INTRODUCTION
Aging is often accompanied by decline in aspects of cognitive functioning, in particular memory and executive function (EF) (Carlson, Xue, Zhou, & Fried, Reference Carlson, Xue, Zhou and Fried2009), and by atrophy in cortical and subcortical brain regions key to these cognitive functions (Bugg & Head, Reference Bugg and Head2011; Raz et al., Reference Raz, Lindenberger, Rodrigue, Kennedy, Head, Williamson and Acker2005; Salat et al., Reference Salat, Tuch, Greve, van der Kouwe, Hevelone, Zaleta and Dale2005; Walhovd et al., Reference Walhovd, Fjell, Reinvang, Lundervold, Dale, Eilertsen and Fischl2005). As such, there is great interest in strategies to slow down cognitive decline and brain atrophy. One area of interest is physical exercise (Hillman, Erickson, & Kramer, Reference Hillman, Erickson and Kramer2008), especially aerobic training (AT; e.g., walking). AT has been shown to improve cognitive functioning (Colcombe & Kramer, Reference Colcombe and Kramer2003; Kramer et al., Reference Kramer, Hahn, Cohen, Banich, McAuley, Harrison and Colcombe1999), to reduce atrophy in the hippocampus (Erickson et al., Reference Erickson, Voss, Prakash, Basak, Szabo, Chaddock and Kramer2011; Ten Brinke et al., Reference Ten Brinke, Bolandzadeh, Nagamatsu, Hsu, Davis, Miran-Khan and Liu-Ambrose2015), and to reduce atrophy of white matter (WM) and cortical gray matter (GM) (Colcombe et al., Reference Colcombe, Erickson, Scalf, Kim, Prakash, McAuley and Kramer2006; Voss, Heo, et al., Reference Voss, Heo, Prakash, Erickson, Alves, Chaddock and Kramer2013).
In recent years, there has been growing interest in resistance training (RT; e.g., lifting weights) as a tool to improve cognition and prevent brain volume loss in the elderly (Cassilhas et al., Reference Cassilhas, Viana, Grassmann, Santos, Santos, Tufik and Mello2007; Liu-Ambrose et al., Reference Liu-Ambrose, Nagamatsu, Graf, Beattie, Ashe and Handy2010; Liu-Ambrose, Nagamatsu, Voss, Khan, & Handy, Reference Liu-Ambrose, Nagamatsu, Voss, Khan and Handy2012; Nagamatsu, Handy, Hsu, Voss, & Liu-Ambrose, Reference Nagamatsu, Handy, Hsu, Voss and Liu-Ambrose2012). RT has been shown to increase insulin-like growth factor-1 (IGF-1) in the serum of older adults (Cassilhas et al., Reference Cassilhas, Viana, Grassmann, Santos, Santos, Tufik and Mello2007; Parkhouse, Coupland, Li, & Vanderhoek, Reference Parkhouse, Coupland, Li and Vanderhoek2000; Vale et al., Reference Vale, de Oliveira, Pernambuco, de Meneses, Novaes Jda. and de Andrade Ade2009). IGF-1 mediates the effects of RT on increased muscle mass (Adams & Haddad, Reference Adams and Haddad1996) but also passes through the blood brain barrier (Carro, Nunez, Busiguina, & Torres-Aleman, Reference Carro, Nunez, Busiguina and Torres-Aleman2000; Cotman, Berchtold, & Christie, Reference Cotman, Berchtold and Christie2007). In addition to promoting neurogenesis in the hippocampus (Trejo, Carro, & Torres-Aleman, Reference Trejo, Carro and Torres-Aleman2001), IGF-1 promotes brain myelination via oligodendrocyte development (O’Kusky & Ye, Reference O’Kusky and Ye2012).
We previously conducted a single-blind, randomized controlled trial (RCT) of 155 community-dwelling older women to test the effects of 52 weeks of once- or twice-weekly RT in comparison to an active control (twice-weekly balance-and-tone training [BAT]) on cognitive functioning (Liu-Ambrose et al., Reference Liu-Ambrose, Nagamatsu, Graf, Beattie, Ashe and Handy2010). The rationale for the study design was to determine the minimum frequency of RT necessary to promote cognition in this population. We have shown that both frequencies of RT improves Stroop performance (Stroop, Reference Stroop1935)—a measure of EF—immediately following the intervention (Liu-Ambrose et al., Reference Liu-Ambrose, Nagamatsu, Graf, Beattie, Ashe and Handy2010) and that this effect is sustained at 2-year follow-up (Davis et al., Reference Davis, Marra, Beattie, Robertson, Najafzadeh, Graf and Liu-Ambrose2010). From a subset of participants who underwent structural magnetic resonance imaging (MRI) scans, we also reported that whole brain volume was reduced following the intervention in both the once- and twice-weekly RT groups relative to BAT (Liu-Ambrose et al., Reference Liu-Ambrose, Nagamatsu, Graf, Beattie, Ashe and Handy2010).
In the current study, we conducted a secondary analysis of data from this RCT and provide additional data not reported previously to address outstanding questions. First, what is the long-term impact of RT on EF and on verbal memory? We were interested in studying both EF and memory in light of previous studies showing that AT might impact both domains, as well as the neural correlates of each. We examined verbal memory, in particular, because of the greater ease of assessment in comparison to visuo-spatial memory (which generally requires a computer-based assessment). We used a latent variable approach to address this question, which uses multiple measures of a given construct (e.g., EF or memory) to create latent variables; these latent variables reflect the common processes shared across the cognitive tasks. The advantage of this approach is to reduce task-specific variation and measurement error (Salthouse, Reference Salthouse2011), and to minimize the number of statistical tests undertaken. Second, what is the long-term impact of RT on cortical brain volume and on hippocampal volume? In addressing this question, we sought to determine whether the RT-induced whole-brain volume reduction noted previously (Liu-Ambrose et al., Reference Liu-Ambrose, Nagamatsu, Graf, Beattie, Ashe and Handy2010) applied specifically to cortical volume and to the hippocampus and whether these effects persisted at the 2-year follow-up. Because RT might have effects on WM and GM, we analyzed cortical GM and WM separately. Importantly, both cortical GM and WM volumes show age-related reductions (Bugg & Head, Reference Bugg and Head2011; Walhovd et al., Reference Walhovd, Fjell, Reinvang, Lundervold, Dale, Eilertsen and Fischl2005) and are reduced in persons with dementia compared to their healthy peers (Salat et al., Reference Salat, Greve, Pacheco, Quinn, Helmer, Buckner and Fischl2009; Thompson et al., Reference Thompson, Hayashi, De Zubicaray, Janke, Rose, Semple and Doddrell2003). Third, are concurrent changes in cognition and brain volume correlated, and are changes in brain and cognition correlated with concurrent changes in muscle power? Addressing this third set of questions will help determine the degree to which the effects of RT are interdependent and whether these effects can be attributed to improved muscle strength.
METHODS
The 52-week exercise intervention occurred from May 2007 to April 2008 and in April 2009, participants were invited back for a 2-year follow-up assessment. A CONSORT is reported in Figure 1, which shows the available data at each time point. Ethical approval was obtained from the Vancouver Coastal Health Research Institute and the University of British Columbia’s Clinical Research Ethics Board. The RCT was registered at clinicaltrials.gov (NCT00426881). Assessors were blinded to the participants’ exercise training assignment.
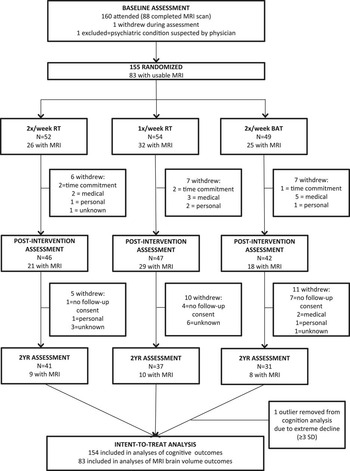
Fig. 1 CONSORT diagram for the study showing completion of cognitive outcomes and MRI scans (subset).
Participants
One hundred fifty-five women aged 65–75 years, who lived in Vancouver, British Columbia, were randomized to one of the three exercise interventions. Of the 88 women who underwent baseline MRI scans, data from 83 were of sufficient quality to be included in the analyses below. All participants lived independently in the community, had intact cognitive functioning and acceptable visual acuity with or without corrective lenses, and spoke fluent English. Interested individuals were excluded for the following reasons: (1) presence of a medical condition contraindicating physical exercise; (2) participation in resistance training in the past 6 months; (3) evidence of neurological disease, stroke, or depression; (4) receiving estrogen or testosterone therapy. All participants provided written informed consent.
Exercise Interventions
RT and BAT classes were led by certified fitness instructors at a local YMCA and the Center for Hip Health and Mobility research center. Fitness instructors tracked training records, and a research assistant, who was not involved in delivering the program, determined treatment fidelity using a standard form. Each class was 60 min long, including a 10-min warm-up and 10-min cool-down. The RT program entailed a progressive, high intensity protocol using Keiser pressurized air system and free weights. Non-machine exercises included mini-squats, mini-lunges, and lunge walks. The intensity of the training was set at two sets of six to eight repetitions and was increased using the 7RM method, when two sets of six to eight repetitions were completed with proper form and without discomfort. The BAT program included stretching, range-of-motion, core-strength, balance, and relaxation exercises. Only body weight was used with no added loading. The intent of the BAT program was to provide an active control for factors secondary to the focus of the RT program, including in-person contact and socialization.
Measures
Descriptive variables
The following variables were collected at baseline and were used to determine whether the treatment conditions were equivalent and to predict dropout at the 2-year follow-up. Age in years and education were assessed by self-report. Waist-to-hip ratio was determined by measuring the widest part of the hip circumference and the waist just above the navel in centimeters. Depression was assessed by the 15-item Geriatric Depression Scale (Yesavage et al., Reference Yesavage, Brink, Rose, Lum, Huang, Adey and Leirer1982). Global cognition was assessed with the Mini-mental State Examination (Cockrell & Folstein, Reference Cockrell and Folstein1988). The number of comorbid conditions related to physical functioning was determined using the Functional Comorbidity Index (Groll, To, Bombardier, & Wright, Reference Groll, To, Bombardier and Wright2005). Physiological falls risk was assessed with the Physiological Profile Assessment (Lord, Menz, & Tiedemann, Reference Lord, Menz and Tiedemann2003). General mobility was assessed via the Short Physical Performance Battery (Guralnik, Ferrucci, Simonsick, Salive, & Wallace, Reference Guralnik, Ferrucci, Simonsick, Salive and Wallace1995).
Verbal memory
The Rey Auditory Verbal Learning Test (Spreen & Strauss, Reference Spreen and Strauss1998) assessed verbal memory functioning. Participants were read a list of 15 common words five times. Immediately after each time, participants were asked to recall as many words as possible. After the fifth time, an interference list was presented, after which participants were asked to recall as many words as possible from the original list. After a 20-min delay, the participants were asked to recall again the original word list. Finally, a story that uses all the words from the original list was presented, and the participant was asked to identify words recognized from that list. Although several performance indices can be derived from this assessment, we used three to focus on verbal memory: (1) Recall after interference; (2) delayed recall; and (3) recognition of words from the story. Recognition scores were skewed at each time point (−1.6 to −3.5) and were log10 transformed to better normalize their distributions.
Executive function
Four separate measures of EF were included. On the Stroop Test (Stroop, Reference Stroop1935), participants first read out words printed in black ink (e.g., BLUE). Second, they named the display color of colored X’s. Finally, they were shown a page with color words printed in incongruent colored inks (e.g., the word “BLUE” printed in red ink). Participants were asked to name the ink color in which the words were printed (while ignoring the word itself). We recorded the time participants took to read the items in each condition and calculated the time difference between the third condition (Incongruent) and the second condition (Neutral). The Trail Making Test asks participants to draw lines connecting encircled numbers sequentially (Part A) or alternating between numbers and letters (Part B) (Spreen & Strauss, Reference Spreen and Strauss1998). The difference in time to complete Part B and Part A was calculated, with smaller difference scores indicating better cognitive flexibility. Backward digit span required participants to correctly reproduce verbally a progressively longer random number sequence in reverse order (Wechsler, Reference Wechsler1980). On the Digit Symbol Substitution Test, participants are provided a code table with nine distinct symbols associated with the numbers 1–9 and 1 min to fill in as many symbols in a matrix of numbers below (Spreen & Strauss, Reference Spreen and Strauss1998).
Muscular function
In a subset of eligible participants (n=99 at baseline)—who were without preexisting knee, hip, or back condition based on a screen by the study physician—peak muscle strength was assessed using an air-pressured digital resistance leg press machine. These participants completed leg press extensions at six relative loads of their single-repetition maximum lift (1-RM), that is, 40%, 50%, 60%, 70%, 80%, and 90%. Participants performed the concentric portion of the leg press as rapidly as possible and then slowly lowered the load during a 3-s count. Participants completed 3 repetitions at each relative 1-RM load, with a 30-s rest between repetitions. The leg press recorded the power produced (in watts), and the peak power was used in subsequent analyses.
Structural MRI Data Acquisition, Data Processing, and Analysis
T1-weighted structural MRI data were acquired on a research-dedicated Philips 3 Tesla (T) Achieva MRI scanner (Philips Medical Systems, Best, The Netherlands) at the UBC MRI Research Center. Brain volume estimates were obtained using the FreeSurfer image analysis suite, which uses an automated labeling system based on probabilistic information from a manually labeled training set (Desikan et al., Reference Desikan, Segonne, Fischl, Quinn, Dickerson, Blacker and Killiany2006; Fischl et al., Reference Fischl, Salat, Busa, Albert, Dieterich, Haselgrove and Dale2002). To extract reliable volume estimates, images were processed with the longitudinal stream in FreeSurfer (Reuter, Schmansky, Rosas, & Fischl, Reference Reuter, Schmansky, Rosas and Fischl2012), which creates an unbiased within-subject template space and image from robust, inverse consistent registration (Reuter, Rosas, & Fischl, Reference Reuter, Rosas and Fischl2010). Skull stripping, Talairach transforms, atlas registration, spherical surface maps, and parcellations were initialized with common information from the within-subject template, which improves statistical power and reliability (Reuter et al., Reference Reuter, Schmansky, Rosas and Fischl2012). Cortical WM volume parcellation was performed using a FreeSurfer tool described by Salat and colleagues (Salat et al., Reference Salat, Greve, Pacheco, Quinn, Helmer, Buckner and Fischl2009), which assigns a WM label based on the nearest cortical parcellation and applies a distance constraint to avoid inclusion of WM from the centrum semiovale and periventricular regions. Areas segmented as WM hypo-intensities (i.e., dark spots) were subtracted out of the cortical WM volume (Westlye et al., Reference Westlye, Walhovd, Dale, Bjornerud, Due-Tonnessen, Engvig and Fjell2010). We examined cortical WM, cortical GM, and hippocampal volume. All subjects underwent manual checking following the automated segmentation by one of the co-authors (B.C.K.), who was blinded to the subjects’ treatment condition. No changes resulted from the manual checks.
Statistical Analyses
The main analyses were conducted in Mplus 7.3 (Muthén & Muthén, 2014). For the primary outcomes of EF and verbal memory, we used second-order latent change-regression models (McArdle, Reference McArdle2009) as depicted in Figure 2a and 2b, respectively. In these models, a latent score was created at each time point and then a latent change score was computed. In both of these models, strong longitudinal invariance was supported by acceptable model fit (root mean square error of approximation ≤0.05 and comparative fit index >.95) (Hu & Bentler, Reference Hu and Bentler1998), in which factor loadings and intercepts were set to be equivalent for each cognitive measure across time (McArdle, Reference McArdle2009). Residual variances in the observed variables were allowed to correlate across time. For both latent change-regression models, the change score is adjusted for baseline scores. Two dummy-coded variables were created to represent treatment condition in the models and to test the effects of resistance training (either once- or twice-weekly) against the balance-and-tone condition. Specifically, the bold lines in Figure 2 test whether the change in the outcome from baseline to 1-year follow-up (i.e., immediately post-intervention) and from baseline to the 2-year follow-up (i.e., 1 year after the intervention ended) was significantly different for those participants assigned to RT compared to those assigned to BAT. For the MRI brain volume outcomes, we used a simpler analytic approach given the smaller sample size. Here, we used multiple linear regression to determine whether RT (either once- or twice-weekly) compared to BAT predicted changes in the MRI variables from baseline to post-intervention and to 2-year follow-up. A separate model was created for each brain region of interest, and change scores were adjusted for baseline score for the brain region of interest, as well as baseline intracranial volume. The same dummy-coded treatment variable described previously was used in these analyses. Similarly, multiple linear regression was used to examine the impact of RT on peak muscle power, controlling for baseline power output.

Fig. 2 Diagrams of the latent change-regression models used to evaluate the impact of once- and twice-weekly resistant training, in comparison to twice-weekly balance and toning, on memory (a) and executive function (b).
For these analyses, maximum likelihood estimation with robust standard errors was used, which handles missing data via implicit imputation within the estimation process under the assumption that data are missing at random (Enders, Reference Enders2013). Thus, the analyses are intent-to-treat, in which all individuals with baseline data are included. This approach has been shown to provide less biased estimates and to increase generalizability in comparison to deletion approaches that remove individuals with missing follow-up data (Elobeid et al., Reference Elobeid, Padilla, McVie, Thomas, Brock, Musser and Allison2009). In the analyses, we included baseline Short Physical Performance Battery (SPPB) score, education, and exercise class attendance rate as missing data correlates because these variables predicted drop-out at the 2-year follow-up (see the Results section below). As missing data correlates, these variables are allowed to correlate with the outcome variables to improve the plausibility of the missing at random assumption (Collins, Schafer, & Kam, Reference Collins, Schafer and Kam2001). We also conducted follow-up sensitivity analyses, in which we excluded individuals without observed data at the 2-year follow-up to determine whether the estimates of treatment effects were similar in the intent-to-treat and “completers” analyses. In evaluating the treatment effects on change in our outcomes over time, we report standardized difference scores (d), which provide an estimate of the treatment effect size (.20 is small; .50 is moderate; .80 is large) (Cohen, Reference Cohen1992). Given that multiple outcomes were analyzed, we used the Benjamini-Hochberg false discovery rate (FDR) correction (Benjamini & Hochberg, Reference Benjamini and Hochberg1995) in the primary analyses.
RESULTS
Table 1 provides the baseline characteristics of the sample separated by treatment condition. There were no between-group differences in any of the baseline descriptive variables (ps>.10). Completion of the post-intervention cognitive assessment (n=135; 87% of baseline sample) and of the 2-year follow-up cognitive assessment (n=109; 70% of baseline sample) did not differ by treatment group (ps>.22). However, individuals with missing data at the 2-year follow-up cognitive assessment had lower exercise attendance rates, lower educational attainment, and lower SPPB scores at baseline (p<.05). Among the MRI subsample, completion of the post-intervention MRI assessment (n=68; 82% of baseline sample) and of the 2-year follow-up MRI assessment (n=28; 34% of baseline subsample) did not differ by treatment group (ps>.19), but individuals with lower attendance rates were less likely to complete the 2-year MRI assessment (p=.035). Class attendance did not differ significantly by treatment group, either in the overall cohort or MRI subset (p ≥.10). Attendance rates for the BAT were 62% and 65% (for the overall cohort and MRI subset, respectively), 71% and 70% for the once-weekly RT, and 70% and 71%, for the twice-weekly RT. Women who were included in the MRI subsample did not differ from women who were not included on any of the covariates or study variables, with the exception that women in the MRI subsample had higher baseline DSST scores (average score of 34 vs. 31; p=.008).
Table 1. Descriptive statistics by treatment group

* All p values computed from one-way analysis of variance or chi-square test (education and percentages with 2-year cognitive or brain MRI data).
a Sum of the following categories: bisphosphonates, antidepressants, calcium, vitamin D, folate, and vitamin B12.
b Percentages are computed based on the subset with baseline MRI data (n=83), not the entire study sample (n=155).
BAT=balance-and-tone condition; 1XRT=once-weekly resistance training; 2XRT=twice-weekly resistance training; FCI=Functional Comorbidity Index; GDS=Geriatric Depression Scale; MMSE=Mini-mental state examination; MRI=Magnetic resonance imaging; PPA=Physiological Profile Assessment; SPPB=short physical performance battery.
One individual from the BAT group showed extreme decline (≥3 SD from average) in EF and memory from baseline to 2-year follow-up; this person was removed from the analyses of cognition due to positive bias of the treatment effects. Descriptive statistics for cognitive and brain measures across time and separated by treatment group can be found in the Online Supplement in Supplementary Tables 1–3. First, descriptive statistics are provided for all available observations at each time point and then are limited to the subset of participants who completed the 2-year assessment.
Latent memory and EF were moderately correlated at baseline (r=.27; p=.001); however, change in EF and memory from baseline to post-intervention and from baseline to 2-year follow-up were not correlated, when accounting for baseline scores and treatment group (partial correlation [pr]=.07 and −.03, respectively; ps>.37), which indicates that they are different constructs showing distinct change over time. Table 2 summarizes the treatment effects estimated from the regression models. Twice-weekly RT positively impacted change in memory from baseline to 2-year follow-up in comparison to BAT (d=.45; p=.002). Once-weekly RT positively impacted change in EF post-intervention (d=.35; p=.002), and this impact persisted at 2-year follow-up (d=.48; p=.002). Twice-weekly RT trended toward positively impacting EF post-intervention (d=.24; p=.046), but this effect was not significant when applying the FDR correction. Nevertheless, twice-weekly RT did positively impact change in EF at the 2-year follow-up (d=.31; p=.005). To visualize these effects, Figure 3 plots the distribution of change in memory and EF scores by treatment group from baseline to post-intervention and from baseline to 2-year follow-up.

Fig. 3 Impact of treatment conditions on change in memory and executive function from baseline to post-intervention (a,b) and from baseline to 2-year follow-up (c,d). Kernel density curves were created using the graphical tool ggplot2 in R. The dashed vertical lines represent the median score for each distribution. **p<.01.
Table 2. Effects of resistance training on cognition, muscle power, and brain volume

a Significant after using Benjamini-Hochberg false discovery rate correction for 14 tests per treatment contrast (i.e., 1xRT vs. BAT and 2xRT vs. BAT).
1XRT=effect of once-weekly resistance training compared to balance-and-tone training; 2XRT=effect of twice-weekly resistance training compared to balance-and-tone training;BL=baseline; EF=executive function; GM=gray matter; HC=hippocampus; ICV=intracranial volume; WM=white matter.
Table 2 also provides the effects of RT on peak muscle power and on brain volumes. Twice-weekly RT (but not once-weekly RT) increased peak muscle power at both 1-year and 2-year follow-up (d=.52 and .27, respectively; p<.01). There was no evidence for RT to reduce cortical GM or hippocampal atrophy post-intervention or at the 2-year follow-up. In contrast, twice-weekly RT reduced cortical WM atrophy compared to BAT at 2-year follow-up (d=.45; p=.009). When expressed as a percent change from baseline, women in BAT had a 2.0% decrease in cortical WM volume over the 2-year period, whereas women in twice-weekly RT had a 0.8% decrease and women in once-weekly RT had a 1.5% decrease in cortical WM. The effects of RT on muscle power and on cortical WM volume are depicted in Figure 4.

Fig. 4 Impact of treatment conditions on change in peak muscle power and white matter volume from baseline to post-intervention (a,b) and from baseline to 2-year follow-up (c,d). Peak muscle power (in watts) values have been divided by 100. Cortical white matter volumes are expressed in cm3. Kernel density curves were created using the graphical tool ggplot2 in R, and the dashed vertical lines represent the median score for each distribution. **p<.01. ***p<.001.
We next conducted sensitivity analyses, in which we limited the study sample to those individuals who completed the 2-year assessment. The long-term effects on cognition and cortical WM volume were replicated in this smaller sample; however, we did not observe that twice-weekly RT increased peak muscle power at the 2-year follow-up (see Supplementary Table 4). We then conducted a second set of sensitivity analyses, in which we added baseline and 2-year medication and supplement use as an additional covariate. As noted in Table 1, the twice-weekly RT group used fewer medications and supplements at year 2 (p=.006 for comparison to once-weekly RT and p=.077 for comparison to BAT) but not at baseline. All significant treatment effects on EF, memory, cortical WM volume, and on muscle power remained when controlling for baseline and 2-year the medication and supplement use (not shown). In a final set of analyses, we computed partial correlations to determine the association between concurrent change among the outcome variables, controlling for treatment condition and the baseline scores. We did not impute missing values for these analyses. As shown in Table 3, no correlation exceeded a moderate size, and none reached significance when applying the FDR correction.
Table 3. Partial correlation analyses
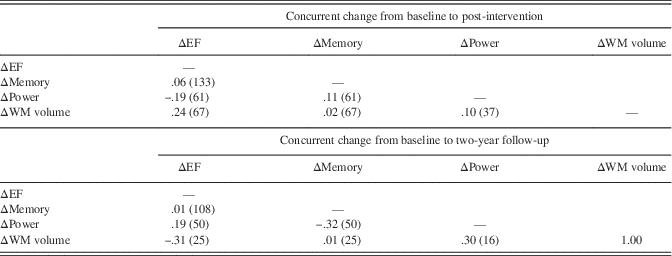
Note. Correlations are partialled for baseline scores and treatment assignment. The number of individuals factored into each correlation are shown in parentheses after the correlation value. No correlations were significant after applying Benjamini-Hochberg false discovery rate correction for 12 correlations.
EF=executive function; WM=white matter.
DISCUSSION
Our results suggest that RT might have long-term impacts on cognitive and brain health. The most robust finding was that RT—either once- or twice-weekly over 52 weeks—had a positive impact on EF that persisted one year following RT cessation. EF encompasses those cognitive processes that are critical to controlled, goal-oriented behavior (Banich, Reference Banich2009), and EF deficits have been shown to be a marker for future mobility (Hsu, Nagamatsu, Davis, & Liu-Ambrose, Reference Hsu, Nagamatsu, Davis and Liu-Ambrose2012) and memory impairment (Carlson et al., Reference Carlson, Xue, Zhou and Fried2009) in older adults. Thus, the improvement in EF might have important and positive implications for the future functioning of the RT participants. We also found that twice-weekly RT improved verbal memory performance. However, this effect was only evident at the 2-year follow-up—and not post-intervention—which raises concerns over the reliability of this effect. Therefore, the degree to which RT might improve memory functioning remains uncertain.
We also observed that the twice-weekly RT program improved peak muscle power at both time points and decreased WM atrophy at the 2-year follow-up. Cortical WM volume includes the afferent and efferent fibers that allow for communication within a cortical region (Salat et al., Reference Salat, Greve, Pacheco, Quinn, Helmer, Buckner and Fischl2009). Age-related WM atrophy is evident in various cortical regions (Salat et al., Reference Salat, Greve, Pacheco, Quinn, Helmer, Buckner and Fischl2009, Reference Salat, Tuch, Greve, van der Kouwe, Hevelone, Zaleta and Dale2005), and this atrophy accelerates with increasing age (Raz et al., Reference Raz, Lindenberger, Rodrigue, Kennedy, Head, Williamson and Acker2005; Salat et al., Reference Salat, Greve, Pacheco, Quinn, Helmer, Buckner and Fischl2009; Walhovd et al., Reference Walhovd, Fjell, Reinvang, Lundervold, Dale, Eilertsen and Fischl2005). Moreover, cortical WM volume is reduced among older adults with dementia compared to their healthy counterparts (Salat et al., Reference Salat, Greve, Pacheco, Quinn, Helmer, Buckner and Fischl2009). Hence, maintaining cortical WM volume might be important for maintaining cognitive functions as one reaches advanced age, and RT might be a low-cost behavioral strategy to decelerate WM atrophy in the aging brain.
In contrast to the effects on cortical WM, we found no evidence that RT had a beneficial effect on cortical GM or on hippocampal volume. Thus, our findings differ from reports that have shown that AT reduces hippocampal atrophy in aged populations (Erickson et al., Reference Erickson, Voss, Prakash, Basak, Szabo, Chaddock and Kramer2011; Ten Brinke et al., Reference Ten Brinke, Bolandzadeh, Nagamatsu, Hsu, Davis, Miran-Khan and Liu-Ambrose2015). Exercise may have effects that are not uniform across brain regions (Erickson et al., Reference Erickson, Voss, Prakash, Basak, Szabo, Chaddock and Kramer2011), and moreover, RT and AT may influence cognition through distinct neurobiological pathways (Cassilhas et al., Reference Cassilhas, Lee, Fernandes, Oliveira, Tufik, Meeusen and de Mello2012). AT has been shown to up-regulate brain-derived neurotrophic factor (BDNF), a neurotrophin that promotes neurogenesis (Voss, Vivar, Kramer, & van Praag, Reference Voss, Vivar, Kramer and van Praag2013), and changes in BDNF levels in the serum correlate with changes in hippocampal volume in older participants in AT (Erickson et al., Reference Erickson, Voss, Prakash, Basak, Szabo, Chaddock and Kramer2011). In contrast, RT increases serum levels of IGF-1 in older adults (Cassilhas et al., Reference Cassilhas, Viana, Grassmann, Santos, Santos, Tufik and Mello2007; Parkhouse et al., Reference Parkhouse, Coupland, Li and Vanderhoek2000; Vale et al., Reference Vale, de Oliveira, Pernambuco, de Meneses, Novaes Jda. and de Andrade Ade2009), but it is unclear whether AT also up-regulates IGF-1, especially among older women. One study found that AT increased IGF-1 in older men but not in older women (Baker et al., Reference Baker, Frank, Foster-Schubert, Green, Wilkinson, McTiernan and Craft2010), whereas another study found that RT, but not AT, increased IGF-1 in older women (Vale et al., Reference Vale, de Oliveira, Pernambuco, de Meneses, Novaes Jda. and de Andrade Ade2009). In addition to promoting hypertrophic adaptation of muscle to RT (Adams & Haddad, Reference Adams and Haddad1996), IGF-1 has been shown to pass through the blood brain barrier where it has neuroprotective effects (Carro et al., Reference Carro, Nunez, Busiguina and Torres-Aleman2000; Cotman et al., Reference Cotman, Berchtold and Christie2007), including promoting brain myelination (Ye, Carson, & D’Ercole, Reference Ye, Carson and D’Ercole1995) and aiding recovery of myelin following pathological insult (Mason, Ye, Suzuki, D’Ercole, & Matsushima, Reference Mason, Ye, Suzuki, D’Ercole and Matsushima2000). This evidence may explain why we found selective effects to older women’s cortical WM volume. However, we caution that the absence of serum collection precludes us from determining whether IGF-1 levels were increased and then whether IGF-1 levels mediated the effects reported herein. Moreover, exercise might exert its effects via other pathways (including combinations of pathways), for example, by reducing cardiometabolic risk factors (e.g., hypertension, blood glucose levels), systemic inflammation, or stress hormones (Cotman et al., Reference Cotman, Berchtold and Christie2007).
One of the strengths of this study was the use of a latent variable approach to analyze the cognitive outcomes. This approach allowed us to minimize measurement error and reduce the number of statistical tests undertaken, and to specify that RT impacts the underlying EF and memory processes required across various EF and verbal memory indices. A second strength was the inclusion of a follow-up assessment one year after the intervention ended. Very few studies of exercise training report long-term follow-up data, and thus, the time-course of exercise-induced effects to brain and cognition are largely unknown. In one notable example, Lautenschlager and colleagues (Reference Lautenschlager, Cox, Flicker, Foster, van Bockxmeer, Xiao and Almeida2008) found that the effects of a 6-month home-based exercise program on memory functioning were evident, although weakened, at a 2-year follow-up among older adults at risk for Alzheimer’s disease. This suggests that exercise can have an enduring impact on cognitive functioning; however, it remains uncertain how these effects are sustained. We were unable to identify any underlying factors that might explain the long-term effects on cognition. That is, our correlation analysis did not yield any significant associations among the study variables that would explain why cognitive function is enhanced as a result of RT. Thus, the observed treatment effects on cognition, WM volume, and muscle strength appear to be largely independent of one another. In a previous study using the same study sample, we found that there were no differences in physical activity levels among the exercise groups during the follow-up period, based on monthly self-report assessments of physical activity collected throughout that period (Best, Nagamatsu, & Liu-Ambrose, Reference Best, Nagamatsu and Liu-Ambrose2014). This makes it unlikely that the observed effects on cognition were mediated by changes in physical activity, although the lack of objective physical activity assessments requires us to make this statement cautiously. Clearly, it is important for future exercise RCTs to include follow-up assessments to better characterize and understand long-term effects
Our current understanding of how exercise promotes cognitive function largely stems from animal studies and is restricted to AT. Thus, once the effect of RT on cognition is firmly established, future studies should include detailed neuroimaging and physiological assessments to identify the underlying changes to the body and brain that explain the changes to cognition. As an example, the inclusion of blood collection would allow one to determine whether circulating levels of various neurotrophins (e.g., IGF-1) or other factors (e.g., pro-inflammatory factors) are altered and whether these changes correlate with changes in cognition. Another line of research should include additional neuroimaging modalities, such as DTI. Although the examination of WM volume might be important based on previous research showing that cortical WM volume decreases with increasing age (Salat et al., Reference Salat, Greve, Pacheco, Quinn, Helmer, Buckner and Fischl2009; Walhovd et al., Reference Walhovd, Fjell, Reinvang, Lundervold, Dale, Eilertsen and Fischl2005) and is reduced among older adults with dementia (Salat et al., Reference Salat, Greve, Pacheco, Quinn, Helmer, Buckner and Fischl2009), DTI is able to quantify the microstructural integrity of the WM, which might be a more sensitive measure of age-related changes in WM than is volumetric analysis (Westlye et al., Reference Westlye, Walhovd, Dale, Bjornerud, Due-Tonnessen, Engvig and Fjell2010).
There are limitations to the current study that should also be addressed in future research. The key limitation is the amount of missing data due to dropout at the 2-year assessment, especially in the MRI data. We addressed this issue in two ways. First, we used an intent-to-treat analytic approach using missing variable correlates to use all participants with baseline data and to improve the plausibility of the assumption that data were missing at random. Second, we conducted sensitivity analysis, in which we limited the study sample to only those with 2-year data. These analyses converged on the same findings; however, having more complete data would have been preferable, and we cannot rule out that our results might be influenced in some way by the missing data. As a result, these results should be considered tentative and require replication. A second limitation is that our sample was fairly homogenous and consisted of independent community-dwelling older women aged 65–75, who were without major physical or cognitive impairment. This reduces our ability to generalize to other populations of older adults. Related to this concern, future research should directly compare the effects of RT between men and women, especially given that the physiological adaptations to exercise might be sex specific (Baker et al., Reference Baker, Frank, Foster-Schubert, Green, Wilkinson, McTiernan and Craft2010). A third limitation is that the relatively low compliance rates suggest that a proportion of participants—especially those in the once-weekly RT program—received a fairly infrequent dose of RT (e.g., once every other week). Finally, the fact that we did not perform any manual editing of the FreeSurfer brain volumes might be a limitation. Errors in the automated segmentation process can occur (e.g., including parts of the skull in the occipital and posterior parietal regions), which might have been overlooked. However, our examination of total cortical volumes, rather than regional volumes, minimizes this concern.
To conclude, our results suggest that both once- and twice-weekly RT have a long-term impact on EF, and that twice-weekly RT has additional positive impacts to memory and to the protection of cortical WM. These results suggest that even relatively infrequent RT (i.e., once per week) might have significant effects to cognition; however, more frequent RT might produce more widespread positive effects to cognition and brain structure. In light of the age-related decline in these aspects of cognition and brain structure, our results suggest that frequent RT can be a behavioral strategy to protect brain and cognition in older women. The underlying mechanisms that explain these effects of RT remain unknown, and therefore, future research will need to explore various possible physiological changes that might account for the positive effects of RT on brain and cognitive health.
Acknowledgments
All authors have no conflicts of interest to declare. This work was supported by operating grants from The Vancouver Foundation (T.L.A., BCM06-0035) and by an Establishment Grant from the Michael Smith Foundation for Health Research (T.L.A., CI-SCH-063 [05-0035]). John R. Best is a Michael Smith Foundation for Health Research and Canadian Institutes of Health Research Post-Doctoral Fellow. Chun Liang Hsu is an Alzheimer’s Society Research Program Doctsoral trainee. Lindsay S. Nagamatsu is a Canadian Institutes of Health Research Post-Doctoral Fellow. Teresa Liu-Ambrose is a Canada Research Chair Tier II in Physical Activity, Mobility, and Cognitive Neuroscience.
Supplementary Material
To view supplementary material for this article, please visit http://dx.doi.org/10.1017/S1355617715000673.