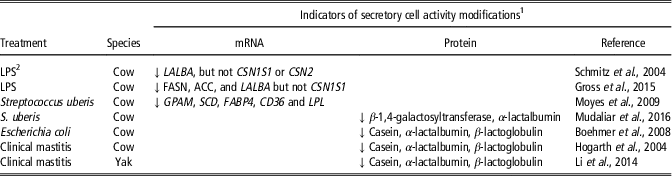
1 Abbreviations are listed in the Supplementary material S1.
2 LPS=Lipopolysaccharides
To understand the role of hormones in the adaptation process of the mammary tissue, milk production was modulated by hormonal manipulations (Table 4). The withdrawal of ovarian steroid secretion following ovariectomy limited the rate of decrease in milk yield after the peak of lactation (Yart et al., Reference Yart, Dessauge, Finot, Barbey, Marnet and Lollivier2012). The reduction of milk yield decline was not accompanied by the modification of milk protein gene expression Boutinaud et al., Reference Boutinaud, Yart, Debournoux, Wiart, Finot, Le Guennec, Marnet, Dessauge and Lollivier2013b. In contrast, long-term inhibition of prolactin secretion using quinagolide, a dopamine agonist, induced a reduction in milk yield (Lacasse et al., Reference Lacasse, Lollivier, Bruckmaier, Boisclair, Wagner and Boutinaud2011) accompanied by decreases in LALBA and CSN3 mRNA levels (Boutinaud et al., Reference Boutinaud, Lollivier, Finot, Bruckmaier and Lacasse2012).
Table 4 Effects of hormonal manipulations on indicators of secretory cell activity in the mammary tissue of dairy cows

1 Abbreviations are listed in the Supplementary material S1.
For most of the challenges, the impact on milk yield (either positive or negative) was associated with a parallel variation of the secretory cell activity. This suggests that the change in cell activity, regulated through the modification of gene expression, enzyme activity and protein synthesis, is generally involved in the adaptation process of the mammary tissue.
Effect of challenges on epigenetic marks
The first study showing the effects of a challenge on epigenetic marks during lactation involved manipulation of the health status. An experimentally induced infection with Escherichia coli induced an increase in the level of methylation of the three CpG sites upstream of the CSN1S1 gene and a condensation of the chromatin associated with a decrease in the CSN1S1 RNA level (Vanselow et al., Reference Vanselow, Yang, Herrmann, Zerbe, Schuberth, Petzl, Tomek and Seyfert2006). Similar effects were observed following infection with S. uberis (Singh et al., Reference Singh, Molenaar, Swanson, Gudex, Arias, Erdman and Stelwagen2012). Milking frequency has also been shown to modify the epigenetic marks on the CSN1S1 locus. During differential milking, the milk yield of the half-udder milked 1× was lower than its contralateral half-udder milked 2×. This reduction in milk yield was associated with greater CpG methylation levels upstream of the CSN1S1 gene and a lower RNA level of this gene in the 1×-milked half-udder than in the 2×-milked half-udder (Nguyen et al., Reference Nguyen, Boutinaud, Pétridou, Gabory, Pannetier, Chat, Bouet, Jouneau, Jaffrezic, Laloë, Klopp, Brun, Kress, Jammes, Charlier and Devinoy2014). In contrast, a severe and long-term feed restriction during early lactation in dairy cows did not affect the levels of CSN1S1 mRNA and CpG methylation upstream of this gene (Boutinaud et al., Reference Boutinaud, Lollivier, Dessauge, Devinoy and Singh2016). Although still scarce, studies evaluating epigenetic modulation in the mammary tissue suggest that epigenetic modifications are involved in the adaptive response of the mammary tissue to a challenge and modulate the secretory activity of MEC.
Effect of challenges on mammary cell number
The effect of milking frequency on mammary cell number is not always consistent from one study to another (Table 5). Nevertheless, when milking frequency is increased, it generally induced an increase in milk yield associated with an increase in the number of MEC in the tissue, and the contrary is observed when the milking frequency is reduced. Increasing milking frequency generally increases the rate of cell proliferation and reduces that of apoptosis (Table 5). In some studies, however, cell proliferation or cell apoptosis was not affected with either an increase or a decrease in milking frequency (Nørgaard et al., Reference Nørgaard, Sørensen, Sørensen, Andersen and Sejrsen2005; Wall and McFadden, Reference Wall and McFadden2012; Ben Chedly et al., Reference Ben Chedly, Lacasse, Marnet and Boutinaud2013). Furthermore, cell proliferation was surprisingly reduced (Bernier-Dodier et al., Reference Bernier-Dodier, Delbecchi, Wagner, Talbot and Lacasse2010), and cell apoptosis surprisingly increased by an increased milking frequency (Connor et al., Reference Connor, Siferd, Elsasser, Evock-Clover, Van Tassell, Sonstegard, Fernandes and Capuco2008). In most studies, milking frequency affected the expression of genes involved in cell proliferation and apoptosis, regardless of whether the analysis used the target-gene or transcriptomic approach (Table 5). Other indicators also suggest variations in cell number in the mammary tissue following modification of milking frequency. For instance, mean alveolar area, or the number of MEC per alveolus, were increased by greater milking frequency (Hillerton et al., Reference Hillerton, Knight, Turvey, Wheatley and Wilde1990; Boutinaud et al., Reference Boutinaud, Rousseau, Keisler and Jammes2003). In line with this, total DNA content, or DNA concentration, was also shown to increase in the mammary gland of dairy goats when milking frequency was increased (Boutinaud et al., Reference Boutinaud, Rousseau, Keisler and Jammes2003). However, this was not observed by Nørgaard et al. (Reference Nørgaard, Sørensen, Sørensen, Andersen and Sejrsen2005) in a study performed in dairy cows. For MEC exfoliation, 7 days of 1× milking in comparison with 2× milking did not affect MEC exfoliation in dairy goats and cows, whereas several weeks of 1× milking induced a marked increase in MEC exfoliation in goats (Herve et al., Reference Herve, Quesnel, Lollivier and Boutinaud2016). Thus, mammary cell number appears to be generally affected by milking frequency.
Table 5 Effects of the modification of milking frequency on indicators of cell number variation in the mammary tissue of dairy cows and dairy goats (1×, 2×, 3× or 4× for milking one, two, three or four times daily, respectively)

1 1×, 2×, 3× or 4× for milking one, two, three or four times daily, respectively.
2 Abbreviations are listed in the Supplementary material S1.
3 Target-gene approach was taken.
4 Transcriptomic approach was taken.
Similarly to milking frequency, feed restriction affected the indicators of variation of cell number differently depending on the study (Table 6). Indeed, the rate of mammary cell proliferation was reported to be decreased by a restriction of the energy in the diet (Nørgaard et al., Reference Nørgaard, Sørensen, Sørensen, Andersen and Sejrsen2005) but not by a feed restriction (Dessauge et al., Reference Dessauge, Lollivier, Ponchon, Bruckmaier, Finot, Wiart, Cutullic, Disenhaus, Barbey and Boutinaud2011; Herve et al., Reference Herve, Quesnel, Veron, Portanguen, Gross, Bruckmaier and Boutinaud2018). In addition, the rate of cell apoptosis was enhanced by feed restriction during early lactation (Dessauge et al., Reference Dessauge, Lollivier, Ponchon, Bruckmaier, Finot, Wiart, Cutullic, Disenhaus, Barbey and Boutinaud2011) but not when applied in a later stage of lactation (Herve et al., Reference Herve, Quesnel, Veron, Portanguen, Gross, Bruckmaier and Boutinaud2018) or by energy restriction (Nørgaard et al., Reference Nørgaard, Sørensen, Sørensen, Andersen and Sejrsen2005). The effects of feed restriction on MEC exfoliation are also not consistent. The exfoliation of MEC was enhanced during a 4 weeks feed restriction (Herve et al., Reference Herve, Quesnel, Veron, Portanguen, Gross, Bruckmaier and Boutinaud2018) but not after 2 weeks of feed restriction of similar intensity (Herve et al., Reference Herve, Quesnel, Lollivier and Boutinaud2016). The finding that cell apoptosis and proliferation and MEC exfoliation are most often affected by feed restriction suggests that the number of mammary cells decreases with milk yield during feed restriction, as also suggested by the decrease in total DNA content (Dessauge et al., Reference Dessauge, Lollivier, Ponchon, Bruckmaier, Finot, Wiart, Cutullic, Disenhaus, Barbey and Boutinaud2011).
Table 6 Effects of the modification of the feeding level on indicators of cell number variation in the mammary tissue of dairy cows

1 Abbreviations are listed in the Supplementary material S1.
2 FR, feed restriction.
Mastitis may also affect MEC number in the mammary tissue. The reduced ability of cows with mastitis to synthesize and secrete milk has been related to an increased apoptosis and sloughing of MEC in the mammary tissue (Akers and Nickerson, Reference Akers and Nickerson2011). In addition, endotoxin administration strongly increased the percentage of milk MEC in the total somatic cell population (Wagner et al., Reference Wagner, Jones and Apley2009). This finding also suggests that mastitis affects the number of mammary cells by influencing MEC exfoliation.
Hormonal manipulations also affected indicators of cell number variations in the mammary tissue (Table 7). Greater milk yield in ovariectomized cows than in control cows was associated with a decrease in the expression of the enzyme poly ADP ribose polymerase (PARP) protein (Yart et al., Reference Yart, Dessauge, Finot, Barbey, Marnet and Lollivier2012), which intervenes in DNA fragmentation during one of the final stages of cell death. This effect was accompanied by lower MEC exfoliation (Boutinaud et al., Reference Boutinaud, Yart, Debournoux, Wiart, Finot, Le Guennec, Marnet, Dessauge and Lollivier2013b). Both indicators suggest lower losses of MEC in the mammary tissue in ovariectomized cows. The lower milk yield in cows treated with a prolactin inhibitor in comparison with untreated cows was associated with either a decrease (Boutinaud et al., Reference Boutinaud, Lollivier, Finot, Bruckmaier and Lacasse2012) or no variation (Lollivier et al., Reference Lollivier, Lacasse, Angulo Arizala, Lamberton, Wiart, Portanguen, Bruckmaier and Boutinaud2015) in the rate of cell proliferation, an increase in the rate in cell apoptosis (Boutinaud et al., Reference Boutinaud, Lollivier, Finot, Bruckmaier and Lacasse2012) and an increase (Lollivier et al., Reference Lollivier, Lacasse, Angulo Arizala, Lamberton, Wiart, Portanguen, Bruckmaier and Boutinaud2015) in MEC exfoliation. Taking together, these effects suggest a reduction in mammary cell number with the prolactin inhibitor.
Table 7 Effects of hormonal manipulations on indicators of cell number variation in the mammary tissue of dairy cows

1 Abbreviations are listed in the Supplementary material S1.
The adaptation of the mammary tissue during a challenge does not necessarily involve all processes related to modifications in cell number (proliferation, death and MEC exfoliation), but most of the indicators show a change in the number of cells in the same direction as the change in milk production. The fact that variations in gene expression involved in cell turnover were always observed, whereas the rates of cell proliferation and apoptosis were not always affected, may be due to methodological limits of the immunohistological analyses or due to the lack of representativeness of the mammary tissue samples collected. Indeed, many of the studies were conducted using mammary biopsy. This would not have captured changes in total cell number or in rates of quiescence and senescence within the whole gland (Wall and McFadden, Reference Wall and McFadden2008).
Initial conclusion about the adaptation processes of the mammary tissue when lactation is challenged
The cellular mechanisms underlying mammary tissue plasticity in response to challenges are diverse. The mammary tissue adapts to the challenges by modifying its secretory activity and/or number of cells. Part of the mechanisms responsible for the change in secretory activity involves epigenetic modifications. In most studies, not all processes are studied at once, and there is no clear description of each potential process of adaptation. Moreover, the lack of variation of some indicators in some studies suggests that not all mechanisms are involved in the milk yield response at the same time. The response of the mammary tissue could depend on the type of challenge (energy or global feed restriction for cell proliferation and cell death), the intensity of the challenge (as observed for feed restriction), the length of the challenge (number of weeks of modified milking frequency for enzyme activity or number of weeks of feed restriction for MEC exfoliation), and the stage of lactation when the challenge is applied (observed for both feed restriction and changes in milking frequency). For some challenges, the adaptation of the mammary tissue also differs according to species, as seems to be the case for the effect of milking frequency on total DNA content. Since the adaptation of the mammary tissue is time-dependent, it is important to develop experimental designs that provide the possibility of performing dynamic studies. This would permit catching the window of time when the adaptive phenomenon occurs. In most of the studies, the analysis of mammary functions was performed at steady state, when the adaptation had already occurred, which most likely explains why no effects were observed. The most appropriate timing for these analyses may be when milk yield begins to be affected.
Do the mechanisms underlying mammary tissue plasticity participate to the carryover effects?
Theoretical impact of challenges on the resilience of the mammary tissue
The first two parts of this review focused on the first step of the process of adaptation, that is resistance, to explain how the mammary tissue adapts to a challenge. It has been shown that the mammary tissue is a deformable system and that its adaptation to new conditions is accompanied by a variation in the amount of milk produced. The next part of this review focuses on the resilience of the mammary tissue and discusses whether the adaptation of the tissue is reversible or not. Irreversible processes could explain the carryover effects on milk yield when the challenge is terminated. The effects may become irreversible when the intensity of the challenge (length or strength) reaches a threshold that could lead to homeostatic ruptures. Most of the works dedicated to the effects of challenges have analyzed the effects on mammary functions only during the application of the challenge. It is, therefore, not possible to deduce the permanency of the effects on mammary functioning from those experiments. For a long time, it has been assumed that short-term modulations of milk production without carryover effects were due to changes in cell activity, while modulations of lactation that extended beyond the end of the challenge were due to changes in the number of MEC. However, a recent discovery has shown that environmental events can lead to stable changes in gene expression through modification of DNA methylation. This suggests that carryover effects might not only due to changes in the number of MEC. Therefore, it is necessary to not only determine if a challenge has carryover effects but also the mechanisms involved. We will focus on the effect of milking frequency and feed restriction, which are the most investigated challenges.
Changing milking frequency: an interesting model to investigate mammary tissue resilience
The carryover effects of changing milking frequency on milk yield have been described in the literature (Stelwagen, Reference Stelwagen2001; Rémond and Pomiès, Reference Rémond and Pomiès2005; Wall and McFadden, Reference Wall and McFadden2012; Charton, Reference Charton2017). Such effects clearly depend on the duration of the treatment. Indeed, reducing milking frequency from 2× to 1× for 1 week has no persistent effect on milk yield, whereas a residual milk loss of 10% was observed after 7–10 weeks of 1× milking (Rémond and Pomiès, Reference Rémond and Pomiès2005). The impact of the modification of milking frequency for a short period is also modulated by the lactation stage during which the challenge is applied. The deleterious impact of a short-term application of 1× milking was greater during early lactation than at a later stage of lactation (Rémond and Pomiès, Reference Rémond and Pomiès2005). With respect to a persistent increase in milk yield with increased milking frequency, Wall and McFadden (Reference Wall and McFadden2012) suggested that the timing (between day 7 and 21 of lactation) may be more critical than the duration. The mammary gland may be especially responsive to the stimulus of milk removal for a window of time at the beginning of lactation. Frequent milking during this time can, indeed, elicit a persistent increase in milk production for the remainder of the lactation (Wall and McFadden, Reference Wall and McFadden2008). However, one study reported that increased milking frequency for the first 3 weeks induced only a transient carryover effect (over less than 2 weeks) (Nørgaard et al., Reference Nørgaard, Sørensen, Sørensen, Andersen and Sejrsen2005).
In parallel with a more pronounced carryover effect when milking frequency challenge is longer (Rémond and Pomiès, Reference Rémond and Pomiès2005), it was suggested that milking frequency first induced changes in secretory activity followed by an effect on the number of mammary cells (Stelwagen, Reference Stelwagen2001). These observations argue in favor of the idea that the variation in cell number during a milking frequency challenge induces persistent effects. Accordingly, a persistent increase in milk yield occurred when cell proliferation tended to be increased by more frequent milking (Hale et al., Reference Hale, Capuco and Erdman2003). In addition, no carryover effect was observed after increased milking frequency when a lack of effect on cell number had been observed (Nørgaard et al., Reference Nørgaard, Sørensen, Sørensen, Andersen and Sejrsen2005). However, a persistent effect on milk yield was also observed even though no changes in mammary proliferation and apoptosis rate was measured during the challenge period (Wall and McFadden, Reference Wall and McFadden2012). This contrasts with the idea that the change in MEC number is what causes the carryover effects. Moreover, the induction of MEC exfoliation during 1× milking in dairy goats was not accompanied by a carryover effect on milk yield (Ben Chedly et al., Reference Ben Chedly, Lacasse, Marnet and Boutinaud2013). The carryover effect of milking frequency could thus be explained by other mechanisms than change in cell number. Indeed, two studies performed in bovine showed that the carryover effect on milk yield induced by a 1× milking period (Grala et al., Reference Grala, Phyn, Kay, Rius, Littlejohn, Snell and Roche2011) or a 3× milking period (Wall et al., Reference Wall, Bond and McFadden2013) were related to a persistent effect on gene expression in the mammary tissue. Grala et al. (Reference Dessauge, Lollivier, Ponchon, Bruckmaier, Finot, Wiart, Cutullic, Disenhaus, Barbey and Boutinaud2011) reported persistent reductions in CSN1S1, LALBA, FASN, ACACA mRNA levels after 1×, whereas Wall et al. (Reference Wall, Bond and McFadden2013) observed a persistent modification of genes related to IGF-I signaling pathway after 3×. In goats, the absence of carryover effect when goats were switched back to 2× milking after the application of 4 weeks of 1× milking was associated with a recovery of LALBA gene expression (Ben Chedly et al., Reference Ben Chedly, Lacasse, Marnet and Boutinaud2013). These results suggest that carryover effects on milk production can be due to a persistent regulation of transcription. The carryover effect of a period of 1× milking has been related to an increase in DNA methylation in the distal region of the CSN1S1 gene (Nguyen et al., Reference Nguyen, Boutinaud, Pétridou, Gabory, Pannetier, Chat, Bouet, Jouneau, Jaffrezic, Laloë, Klopp, Brun, Kress, Jammes, Charlier and Devinoy2014). The persistent effect on gene expression in the mammary tissue would be the consequence of an imprinting process through epigenetic modifications. However, further studies are necessary to confirm the involvement of epigenetic modifications in persistent effects on milk yield.
Potential mechanisms underlying the resilience of the mammary tissue after modification of feed supply
The long-term effect of feed restriction on milk yield has been well documented. No carryover effect on milk yield has been observed regardless of the duration of the dietary challenge (from 4 days to 4 weeks; Friggens et al., Reference Friggens, Emmans, Kyriazakis, Oldham and Lewis1998; Bjerre-Harpøth et al., Reference Bjerre-Harpøth, Friggens, Thorup, Larsen, Damgaard, Ingvartsen and Moyes2012) and the stage of lactation (early, mid- or late lactation; Bjerre-Harpøth et al., Reference Bjerre-Harpøth, Friggens, Thorup, Larsen, Damgaard, Ingvartsen and Moyes2012). Accordingly, a recent study showed no carryover effect of a 20% feed restriction of 4 weeks (Herve et al., Reference Herve, Quesnel, Veron, Portanguen, Gross, Bruckmaier and Boutinaud2018). This lack of persistent effect on milk yield was observed despite the negative effect of feed restriction on the number of MEC in the mammary tissue (as indicated by a higher rate of MEC exfoliation into milk; Herve et al., Reference Herve, Quesnel, Veron, Portanguen, Gross, Bruckmaier and Boutinaud2018). It is noteworthy that this was accompanied by an increase in the release of prolactin into blood at milking when cows were switched back to an ad libitum diet. Since prolactin is known to have galactopoietic effects, its enhanced release during the period following feed restriction may have counteracted the negative effect of the challenge on mammary cell number. This suggests that the mammary tissue, after a period of adaptation during the dietary challenge, enters a new process of adaptation when the animal is switched back to an ad libitum diet.
Some factors responsible for individual variability in the adaptation of the mammary gland to challenges
The study of individual response in the adaptation of the mammary tissue to reduced milking frequency such as during a 24-h milking interval revealed relationships between carryover effects on milk yield and some traits of the animal. Even if on average no carryover effect was observed after 1 day of 1× milking, the recovery of milk yield when 2× milking was resumed differed among cows. Cows were clustered according to their ability to recover milk yield after a 24-h milking interval. Cows that recovered poorly (on average 63.9% of the milk they had lost) exhibited a lower estimated milk yield potential or an initially inflamed udder (as indicated by a higher occurrence of initial milk interleukin-8; Charton et al., Reference Charton, Larroque, Robert-Granié, Pomiès, Leclerc, Friggens and Guinard-Flament2016). Therefore, several traits related to the physiological status of the mammary tissue (inflammation status, genetic parameters and stage of lactation) modulate the adaptation of the mammary tissue to a challenge and could result in carryover effects on milk yield. Thus, probably also for other challenges, the physiological status of the mammary tissue has to be taken into account to understand the mammary tissue plasticity.
Conclusion
The cellular mechanisms underlying mammary tissue plasticity during lactation may involve different cellular processes, such as modifications of secretory activity, epigenetic marks and mammary cell number. The processes will be different depending on the nature of the challenge (type, intensity and duration) and on animal-related factors (e.g., health, genetic background and stage of lactation). Depending on these conditions, the mammary tissue shows elastic properties, that is, non-permanent deformation or undergoes some irreversible modifications of milk production. The non-permanent deformation is observed with dietary challenges, for instance. The irreversible modification can either be beneficial (e.g., after an increase in milking frequency during early lactation) or deleterious (e.g., after a reduction in milking frequency for a long period). However, the lack of suitable techniques for dynamic and complete analysis of the phenomena of adaptation has certainly limited a holistic and integral view of the physio-cellular adaptations in bovine mammary tissue. When considered alone, the cellular mechanisms underlying mammary tissue plasticity during challenges are not sufficient to understand the carryover effects on milk production. Epigenetic modifications may be an exception, since they could explain the residual effects of the modifications induced by changes in milking frequency. Special attention should thus be paid to epigenetic modifications because little is known about the persistency of these marks during lactation. Further investigations on the role of stem cells in mammary tissue plasticity need to be performed. Better knowledge of the mechanisms involved in that phenomenon will help us understand more about the high plasticity of mammary tissue, and especially the one that is observed during early lactation. The impact of that mammary tissue plasticity on animal productivity, longevity and robustness should also be addressed in the future.
Acknowledgments
The authors are very grateful to Pierre-Guy Marnet (Agrocampus Ouest, Rennes, France); Hedi Ben Chedly (AgroSup Dijon, Dijon, France); Eve Devinoy, Minh Nguyen, Laurent Galio, Clémence Kress, Denis Laloë, and Florence Jaffrezic (INRA, Jouy-en-Josas, France); and Kuljeet Singh (AgResearch, Hamilton, New Zealand) for their collaboration. The authors are grateful to Mary Varcoe, from the Translation Bureau, Public Services and Procurement Canada, for her careful editing of this manuscript. This review is based on a presentation at the International Workshop on the Biology of Lactation in Farm Animals (BOLFA), 25- 26 August 2018 preceding the 69th Annual Meeting of the European Federation of Animal Science (EAAP), Dubrovnik, Croatie. The abstract of this communication has been deposited in Prodinra https://prodinra.inra.fr/record/454711.
Declaration of interest
The authors declare that there is no conflict of interest for this manuscript.
Ethics statement
None.
Software and data repository resources
None of the data were deposited in an official repository.
Supplementary material
To view supplementary material for this article, please visit https://doi.org/10.1017/S1751731119000624