Anxiety disorders are a prevalent form of mental illness Reference Kessler, Chiu, Demler, Merikangas and Walters1 with a substantial burden of suffering. Reference Mendlowicz and Stein2 Prior research has often compared brain function in one anxiety diagnosis to that of a non-anxious comparison group to isolate functional differences relating to that disorder, producing convergent, although not always consistent, evidence of abnormal function of limbic structures such as the amygdala and insula to emotional stimuli. Reference Beutel, Stark, Pan, Silbersweig and Dietrich3–Reference Hoehn-Saric, Schlund and Wong6 Such comparisons have been useful in identifying neural systems that are dysfunctional in specific anxious diagnoses, but a different approach is needed for delineating functional differences common across anxiety disorders or unique to a specific type of anxiety. Given the comorbidity among anxiety diagnoses Reference Kessler, Chiu, Demler, Merikangas and Walters1 and the lack of disorder-specific developmental predictive validity, Reference Malcarne, Hansdottir, Merz, Ingram and Price7 it may be more useful to conceptualise specific diagnoses as reflecting a common core affective neural dysfunction augmented by higher-order functional phenotypes that orient individuals to a specific focus of anxiety.
This conceptualisation is consistent with the focus of the Research Domain Criteria project (RDoC; http://nimh.nih.gov/research-priorities/rdoc/index.shtml), the manifestation of a strategic plan recently instituted by the National Institute of Mental Health to classify psychopathology based upon dimensions of observable behaviour and neurobiological measures. Under this rubric, the classification of mental disorders will shift away from a basis on constellations of frequently overlapping symptom clusters towards a focus on dysfunction of underlying dimensions of behaviour and corresponding neurobiological systems that may span multiple diagnoses as currently defined by the DSM-5. 8 Existing evidence suggests the current diagnostic classification of anxiety disorders is primarily characterised by a dysfunctional negative valence system, one of the current domains defined in the RDoC project. This domain is further subdivided into several constructs, of which the most applicable to ‘anxiety disorders’ include acute threat (i.e. fear), potential harm (i.e. anxiety) and sustained threat. However, the degree to which dysfunction of this negative valence system and its underlying neural substrates may vary in predominance across different diagnoses of anxiety disorders is still largely unexplored. This variability can initially be investigated through comparing brain function among people with different principal anxiety disorders and non-anxious participants. The DSM diagnoses of generalised anxiety disorder (GAD), panic disorder and social anxiety disorder (SAD) are prime candidates for investigation since they are highly prevalent and often comorbid, Reference Kessler, Chiu, Demler, Merikangas and Walters1 do not have an aetiology stemming from a specific antecedent event (for example as in post-traumatic stress disorder (PTSD)), display widely generalised fear/anxiety responses (unlike specific phobia), are characterised by hyperactivity of lower-order limbic substrates Reference Beutel, Stark, Pan, Silbersweig and Dietrich3,Reference Shah, Klumpp, Angstadt, Nathan and Phan4,Reference Nitschke, Sarinopoulos, Oathes, Johnstone, Whalen and Davidson9 (unlike obsessive-compulsive disorder (OCD)), Reference Alptekin, Akdede, Akvardar, Erol and Columbus10 but each is distinguished by a different focus of fear/anxiety and a different cardinal symptom manifestation. In particular, OCD and PTSD have been reclassified in DSM-5 out of anxiety disorders and into their own respective categories of obsessive-compulsive and related disorders and trauma and stressor-related disorders as a result of the increasing recognition of these disorders as being distinct from more ‘pure’ manifestations of anxiety. Individuals with OCD are known to display a unique neural dysfunction of cortico-thalamo-striatal circuits that are thought to underlie the obsessive thoughts and repetitive behaviours characteristic of this syndrome, Reference Alptekin, Akdede, Akvardar, Erol and Columbus10 which is relatively distinct from the limbic dysfunction thought to underlie SAD, panic disorder and GAD. PTSD is also conceptually distinct from the anxiety disorders in that its aetiology is tied to the experience of one or more discrete traumatic events, and its diagnostic criteria in the DSM-5 specifically incorporate symptoms that are relatively distinct from those associated with anxiety, such as dissociation, emotional numbing, restricted range of affect, reckless/self-destructive behaviour and memory difficulties. Although the limbic dysfunction seen in PTSD may overlap with that of the anxiety disorders of GAD, SAD and panic disorder, its functional neuroanatomy may also be distinguished from these disorders by hypoactivation of medial prefrontal regions. Reference Etkin and Wager5 Taken together, the exploration of common and distinct neural phenotypes within GAD, panic disorder and SAD may ultimately be useful in understanding those neural systems and their corresponding research domain criteria that are implicated in a specific psychopathological manifestation of anxiety. Such studies will ultimately be useful in bridging the gap between the current symptom cluster-based classification of mental illness (as exemplified by the DSM-5) and a behavioural dimension-based system rooted in neurobiology and behavioural science.
The purpose of this study is to delineate common and distinct abnormalities among participants with GAD, panic disorder, SAD and a healthy control group during the processing of emotional stimuli. As in a large portion of affective imaging studies, we utilised emotional facial expressions to probe the neural substrates underlying emotional processing. From the theoretical perspective defined by the RDoC project, we expected all of these individuals with anxiety diagnoses to display dysfunction of the neurobiological substrates implicated in negative valence systems, the domain we conceptualise as a core underlying characteristic of anxiety and fear. More specifically, and consistent with prior findings, Reference Etkin and Wager5,Reference Hoehn-Saric, Schlund and Wong6,Reference Nitschke, Sarinopoulos, Oathes, Johnstone, Whalen and Davidson9 we expected all anxiety groups (v. controls) to display increased activity of certain affective brain structures (such as the amygdala) during targeted processing of emotional expressions. We conceptualised different anxiety disorder diagnoses as reflecting a core dysfunction of the negative valence system domain overlaid by dysfunction of other RDoC domains that result in a particular focus or manifestation of anxiety or fear. More specifically, we expected GAD to be characterised by additional dysfunction in the cognitive systems domain, SAD to be characterised by additional dysfunction in the systems for social processes domain and panic disorder to be characterised by additional dysfunction in the arousal/regulatory systems domain. From this theoretical perspective we developed hypotheses to focus on specific neuroanatomical substrates implicated in prior imaging studies. Consistent with the potential role of the insula in panic attacks, Reference Dresler, Hahn, Plichta, Ernst, Tupak and Ehlis11 the role of the dorsal anterior cingulate in worry, Reference Paulesu, Sambugaro, Torti, Danelli, Ferri and Scialfa12 and the role of the posterior superior temporal sulcus in social information processing/evaluation and dysfunction in that region in SAD, Reference Gentili, Gobbini, Ricciardi, Vanello, Pietrini and Haxby13 we expected the panic disorder, GAD and SAD groups to display diagnosis-specific abnormalities in these respective regions.
Table 1 Demographic characteristics by diagnostic group

GAD group
(n = 15) |
Panic disorder group
(n = 15) |
SAD group
(n = 14) |
Control group
(n = 15) |
F/χ2 | P | Pair-wise comparisons Footnote a |
|
---|---|---|---|---|---|---|---|
Age, years: mean (s.d.) | 33.93 (10.55) | 27.00 (7.00) | 25.43 (8.55) | 30.00 (10.21) | 2.430 | 0.075 | GAD > SAD |
Years of education, mean (s.d.) | 15.87 (2.20) | 14.87 (1.60) | 14.24 (1.97) | 16.00 (1.96) | 5.057 | 0.004 | GAD, control > SAD |
Gender, n | 1.475 | 0.688 | N/A | ||||
Women | 12 | 10 | 10 | 9 | |||
Men | 3 | 5 | 4 | 6 | |||
Ethnicity, n | 4.755 | 0.191 | N/A | ||||
African-American | 0 | 0 | 1 | 0 | |||
Asian | 3 | 1 | 4 | 3 | |||
White | 11 | 9 | 5 | 9 | |||
Latin-American | 0 | 0 | 2 | 1 | |||
Native American | 0 | 1 | 0 | 0 | |||
Other | 1 | 4 | 2 | 2 | |||
STAI, total score: mean (s.d.) | 53.20 (8.47) | 42.80 (6.07) | 49.64 (6.89) | 29.90 (5.04) | 27.12 | <0.001 | GAD, panic disorder, SAD > control; GAD > panic disorder |
GAD, generalised anxiety disorder; SAD, social anxiety disorder; n/a, not applicable; STAI, Spielberger State–Trait Anxiety Inventory.
a. Pair-wise comparisons lists significant differences among groups after correction for multiple comparisons (P < 0.05).
Method
Participants
Following approval of the University of California-San Diego Institutional Review Board, 59 adults were recruited through local advertisement and clinical referrals to participate after providing informed consent. Participants with GAD (n = 15), panic disorder (n = 15) and SAD (n = 14) were all treatment-seeking and recruited to participate in intervention studies. Healthy comparison participants (control group, n = 15) were recruited to undergo functional magnetic resonance imaging (fMRI) (Table 1). Experienced PhD and Master’s-level clinicians established DSM-IV psychiatric diagnoses 14 using the structured diagnostic Mini International Neuropsychiatric Interview. Reference Sheehan, Lecrubier, Sheehan, Amorim, Janavs and Weiller15 Psychiatric exclusion criteria included lifetime diagnosis of psychotic disorder, organic mental disorder, intellectual disability, bipolar I disorder, substance dependence in the year and substance misuse in the past month. For the control group additional exclusionary criteria were lifetime diagnosis of mood/anxiety disorders, eating disorders or substance dependence. All participants were required to be psychotropic medication-free for a minimum of 6 weeks prior to participation (2 weeks for benzodiazepines). Patients undergoing current psychotherapy for anxiety and/or mood symptoms were excluded. Participants with anxiety who met current diagnostic criteria for more than one anxiety disorder and/or comorbid major depression were not included in the current investigation. Past history of major depression was permitted, which amounted to 6 participants in each diagnostic group. Thus, within each patient group each participant met current diagnostic criteria only for the index anxiety disorder.
Task
Participants completed a modified version of the Emotion Face Assessment Task. Reference Paulus, Feinstein, Castillo, Simmons and Stein16 For each 5 s trial, participants were presented with a target face on the top of the screen and instructed to match the facial expression to one of two faces presented below through a button box. Blocks consisted of six consecutive trials wherein the target face was angry, happy or fearful. A sensorimotor control condition, in which a target shape was presented and participants were told to pick the matching shape, was also presented in similar format. Each target condition was presented in three blocks of six trials each in pseudorandomised order, with an 8 s fixation cross presented between each block and at the beginning and end of the task.
fMRI data acquisition
Data were collected using fMRI image parameters sensitive to BOLD contrast on a 3.0T GE Signa EXCITE (GE Healthcare, Milwaukee, Wisconsin) scanner (T2*-weighted echo planar imaging, repetition time (TR) = 2000 ms, echo time (TE) = 32 ms, field of view (FOV) = 250 × 250 mm, 64 × 64 matrix, 30 2.6 mm axial slices with 1.4 mm gap, 256 repetitions). A high-resolution T1-weighted image (172 sagittally acquired spoiled gradient recalled 1 mm thick slices, inversion time (TI) = 450 ms, TR = 8 ms, TE = 4 ms, flip angle 12°, FOV = 250 × 250 mm) was also collected from each participant. Images were corrected for non-simultaneous slice acquisition in each volume.
Preprocessing/individual analysis
Data were processed using AFNI version AFNI_2011_12_21_1014 for Linux. Reference Cox17 Voxel time-series data were coregistered to an intra-run volume, then to the anatomical of each participant and corrected for artefact intensity spikes. Those time points with greater than two standard deviations more voxel outliers than the participant’s mean were excluded from analysis. Rotational parameters (roll, pitch and yaw) were used as nuisance regressors for motion artefact. Time-series data were normalised to Talairach coordinates, Reference Lancaster, Woldorff, Parsons, Liotti, Freitas and Rainey18 and a Gaussian smoothing filter with a full-width at half maximum (FWHM) of 4 mm was applied to each participant’s time series. A deconvolution analysis was conducted in which the orthogonal regressors of interest were target trials of: (a) happy faces; (b) angry faces; (c) fearful faces; and (d) shapes. The outcome measures of interest were activation magnitudes for the within-subject contrasts of each target emotion type v. the shape-matching baseline condition. Regressors of interest were convolved with the haemodynamic response. Baseline and linear drift variables were entered into the regression model. The average voxelwise response magnitude was estimated using AFNI’s 3dDeconvolve program. Beta coefficients for each regressor were normalised to voxelwise % signal changes (%SCs) before being carried to second-level analysis.
Group-level analysis
One-sample t-tests were used to identify task-dependent activations. To frame task-dependent brain function in the context of an RDoC construct, we used robust regression (while controlling for age and years of education) to examine the continuous relationship of voxelwise emotional valence-related differential brain activation (i.e. fear v. happy and angry v. happy) across participants with a self-reported measure of trait anxiety from the Spielberger State–Trait Anxiety Inventory (STAI). Reference Spielberger19 We chose this measure because of its conceptual and empirical relationship with negative affectivity, Reference Ball, Sullivan, Flagan, Hitchcock, Simmons and Paulus20 a construct closely related to the negative valence systems domain of RDoC. To identify diagnostic differences, voxelwise %SCs were subjected to a linear mixed-effects analysis conducted within R version 2.13.0 for Windows. 21 Group and emotion type (i.e. angry v. oval, fear v. oval, etc.) were entered as fixed factors in conjunction with a random intercept resulting in a 4 (group) × 3 (emotion) factorial design. Age and years of education were also entered into the model as covariates of no interest. The outcome measures of interest were the omnibus F-values for group (i.e. emotion-processing group differences across all emotional face types) and the group emotion interaction effects for two contrast vectors specifying the differences between: (a) fear v. oval and happy v. oval; and (b) angry v. oval and happy v. oval (i.e. processing negatively v. positively valenced emotional faces). This statistic allows for the detection of significant differences between two or more of the four groups on a voxelwise level, and post hoc pair-wise comparisons were used to identify which groups differed from one another. We explored the contrast of processing negatively v. positively valenced emotion types in addition to general emotion-processing effects because: (a) these contrasts provide greater specificity of content-related emotion processing differences by controlling for face-processing and emotion non-specific effects; and (b) they are most comparable with prior studies that have used happy or neutral faces as comparator conditions. Such valenced emotion contrasts have proven useful in prior studies in eliciting group differences in anxiety-relevant brain structures. Reference Fonzo, Simmons, Thorp, Norman, Paulus and Stein22
Task-dependent activity was defined using single-sample t-tests against the null hypothesis for examining general emotion processing (all emotional face types v. ovals) as well as differential emotion effects for valence-related contrasts (fear v. happy and angry v. happy). Effects were thresholded on a voxelwise level at P<0.05 in the group-difference regions of interest (ROI) analyses, and at more rigorous voxelwise thresholds of P<0.01 in the whole-brain group-difference analysis and P<0.001 in task-effect analyses to increase spatial specificity of clustered effects. In addition to a whole-brain exploratory analysis, a priori hypotheses were tested through ROI analyses conducted on emotional- and social-processing brain regions implicated by our hypotheses: bilateral insula, bilateral amygdala, anterior cingulate and the bilateral posterior superior temporal cortex. ROI masks were based upon both anatomical criteria and standardised locations taken from the Talairach atlas Reference Talairach and Tournoux23 (see online supplement DS1 for further details).
A threshold adjustment based upon Monte-Carlo simulations was used to correct for multiple comparisons. A priori voxelwise probability of P<0.05 with a 4 mm search radius and cluster size of 256 μL for the amygdala, 448 μL for the insula and anterior cingulate, and 576 μL for the posterior superior temporal ROI maintained the a posteriori probability at P<0.007 in each limbic ROI (P<0.05 across all ROIs). A cluster volume of 704 μL for the whole-brain analysis resulted in a posteriori probability of P<0.05. The corrected voxelwise probabilities for each region are: amygdala (P = 0.0008); insula (P = 0.0001); anterior cingulate (P = 0.0001); posterior superior temporal sulcus (P = 0.00006) and whole brain (P = 0.000013). Average %SCs were extracted from significant group-effect clusters and subjected to further analysis/visualisation in IBM SPSS 19.0 for Windows. Post hoc pair-wise comparisons were used to examine patterns of group differences, and a Bonferroni-corrected P<0.05 was used as the criterion of significance.
Results
Task-dependent behaviour and activation
A multivariate ANOVA revealed no significant differences among groups in average task reaction time or accuracy (all Ps>0.05). In general, the task activated the expected limbic regions (see online Tables DS2–4 for details). General emotion processing (all faces v. ovals) resulted in activation of the bilateral amygdala and insula. Processing negative emotional valence (matching to fear v. happy and matching to angry v. happy) activated the bilateral anterior insula and deactivated the perigenual anterior cingulate.
Relationship of brain activation to negative affectivity
Trait anxiety measures were available for 44 of the 59 participants that underwent fMRI (10 in the GAD group, 12 in the panic disorder group, 10 in the control group and 12 in the SAD group). All participants with anxiety disorders reported higher levels of trait anxiety relative to the control group, with the GAD group also reporting higher levels relative to the panic disorder group, but not the SAD group. The panic disorder and SAD groups did not differ from one another. (F = 27.12, P<0.001; GAD, panic disorder, SAD groups>control group; GAD group>panic disorder group; all pair-wise comparisons significant after Bonferroni correction; Table 1).
Valence-related differential activation (fear v. happy)
ROI. There was a significant positive relationship between trait anxiety and the contrast of activation to fear v. happy faces in the right amygdala across all participants (Fig. 1). Post hoc extractions revealed this effect was not driven by the fear (Spearman’s ρ = 0.23, P = 0.14) or the happy (Spearman’s ρ = –0.08, P = 0.60) conditions alone, but the contrast of the two (Spearman’s ρ = –0.41, P = 0.006). There were no other significant relationships with activation in other ROI.
Exploratory whole-brain analyses. Exploratory analyses revealed additional positive relationships between trait anxiety and brain activation in the bilateral dorsolateral prefrontal cortex and the right hippocampus/parahippocampal gyrus (Table 2). Post hoc extractions revealed these effects were the result of relationships present during the fear-processing condition (right prefrontal cortex: Spearman’s r = 0.35, P = 0.02; left prefrontal cortex: Spearman’s r = 0.31, P = 0.04; right hippocampus: Spearman’s r = 0.30, P = 0.05).

Fig. 1 Common increased right amygdala activation in generalised anxiety disorder (GAD), panic disorder (PD) and social anxiety disorder (SAD) and relationship to negative affectivity.
(a) and (b) differential activation for the interaction of group with the linear contrast of fear v. ovals against happy v. ovals. Error bars represent +/- 1 standard error. (c) and (d) continuous relationship between amygdala activation to fear v. happy and trait anxiety. Spearman’s ρ = 0.409, P = 0.006. HC, health control; au, arbitrary units.
Valence-related differential activation (angry v. happy)
ROI. There was a positive relationship between trait anxiety and activation in the perigenual anterior cingulate during the contrast of angry v. happy faces. Post hoc extractions revealed this effect was because of a negative relationship between trait anxiety and activation to happy faces (Spearman’s ρ = –0.32, P = 0.04). There was also a positive relationship between trait anxiety and activation in the left posterior superior temporal cortex. Post hoc extractions revealed this effect was not arising from the angry (Spearman’s ρ = 0.25, P = 0.10) or happy (Spearman’s ρ = 0.10, P = 0.51) condition alone, but from the contrast of the two (Spearman’s ρ = 0.44, P = 0.003).
Exploratory whole-brain analyses. Exploratory analyses revealed additional positive relationships between trait anxiety and brain activation in the left supplementary motor area and the left postcentral gyrus (Table 2). The effect in the left supplementary motor area arose from neither condition alone (angry v. oval: Spearman’s ρ = 0.25, P = 0.10; happy v. oval: Spearman’s ρ = –0.10, P = 0.50), but the contrast of the two (Spearman’s ρ = 0.50, P = 0.001). The effect in the postcentral gyrus arose only from the angry face condition (Spearman’s ρ = 0.38, P = 0.01).
Anxiety-disorder-related activation differences
General emotion processing (faces v. ovals)
ROI. Across all emotional face types, the panic disorder group displayed greater activation of the right posterior insula (panic disorder group>GAD, control, SAD groups; all pair-wise
Table 2 Relationships between trait anxiety and emotional valence-related brain activation Footnote a

Contrast
and mask |
Hemisphere | Region | Volume, μL |
x | y | z | Voxelwise statistics, mean (s.d.) | Extracted correlation | ||
---|---|---|---|---|---|---|---|---|---|---|
t | P | Spearman’s ρ | P | |||||||
Fear v. happy | ||||||||||
ROI | Right | Amygdala | 256 | 20 | –7 | –13 | 2.67 (0.44) | 0.016 (0.018) | 0.41 | 0.006 |
Whole brain | Right | Middle frontal gyrus (dorsolateral) | 1088 | 30 | 16 | 23 | 2.46 (0.42) | 0.024 (0.015) | 0.54 | < 0.001 |
Whole brain | Right | Hippocampus/parahippocampal
gyrus |
768 | 36 | –23 | –8 | 2.45 (0.24) | 0.021 (0.011) | 0.49 | 0.001 |
Whole brain | Left | Superior frontal gyrus/middle
frontal gyrus |
704 | –20 | 15 | 39 | 2.57 (0.42) | 0.019 (0.015) | 0.45 | 0.002 |
Angry v. happy | ||||||||||
ROI | Left | Superior temporal gyrus | 1024 | –51 | –18 | 1 | 2.26 (0.68) | 0.033 (0.021) | 0.44 | 0.003 |
ROI | Left/right | Anterior cingulate (perigenual) | 448 | –2 | 39 | 0 | 2.76 (0.68) | 0.019 (0.021) | 0.56 | < 0.001 |
Whole brain | Left | Cingulate gyrus/medial frontal
gyrus (supplementary motor area) |
1600 | –19 | 5 | 42 | 2.58 (0.40) | 0.019 (0.015) | 0.50 | 0.001 |
Whole brain | Left | Postcentral gyrus | 832 | –30 | –26 | 39 | 2.60 (0.34) | 0.017 (0.012) | 0.51 | < 0.001 |
ROI, regions of interest.
a. x, y, and z are the Talairach coordinates for the cluster centre of mass; voxelwise statistics report mean t and P with standard deviations in parentheses; extracted correlation lists non-parametric correlations of trait anxiety scores against extracted cluster values; locational descriptors in parentheses do not denote actual anatomical distinctions but are based upon the relative location of the cluster in standardised space.
comparisons significant; Fig. 2) and the right posterior superior temporal gyrus (panic disorder group>GAD, control, SAD groups; all pair-wise comparisons significant). There were no differences observed in the amygdala or anterior cingulate.
Exploratory whole-brain analyses. Exploratory analyses revealed additional foci of group differences in other brain structures such as the left supramarginal gyrus/inferior parietal lobule (panic disorder group>control, SAD groups; GAD group>control group), right supramarginal gyrus/inferior parietal lobule (panic disorder, GAD groups>control, SAD groups), and left cerebellum (panic disorder group>GAD, control, SAD groups). Differences
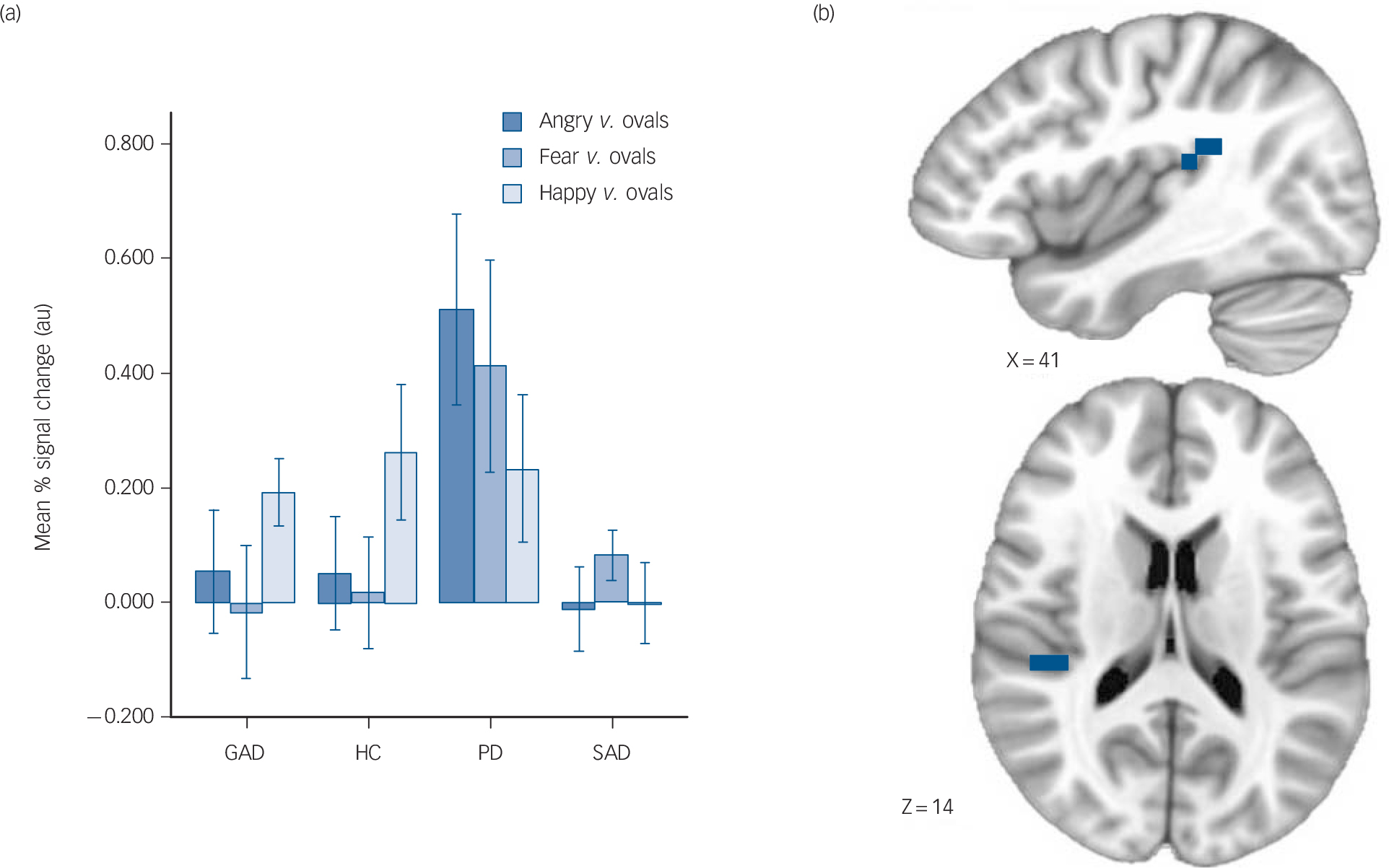
Fig. 2 Increased posterior insula activation in panic disorder.
(a) Mean % signal change in generalised anxiety disorder (GAD), control (HC), panic disorder (PD) and social anxiety disorder (SAD) groups (error bars represent ± 1 standard error), (b) brain images depict differential activation for the main effect of group collapsed across face types.
were also observed in the left precentral gyrus/supplementary motor area (panic disorder group>GAD, control, SAD groups), left dorsolateral prefrontal cortex (GAD, control, SAD groups>panic disorder group), and left precuneus/inferior parietal lobule (panic disorder, GAD groups>control, SAD groups) (Table 3).
Valence-related differential activation (fear v. happy)
ROI. Analyses aimed at isolating emotional valence-related differences between processing of fear and happy revealed interactions with diagnostic group in the right posterior insula. In this region the panic disorder group displayed greater positive differential activation between the fear and happy conditions relative to the GAD and control groups, but not the SAD group; the SAD group did not significantly differ from any other group. Additionally, in the right amygdala participants from all anxiety groups (GAD, panic disorder and SAD) displayed greater positive (or less negative) differential activation between processing fear and happy conditions relative to the control group (Fig. 1). That is, all the following pair-wise comparisons for activation in this region were significant: GAD group>control, panic disorder groups>control group, and SAD group>control group.
There are two notable aspects to this finding. First, this effect reflects greater preferential activation of the right amygdala to happy faces relative to fearful faces in the control group, whereas in the anxiety groups amygdala activation to both face types was nearly equivalent. Second, differences in magnitude of activation for each face type (fearful and happy) v. the sensorimotor control condition between the anxiety groups and the control group were most pronounced in the fearful face condition, reflecting an enhanced amygdalar sensitivity to fear cues in participants with anxiety. The panic disorder group also displayed greater positive differential activation between processing fear and happy in the right posterior superior temporal gyrus relative to the SAD and control groups; differences between these conditions for the GAD group did not differ from those of any other group. However, in the left posterior middle/superior temporal gyrus the panic disorder and SAD groups both displayed greater positive differential activation between processing fear and happy relative to the control group; the GAD group did not display differential activation that differed from any other group.
Exploratory whole-brain analyses. Exploratory analyses revealed an additional effect in the bilateral medial cerebellum in which all the anxiety groups displayed greater activation compared with the control group (Table 4).
Valence-related differential activation (angry v. happy)
Region of interest analyses. Analyses aimed at isolating emotional valence-related differences between processing angry and happy revealed interactions with diagnostic group in the right posterior insula. In this region, participants with panic disorder displayed greater positive differential activation between processing angry and happy relative to all three other groups. Additionally, in the right posterior middle/superior temporal gyrus the panic disorder group displayed greater positive differential activation between processing angry and happy relative to all three other groups. There were no group differences observed in the amygdala or anterior cingulate.
Exploratory whole-brain analyses. Exploratory analyses revealed additional foci of group differences in other brain structures such as the left postcentral gyrus (panic disorder, SAD groups>GAD, control groups) and the left supplementary motor area (panic disorder group>GAD, control, SAD groups) (Table 5).
Discussion
To our knowledge, this is the first study to differentiate functional abnormalities that are common across diagnostic categories from those that are unique to a specific diagnosis in individuals with GAD, panic disorder and SAD. Across patients with anxiety disorders compared with the control group, there was an increased activation in the amygdala to the processing of fearful faces relative to happy faces, and greater activation in the right amygdala was related to greater levels of negative affectivity (i.e. trait anxiety). Specific to the panic disorder diagnosis, there was a unique hyperactivation of the posterior insula collapsed across all emotional face types as well as in analyses isolating differential activation between processing of angry and happy faces. The results are consistent with the view that all three anxiety disorders share a pattern of abnormal amygdalar processing of facial fear cues, whereas panic disorder is characterised by a unique dysfunction of core interoceptive neural substrates. Taken together, functional brain imaging supports a dual-process model of anxiety composed of a general dysfunction of bottom-up stimulus processing and a specific contribution of context-relevant processes supported by distinct neural substrates.
Common amygdala dysfunction in GAD, panic disorder and SAD
The finding for amygdalar differences common to all disorders is consistent with prior studies demonstrating amygdalar functional abnormalities in clinical anxiety manifestations relative to healthy controls across varying paradigms and samples. Reference Beutel, Stark, Pan, Silbersweig and Dietrich3,Reference Nitschke, Sarinopoulos, Oathes, Johnstone, Whalen and Davidson9,Reference Stein, Goldin, Sareen, Zorrilla and Brown24 However, to our knowledge this study provides initial evidence that the amygdala displays uniform abnormalities across several manifestations of clinical anxiety within the same emotion-processing paradigm. There are two aspects to this finding deserving of discussion. First, participants in the anxiety groups displayed more equivalent amygdala activation to processing happy faces and fearful faces compared with the control group, who displayed proportionately greater amygdala activation to processing happy relative to fear. Second, the magnitude of activation for each emotion condition v. the sensorimotor baseline revealed greater amygdala activation to fearful faces in all anxiety groups, whereas amygdala activation to happy faces was roughly equivalent between the anxiety groups and the control group. Thus, those in the anxiety groups displayed both a greater amygdalar responsivity to fear cues and also failed to show the ostensibly normative amygdalar activation preference to positive facial stimuli. This suggests a dual abnormality wherein amygdala activation to negative valence processing is heightened across the anxiety diagnoses investigated here, and this is overlaid upon a lack of preferential amygdala responsivity to positive valence processing seen in the control group.
This amygdalar activation preference to happy faces in the control group was an unexpected finding, although examination of the contrast task-effect within the control group alone confirmed greater amygdala activation to processing happy relative to fear. Although the amygdala is generally thought to be most responsive to fearful faces, evidence indicates it also responds to happy facial expressions and expressions of humour. Reference Costafreda, Brammer, David and Fu25 A recent meta-analysis found the amygdala responds more strongly to positive than negative emotional stimuli in healthy participants (particularly happy compared with fearful faces), Reference Sergerie, Chochol and Armony26 consistent with the current results but inconsistent with other meta-analyses. Reference Costafreda, Brammer, David and Fu25 Although the amygdala plays a crucial role in the induction of fear responses, Reference Feinstein, Adolphs, Damasio and Tranel27 findings for its responsivity to both positive and negative stimuli have prompted a reconceptualisation of the role of the amygdala away from negative affectivity alone towards more general saliency detection. Reference Costafreda, Brammer, David and Fu25 There are several potential complementary explanations for the counterintuitive finding of greater preferential amygdala activation to happy face-matching trials in healthy controls and the lack of such a finding in participants with anxiety disorders. First, in the current paradigm happy faces may serve as a more salient socioemotional cue to non-anxious participants, consistent with experimental findings for an attentional bias toward mood-congruent facial stimuli. Reference Becker and Leinenger28 Second, the incidental processing of the angry/fearful emotional distractor on emotion matching trials with happy facial expressions may
Table 3 Anxiety-related functional abnormalities for processing all faces v. shapes

Mask | Hemisphere | Region | Volume, μL | x | y | z | Voxelwise statistics, mean (s.d.) | Group, estimated marginal means | ||||
---|---|---|---|---|---|---|---|---|---|---|---|---|
F | P | GAD | Control | Panic disorder | SAD | |||||||
ROI | Right | Insula (posterior) | 448 | 42 | –29 | 16 | 3.74 (0.65) | 0.020 (0.016) | 0.08 | 0.11 | 0.39 | 0.03 |
ROI | Right | Superior temporal gyrus | 2240 | 49 | –35 | 14 | 3.86 (0.92) | 0.020 (0.014) | 0.10 | 0.07 | 0.34 | –0.03 |
Whole brain | Left | Supramarginal gyrus/inferior parietal lobule | 2240 | –49 | –42 | 36 | 5.39 (1.10) | 0.004 (0.003) | 0.01 | –0.16 | 0.11 | –0.08 |
Whole brain | Right | Inferior parietal lobule/superior
parietal lobule/ supramarginal gyrus |
2176 | 36 | –51 | 47 | 5.21 (0.91) | 0.004 (0.003) | 0.07 | –0.12 | 0.15 | –0.16 |
Whole brain | Left | Precentral gyrus/middle frontal
gyrus (supplementary motor area) |
1152 | –19 | –14 | 57 | 5.37 (1.00) | 0.004 (0.003) | –0.01 | –0.06 | 0.10 | 0.03 |
Whole brain | Left | Inferior semi-lunar lobule | 832 | –35 | –67 | –35 | 5.59 (1.48) | 0.004 (0.003) | 0.04 | 0.22 | 0.85 | 0.16 |
Whole brain | Left | Inferior frontal gyrus (dorsolateral) | 832 | –37 | 25 | 16 | 5.65 (1.03) | 0.003 (0.003) | 0.11 | 0.02 | –0.17 | 0.19 |
Whole brain | Left | Precuneus/inferior parietal lobule | 832 | –34 | –63 | 39 | 5.47 (1.48) | 0.004 (0.003) | 0.20 | –0.04 | 0.21 | –0.01 |
ROI, regions of interest.
a. x, y, and z are the Talairach coordinates for the cluster centre of mass; voxelwise statistics report mean t and P with standard deviations in parentheses; estimated column lists estimated marginal means using extracted cluster values; locational descriptors in parentheses do not denote actual anatomical distinctions but are based upon the relative location of the cluster in standardised space.
Table 4 Anxiety-related functional abnormalities for processing fear v. happy faces

Mask | Hemisphere | Region | Volume, μL | x | y | z | Voxelwise statistics, mean (s.d.) | Group, estimated marginal means (fear–happy) | ||||
---|---|---|---|---|---|---|---|---|---|---|---|---|
F | P | GAD | Control | Panic disorder | SAD | |||||||
ROI | Right | Superior temporal gyrus | 1088 | 53 | –28 | 7 | 3.91 (1.19) | 0.020 (0.015) | –0.01 | –0.09 | 0.25 | –0.09 |
ROI | Left | Middle/superior temporal gyri | 896 | –53 | –36 | 1 | 3.53 (0.57) | 0.021 (0.014) | 0.01 | –0.16 | 0.13 | 0.13 |
ROI | Right | Insula (posterior) | 576 | 43 | –31 | 19 | 3.02 (0.35) | 0.035 (0.012) | 0.01 | –0.16 | 0.11 | –0.08 |
ROI | Right | Amygdala | 256 | 25 | –6 | –12 | 3.45 (0.58) | 0.023 (0.018) | 0.04 | –0.20 | 0.15 | 0.06 |
Whole brain | Left/right | Inferior semi-lunar lobule/cerebellar tonsil | 832 | –2 | –62 | –36 | 4.68 (0.49) | 0.005 (0.002) | 0.11 | –0.98 | 0.16 | 0.18 |
ROI, regions of interest.
a. x, y, and z are the Talairach coordinates for the cluster centre of mass; voxelwise statistics report mean t and P with standard deviations in parentheses; estimated column lists estimated marginal means for fear v. happy using extracted cluster values; locational descriptors in parentheses do not denote actual anatomical distinctions but are based upon the relative location of the cluster in standardised space.
Table 5 Anxiety-related functional abnormalities for processing angry v. happy faces

Mask | Hemisphere | Region | Volume, μL | x | y | z | Voxelwise statistics, mean (s.d.) | Group, estimated marginal means (angry–happy) | ||||
---|---|---|---|---|---|---|---|---|---|---|---|---|
F | P | GAD | Control | Panic disorder | SAD | |||||||
ROI | Right | Middle/superior temporal gyri | 1216 | 56 | –34 | 4 | 3.25 (0.45) | 0.028 (0.014) | –0.03 | –0.06 | 0.31 | 0.11 |
ROI | Right | Insula (posterior) | 448 | 51 | –31 | 20 | 3.00 (0.36) | 0.036 (0.013) | –0.12 | –0.22 | 0.33 | –0.03 |
Whole brain | Left | Postcentral gyrus | 2368 | –36 | –32 | 48 | 5.76 (1.30) | 0.002 (0.002) | –0.07 | –0.11 | 0.18 | 0.03 |
Whole brain | Left | Middle frontal gyrus/precentral gyrus (supplementary motor area) | 960 | –20 | –16 | 60 | 5.74 (1.36) | 0.003 (0.004) | –0.04 | –0.04 | 0.14 | –0.10 |
ROI, regions of interest.
a. x, y, and z are the Talairach coordinates for the cluster centre of mass; voxelwise statistics report mean t and P with standard deviations in parentheses; estimated column lists estimated marginal means for angry v. happy using extracted cluster values; locational descriptors in parentheses do not denote actual anatomical distinctions but are based upon the relative location of the cluster in standardised space.
have resulted in a greater elevation in amygdala reactivity in the control group relative to the anxiety groups, potentially because of differences in depth of level of negative-emotion processing. Meta-analytic evidence indicates that greater attentional processing of emotional stimuli was associated with decreased amygdala activation relative to passive processing. Reference Costafreda, Brammer, David and Fu25 Given the attentional bias displayed by participants with anxiety disorders towards threatening facial expressions, Reference Bradley, Mogg, Falla and Hamilton29 it is possible that enhanced attentional processing may have resulted in diminished amygdala reactivity to the threatening emotional distractor relative to the control participants. Third, preferential amygdala activation to the happy face-matching trials in control participants may have arisen as a consequence of both processes (i.e. greater emotional salience of happy faces and reduced attentional processing of negative emotional distracters). Ultimately, the precise mechanisms underlying this differential activity cannot be disambiguated using this experimental approach and would necessitate other data such as eye-tracking to stimulus cues.
However, when contrasting these emotion conditions separately against the sensorimotor baseline, amygdala activation to fearful faces was greater in all the anxiety groups, whereas activation to happy faces was similar between the anxiety groups and control group. This finding is more consistent with an extensive literature demonstrating amygdalar abnormalities to fear cues in several manifestations of anxiety, Reference Etkin and Wager5 and it suggests aberrant amygdala activation to the processing of fear-conveying threat cues is a common neural phenotype across the anxiety disorders investigated here. Given that the amygdala is implicated both in the research domain criteria of positive and negative valence systems as well as the dual abnormality observed here, these results may suggest an alteration in both domains as characterising diagnoses of anxiety disorders. Future studies may wish to incorporate varying levels of both appetitive and aversive emotional stimuli to disentangle dysfunction in these two domains. It should be noted this finding may not generalise to OCD (characterised by a unique pattern of corticothalamostriatal dysfunction) Reference Alptekin, Akdede, Akvardar, Erol and Columbus10 or PTSD, although findings for the latter diagnosis generally support a similar abnormality. Reference Etkin and Wager5
Unique insular dysfunction in panic disorder
The panic disorder group displayed a unique hyperactivity of the posterior insula across emotional face conditions and also while targeting angry v. happy faces, potentially implicating insula dysfunction as a disorder-distinguishing neural phenotype. The localisation of unique differences to the posterior portion of the insula suggests an abnormality of ‘core interoceptive’ processes underlying the lowest-order representation of internal body states, Reference Craig30 consistent with an increased sensitivity to distressing body sensations in panic disorder. Reference Domschke, Stevens, Pfleiderer and Gerlach31 This suggests a potential abnormal representation of body signals in panic disorder, which is notable given increased insula function is a neural correlate of spontaneous panic attacks. Reference Dresler, Hahn, Plichta, Ernst, Tupak and Ehlis11 Insular functional abnormalities are also consistent with structural studies demonstrating abnormal insular grey matter volumes/densities in participants with panic disorder. Reference Uchida, Del-Ben, Busatto, Duran, Guimaraes, Crippa, Araujo, Santos and Graeff32 Taken together, these findings suggest the exaggerated visceralisation of emotional states during targeted processing of negatively valenced facial emotions may be one manifestation of increased sensitivity to distressing body sensations in panic disorder. Reference Domschke, Stevens, Pfleiderer and Gerlach31 Notably, participants with panic disorder showed increased activation in posterior/inferior parietal lobe and posterior cingulate, which is consistent with prior studies in panic disorder demonstrating abnormal parietal responses to serotonergic modulation Reference Meyer, Swinson, Kennedy, Houle and Brown33 and increased posterior cingulate activation to threat-related words. Reference Maddock, Buonocore, Kile and Garrett34 The posterior cingulate and parietal cortices have both been implicated in processing visceral aversive states such as pain and disgust, Reference Benuzzi, Lui, Duzzi, Nichelli and Porro35,Reference Tölle, Kaufmann, Siessmeier, Lautenbacher, Berthele and Munz36 and the increased activation here for participants with panic disorder further supports the proposition that people with this anxiety disorder respond to threat-conveying facial emotional cues with a relatively greater processing emphasis on the integrity of one’s visceral state. In conjunction with the common anxiety-related abnormality of exaggerated amygdala reactivity, these results support a dual-process model of neural dysfunction in panic disorder characterised by both exteroceptive and interoceptive hypersensitivity to threat-conveying emotional cues, and they further suggest the prominence of neural dysfunction underlying interoceptive processing may serve to distinguish panic disorder from the other anxiety disorders investigated here.
Absence of disorder-specific differences in GAD
Contrary to expectations, there were no unique patterns of GAD-specific abnormalities observed in the dorsal anterior cingulate cortex during general or threat-targeted emotion processing trials. Given the importance of this region in worry Reference Paulesu, Sambugaro, Torti, Danelli, Ferri and Scialfa12 and negative emotional expression, Reference Etkin, Egner and Kalisch37 we hypothesised this region would show disorder-specific abnormalities in GAD as a result of the prominence of worry as the cardinal symptom manifestation of this disorder. Additionally, other studies of emotional conflict adaptation and implicit emotion regulation have demonstrated GAD-related abnormalities in the anterior cingulate and related medial prefrontal cortices, Reference Etkin and Schatzberg38,Reference Etkin, Prater, Hoeft, Menon and Schatzberg39 although these abnormalities were also shared in patients with major depressive disorder. We speculate that the lack of observed differences may be caused by the inability of this task to robustly engage medial prefrontal regions, suggested by the lack of significant task-dependent activation during emotion processing collapsed across emotion conditions as well as that isolating the targeting of threat. In fact, these regions showed significant deactivations during the contrast of these conditions, consistent with midline deactivation observed during task-directed behaviour. Reference Gusnard, Akbudak, Shulman and Raichle40 Evidence suggests the anterior cingulate and medial prefrontal regions are heavily implicated in mental processes necessitating regulation/expression of emotional state and/or adaption to conflicting stimuli, Reference Etkin, Egner and Kalisch37 and therefore the emotional recognition and matching processes elicited by the task design may not be sufficient to readily engage the mental processes subserved by these regions. Future studies in these diagnostic groups that evoke greater medial prefrontal engagement through processes such as emotion regulation or worry induction may prove more useful in highlighting abnormalities in these regions that may be unique or specific to GAD.
Posterior temporal dysfunction in panic disorder and SAD
The SAD and panic disorder groups both showed greater activation of the left posterior superior temporal sulcus, a posterior portion of the temporal lobes, relative to the control group while targeting fear v. happy faces. This converges with a prior finding for increased posterior superior temporal sulcus activation during emotional face processing in SAD, Reference Gentili, Gobbini, Ricciardi, Vanello, Pietrini and Haxby13 interpreted as dysfunctional social-information processing. The posterior superior temporal sulcus is thought to be important for the extraction of social/emotional information communicated by non-identity face characteristics (for example direction of gaze and facial expression). Reference Haxby, Hoffman and Gobbini41 This may suggest panic disorder and SAD share a dysfunctional temporal social-information processing system, a neuroanatomical component of the research domain criteria of systems for social processes. This idea may seem more intuitive for SAD compared with panic disorder, given the importance of social stimuli to aetiological theory of the disorder, but it is important to note this shared dysfunction may reflect a characteristic from earlier stages of development. Notable is the developmental relationship between childhood separation anxiety disorder (characterised by extreme reactivity to separation from an attachment figure) and later development of panic disorder, Reference Malcarne, Hansdottir, Merz, Ingram and Price7 a relationship largely subsumed by biological influences Reference Battaglia, Pesenti-Gritti, Medland, Ogliari, Tambs and Spatola42 and potentially indicative of abnormal processing of social information as an abnormality with heterotypic continuity (i.e. different manifestations across the lifespan). However, we did not assess for the presence of separation anxiety disorder in childhood in our adult anxiety groups and can therefore only speculate about the relationship between posterior superior temporal sulcus abnormalities and interpersonal disorders in those with panic disorder. Also notable is that the panic disorder group demonstrated a greater pervasiveness of posterior temporal hyperactivations relative to any other group, displaying greater activation in all three contrast conditions and even in comparison with the SAD group. The clinical significance of these prominent posterior temporal abnormalities in panic disorder is currently unclear, although future studies in panic disorder and SAD populations may wish to target these regions as an a priori focus of investigation.
Limitations
This study has several limitations. First, the task used here does not directly isolate effects related to the target emotional expression as a result of the presence of a non-congruent face (i.e. the distractor) on each trial. Participants must engage in several mental computations for matching, and group differences may arise because of the assessment of the target/matching face, inhibition of the distractor, or both. Thus, the results of this study are not directly comparable with those presenting single faces. Second, although the combined sample size was substantial, the number of participants within each group was relatively small and may have limited power to detect additional group differences. Furthermore, each task condition was presented in only three independent blocks, which may have rendered the task design underpowered to detect all true group differences. Thus, results should be viewed as preliminary at the present time, i.e. only indicative of the largest effects. Third, the diagnostic samples were not equally matched on age or years of education, although we did statistically control for potential confounds in the analyses. Fourth, study participants were composed primarily of woman. Thus, these results may not necessarily generalise to predominantly male samples.
Implications
In aggregate, this study produced neuroanatomical evidence for a common dysfunction across anxiety disorders in the research domain(s) of negative valence systems, with potential diagnosis-specific dysfunctions in the associated research domains of arousal and regulatory systems (in panic disorder) and systems subserving social processes (shared by panic disorder and SAD). We must emphasise these preliminary results are likely not representative of the entire spectrum of common and distinct abnormalities that define the spectrum of pathological anxiety manifestations. Rather, this study serves to illustrate the utility of multidiagnostic comparisons in delineating abnormalities in research domains that are shared, distinct and ‘semi-distinct’ (i.e. shared by some but not all disorders) within a particular diagnostic ‘family’. Ultimately, future studies with a similar methodology will be important for the development and validation of transdiagnostic, dimensional characterisations of mental illness as described in the RDoC project.
eLetters
No eLetters have been published for this article.