Introduction
Haemonchus contortus has been used as a biological nematode model for the study of different genetic mechanisms involved in anthelmintic resistance (Gilleard Reference Gilleard2013; Playford et al. Reference Playford, Smith, Love, Besier, Kluver and Bailey2014). For decades, ivermectin (IVM), a macrocyclic lactone (ML) drug, has been used as an effective dewormer to reduce gastrointestinal nematodes (GIN) from infected ruminants (Sutherland Reference Sutherland and Campbell1990; Suarez Reference Suarez2002; Laing et al. Reference Laing, Gillan and Devaney2017). Haemonchosis is a disease caused by the nematode H. contortus mainly as a result of its blood-feeding habit and the invasion process exacerbating inflammatory cell infiltration. In addition, H. contortus is a highly prevalent (> 80%) disease in tropical and temperate regions (Favero et al. Reference Fávero, Buzzulini, Cruz, Felippelli, Maciel, Salatta, Siniscalchi, Lopes, Teixeira, Soares, de Oliveira and da Costa2016; Besier et al. Reference Besier, Kahn, Sargison and Van Wyk2016; Ruiz-Huidobro et al. Reference Ruiz-Huidobro, Sagot, Lugagne, Huang, Milhes, Bordes, Prévot, Grisez, Gautier, Valadier, Sautier and Jacquiet2019). Therefore, grazing ruminants are frequently treated with anthelmintics in order to decrease GIN infection. However, the efficacy of anthelmintic drugs such as ivermectin and albendazole (benzimidazole anthelmintic family) has decreased, thus increasing the anthelmintic resistance problem (Martin et al. Reference Martin, Dube, Karlsson Lindsjö, Eydal, Höglund, Bergström and Tydén2020).
The study of anthelmintics and resistance mechanisms has produced important advances in the drugs that are frequently used, such as the benzimidazole family, where specific nucleotide changes were identified on the 200/167 (TTC/TAC) and 198 codons of the β-tubulin gene (Kwa et al. Reference Kwa, Veenstra and Roos1994; Silvestre and Cabaret Reference Silvestre and Cabaret2002; Ghisi et al. Reference Ghisi, Kaminsky and Mäser2007; Encalada-Mena et al. Reference Encalada-Mena, Tuyub-Solis, Ramirez-Vargas, Mendoza-de-Gives, Aguilar-Marcelino and López-Arellano2014; Dilks et al. Reference Dilks, Hahnel, Sheng, Long, McGrath and Andersen2020). For the ML anthelmintic family, different genetic mechanisms have been identified, most of them with IVM.
Ivermectin is one of the main anthelmintic drugs used by farmers. However, the nematicidal efficacy of IVM has decreased due to resistance problems worldwide. The mechanisms of IVM resistance (IVMr) in the Trichostrongylidae nematode family (e.g., Trichostrongylus, Teladorsagia, Cooperia, and Haemonchus) appear to be determined by more than a single gene (Köhler Reference Kohler2001; Laing et al. Reference Laing, Gillan and Devaney2017). The study of IVMr mechanisms involved nucleotide changes related to cytochrome oxidase genes (enzyme metabolisms), alterations in the genes of H. contortus coding for GluCl- channel subunits (proposed sites of ML), and the upregulation of P-glycoprotein (P-gp) genes as membrane transporters, among others (Khan et al. Reference Khan, Nisar, Yuan, Luo, Dou, Liu, Zhao, Li, Ahmad, Mehmood and Feng2020; Jakobs et al. Reference Jakobs, Yilmaz and Krücken2022). Recently, relevant notifications of IVMr mechanisms and P-gp suggest the reduction of resistance through competitive assays of plant metabolites and genetic mechanisms on P-gp receptors as the main target (Ménez et al. Reference Ménez, Alberich, Courtot, Guegnard, Blanchard, Aguilaniu and Lespine2019; Araujo et al. 2022). P-gp genes belong to the superfamily of the ATP-binding cassette (ABC transporters), and its relation with IVMr is because of the increased number of P-gp transcripts on the GIN receptors. Several studies were carried out on Caenorhabditis elegans as a free-living nematode and on H. contortus as a laboratory model (Ménez et al. Reference Ménez, Alberich, Courtot, Guegnard, Blanchard, Aguilaniu and Lespine2019; Jakobs et al. Reference Jakobs, Yilmaz and Krücken2022). Nevertheless, the endoparasite stages of GIN that affect grazing ruminants have to overcome with the host mechanisms of protection to achieve the adult stage, which is different than the C. elegans life cycle. At present, anthelmintic drugs are still the method of control against GIN of domestic ruminants. Therefore, the aim of the present study was to compare the relative gene expression of Haemonchus contortus P-glycoprotein (Hco-pgp) genes between the fourth (L4), infective (L3), and transitory infective (xL3) larval stages as laboratory models to study ivermectin (IVM) resistance.
Material and methods
Haemonchus contortus history strains
Haemonchus contortus resistant (IVMr) and susceptible (IVMs) strains have been conserved under cryopreservation since 2012 and 1990, respectively (Gonzalez-Garduño et al. Reference González-Garduño, Torres-Hernández, López-Arellano and Mendoza-de Gives2012; Campos et al. Reference Campos-Ruelas, Herrera-Rodríguez, Quiroz-Romero and Olazarán-Jenkins1990). When a lamb was infected with H. contortus IVMr or IVMs strain, infective larvae (L3) were collected and processed immediately to conserve them under cryopreservation. This procedure was performed two to three times per year to refresh the biological material. The H. contortus strains were characterised based on their phenotype traits with respect to mortality percentage after exposed to IVM under in vitro conditions. Criterion to classify IVMr strain was based on a low mortality (5%) caused by IVM. In addition, the relative and differential gene expression profile to IVMr and IVMs was determined per each H. contortus strains (Reyes-Guerrero et al. Reference Cedillo-Borda, López-Arellano and Reyes-Guerrero2020, Reference Reyes-Guerrero, Jiménez-Jacinto, Alonso-Morales, Alonso-Díaz, Maza-Lopez, Camas-Pereyra, Olmedo-Juárez, Higuera-Piedrahita and López-Arellano2023). Data obtained from the massive transcriptomic sequence were submitted at NCBI database (Bioproject PRJNA877658, https://www.ncbi.nlm.nih.gov) and each strain was identified as BioSample access number SAMN30717925 (IVMr) and SAMN30717926 (IVMs). Both H. contortus strain were conserved at the National Centre for Disciplinary Research in Animal Health and Innocuity from INIFAP-Agriculture, Mor. México.
Parasitological techniques
Production of H. contortus L3
Two Haemonchus contortus strains, IVMs and IVMr, were collected from two H. contortus egg-donor sheep. Two donors, Pelibuey 4-month-old lambs, were infected with 350 L3 larvae per kilogram of body weight orally. Faecal samples were collected directly from the rectum 21 days post-infection. The faecal samples were processed using the faecal culture technique in plastic bowls after seven days at 25°C. Then, L3 larvae, per strain, were recovered by the Baermann funnel technique and filtered through lens paper (Liébano-Hernández Reference Liébano2004; Cedillo-Borda et al. Reference Cedillo-Borda, López-Arellano and Reyes-Guerrero2020). Larvae were cleaned using 40% sucrose and centrifugation at 1500 g for 5 min (Contreras-Ochoa et al. Reference Contreras-Ochoa, Lagunas-Martínez, Reyes-Guerrero, Bautista-García, Tello-López, González-Garduño and López-Arellano2019; Cedillo-Borda et al. Reference Cedillo-Borda, López-Arellano and Reyes-Guerrero2020). To eliminate the second moult, larvae were treated with 0.187% sodium hypochlorite for 10 min at 25°C and rinsed several times with distilled water. The L3 larvae were counted and used immediately.
Meriones unguiculatus and H. contortus infection
Three male 2-month-old gerbils weighing 25 g were obtained from the National Institute of Public Health, Cuernvaca, Morelos, Mexico. In a previous study, all gerbils were dewormed with albendazole (Pfizer, USA), administered orally at 50 μl per day for 5 days. In a preliminary test, the optimal oral dose of L3 to infect gerbils, per strain, was determined to be 10,000 exsheathed larvae to establish a good infection. Similarly, the optimal date after infection to sacrifice the gerbils in order to collect the first endoparasite stage from the digestive tract was 12–13 days post-infection (De Jesús-Gabino et al. Reference De Jesús-Gabino, Mendoza-de Gives, Salinas-Sánchez, López-Arellano, Liébano-Hernández, Hernández-Velázquez and Valladares-Cisneros2010; Zamilpa et al. Reference Zamilpa, García-Alanís, López-Arellano, Hernández-Velázquez, Valladares-Cisneros, Salinas-Sánchez and Mendoza-de Gives2019).
Hamonchus contortus L 3 in vitro culture
The in vitro culture of H. contortus L3 to develop at xL3 was performed with 30,000 unsheathed and cleaned L3. Larvae were treated with 100 μl of antibiotic–antimycotic 100× (GibcoTM, USA) and amphotericine B (Thermo Fisher Scientific, USA) in 20 ml of sterile phosphate-buffered saline (PBS) at pH 7.4 and incubated overnight at 4°C. Then, larvae were retrieved by centrifugation at 1500 g for 5 min, to be incubated at 37°C in a 5% CO2 atmosphere for 21 days. Clean larvae were kept within 125 culture bottles containing 30 ml of Hanks’ Balanced Salt Solution (HBSS, Thermo Fisher Scientific, USA), 150 μl of antibiotic–antimycotic, 300 μl of amphotericine B, and 5 μl of sheep red blood cells that were previously washed with VyM solution (Rojas-Martinez et al. Reference Rojas-Martínez, Rodríguez-Vivas, Figueroa-Millán, Acosta-Viana, Gutiérrez-Ruiz and Álvarez-Martínez2016; Cedillo-Borda et al. Reference Cedillo-Borda, López-Arellano and Reyes-Guerrero2020). The HBSS was changed every third day under sterile conditions.
Morphometric characteristics of H. contortus xL 3 and L 4
Twenty-five H. contortus xL3 and L4 stages were collected from in vitro assays and from infected gerbils, respectively, to confirm the morphometric characteristics. After collection, larvae were kept in PBS at pH 7.4 and 37°C, with each stage immediately set on slides to measure body length and width and to identify the main morphological characteristics from each stage. Measurements were achievement using a Leica CTR6 LED microscope at 4× and 20× magnification.
Resistance of IVM studies using P-gp genes on H. contortus by reverse transcription quantitative real-time polymerase chain reaction (RT-qPCR)
RNA extraction and RT technique
Total RNA (TRNA) was collected from 5000, 2000, and 200 H. contortus L3, xL3, and L4 resistant and susceptible to IVM, respectively, in order to treat them with TRIzol (Invitrogen, Carlsbad CA, USA), following the manufacturer’s instructions. Larvae were disrupted by two sets of 40 s at 400 rpm using 1-mm zirconium beads in a BeadBug microtube D1030 homogenizer (Benchmark Scientific, South Plainfield, USA). The RNAT concentration was calculated by spectrophotometry using a NanoPhotometer NP80 (IMPLEN, USA), and the integrity of TRNA was determined using ethidium bromide staining via 2.5% agarose gel electrophoresis. Then, RT was performed for cDNA synthesis at 300 ng of TRNA concentration using the commercial ImProm-II Reverse Transcription System® (Promega, Madison, USA), following the manufacturer’s instructions and the procedure cited by Cedillo-Borda et al. (Reference Cedillo-Borda, López-Arellano and Reyes-Guerrero2020) and Bonilla-Suárez et al. (2022). Three individual samples of each larval stage and each strain were collected to obtain the TRNA. In order to obtain the necessary quantity of TRNA, the three samples were mixed to achieve the amount required to cDNA synthesis assays.
RT-qPCR assays
Nucleotide sequences were synthesised from partial encoding domain sequences localised in the union of nucleotides from Hco-pgp genes of H. contortus. The RT-qPCR assays were performed in triplicate per gene for each H. contortus larval stage (Reyes-Guerrero et al. Reference Reyes-Guerrero, Cedillo-Borda, Alonso-Morales, Alonso-Díaz, Olmedo-Juárez, Mendoza-de-Gives and López-Arellano2020; Cedillo-Borda et al. Reference Cedillo-Borda, López-Arellano and Reyes-Guerrero2020). The qPCR reaction for each Hco-pgp and housekeeping gene (β-tubulin and GAPDH) was carried out at a final volume of 20 μl in 0.2-ml microtubes. The reaction was prepared using GoTaq® RT-qPCR Master Mix (Promega, Madison USA), 20 μM oligonucleotides, and 300 ng of cDNA. The PCR conditions were as follows: initial denaturation at 95°C for 5 min, followed by 40 cycles of denaturation at 95°C for 10 s, annealing at 63°C for 15 s, and extension at 72°C for 20 s. The procedure ended with a step to determine the dissociation temperature, with a temperature increase from 65 to 95°C (Cedillo-Borda et al. Reference Cedillo-Borda, López-Arellano and Reyes-Guerrero2020). RT-qPCR was carried out in a Rotor-Gene 6000 thermocycler (Corbet Research, Hilden, Germany) to amplify the PCR products at 470 and 510 nm. The oligonucleotide sequences of Hco-pgp and housekeeping genes used in the present study are shown in Table 1.
Table 1. Primers and sequences used for reverse transcription – real-time qPCR analysis for H. contortus stages from both strains

Hco = Haemonchus contortus; pgp = P-glycoprotein genes; GAPDH and β-tubulin = housekeeping genes
Relative expression of Hco-pgp genes
For relative expression study, the comparative threshold cycle (CT) method was used (Pfaffl Reference Pfaffl2001; Livak and Schmittgen Reference Livak and Schmittgen2001). Two H. contortus strains, IVMr and IVMs, were used to perform two independent molecular experiments of Hco-pgp genes as follows: Experiment 1) comparison of xL3 and L4 (IVMr) vs L3 (IVMr) as control group, respectively; and Experiment 2) comparisons of xL3 (IVMr) vs xL3 (IVMs), and L4 from gerbils (IVMr) vs L4 from gerbils (IVMs). The gene expression analysis was based on the number of cycles at which the amplification plot crossed the threshold. Raw data from three larval stages – L3, xL3, and L4 of H. contortus – were collected from each assay, and the references were analysed to obtain the magnitude of change and the p value. Based on previous data (unpublished) from the geometric mean analysis of housekeeping genes β-actin, β-tubulin, and GAPDH, we evaluated these two last genes that eventually showed higher stabilization (as reference genes) with respect to their relative expression. The CT values for β-tubulin and GAPDH genes were subtracted from the CT values for each target expression gene to normalise the mRNA content and provide a relative expression value for each Hco-pgp gene. This value was defined as ∆CT. Gene expression from the xL3 and L4 H. contortus stages was compared to the gene regulation of L3 stage according to Reyes-Guerrero et al. (Reference Reyes-Guerrero, Cedillo-Borda, Alonso-Morales, Alonso-Díaz, Olmedo-Juárez, Mendoza-de-Gives and López-Arellano2020). To assess the effects of IVM, the means of ∆CT from H. contortus larval stages were subtracted from the mean ∆CT control group, identified as H. contortus L3 IVMr. These values were defined as ∆∆CT. The relative increases or decreases were then calculated as 2∆∆CT. The magnitude of change was analysed by Student’s t-test (p = 0.05), using the ΔΔCT for each technical replicate (n = 3) of each target gene, housekeeping genes, and control group via the Qiagen GeneGlobe platform (https://www.qiagen.com).
Statistical analysis
The length and width of L3, xL3, and L4 were analysed by ANOVA using a completely randomised design in the general linear model (Statistical Analysis System, version 8.9, USA). The difference between treatments was compared with the Tukey test (p < 0.05).
Results
Hamonchus contortus xL 3 and L 4 morphometric characteristics
Twenty-five H. contortus L3, xL3, and L4 stages were used to measure the total length, width, and body size at each stage under study. Table 2 shows the significant differences (p < 0.05) between the three larval stages. Important differences were observed in H. contortus L3 and xL3 compared with L4, the endoparasite stage collected from gerbils 13 days post-infection. However, no significant p value (p > 0.05) was observed between the H. contortus L3 and xL3 measurements for any morphological parameter. Nevertheless, important morphological changes were detected from the H. contortus stages. For example, an increased level of around 150% was determined to be H. contortus L4 from the total body length measurement in comparison with the L3 reference larval stage, followed by the xL3 stage showing a 22% enlarged body size in comparison with L3. In addition, morphological changes were observed for each H. contortus stage under study (Figure 1a–c). The oral cavity displayed different shapes, with a round sealed oral cavity observed for the L3 stage; this larval stage is also identified using lipoid granule staining. The H. contortus xL3 and L4 showed important morphological changes, where a semi-square oral cavity appeared to be developing and, together with the oesophagus, intestinal tube, and the anal aperture, all of them were well defined.
Table 2. Morphometric comparision of three Haemonchus contortus larval stages colected from in vitro culture and from infected Meriones unguiculatus
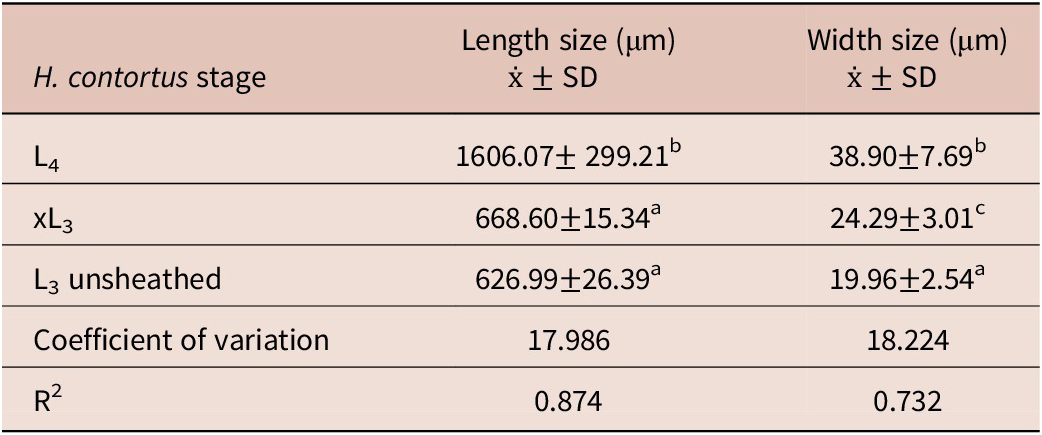
Means with different letters showed significance differences (p < 0.05); SD = standard deviation; R2 = correlation coefficient
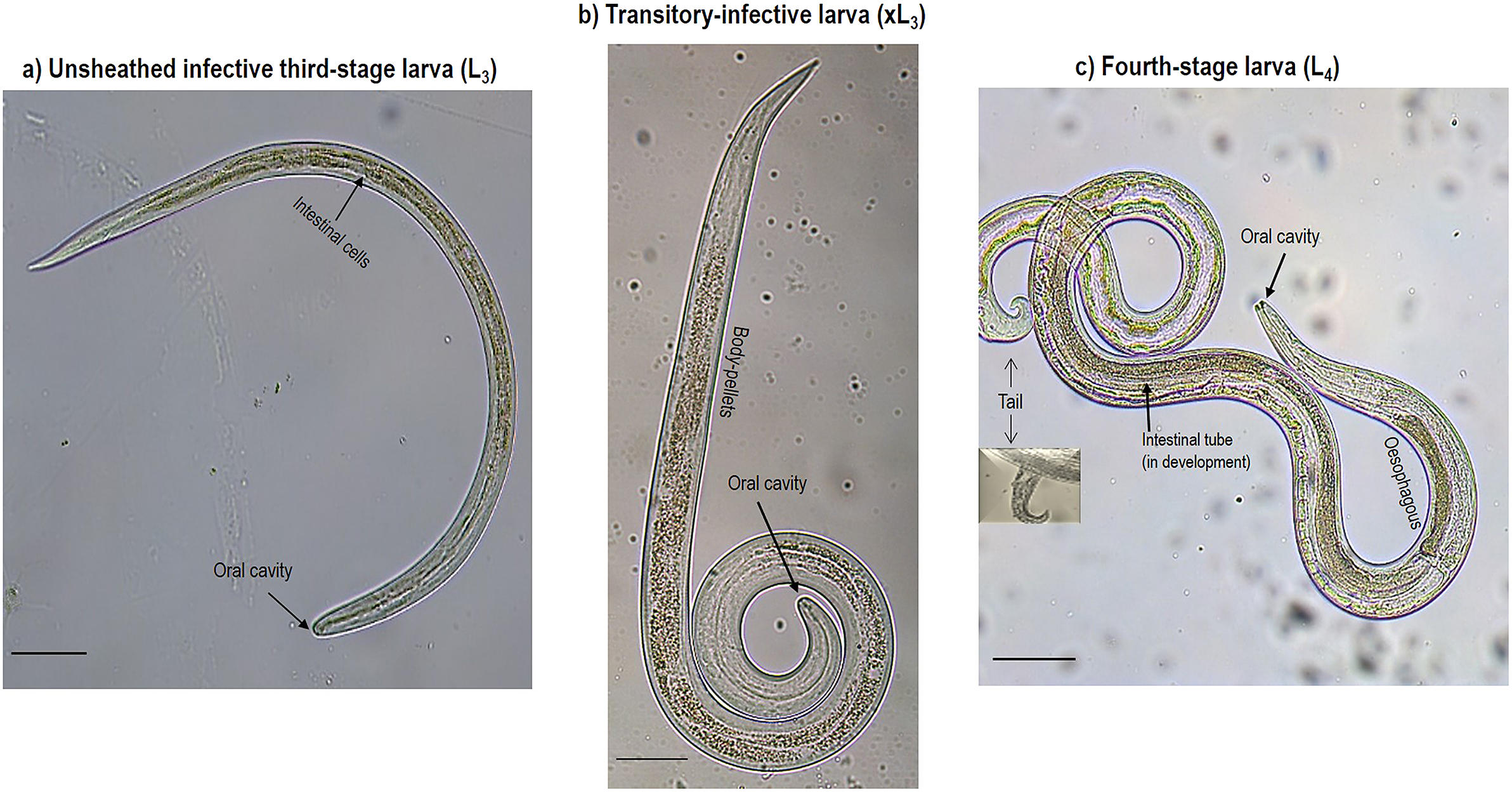
Figure 1a–c. Morphology description of Haemonchus contortus larval stages. a) H. contortus unsheathed third-stage larva (L3); b) transitroly-infective larva (xL3); c) fourth stage larva (L4). Bar scale = 20x.
Relative expression level of Hco-pgp genes
Comparative relative expression level between H. contortus stages IVMr
Data collected from the comparative analysis of Hco-pgp genes between H. contortus xL3 and L4 in comparison with L3 are shown in Table 3. Four Hco-pgp genes (1, 2, 3, and 4) were upregulated (p < 0.05) after the comparative analysis between xL3 and L4 stages, with L3 as the control group. Moreover, Hco-pgp genes 1, 2, 3, and 4 increased the level of expression in both larval stages, with the major expression displayed by Hco-pgp4 (463.83-fold change) corresponding to the L4 stage. Moreover, a comparative analysis was carried out for L4 and xL3, the latter used as the reference stage instead of L3, displaying overexpression of Hco-pgp genes 1, 2, 3, 4, 9, and 16 with 6.43–118.6-fold changes (p < 0.05).
Table 3. Comparative relative expression analysis of Hco-pgp genes from H. contortus between larval stages resistant to ivermectin and between resistant and susceptible larvae strains to ivermectin

Hco-pgp = Haemonchus contortus P-glycoprotein genes; L3 = infective stage used as control group; xL3 = transitory infective larval stage collected from in vitro culture; L4 = fourth endoparasite stage collected from infected gerbils; IVM = ivermectin; rr = resistant to IVM; ss = susceptible to IVM (used as control); bold letters = upregulation; italic letter: sub-regulated; black: normalized; * = p < 0.05; ** = p < 0.001
Comparative expression level between H. contortus stages IVMr vs IVMs
The comparative analyses of Hco-pgp genes between H. contortus xL3 and L4 larvae stages from the IVMr strain in comparison with the IVMs strain are shown in Table 3. Most of the Hco-pgp genes were upregulated (p < 0.05) after the comparative analysis between xL3 and L4 stages with their respective IVMs larvae as control group, except Hco-pgp9 and 14 that were downregulated for the xL3 stage. The Hco-pgp4 gene showed 68.29- and 188.7-fold change as maximum expression level in IVMr xL3 and L4 stages vs same stages of IMVs, respectively. Lower levels of expression were shown by Hco-pgp9: 0.03- and 39.88-fold changes for xL3 and L4, respectively. Meanwhile, for Hco-pgp16, 2.13- and 23.83-fold changes, for xL3 and L4, were recorded respectively.
Discussion
The occurrence of anthelmintic resistance has increased worldwide in the livestock industry and has affected animal health and farmer economy (Preston et al. Reference Preston, Jabbar and Gasser2016; Wit et al. Reference Wit, Dilks and Andersen2021). The use of anthelmintics is necessary in endemic regions to reduce the prevalence of GIN and the damage on grazing ruminants. However, the deworming procedure should be followed by a parasite diagnosis for prudent use of the proper anthelmintic drug (Kotze et al. Reference Kotze, Gilleard, Doyle and and Prichard2020). For IVMr, it is important to consider that P-gp genes are ATP-dependent efflux of various structurally unrelated exogenous compounds as a protective function against xenobiotic toxicity. Moreover, due to its broad specificity, P-gp plays a relevant role in the drug interactions: for instance, with IVMr problems, this function might increase the efflux of anthelmintics and therefore reduce their efficacy (Kotze et al. Reference Kotze, Gilleard, Doyle and and Prichard2020; Whittaker et al. Reference Whittaker, Carlson, Jones and Brewer2017; Ardelli Reference Ardelli2013).
Overexpression of different P-pg genes is one of the main mechanisms related to IVMr in parasitic nematodes on equids and domestic ruminants: for instance, Parascaris spp. and P-gp genes 11 and 16 (Martin et al. Reference Martin, Dube, Karlsson Lindsjö, Eydal, Höglund, Bergström and Tydén2020; Dube et al. Reference Dube, Hinas, Roy, Martin, Åbrink, Svärd and Tydén2022), Cooperia spp. and Teladorsagia circumcincta with Coo-pgp9 and Tci-pgp9, respectively (Dicker et al. Reference Dicker, Nisbet and Skuce2011; Areskog et al. Reference Areskog, Engström, Tallkvist, von Samson-Himmelstjerna and Höglund2013; Turnbull et al. Reference Turnbull, Jonsson, Kenyon, Skuce and Bisset2018), and diverse Hco-pgp genes (2, 9, 13, and 16) displayed upregulation on different H. contortus strains associated with IVMr (Godoy et al. Reference Godoy, Che, Beech and Prichard2015a; Godoy et al. Reference Godoy, Lian, Beech and Prichard2015b; Godoy et al. Reference Godoy, Che, Beech and Prichard2016; David et al. Reference David, Lebrun, Duguet, Talmont, Beech, Orlowski, André, Prichard and Lespine2018; Reyes-Guerrero et al. Reference Reyes-Guerrero, Cedillo-Borda, Alonso-Morales, Alonso-Díaz, Olmedo-Juárez, Mendoza-de-Gives and López-Arellano2020). At present, the development of parasitic stages in in vitro culture and endoparasite stages collected from infected gerbils indicated the use of biological models to study anthelmintic resistance mechanisms like IVMr and P-gp genes (Geary Reference Geary2016; Niuciura et al. Reference Niciura, Minho, McIntyre, Vieira, Hiromi, Novita, de Souza Chagas and Talamini do Amarante2023). In our study, the overexpression of P-gp genes was associated to H. contortus xL3 and L4. Although P-gp genes 9 and 10 were independently overregulated on H. contortus xL3 or L4, this result might be associated with the gene diversity present in GIN (Yang et al. Reference Yang, Guo, Zhang, Huang, Chen and Du2017; Ma et al. Reference Ma, Wang, Korhonen, Ang, Williamson, Young, Stroehlein, Hall, Koehler, Hofmann and Gasser2018; Sallé et al. Reference Sallé, Doyle, Cortet, Cabaret, Berriman, Holroyd and Cotton2019; Doyle et al. Reference Doyle, Tracey, Laing, Holroyd, Bartley, Bazant, Beasley, Beech, Britton, Brooks, Chaudhry, Maitland, Martinelli, Noonan, Paulini, Quail, Redman, Rodgers, Sallé, Shabbir, Sankaranarayanan, Wit, Howe, Sargison, Devaney, Berriman, Gilleard and Cotton2020). The P-gp genes are strongly associated with IVMr in different GIN of ruminants (Kotze et al. Reference Kotze, Gilleard, Doyle and and Prichard2020; Martin Reference Martin, Robertson and Choudhary2021). Due to the importance of P-gp genes related to ML, diverse studies have been carried out to know more about the mechanisms of resistance through the competency substrate studies using transfected mammal cells for overexpressing Hco-pgp9 and 16 in adult stages (Godoy et al. Reference Godoy, Che, Beech and Prichard2015a, Reference Godoy, Che, Beech and Prichard2016). These studies suggest the interaction between Hco-pgp and IVM efflux related to resistance. In addition, an increased number of transcripts were observed in Hco-pgp3 and 10 in H. contortus L4 and adult stages (Issouf et al. Reference Issouf, Guégnard, Koch, Le Vern, Blanchard-Letort, Che, Beech, Kerboeuf and Neveu2014). The results showed by these studies showed similarities with our data using xL3 and L4 as a laboratory model to study IVM resistance. In addition, previous studies notified the homology between Hco-pgp3 and Hco-pgp4 genes that may suggest that they correspond to the same gene (Issouf et al. Reference Issouf, Guégnard, Koch, Le Vern, Blanchard-Letort, Che, Beech, Kerboeuf and Neveu2014; Gerhard et al. Reference Gerhard, Krücken, Neveu, Charvet, Harmache and von Samson-Himmelstjerna2021). Interestingly, in this study, the Hco-pgp4 showed the highest level of relative expression suggesting other genetic mechanisms, such as polymorphisms (SNPs), that could be involved in anthelmintic resistance for a specific anthelmintic drug, such as IVM.
However, the nematode C. elegans is one of the main laboratory models for the study of P-gp genes and ML resistance drugs (Harris et al. Reference Harris, Antoshechkin, Bieri, Blasiar, Chan, Chen, De La Cruz, Davis, Duesbury, Fang, Fernandes, Han, Kishore, Lee, Müller, Nakamura, Ozersky, Petcherski, Rangarajan, Rogers, Schindelman, Schwarz, Tuli, Van Auken, Wang, Wang, Williams, Yook, Durbin, Stein, Spieth and Sternberg2010; Menéz et al. 2019; Lu et al. Reference Lu, Lai, Liao and Tsai2020). However, as a P-gp identifier, C. elegans has some limitations in its genome compared with GIN of ruminants. Fourteen P-gp genes are identified, but some of them are absent, such as P-gp16 (Williamson and Wolstenholme Reference Williamson and Wolstenholme2012; Lamassiaude et al. Reference Lamassiaude, Courtot, Corset, Charvet and Neveu2022). Thus, the study of endoparasitic stages is crucial since they share a close relationship with their host and they could be used as one of the main anthelmintic targets (Reyes-Guerrero et al. Reference Reyes-Guerrero, Olmedo-Juárez and Mendoza-de Gives2021).
Haemonchus contortus xL3 and L4 used as laboratory models have some advantages because of the short collection period and relatively easy laboratory maintenance (Hutchinson and Slocombe Reference Hutchinson and Slocombe1976; Yang et al. Reference Yang, Guo, Zhang, Huang, Chen and Du2017). Moreover, specific differences have been observed between different H. contortus larval stages using animal laboratory models such as gerbils, mice, rabbits, and guinea pigs compared with the stages from sheep as a definitive host (Table 4). Interestingly, in this study, we observed similar characteristics with those larvae in the development of H. contortus in sheep and other laboratory models – for instance, the L4 length and thicknesses and some main morphological features (i.e., digestive tract, crystalline inclusions, etc.). Also, in some studies, similar pathological lesions have been observed on infected stomachs with H. contortus from laboratory models with the abomasum from infected sheep (Table 4). These results suggest the importance of using laboratory animal models as useful tools to study anthelmintic resistance. These animal models could be used to establish specific methods of diagnosis (i.e., using molecular markers or biomarkers) and in studies focused to maintain the efficacy of anthelmintics. Ruminants grazing on contaminated pastures are continuously exposed to GIN; therefore, good practice for using anthelmintic drugs should be established in the husbandry systems (Miller et al. Reference Miller, Waghorn, Leathwick, Candy, Oliver and Watson2012; Besier et al. Reference Besier, Kahn, Sargison and Van Wyk2016; Kotze et al. Reference Kotze, Gilleard, Doyle and and Prichard2020). The present study provides information that contributes to the control of GIN on ruminants and the use of laboratory models as the first step.
Table 4. Review of morphometric characteristic notifications of the fourth larval stage of H. contortus

Conclusion
H. contortus xL3 and L4 stages were evaluated to identify the expression level of RNA transcripts on a H. contortus strain resistant and a susceptible strain to IVM with respect to IVMr L3 larval stage. Confirmation of P-gp genes was observed in most of the evaluated genes. This fact suggests that xL3 or L4 stage could be used for future IVM resistance studies. In addition, in this study, a higher level of relative expression of Hco-pgp genes in H. contortus IVMr from infected gerbils was observed. This result could be highly associated with IVM resistance phenotype. However, analyses about Hco-pgp genes in H. contortus are still necessary to understand their functionality in H. contortus larvae obtained from laboratory animal models. The problem of resistance to different anthelmintic drugs is highly dispersed, and drugs such as the frequently used IVM should be used prudently because they have a broad spectrum of action and their conservation is important.
Author statement
Reyes-Guerrero DE: Conceptualisation, methodology, investigation, validation, writing – original draft; writing – review and editing. Maza-Lopez J: methodology, investigation, validation, writing – original draft. Higuera-Piedrahita RI: conceptualisation, investigation, writing – review and editing. Mendoza-de-Gives P: formal analysis, writing – review and editing, visualisation. Camas-Pereyra R: methodology, formal analysis, visualisation. López-Arellano ME: conceptualization, investigation, writing – review and editing, supervision, project administration, funding acquisition.
Financial support
DE Reyes-Guerrero received a scholarship from the Mexican institution CONACYT [grant number 384195/631929, 2020–2024]. This project received financial support from the Mexican institutions CONACYT-SEP [grant number 287598, 2021] and CENID-SAI, INIFAP [grant number 9495336224, 2021].
Competing interest
The authors declare no conflict of interest.
Ethical standard
Experimental sheep and gerbils were treated following the Norma Oficial Mexicana (Official Rule Number) NOM-051-Z00-1995 (www.senasica.gob.mx), the Ley Federal of Sanidad Animal (Federal Law for Animal Health (www.diputados.gob.mx/LeyesBiblio/ref/Ifsa.htm), and the National University Autonomous of Mexico Num. MC-2018/2-14. The authors assert that all procedures contributing to this work comply with the ethical standards of the relevant national and institutional guides on the care and use of laboratory animals.
Disclosure
The authors of this manuscript have no financial or personal relationship with other people or organization that could be inappropriately influence of the content of this paper.