There is considerable concern about the continuing rise in the prevalence of type 2 diabetes (T2D), which in the UK increased from about 4000 age-standardised prevalence rates per 100 000 in 1990 to about 9000 age-standardised prevalence rates per 100 000 in 2020 in men(Reference Goodall, Alazawi and Hughes1). The trend in women is similar, albeit at a slower rate and with some evidence of plateauing at about 7000 age-standardised prevalence rates per 100 000 from 2015(Reference Goodall, Alazawi and Hughes1). Diabetes UK(Reference Diabetes2) reports that treating diabetes costs the National Health Service approximately £14 billion per year and no doubt there will be additional indirect costs. Across the European Union there is considerable variability in the rate of standardised prevalence change over the past 30 years although for most European Union countries it has been less than that in the UK. Interestingly, in the UK and the European Union, increases in T2D prevalence have not been accompanied by an increase in associated mortality and indeed with a small reduction in some countries. However, certification of the cause of death in subjects with diabetes-induced CVD is complicated, with many not certified to have died from diabetes but from CVD(Reference McEwen, Lee and Backlund3). Indeed subjects with T2D have two times the risk of CVD which is indeed often the actual cause of death(Reference Abdul-Ghani, DeFronzo and Del Prato4). Partly because of the close link between T2D and CVD, it is now common to include both under the umbrella term cardiometabolic diseases and this term is used in parts of the present paper.
Obesity, lack of physical activity and age are major risk factors for developing T2D with regular exercise being associated with up to a 50 % reduction in the relative risk (RR)(Reference Sanders5). Diet is a further risk factor for T2D, in part because together with exercise, diet is related to obesity and also because dietary composition can influence insulin sensitivity which generally declines as part of the aetiology of T2D.
Whilst the mortality rate from CVD in the UK has fallen considerably over the past 50 years they remain the largest cause of death in UK males(6). In addition, CVD remain the greatest cause of death in the European Union and has a major impact on healthcare costs(7).
This short review concentrates on the associations between dairy foods and the risk of T2D and CVD. In addition, since dairy foods are often the greatest dietary source of SFA, the review will also look at recent evidence which casts doubt that dairy SFA are a risk factor for CVD.
Dairy consumption and risk of type 2 diabetes and CVD
Type 2 diabetes
Some early studies(Reference Pereira, Jacobs and Van Horn8–Reference van Meijl, Vrolix and Mensink10) strongly suggested that consumption of dairy foods can moderate components of the metabolic syndrome which represents a set of risk factors including insulin resistance and abdominal obesity both of which can substantially increase the risk for T2D. A number of meta-analyses(Reference Tong, Dong and Wu11–Reference Aune, Norat and Romundstad13) also concluded inverse associations between total dairy and low-fat dairy consumption and T2D. More recently, Imamura et al. reported on the association between biomarkers of dairy fat consumption and the incidence of T2D in a pooling study involving sixteen prospective studies(Reference Imamura, Fretts and Marklund14). In total this involved data from almost 64 000 adults of which about 15 000 became type 2 diabetics during the 20 years follow-up. Overall, the project showed that circulating fatty acid biomarkers of dairy fat intake (15 : 0, 17 : 0, trans 16 : 1n-7) were each associated with a lower risk of developing T2D independently of major risk factors for T2D such as age and obesity. Imamura et al. estimated that subjects with higher concentrations of the sum of the three biomarkers had a 29 % (95 % CI: 21, 37) lower risk of T2D than those with lower concentrations(Reference Imamura, Fretts and Marklund14). These findings supported the earlier work(Reference Mozaffarian, de Oliveira Otto and Lemaitre15) which also showed an inverse association between circulating trans 16 : 1n-7 and incident T2D. Unfortunately the fatty acid biomarker technique cannot differentiate between different dairy foods, nevertheless this major study strongly pointed to beneficial effects of dairy foods on the risk of T2D. There were indications that the benefit was stronger in women than men.
Guo et al.(Reference Guo, Givens and Astrup16) reviewed the evidence from a range of meta-analyses of cohort studies plus some recently published cohort studies which examined the association of dairy foods and T2D. The overall conclusions indicated that dairy food consumption has a neutral or moderately beneficial effect on glucose homoeostasis and T2D risk. It was also concluded that the meta-analysis of Soedamah-Muthu and de Goede(Reference Soedamah-Muthu and de Goede17) showed good evidence of a non-linear inverse association between yoghurt consumption and T2D risk (RR 0·86, 95 % CI: 0·83, 0·90) at an intake of 80 g/d compared with no yoghurt consumption. This conclusion was supported by another study(Reference Drouin-Chartier, Li and Korat18) which, based on the combination of three large US cohorts (34 224 men; 158 128 women), showed that increasing yoghurt intake by >0·5 servings/d was associated with a moderately reduced risk (RR 0·89, 95 % CI: 0·82, 0·96) of T2D. Guo et al.(Reference Guo, Givens and Astrup16) noted that whilst some earlier studies with cheese showed a similar association to that of yoghurt, some more recent ones did not and indeed one(Reference Drouin-Chartier, Li and Korat18) reported an increased risk of T2D associated with consumption of >0·5 servings/d of cheese (RR 1·09, 95 % CI: 1·02, 1·16). The systematic review and meta-analysis of seven prospective studies (nine populations) on fermented dairy products and cardiometabolic diseases(Reference Companys, Pla-Pagà and Calderón-Pérez19) reported that yoghurt consumption was associated with a 27 % reduction in the risk of T2D (RR 0·73, 95 % CI: 0·70, 0·76) although in three prospective studies cheese intake was associated with an increase in the risk of T2D (RR 1·24, 95 % CI: 1·03, 1·49) although this association was NS in any of the three individual studies. A recent study(Reference Wuni, Lakshmipriya and Abirami20) also showed a benefit of fermented dairy (≥2 cups/d) over unfermented dairy (≥4 cups/d) for a reduced risk of the metabolic syndrome in Asian Indians.
Overall, the evidence from prospective studies (Table 1) is suggestive that dairy foods are generally associated with a neutral risk of T2D apart from yoghurt which is indicative of a reduced risk. An understanding of the mechanism(s) involved in the consistent beneficial effects of yoghurt consumption on risk of T2D remains incomplete. Fernandez et al.(Reference Fernandez, Panahi and Daniel21) suggest that calcium (Ca), protein, bioactive nutrients and live cultures present in yoghurts are likely to be contributors, but admit that their role within the food matrix is not known. Given the increasing prevalence of T2D and the consistent beneficial association with yoghurt consumption suggests that this topic needs to be investigated with urgency. Interestingly, a recent risk analysis(Reference Cohen, Bylsma and Movva22) indicates that 200 000 US disability-adjusted life years (4·5 %) due to T2D may be prevented by increased dairy consumption.
Table 1. Selection of dose–response meta-analyses examining the association of dairy food consumption and the risk of cardiometabolic diseases
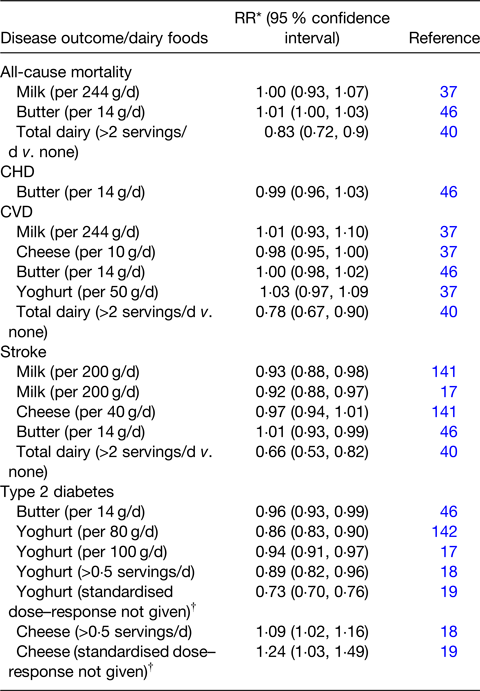
Adapted from(Reference Givens, Grundy and Wilde41) and extended with additions based on this review.
* Relative risk. Note: equivalent values for Ref. Reference Dehghan, Mente and Rangarajan40 are hazard ratios.
† May be based on highest v. lowest in each study.
Milk proteins are rich sources of branched-chain amino acids (BCAA), leucine in particular. Whey protein in particular is used extensively to provide an anabolic stimulus, mediated mainly by leucine, to muscle protein synthesis in sports people(Reference Jackman, Witard and Philp23) and the elderly(Reference Gorissen and Witard24). Recent data(25) indicate the world market for whey protein was worth approximately $8.7 billion in 2019 which is expected to increase by a compound growth rate of 9·8 % up to 2027. Europe is the largest market (34 %) with Germany, France, Spain and Italy followed by the UK consuming the largest proportion. Nutritional supplements have the greatest market share of whey proteins. It is therefore concerning that circulating BCAA concentrations have often been associated with an increased risk of T2D in cross-sectional and prospective studies(Reference Wang, Larson and Vasan26–Reference Yu, Song and Zhu29) and with an increased risk of CVD(Reference Ruiz-Canela, Toledo and Clish30,Reference Tobias, Lawler and Harada31) . In the case-cohort study(Reference Ruiz-Canela, Toledo and Clish30), BCAA were particularly associated with stroke risk in a high CVD risk population. The Tobias et al. study(Reference Tobias, Lawler and Harada31) showed that the BCAA–CVD association was about 20 % higher in women who developed T2D before CVD, but represented a risk similar to, but independent of, circulating LDL-cholesterol (LDL-C). It was suggested that BCAA may represent a biochemical phenotype of CVD risk which is common to T2D and may be related to early excess adiposity-related activity. A recent meta-analysis(Reference Solon-Biet, Griffiths and Fosh32) using data mainly from mice studies confirmed that increasing dietary BCAA intake increases circulating BCAA concentration in a curvilinear fashion up to a diet BCAA concentration of about 0·5 kJ/g and a plateau blood concentration of about 40 μg/ml. This plateau effect is thought to be due to activation of branched-chain α-keto acid dehydrogenase, a mitochondrial enzyme complex found in the liver and muscle, which reduces blood BCAA concentration. Increased BCAA intake increased plasma insulin concentrations and homoeostatic model assessment of insulin resistance, and impaired glucose tolerance but was associated with reduced food and energy intake(Reference Solon-Biet, Griffiths and Fosh32). Higher BCAA intake has been associated with a lower prevalence of overweight or obesity in human subjects(Reference Qin, Xun and Bujnowski33).
Given the extensive use of whey protein in sport and other activities, the impact its BCAA may have on T2D and CVD risk in otherwise healthy young and old subjects warrants urgent attention.
CVD
A range of systematic reviews with meta-analyses on the outcomes of prospective cohort studies looking at the association between dairy foods and risk of CVD have been published over the past 20 years. Those published in the first 10 years of this century concluded that generally, high milk consumption did not increase the RR for CHD compared with low consumers(Reference Elwood, Pickering and Givens34). A further meta-analysis(Reference Mente, de Koning and Shannon35) combined data from prospective cohort and clinical studies and also showed no significant increase in the RR of CHD in high v. low milk consumers (RR 0·94, 95 % CI: 0·75, 1·13). In recent years, the food dose–risk response meta-analysis methodology has been developed(Reference Soedamah-Muthu, Guo and Givens36) which provides more insight into the associations between food intake and disease risk and provides information on the shape (e.g. non-linear) of the association. Such studies include a number(Reference Soedamah-Muthu and de Goede17,Reference Soedamah-Muthu, Guo and Givens36,Reference Guo, Astrup and Lovegrove37) , which looked primarily at risk of CVD and other outcomes. In addition, two others(Reference Poppitt38,Reference Nestel and Mori39) have published extensive reviews on the subject of dairy food consumption and human health.
The PURE study(Reference Dehghan, Mente and Rangarajan40) involved about 137 000 subjects aged 35–70 years living in twenty-one countries across five continents. After a 9·1 years of follow-up it examined the association between consumption of dairy products (total and milk, yoghurt and cheese) with non-CVD and CVD mortality and the risk of CVD. Higher consumption of total dairy foods (>2 servings/d v. none) was associated with lower risks of all-cause mortality (ACM) (hazard ratio (HR) 0·83, 95 % CI: 0·72, 0·96; P trend = 0·0052), non-CVD mortality (HR 0·86, 95 % CI: 0·72, 1·02, P trend = 0·046), CVD mortality (HR 0·77, 95 % CI: 0·58, 1·01, P trend = 0·029), major CVD events (HR 0·78, 95 % CI: 0·67, 0·90; P trend = 0·0001) and stroke (HR 0·66, 95 % CI: 0·53, 0·82, P trend = 0·0003). Greater consumptions of milk and yoghurt, but not cheese, were associated with a lower risk of the combination of mortality or major CVD events. No significant association was seen with myocardial infarction (MI). In addition, butter intake was low and not significantly associated with disease outcomes. Whilst the outcomes are broadly in agreement with earlier studies and meta-analyses, this is believed to be the first study to involve such large and diverse sets of subjects with substantial variation in habitual intake of dairy and other foods between countries.
Givens(Reference Givens, Grundy and Wilde41) summarised the outcomes of a number of dose–response meta-analyses on associations of various dairy food types with ACM, CVD and stroke. With some additions, these are summarised in Table 1 and overall are in good agreement with earlier findings that dairy food intake has a rather neutral association with the risk of CVD, although there are now indications of a negative association for consumption of milk and total dairy with the risk of stroke. Recent findings(Reference Sellem, Flourakis and Jackson42) agree with these findings reporting no significant associations between dairy intakes and risk of total CVD and CHD but also showing that an intake of at least 160 g/d fermented dairy foods (e.g. cheese and yoghurt) was associated with a reduced risk of cerebrovascular diseases compared with consumption of less than 57 g/d (HR 0·81, 95 % CI: 0·66, 0·98). The recent findings of Olsson et al.(Reference Olsson, Larsson and Höijer43) in about 80 000 Swedish participants agreed with the results of Sellem et al.(Reference Sellem, Flourakis and Jackson42) finding that milk consumption up to 800 g/d (compared with 100 g/d) was not clearly associated with risk of stroke (all types together), but found contrary results for milk and haemorrhagic stroke where an intake of 800 g/d (compared with 100 g/d) had an HR of 1·19 (95 % CI: 1·03, 1·36). No associations were seen for fermented milk. Similarly, another study(Reference Guo, Givens and Heitmann44) also found no associations between total fermented dairy and ACM and CVD but found that high intake of fat-reduced milk was associated with a reduced risk of CVD (HR 0·84, 95 % CI: 0·68, 1·03, P trend = 0·03) and ACM (HR 0·77, 95 % CI: 0·61, 0·97, P trend = 0·004) compared with low intake. An umbrella review(Reference Guo, Zhu and Tian45) of seventy-five meta-analyses reported on the association of food groups and risk of stroke. For ischaemic stroke, the association with high dairy v. low dairy intake was protective (RR 0·79, 95 % CI: 0·78, 0·96) with similar results for haemorrhagic stroke (RR 0·75, 95 % CI: 0·60, 0·94) although these results were related to only one meta-analysis. These findings highlight the importance of not assuming that all dairy products are the same and the importance of meta-analyses to examine the evidence from a range of different studies. There are few studies examining the association of butter consumption with CVD, but a dose–response meta-analysis(Reference Pimpin, Wu and Haskelberg46) indicated no significant association between butter consumption and ACM, CVD, CHD or stroke. However this meta-analysis involved data from only a few studies (CVD n 4; CHD n 3; stroke n 3) and clearly further research on butter is required including a better understanding of the butter SFA-mediated increased HDL functionality(Reference Brassard, Tessier-Grenier and Allaire47) discussed later.
Overall, the findings from prospective cohort studies provide no consistent evidence of an increased risk of CVD/CHD with increased consumption of dairy foods, despite most of these foods often being the greatest dietary source of SFA intake. To many, this is counterintuitive being contrary to the believed well-established link between SFA intake, serum LDL-C and CVD. However there are emerging factors in dairy foods that may reduce the risks of CVD, some are independent of blood lipid changes and others that moderate the effect of blood lipids. Notably, a recent theoretical health risk analysis(Reference Cohen, Bylsma and Movva22) indicated that in the United States, 850 000 disability-adjusted life years (or 5·0 % estimated years of healthy life lost) due to CVD could be prevented by increased dairy food consumption. Equivalent disability-adjusted life years that may be prevented for stroke and hypertension were approximately 210 000 (6·0 %) and 74 000 (5·5 %), respectively(Reference Cohen, Bylsma and Movva22).
Effects on blood pressure and haemodynamics
In the UK approximately 30 % adults are estimated to be hypertensive(48) and hypertension is the largest single risk factor for CVD development, and stroke in particular(49). It is also the third biggest risk factor for premature death after smoking and poor diet. Gene polymorphisms, nutrition, the environment and interactions between these factors contribute to the development of hypertension as does advancing age and associated increased stiffness of the large arteries.
Engberink et al.(Reference Engberink, Geleijnse and de Jong50) reported an inverse association between low-fat dairy intake and risk of hypertension in older adults; others have shown that both low- and high-fat milk products lead to hypotensive effects(Reference Ralston, Lee and Truby51). Also, results from the Caerphilly prospective study showed that when compared with non-milk drinkers, men who consumed >586 ml milk daily had on average a 10·4 mm Hg lower systolic blood pressure (SBP) after a 22·8 year follow-up(Reference Livingstone, Lovegrove and Cockcroft52). More recently the MEPHASOUS study(Reference Mansouri, Pahlavani and Sharifi53) involving 67 011 university students showed that the risk of hypertension (classified as ≥140/90 mm Hg) in highest dairy consumers was significantly and substantially reduced compared with low consumers (OR 0·15, 95 % CI: 0·13, 0·18, model 4 with extensive confounders). This effect was seen in males, females, in those with normal bodyweight and those overweight or obese and despite only 6·9 % students involved being classified as hypertensive. Dairy foods included milk, cheese and yoghurt although data on individual foods were not collected. The authors suggested that students often have different dietary behaviours than others and perhaps dairy replaced some sodium-rich foods/snacks. There is limited information on the effects of different dairy products on blood pressure (BP) perhaps especially normal v. fat-reduced types. Schmidt et al.(Reference Schmidt, Cromer and Burhans54) reported on a parallel design randomised controlled trial (RCT) which compared the effects of diets with low dairy, full-fat dairy and low-fat dairy in subjects with the metabolic syndrome. The results showed no effect on diastolic blood pressure, but a significant effect on SBP (P = 0·048), with a trend for a decrease in the low-fat dairy treatment (−1·6(sd 8·6) mm Hg) compared with the limited-dairy diet (+2·5(sd 8·2) mm Hg). The mean change in SBP for the full-fat dairy (−5·4(sd 16·1) mm Hg) was not significantly different to the other two treatments indicating no adverse effect of full-fat dairy on BP.
The main milk protein types, whey protein and casein have been shown to have valuable hypotensive effects(Reference Kris-Etherton, Grieger and Hilpert55), particularly on hypertensive subjects. An 8-week RCT in mildly hypertensive volunteers(Reference Fekete, Giromini and Chatzidiakou56) showed that whey protein isolate (2× 28 g/d, mixed with water) had a greater hypotensive effect on 24 h ambulatory SBP (−2·0(sem 0·7) mm Hg) and diastolic blood pressure (−2·9(sem 1·1) mm Hg) than casein and the effects were seen with central and peripheral SBP. The hypotensive effect of whey protein was also seen in an acute postprandial study(Reference Fekete, Giromini and Chatzidiakou57). A number of mechanisms involved in the hypotensive effect of milk and its components have been suggested(Reference Fekete, Givens and Lovegrove58). Peptides released during digestion of casein and whey proteins are known to have hypotensive effects by inhibiting the function of the angiotensin-I-converting enzyme leading to reduced production of the vasoconstricting angiotensin-II(Reference FitzGerald and Meisel59) which reduces its stimulation of the vasoconstrictive peptide endothelin-1. It was subsequently shown(Reference Maes, Van Camp and Vermeirssen60) that the Ala-Leu-Pro-Met-His-Ile-Arg peptide from β-lactoglobulin (a whey protein) was able to substantially reduce the release of endothelin-1 by pig endothelial cells. As noted earlier(Reference Schmidt, Cromer and Burhans54) there is little evidence for differential effects of low- v. high-fat dairy foods on hypertension. A recent review(Reference Price, Jackson and Lovegrove61) highlighted some inconsistencies between studies, such that some show little effect of whey protein on BP although it seems that factors including baseline BP, weight loss and obesity during the study period may influence the effect on BP(Reference Price, Jackson and Lovegrove61).
It is now understood that arterial stiffness, especially of the large vessels, is an important arterial phenotype and an excellent predictor of future CVD events(Reference Oh62). It is also an independent predictor of hypertension which it tends to precede and is known to be affected by diet(Reference Kesse-Guyot, Vergnaud and Fezeu63). The measurement of carotid to femoral pulse wave velocity is regarded as the gold standard for estimating arterial stiffness and can directly predict CVD events(Reference Vlachopoulos, Aznaouridis and Stefanadis64). Livingstone et al.(Reference Livingstone, Lovegrove and Cockcroft52), using data from the Caerphilly prospective study following a 22·8-year follow-up, showed for the first time in a longitudinal study that dairy product consumption (not including butter) was associated with a reduced augmentation index, another valuable indicator of arterial stiffness, by 1·9 % units lower (P = 0·021) in men with the highest dairy consumption relative to the lowest consumers. A cross-sectional study also reported that consumption of dairy foods was negatively associated with pulse wave velocity(Reference Crichton, Elias and Dore65). The cross-sectional study by Ribeiro et al.(Reference Ribeiro, Mill and Cade66) showed that consumption of total dairy foods was also inversely associated with carotid to femoral pulse wave velocity (−0·13 m/s) and pulse pressure (−1·3 mm Hg) with similar outcomes from consumption of low-fat dairy, fermented dairy and cheese. A systematic review and meta-analysis of cross-sectional studies(Reference Diez-Fernández, Álvarez-Bueno and Martínez-Vizcaíno67) examined the effects of dairy, cheese and milk on arterial stiffness also showed that dairy foods do not have detrimental effects on pulse wave velocity. It has also been confirmed that SFA do not impair arterial stiffness in healthy subjects(Reference Sanders, Lewis and Goff68).
The effects of dairy foods on BP, arterial stiffness and other aspects of haemodynamics are important in the overall understanding of dairy consumption and its association with CVD risk.
Effects of dairy food matrix on blood lipids
Historically the understanding of the relationship between foods/diets and consumer health has been based on separate assessments of the supply of energy and individual nutrients including protein, fat, carbohydrates and micronutrients. This so-called reductionist approach has given rise to incorrect concept of ‘good’ and ‘bad’ nutrients with the same for the foods they provide. Rose(Reference Rose, Bock and Goode69) suggested that this reductionist approach may explain why some research can lead to ‘spurious localisation’ and ‘misplaced causality’. There is increasing evidence that the interactions between micro- and macrostructures of foods and their nutrients need to be taken into account as they can affect key functions such as digestion and absorption. This is the concept of the so-called food matrix. The dairy food matrix was extensively examined in 2017 by a working group organised by the Universities of Copenhagen and Reading and its findings were published(Reference Thorning, Bertram and Bonjour70). Various aspects of dairy matrix effects have been updated in more recent publications(Reference Feeney, McKinley and Givens71–Reference Feeney, Lamichhane and Sheehan74).
Feeney and McKinley(Reference Feeney, McKinley and Givens71) described a range of issues which link the dairy food matrix to aspects of health including CVD and bone health. To date, the best example of dairy food matrix effect is the now well-documented differential effect of hard cheese and butter when providing the same quantity of fat and SFA yet give very different blood lipid responses(Reference Hjerpsted, Leedo and Tholstrup75,Reference Vissers, Rijksen and Boer76) . Essentially, butter leads to a predictable rise in serum total cholesterol (TC) and LDL-C, whilst the cheese generates little, none or negative LDL-C responses.
A striking example of these effects is given in the RCT of Feeney et al.(Reference Feeney, Barron and Dible77). This was a 6-week randomised parallel design intervention with a daily intake of 40 g dairy fat within three food matrices all matched for energy, protein, fat, lactose and Ca. The matrices comprised (daily) of 120 g full-fat Irish Cheddar cheese (T1), 120 g reduced-fat Irish Cheddar cheese plus 21 g butter (T2) and 49 g butter plus 30 g calcium caseinate powder and a Ca supplement of 500 mg calcium carbonate (CaCO3) (T3). The key results for the responses in blood lipids are given in Table 2 and clearly show the impact that the constructed matrix made to the cholesterol responses. Interestingly, these were all in a downward direction but the greater effect on the full-fat cheese was clear.
Table 2. Changes in blood lipids from baseline to end of 6-week intervention when 40 g/d dairy fat were provided in three types of dairy products
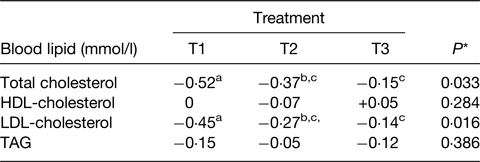
Adapted from(Reference Feeney, Barron and Dible77).
T1, 120 g Irish Cheddar cheese; T2, 120 g low-fat Irish Cheddar cheese + 21 g butter; T3, 49 g butter + 30 g calcium caseinate + 500 mg calcium (as calcium carbonate (CaCO3)) all daily.
* Overall treatment effect.
a,b,cDifferent superscripts represent significant differences between treatments, P < 0·05.
A number of mechanisms have been proposed as contributing to the possible health benefits of the hard cheese matrix but there is good evidence of cheese Ca-fatty acid soaps being synthesised in the digestive tract giving rise to increased faecal fat excretion and hence reduced fat absorbed and response in serum TC and LDL-C(Reference Lorenzen and Astrup78) and is shown in Table 3. Lorenzen and Astrup(Reference Lorenzen and Astrup78) also showed that the high dairy Ca diets also increased faecal bile acid excretion indicating reduced bile acid reabsorption in the ileum and hence less recycling back to the liver. This causes the liver to increase bile acid synthesis from cholesterol leading to a reduction in circulating cholesterol. The synthesis of Ca soaps in the digestive tract following milk consumption was shown a long time ago(Reference Bosworth, Bowditch and Giblin79) and more recently confirmed in a series of in vitro studies(Reference Lamothe, Rémillard and Tremblay80). This also showed that whilst more soaps were created as the Ca:lipid ratio increased, at equal Ca:lipid ratios those dairy products with fairly solid structure (cheese) synthesised more soaps than milk or yoghurt with a more fluid structure. In addition there was evidence that cheese made from homogenised milk produced more soaps than from non-homogenised milk, which may be related to greater NEFA release from the homogenised milk products(Reference Lamothe, Rémillard and Tremblay80). This study, albeit with an in vitro digestion model, does increase the understanding of the Ca-fatty acid saponification process and how this may be influenced by different dairy foods.
Table 3. Faecal excretions resulting from the four dairy dietary treatments

Derived from(Reference Lorenzen and Astrup78).
LC, low calcium, HC, high calcium, HF, high fat, LF, low fat; se, standard error.
* Based on two-way ANOVA.
Relatively recent findings comparing the dairy food matrix of butter and cheese on the differential effects of SFA from butter and cheese showed that the cholesterol efflux capacity (CEC) of HDL was improved in the butter treatment(Reference Brassard, Tessier-Grenier and Allaire47). This is also discussed later. Clearly, the dairy matrix in its various forms needs a more full understanding including further information on specific dairy foods, methods of cheese making, etc.
Saturated fat intake and risk of type 2 diabetes and CVD and events
As noted earlier, dairy foods are often the greatest dietary source of SFA in Western diets which has resulted in dietary guidelines recommending a restriction on dairy food consumption and/or recommending reduced fat dairy foods despite the evidence reviewed earlier. The reasoning for recommending reduced dairy fat consumption relates to the long-held view that a high intake of SFA is a critical risk factor for CVD, mainly on the basis that SFA lead to increased blood TC and LDL-C which in turn results in the development of atherosclerosis. This apparently simple relationship is becoming increasingly questioned(Reference Nestel and Mori39,Reference Astrup, Bertram and Bonjour81–Reference Astrup, Teicholz and Magkos83) with recommendations that dietary guidelines should be food-based(Reference Astrup, Magkos and Bier82). In the UK it is recommended that SFA intake should be no greater than 10 % total energy intake(84) although the UK National Diet and Nutrition Survey(85) clearly shows that on average, the population considerably exceeds the target. The US recommendations(86) also recommend no greater than 10 % but the American Heart Association and American College of Cardiology Guidelines to Reduce Cardiovascular Risk have proposed reducing SFA intake to 5–6 % total energy intake to moderate circulating LDL-C(Reference Eckel, Jakicic and Ard87).
Saturated fat intake and the risk of type 2 diabetes
The possible effect of diet, and fat/fat type in particular, on T2D risk has been the subject of much interest and research over the past 25 years. Early work(Reference Vessby88) created much interest as it showed that replacing SFA with MUFA significantly improved insulin sensitivity, although this was only the case for subjects with a fat intake of <37 % energy intake. A subsequent review of RCT and cohort studies(Reference Riccardi, Giacco and Rivellese89) showed that in five out of seven RCT, replacing SFA with MUFA or PUFA had no significant effect on insulin sensitivity although the studies were with small groups of volunteers, for short periods and involved various methods for measuring insulin sensitivity. In seven longitudinal cohort studies, four indicated that a higher SFA intake was associated with impaired insulin sensitivity although the authors emphasised that this did not prove a cause/effect relationship. Another review of both RCT and observational studies(Reference Risérus, Willett and Hu90) concluded that both types of studies suggest that replacing SFA and trans fatty acids (TFA) with PUFA and/or MUFA improves insulin sensitivity and thus is likely to reduce the risk of T2D. These conclusions were however, not supported by a more recent meta-analysis of observational studies(Reference de Souza, Mente and Maroleanu91) which showed no significant association between SFA and T2D although it did highlight the possible beneficial effect of ruminant TFA. The RCT by Imamura et al.(Reference Imamura, Micha and Wu92) reported that replacing SFA or carbohydrate with PUFA or MUFA improved glucose-insulin homoeostasis particularly in blinded studies. Overall, the effect of PUFA gave the most consistent effect giving improved glycaemic control, insulin resistance and insulin secretion capacity whilst another study concluded there were too few studies on α-linolenic acid to make firm conclusions(Reference Jovanovski, Li and Thanh93) although the meta-analysis of RCT by Gao et al.(Reference Gao, Geng and Huang94) indicated that short-term fish oil supplements were associated with improved insulin sensitivity in subjects with metabolic disorders, a conclusion supported by a review(Reference Rice Bradley95).
More recent data on any association of SFA with T2D risk were provided by the Netherlands cohort of the EPIC study(Reference Liu, van der Schouw and Soedamah-Muthu96) and key results are summarised in Table 4. The key conclusion was that total SFA did not relate to T2D risk as was also the case for 16 : 0 and 18 : 0 which represent the greatest amount of dietary SFA. The 12 : 0 and 14 : 0 SFA were associated with a small reduction of T2D risk but the sum of 15 : 0 and 17 : 0 was associated with a somewhat greater risk reduction. It was also noted that association may be dependent on the type of food providing the SFA with cheese-derived SFA being associated with a reduced T2D risk whilst milk and milk products were neutral (Table 4). This study(Reference Liu, van der Schouw and Soedamah-Muthu96) also showed that substituting SFA with unspecified animal protein (HR per 1 % energy 1·12, 95 % CI: 1·07, 1·17) or carbohydrates (HR per 1 % energy 1·05, 95 % CI: 1·02, 1·08) was associated with a significantly higher risk of T2D. Interestingly, substituting SFA with vegetable protein (HR per 1 % energy 1·15, 95 % CI: 1·03, 1·28) or PUFA (HR per 1 % energy 1·15, 95 % CI: 1·04, 1·27) was also linked to an increased risk of T2D risk although MUFA were neutral. In contrast, the systematic review and meta-analysis by Neuenschwander et al.(Reference Neuenschwander, Barbaresko and Pischke97) found that a high intake of vegetable fat (unspecified types) was associated with a lower T2D risk (summary RR 0·81, 95 % CI: 0·76, 0·88, non-linear response P = 0·028) as was SFA intakes greater than about 17 g/d (summary RR 0·95, 95 % CI: 0·90, 1·00, non-linear response P = 0·023).
Table 4. Multivariable hazard ratios (HR) with 95 % confidence intervals for the associations between the intakes of total SFA, individual SFA and food sources of SFA with incidence of type 2 diabetes in EPIC Netherlands study(Reference Liu, van der Schouw and Soedamah-Muthu96)
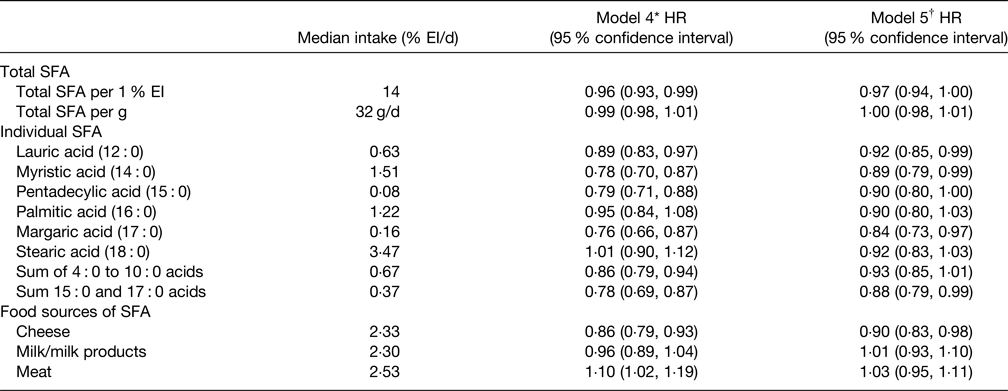
EI, energy intake.
* Adjustments for total energy intake, sex, age, education level, energy-adjusted alcohol consumption level, smoking status, physical activity level, energy-adjusted intake of animal protein, vegetable protein, trans fatty acids, vitamin E, dietary fibre and cholesterol.
† Additional adjustments for BMI and waist circumference.
Sobczak et al.(Reference Sobczak, Blindauer and Stewart98) highlighted that ‘although there is a persistent notion that SFA should be avoided’ the evidence for an association of SFA with T2D is not clear and this may, at least in part, be due to a varying influence of the food supplying the SFA. They also confirmed that T2D is generally associated with higher plasma concentrations of NEFA which is also related to an increased risk of CVD(Reference Sobczak, Blindauer and Stewart98). Details of the mechanisms involved in this NEFA increase are not fully understood but Sobczak et al.(Reference Sobczak, Blindauer and Stewart98) suggest that dietary fats and changes to lipid metabolism are likely to be involved but confirm that circulating 12 : 0, 16 : 0 and 18 : 0, whilst firmly associated with T2D risk, are not necessarily a result of dietary intake. They suggest that the increased plasma SFA in obesity and T2D may be primarily the result of de novo lipogenesis (DNL) from excess carbohydrate. They conclude that since T2D is often linked to dyslipidaemia, including the increased plasma NEFA, emphasis should be given to how these changes can be reversed in the treatment of T2D including the role of lipid lowering drugs(Reference Sobczak, Blindauer and Stewart98).
A recent study investigated the cross-sectional correlation between three dietary patterns and measures of adiposity (a key risk factor for T2D), namely BMI and waist circumference, in 1280 adults from seven European countries in the Food4Me study(Reference Livingstone, Brayner and Celis-Morales99). They also looked at any interaction effects of the obesity-associated FTO gene. They concluded that a dietary pattern high in SFA and discretionary foods and drinks, and low in fibre, was associated with an increased risk of overall and central obesity. It seems possible that diets high in SFA and discretionary foods would also be high in total fat and the higher energy density was responsible for the increased adiposity rather than a specific functional effect of SFA. They also showed that whilst the FTO genotype was linked with an increased risk of obesity, there was no genotype × dietary pattern interaction(Reference Livingstone, Brayner and Celis-Morales99).
Overall, the association of dietary SFA with T2D risk is mixed, although with some tendency for replacing SFA with MUFA/PUFA being associated with improved insulin sensitivity(Reference Liu, van der Schouw and Soedamah-Muthu96,Reference Sobczak, Blindauer and Stewart98) . In addition, it may be that the risk is influenced by the food providing the SFA as suggested by the generally beneficial association between dairy foods and T2D risk noted earlier. It does also seem that more detail is needed on the role of the DNS/carbohydrate pathway in reducing the risk and treatment of T2D.
Saturated fat intake and the risk of CVD
Evidence from prospective cohort studies
The meta-analysis of twenty-one prospective cohort studies involving 347 747 subjects reported that SFA were not associated with an increased risk of CHD (RR 1·07, 95 % CI: 0·96, 1·19), CVD (RR 1·00, 95 % CI: 0·89, 1·11) or stroke (RR 0·81, 95 % CI: 0·62, 1·05)(Reference Siri-Tarino, Sun and Hu100). The authors commented that their meta-analysis identified that there was still insufficient evidence from prospective studies to conclude that dietary SFA is associated with an increased risk of CHD, stroke or overall CVD. The meta-analysis by Chowdhury et al.(Reference Chowdhury, Warnakula and Kunutsor101) involved thirty-two observational studies with fatty acid intakes and seventeen observational studies with circulating fatty acids. The RR for CHD comparing the highest v. lowest SFA intake was neutral (RR 1·03, 95 % CI: 0·98, 1·07). A similar neutrality was observed for MUFA and n-6 PUFA whilst long-chain n-3 PUFA were associated with a reduced risk (RR 0·87, 95 % CI: 0·78, 0·97)(Reference Siri-Tarino, Sun and Hu100). A meta-analysis of twelve prospective cohorts also raised doubts about the validity of SFA being linked with increased CVD risk(Reference de Souza, Mente and Maroleanu91). They found no association between SFA intake and ACM, CVD mortality or ischaemic stroke but perhaps strangely, reported ‘no convincing lack of association’ between SFA and CHD mortality (RR 1·15, 95 % CI: 0·97, 1·36, P = 0·10).
The primary findings of the PURE study(Reference Dehghan, Mente and Rangarajan40) in relation to SFA intake are summarised in Table 5 and indicate that increasing intake of SFA was associated with a significantly reduced risk of total mortality, stroke and non-CVD mortality but had no association with major CVD events, MI or CVD mortality. Increased intakes of MUFA and PUFA were also associated with a reduced risk of total mortality although carbohydrate intake was associated with an increased risk of ACM but not risk of CVD or CVD mortality. The relationships of SFA intake and blood lipids in the PURE study showed that whilst increased SFA intake raised serum TC and LDL-C it also increased HDL-cholesterol (HDL-C) leading to a lower TC:HDL-C ratio, both associated with a reduced CVD risk in support of the observed reduction in ACM and stroke(Reference Mente, Dehghan and Rangarajan102). In addition, serum TAG declined with increasing SFA intake also associated with a lower CVD risk. The key implication of these findings is that the use of either TC or LDL-C as CVD risk markers would lead to an incorrect prediction of CVD-related risk(Reference Astrup, Bertram and Bonjour81). It should be noted that dietary evidence was only obtained at baseline, and overall average total SFA intake was low which may limit widespread applicability.
Table 5. Association between percentage energy intake from SFA and clinical outcomes in the PURE study(Reference Dehghan, Mente and Rangarajan40)
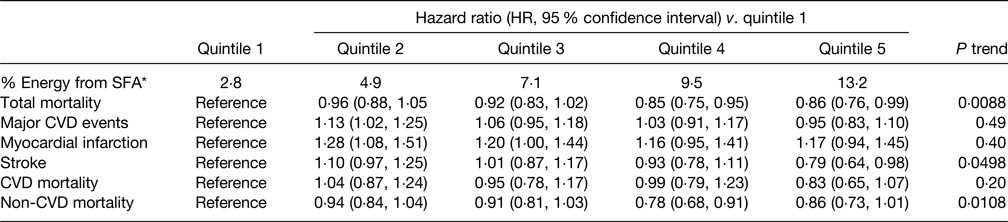
* Median of each quintile.
More recently an umbrella study of nine meta-analyses of prospective studies (a total of 147 studies with about 3·1 million subjects) was published(Reference Heileson103). All but two of the meta-analyses showed that SFA intake was not associated with CHD, whilst two found that replacing SFA with PUFA was associated with a reduced risk of CHD although the overall conclusion from the study was that the meta-analysis of observational studies found no association between SFA intake and CHD(Reference Heileson103).
Mazidi et al. studied the associations between quartiles of total fat, SFA, MUFA and PUFA consumption on the risks for ACM, CHD and stroke-related mortality in 24 144 subjects in the National Health and Nutrition Examination Surveys 1999–2010(Reference Mazidi, Mikhailidis and Sattar104). In addition, they included data from their own study leading to a meta-analysis based on studies published by November 2018. Based on fully adjusted Cox-proportional hazard models in the National Health and Nutrition Examination Surveys prospective study, there were inverse associations between intake (quartile 4 v. quartile 1) of total fat (HR, 0·90, 95 % CI: 0·82, 0·99) and PUFA (HR 0·81, 95 % CI: 0·78, 0·84) with ACM, whilst SFA intake were associated with slightly increased mortality (HR 1·08, 95 % CI: 1·04, 1·11). These results are from the fully adjusted models (two) which adjusted for serum non-HDL-C and dietary cholesterol although it is not known what, if any, effect these had. The meta-analysis involving twenty-nine prospective cohorts (about 1·2 million subjects) showed an inverse association between intake of total fat (HR 0·89, 95 % CI: 0·82, 0·97), MUFA (HR 0·94, 95 % CI: 0·89, 0·99) and PUFA (HR 0·89, 95 % CI: 0·84, 0·94) with ACM, although SFA showed no significant association (HR 1·05, 95 % CI: 0·99, 1·12). No association was seen for total fat (HR 0·93, 95 % CI: 0·80, 1·08) and SFA (HR 0·93, 95 % CI: 0·80, 1·08) with CVD mortality. Total fat had no association with CHD mortality (HR 1·03, 95 % CI: 0·99, 1·09) whereas SFA had a positive association with CHD mortality (HR 1·10, 95 % CI: 1·01, 1·21). Neither MUFA nor PUFA intakes were associated with CVD or CHD mortality but both had inverse associations with stroke mortality (MUFA HR 0·80, 95 % CI: 0·67, 0·96; PUFA HR 0·84, 95 % CI: 0·80, 0·90). No association was seen for SFA and stroke mortality. As the authors mention, single-nutrient studies are complicated by the fact that changes in one may affect another. As a result it is not known if increased MUFA and PUFA intake replaced SFA or whether all were independent changes which could influence the interpretation. This study does add to the limited data on the beneficial association of MUFA with CVD-related mortality and indicates a limited association of SFA with the range of CVD-related outcomes studied(Reference Mazidi, Mikhailidis and Sattar104).
An additional meta-analysis with nineteen cohort studies (about 1 million subjects) has been reported recently(Reference Kim, Je and Giovannucci105). Overall, this reported a non-linear association between SFA and ACM and CVD mortality with the risk increasing up to an SFA intake of 11 % energy intake followed by a plateau or slightly reducing risk. A sub-group analysis which included adjustments for fat intake and serum lipids did not change the associations. Significant inverse associations with ACM were seen for MUFA and PUFA intakes. A scoping study of forty-four systematic reviews of prospective studies mostly on highest v. lowest exposure to dietary fat/fat types on health outcomes was reported(Reference Schwingshackl, Zähringera and Beyerbach106). Overall, this mainly found no association of total fat, MUFA and/or PUFA and SFA with risk of CVD and CHD, and SFA had no association with ACM and a negative association with risk of stroke. This study(Reference Schwingshackl, Zähringera and Beyerbach106) also included a scoping study of RCT which is discussed in the next section.
The evidence from prospective studies on the association of SFA with mortality and CVD events is mixed although most studies/meta-analyses(Reference Dehghan, Mente and Rangarajan40,Reference de Souza, Mente and Maroleanu91,Reference Siri-Tarino, Sun and Hu100,Reference Chowdhury, Warnakula and Kunutsor101,Reference Heileson103,Reference Mazidi, Mikhailidis and Sattar104,Reference Schwingshackl, Zähringera and Beyerbach106) reported a neutral or negative association. The PURE study(Reference Dehghan, Mente and Rangarajan40,Reference Mente, Dehghan and Rangarajan102) is of particular interest as it also provided data on blood lipids, with increased SFA intake increasing serum TC and LDL-C but also increased in HDL-C leading to a reduction in the TC:HDL-C ratio, in agreement with the observed reduction in ACM and stroke. This study indicates that intake of SFA is not a good predictor of CVD risk and that the use of LDL-C as a risk factor in isolation may also be misleading.
Whilst prospective studies are usually regarded as providing less valuable evidence than from RCT, they do have merits including large subject numbers, long study periods, ability to study foods, not just nutrients and hard clinical outcomes. Generally, they cannot prove cause and effect for which RCT are needed. Nevertheless, as recently highlighted(Reference Astrup, Teicholz and Magkos83,Reference DuBroff and de Lorgeril107) , the evidence that prospective studies provide should be seriously considered when dietary guidelines are set as the simple ‘diet-heart hypothesis is now of uncertain validity’(Reference DuBroff and de Lorgeril107).
Evidence from randomised controlled trials
The Sydney heart study and the Minnesota Coronary Experiment were carried out in the period 1966–1973 but have been reanalysed more recently with more up to date statistical techniques(Reference Ramsden, Zamora and Leelarthaepin108,Reference Ramsden, Zamora and Majchrzak-Hong109) . Both were long-term (Sydney median follow-up 39 months; Minnesota ≥12 months) RCT examining the replacement of dietary SFA with linoleic acid-rich PUFA on CVD events and mortality. The Sydney study(Reference Ramsden, Zamora and Leelarthaepin108) reported the PUFA intervention group to have significantly higher ACM rates (HR 1·31, 95 % CI: 1·06, 1·58) than the SFA-rich control (HR 0·70, 95 % CI: 0·53, 0·91) despite the PUFA treatment leading to a greater reduction in blood TC. Similarly, the Minnesota study(Reference Ramsden, Zamora and Majchrzak-Hong109) showed that the PUFA treatment led to significant reductions in TC but this did not lead to a reduction in mortality relative to the SFA-rich control.
Broadly, these two RCT appeared to contradict the traditional diet-heart hypothesis such that whilst SFA replacement led to reductions in TC (and presumably LDL-C which was not reported), these did not lead to reduced mortality. There are however substantial concerns about the value of these studies, including whether the subjects (70 % smokers in Sydney), the settings (psychiatric hospitals and nursing homes) and the diets used are relevant to contemporary populations. Also, a major concern is the fact that both studies used margarines and related products, which in the period of the studies, were rich sources of industrial TFA which were not measured but could have influenced the findings considerably.
The evidence from ten meta-analyses published from 2010 to 2017 was included in a narrative umbrella review of RCT(Reference Heileson103). No evidence was seen of a significant increase in CHD mortality or total mortality related to SFA intake, although three analyses showed reductions in CHD/CVD disease events linked with replacing SFA with PUFA. Heileson(Reference Heileson103) also reviewed the strengths and weaknesses of this set of analyses and identified high between-study heterogeneity and highlighted that some key confounding variables such as changes in TFA and n-3 fatty acids and potential bias from lack of blinding, made collective interpretation of the results difficult. The overall conclusion was that SFA are not independently linked to heart disease, and despite leading to reductions in LDL-C, replacing SFA with PUFA may not be beneficial. This review was also critical of the proposal to replace SFA with PUFA by the Presidential Advisory paper from the American Heart Association(Reference Sacks, Lichtenstein, Wu, Appel, Creager and Kris-Etherton110) as it was felt that the studies used had many design faults and omitted consideration of possible interference by TFA in high-PUFA treatments.
The recent updated Cochrane review and meta-analysis(Reference Hooper, Martin and Jimoh111) assessed the effects of replacing SFA energy intake with carbohydrate, PUFA, MUFA and/or protein on mortality and CVD-related morbidity with data from fifteen RCT (involving sixteen comparisons) that met strict inclusion criteria and totalled almost 60 000 subjects. Interestingly, the Sydney heart study was included but the Minnesota Coronary Experiment was excluded due to its short mean follow-up period. The key results from this meta-analysis were that reductions in dietary SFA had little or no effect on ACM, CVD-related mortality, non-fatal MI and CHD mortality. The findings on total (fatal or non-fatal) MI, stroke and CHD events (fatal or non-fatal) were reported to be unclear due to very low-quality data. The meta-analysis did however find that reducing dietary SFA for at least 2 years led to a significant 21 % reduction in combined CVD events although this was not linked to the risk of CVD-related mortality. Of note is that only three studies were included in the at least 2 years study duration subgroup and there was no significant effect of SFA reduction on CVD events in the up to 24 months, and longer than 48 months subgroups. Overall, no significant differences were found between the replacement of SFA energy with that from PUFA or carbohydrates whilst data on energy replacement by MUFA and protein were extremely limited(Reference Hooper, Martin and Jimoh111).
This meta-analysis also examined the effect of SFA reduction on changes in serum cholesterol. For the studies with suitable data, TC and LDL-C had mean changes of −0·24 (95 % CI: −0·36, −0·13) mmol/l and −0·19 (95 % CI: −0·33, 0·05) mmol/l, respectively, with the greatest reductions seen with replacement by PUFA. Perhaps surprising, only one study with replacement by MUFA was available for TC change and none for LDL-C change. There were no or very limited changes in HDL-C and lipoprotein(a). The observed changes in TC were not related to the primary outcomes of ACM and CVD mortality and TC reduction was only related to CVD events when the reduction was at least 0·2 mmol/l, but this may be important. For secondary outcomes of stroke and non-fatal MI, changes in TC had no significant relationship, but like CVD events, TC reductions of at least 0·2 mmol/l were associated with a lower risk of all MI (fatal and non-fatal) events, but it must be remembered that the evidence quality for MI outcomes was GRADE low and very low.
A scoping study of fifteen RCT showed that isoenergetic replacement of carbohydrate by SFA increased blood TC, LDL-C and HDL-C but reduced TAG(Reference Schwingshackl, Zähringera and Beyerbach106). Replacement of carbohydrate by MUFA and PUFA reduced TC, LDL-C and TAG but increased HDL-C. It was concluded overall, that whilst prospective studies (see previous section) found little or no associations of SFA, MUFA, PUFA with CVD or ACM, the evidence from RCT showed that replacement of SFA with MUFA and/or PUFA generally improved blood lipids and glycaemic control. On this basis it was concluded that current recommendations to replace SFA with MUFA/PUFA should be retained. As discussed earlier and later, reductions in blood lipids, notably LDL-C, do not always lead to the often assumed reduction in CVD risk.
Overall, the findings from RCT broadly agree with the conclusions from the prospective cohort studies that the traditional diet-heart hypothesis may not always be valid.
Are all SFA equal in relation to type 2 diabetes and CVD?
Results from the EPIC-InterAct case-cohort study with 12 132 cases of T2D showed that different plasma phospholipid SFA were independently associated with incident T2D in opposite directions(Reference Forouhi, Koulman and Sharp112). Odd-chain SFA 15 : 0 and 17 : 0 and long-chain SFA 20 : 0, 22 : 0, 23 : 0 and 24 : 0 were inversely associated whilst even-chain 14 : 0, 16 : 0 and 18 : 0 were positively associated. The negative association of plasma phospholipid 15 : 0 and 17 : 0 with T2D risk was seen in women but not in men in the EPIC-Potsdam cohort(Reference Prada, Wittenbecher and Eichelmann113). Both studies highlighted the role of dairy products as exogenous sources of odd-chain SFA, giving support to prospective studies which showed negative associations between dairy consumption and T2D.
Two recent studies(Reference Imamura, Fretts and Marklund114,Reference Murru, Manca and Carta115) highlighted the risks of assuming that plasma and tissue palmitic acid (16 : 0) concentrations are simply a reflection of its dietary intake since some palmitic acid is synthesised by the DNL pathway, primarily from carbohydrate with some from protein and alcohol. Palmitic acid can be elongated to 18 : 0 (stearic acid) and desaturated to palmitoleic acid (16 : 1n-7) and 18 : 0 can be desaturated to 18 : 1n-9 (oleic acid). In healthy subjects rather limited DNL occurs primarily in adipose tissue although certain conditions including insulin resistance and a high dietary intake of carbohydrates, particularly sugars, enhance hepatic DNL substantially, contributing to hyperlipidaemia(Reference Schwarz, Linfoot and Dare116). This is supported by studies showing that replacing dietary SFA with refined carbohydrates is associated with an increased risk of CVD(Reference Siri-Tarino, Chiu and Bergeron117). A pooled analysis of seventeen prospective cohorts showed that all four DNL-related fatty acids were positively associated with incident T2D with RR of the highest v. lowest plasma concentrations being 1·53 (95 % CI: 1·41, 1·66; P < 0·001) for 16 : 0, 1·40 (95 % CI: 1·33, 1·48; P < 0·001) for 16 : 1n-7, 1·14 (95 % CI: 1·05, 1·22; P = 0·001) for 18 : 0 and 1·16 (95 % CI: 1·07, 1·25; P < 0·001) for 18 : 1n-9(Reference Imamura, Fretts and Marklund114). Murru et al.(Reference Murru, Manca and Carta115) concluded that blaming a single nutrient such as 16 : 0, which has recognised physiological actions, and proposing that reducing its dietary intake in order to reduce CVD is too simplistic. They proposed that population guidelines should not be based on single nutrients but consideration of the whole diet. However the role of DNL in the aetiology of T2D would seem to require considerably more investigation.
Others have also highlighted some of the weaknesses associated with simply looking at the effects of total SFA without considering the possible effects of individual SFA. Dawczynski et al.(Reference Dawczynski, Kleber and März118) assessed the meta-analysis of Chowdhury et al.(Reference Chowdhury, Warnakula and Kunutsor101) and showed that two of the eight studies used in relation to the CVD risk associated with SFA included 15 : 0 and 17 : 0 fatty acids in serum phospholipids which are primarily a marker for intake of milk fat. Dairy products in general are known to have neutral or negative associations with CVD risk (Table 1) and it was proposed that these two studies should not have been included in the meta-analysis. Dawczynski et al.(Reference Dawczynski, Kleber and März118) reanalysed the data after the exclusion of these two studies which then produced a positive association of total SFA concentration in blood and coronary events (RR 1·21, 95 % CI: 1·04, 1·40) confirming the importance of not including 15 : 0 and 17 : 0 along with other SFA. As noted earlier these odd-chain SFA have also been shown to have a negative association with T2D suggesting that detailed knowledge of the contributions of specific foods to CVD and T2D risk is important.
SFA–cholesterol–CVD risk relationship
For some considerable time, LDL-C concentration in serum/plasma has been measured to predict the risk of CVD. There is consistent evidence that most SFA increase LDL-C and HDL-C(Reference Mensink, Zock and Kesterm119) and that LDL particles have a causative role in the development of atherosclerotic CVD(Reference Borén, Chapman and Krauss120). There is however increasing uncertainty that lowering LDL-C concentration by reducing dietary SFA intake will inevitably lead to a CVD risk reduction(Reference Dehghan, Mente and Rangarajan40,Reference Mente, Dehghan and Rangarajan102) . There are a number of possible reasons for this.
Is LDL-cholesterol a valid predictor of CVD risk?
The TC:HDL-C ratio has been found to provide a better estimate of CVD risk than LDL-C alone(Reference Quispe, Elshazly and Zhao121) due, at least in part, to its relationship with LDL particle number(Reference Mathews, Mallidi and Kulkarni122). For this reason and as proposed(Reference Quispe, Elshazly and Zhao121) TC:HDL-C should be used to provide additional clinical information to LDL-C in primary prevention especially in high-risk patients. It has also been highlighted that there can be considerable variation in serum LDL-C response to SFA intake between individuals and this may contribute to the uncertainty of the SFA–CVD risk relationship(Reference Griffin, Mensink and Lovegrove123). Griffin et al.(Reference Griffin, Mensink and Lovegrove123) identified a number of metabolic and genetic reasons for this variation and suggested that a blood biomarker of serum LDL-C responsiveness to SFA replacement would improve the estimation of CVD risk. Recently it has also been shown that substantial between-subject responses occurred in TC and LDL-C in subjects who consumed about 40 g dairy fat/d either as butter, cheese or reduced-fat cheese plus butter(Reference O'Connor, Feeney and Bhargava124). They showed that the percentage of change in TC and LDL-C was associated with baseline TC, TAG, body weight and high-sensitivity C-reactive protein. These studies again show an uncertainty in the reliance on LDL-C as a single useful CVD risk predictor.
Role of LDL particle size and density
Another limitation of using LDL-C as a single predictor of CVD risk, relates to the fact that LDL-C contains the cholesterol present in all LDL particles. Yet the small, dense LDL particles, although containing less cholesterol per particle than the large, more buoyant LDL particles, are generally agreed to be more strongly associated with increased atherosclerosis(Reference Arsenault, Lemieux and Despres125,Reference Hoogeveen, Gaubatz and Sun126) . To some extent this has been challenged by the Ludwigshafen risk and cardiovascular health study(Reference Grammer, Kleber and Marz127). This showed for the first time, in 1643 patients referred for coronary angiography and followed up for a median of 9·9 years, that both large and small LDL particles were independently associated with an increased risk of ACM and CVD mortality (small LDL: HR 1·50, 95 % CI: 1·06, 2·12, P = 0·021; large LDL: HR 1·89, 95 % CI: 1·32, 2·70, P < 0·001, both from model 3) compared with particles of intermediate size(Reference Grammer, Kleber and Marz127). Perhaps contributing to the findings, patients with small LDL also had higher concentrations of oxidised LDL and those with buoyant LDL had higher concentrations of the inflammatory markers IL-6 and C-reactive protein than those with intermediate LDL, although the reason for the latter was not known. The significance of this study against the background of evidence confirming the higher risk associated with small-dense LDL(Reference Froyen128) is unclear.
A number of studies have demonstrated that SFA tends to increase the large, buoyant LDL possibly contributing to the reason why some studies(Reference Ramsden, Zamora and Leelarthaepin108,Reference Ramsden, Zamora and Majchrzak-Hong109) have not shown a reduction in risk of CVD and/or mortality when SFA are replaced by PUFA/MUFA. The RCT by Bergeron et al.(Reference Bergeron, Chiu and Williams129) compared diets with high-SFA (about 13–14 % energy intake) with low-SFA diets (about 7–8 % energy intake) which had SFA replaced with MUFA with the differences in SFA content between the high- and low-SFA treatments being achieved mainly by using high-fat dairy foods and butter. There was no significant difference in plasma HDL-C between the two treatments but whilst the high-SFA diet increased plasma LDL-C, this was due entirely to a significant rise in large LDL particles (about 577 v. 542 nmol/l) with no differences in medium, small or very small LDL particles(Reference Bergeron, Chiu and Williams129). This suggests that the effect of SFA from red meat, white meat and dairy (which were used in the diets) on LDL-C could be moderated by the rise in large LDL particles but not small, dense particles. A recent valuable narrative review(Reference Froyen128) examined the evidence from twenty-eight RCT on the effects of fat and fat type on the resulting LDL particle size profile. Overall, it was shown that consuming diets of higher fat and SFA contents led to increased large, buoyant LDL particles and/or a reduction in small LDL particles. A key conclusion was that LDL particle size is primarily affected by high- v. low-fat and high- v. low-SFA diets, although it was emphasised that more work was needed on the effects of individual SFA and the need to consider overall dietary patterns, notably plant-based v. animal-based diets(Reference Froyen128). Nevertheless, these finding may at least partly explain why high-SFA dairy foods are not associated with increased CVD risk, although an RCT involving subjects consuming SFA-reduced, MUFA-enriched (modified) milk, cheese and butter, compared with conventional counterparts, found that whilst the modified foods led to a significantly reduced serum LDL-C response, there were no significant differences in the proportions of small, medium and large LDL particles between dietary treatments(Reference Vasilopoulou, Markey and Kliem130). The impact of the food matrix in various dairy foods on LDL subtypes clearly needs exploration.
In relation to dairy foods, it is of note that a cross-sectional study in 291 healthy Swedish men indicated that dietary fatty acids typically found in dairy foods were associated with less small-dense LDL particles(Reference Sjogren, Rosell and Skoglund-Andersson131). In particular, SFA 4 : 0 to 10 : 0 and 14 : 0 (P < 0·05) in the diet and 15 : 0 and 17 : 0 (markers of dairy fat intake) in serum phospholipids (P < 0·05) were associated with fewer small-dense LDL particles. This study suggested that LDL particle size distribution may be favourably modified by dairy products in the diet, or that other dietary SFA have a detrimental effect and milk SFA attenuate this effect rather than have a positive effect themselves.
Effect of food source of SFA
There is now some evidence that the effect of SFA can depend on the food source. Two prospective studies found that whilst a positive association with CVD risk was seen with SFA from meat, this was not the case for SFA from dairy(Reference Vissers, Rijksen and Boer76,Reference de Oliveira Otto, Mozaffarian and Kromhout132) . A study in a Dutch population with a relatively high intake of SFA from dairy foods showed an inverse association between SFA intake and IHD risk which was primarily driven by SFA from dairy foods (including short- to medium-chain SFA, 14 : 0 and the sum of 15 : 0 and 17 : 0), whilst those from other sources (including meat) were essentially neutral(Reference Praagman, Beulens and Alssema133).
A systematic review and dose–response meta-analysis of 123 prospective studies on the associations between food groups and the risk of CHD, stroke and heart failure was reported by Bechthold et al.(Reference Bechthold, Boeing and Schwedhelm134). This showed that dairy food intake was not associated with risk of CHD (RR 0·99, 95 % CI: 0·92, 1·07), stroke (RR 0·96, 95 % CI: 0·90, 1·01) or heart failure (RR 1·00, 95 % CI: 0·90, 1·10) whilst red, and especially processed meat, were significantly and positively associated with an increased risk of all three disease outcomes. There was little discussion on the relative effects of SFA from the different food groups, but it was stated that the role of SFA in relation to CVD health is ‘controversial’(Reference Bechthold, Boeing and Schwedhelm134).
These observations may be related to food-specific fatty acids or other aspects of the foods including their matrix, but clearly detailed RCT are needed to resolve the reasons for these differential effects.
Functionality of HDL-cholesterol
For the general population there is good evidence that serum HDL-C concentration is inversely associated with CVD and mortality(Reference Di Angelantonio, Sarwar and Perry135) leading to the use of the TC:HDL-C ratio as an improved predictor of CVD risk, based on low HDL-C concentration being associated with increased CVD risk(Reference Sharrett, Ballantyne and Coady136). However it has been reported that men and women with extremely high-serum HDL-C concentrations (>1·9 mmol/l, 95 % CI: 1·4, 2·0; >2·4 mmol/l, 95 % CI: 1·8, 2·5, for men and women, respectively) also have high mortality(Reference Madsen, Varbo and Nordestgaard137) which challenges the use of the TC:HDL-C ratio. Moreover, drug treatments to raise HDL-C have not been associated with reduced ACM, CHD mortality, MI or stroke(Reference Keene, Price and Shun-Shin138). However SFA can increase HDL-C, particularly when they replace carbohydrates(Reference Brassard, Tessier-Grenier and Allaire47), and SFA from butter, but not cheese, have also been shown to increase the CEC of HDL in men but not women, although interestingly, the same study showed that MUFA increased the CEC of HDL in women but not men(Reference Brassard, Tessier-Grenier and Allaire47). This led Griffin and Lovegrove(Reference Griffin and Lovegrove139) to question whether the increased CEC of HDL is in some way a compensation for the increased LDL-C following butter consumption. The study by Brassard et al.(Reference Brassard, Tessier-Grenier and Allaire47) confirmed that the effect of SFA on the LDL-C/HDL-C mediated risk of CVD depends on the food source and possibly the sex of the consumer. However, this and the study by Madsen et al.(Reference Madsen, Varbo and Nordestgaard137) highlight the need for further research to clarify whether HDL-C truly has a CVD protective role.
Overall, despite LDL particles having a causal role for atherosclerotic CVD(Reference Borén, Chapman and Krauss120), there is now evidence that changes in serum LDL-C concentrations alone may not reliably predict future atherosclerotic CVD risk. Whilst the TC:HDL-C ratio may have benefits over LDL-C alone, the facts that the CVD protective effect of HDL-C now seems less certain, the CEC of HDL can vary, SFA tend to increase the less atherogenic large LDL particles and SFA from different foods can have contrasting effects on CVD risk, all cast doubt on the validity of the classical SFA leading to increased LDL-C and hence an increased risk of CVD relationship. This may also explain at least some of reasons that high-SFA dairy foods are not associated with CVD risk. Additional validated risk markers are therefore needed with assessment of vascular endothelial function(Reference Vasilopoulou, Markey and Kliem130) and broader vascular function(Reference Price, Jackson and Lovegrove61) being potentially valuable non-blood lipid targets.
Conclusions
Overall, the prospective studies and RCT included in this review show that SFA from dairy foods are not generally associated with T2D risk, although the consistent inverse association between yoghurt consumption and T2D, together with the increasing prevalence of T2D, means that this is potentially very important. Whilst some mechanisms have been proposed to support this association, more research is needed to improve the evidence which would lead to a better understanding of the type and amount of yoghurt needed for maximum benefit. The association between dairy foods and CVD is generally neutral despite many of the dairy foods being the major source of SFA in many diets. This leads to substantial doubt concerning the validity of the traditional diet-heart hypothesis which positively relates increased SFA intake to increased serum cholesterol and subsequent increased CVD. Studies that included serum cholesterol measurements broadly agree with the positive relationship between SFA intake and LDL-C concentration, but without a consistent subsequent relationship with increased CVD risk, despite LDL being a causal factor in atherosclerotic CVD. There is now some emerging evidence to explain this which is highly relevant to dairy foods. These include the potentially counterbalancing effect of any SFA-stimulated HDL-C, food matrix factors, notably in hard cheese, which reduce fat absorption and moderate increased LDL-C. Other evidence includes SFA being associated with the less atherogenic large buoyant LDL particles and possible counterbalancing hypotensive action of dairy proteins.
Many parts of this emerging evidence are not fully understood or confirmed in adequately powered studies but do point to a need for a more food-based dietary recommendations and a number of respected groups have provided convincing arguments for this and not by simple limits on SFA intake(Reference Astrup, Magkos and Bier82,Reference Krauss and Kris-Etherton140) . Direct or consequential restrictions on dairy food consumption do not seem warranted but there is clear need to better understand the association of the different dairy food types with chronic diseases, particularly for T2D.
Acknowledgements
This review is based primarily on an invited presentation at the UK and Ireland Nutrition Society annual conference in July 2022. I am grateful to the Nutrition Society and members for the invitation and their support for the present paper.
Financial Support
Whilst there was no specific funding for the writing of this review, the author is supported by the University of Reading.
Conflict of Interest
The author has received travel expenses and honoraria in connection with lectures and meetings from the Dairy Council (now Dairy UK), Dutch Dairy Association, European Dairy Association and the International Dairy Federation. He has also been a consultant to the Estonian Bio-competence Centre of Healthy Dairy Products (BioCC), and to the Dairy Council on fats in dairy products and cardiometabolic diseases.
Authorship
The author had sole responsibility for preparation of the present paper.