Introduction
Seagrasses are marine angiosperms that provide key ecological services to coastal ecosystems. Unfortunately, seagrasses are experiencing a global crisis in terms of habitat decline (Waycott et al., Reference Waycott, Duarte, Carruthers, Orth, Dennison, Olyarnik, Calladine, Fourqurean, Heck, Hughes, Kendrick, Kenworthy, Short and Williams2009). Excess nutrients in coastal waters can lead to an increase in sulfide levels in seagrass beds (Ruiz-Halpern et al., Reference Ruiz-Halpern, Macko and Fourqurean2008). H2S is a potent toxin that can easily cross cell membranes and enter the plant (intrusion), potentially inducing seagrass mortality (Koch & Erskine, Reference Koch and Erskine2001). Sulfide intrusion can be assessed using stable sulfur isotope (32S, 34S) measurements. Sulfate-reducing bacteria discriminate against the heavier 34S isotope, preferring the lighter 32S form. This yields sedimentary H2S with a lower 34S isotopic “signal” (Canfield, Reference Canfield2001). This signal can be quantified in plants, providing an estimate of the proportion of tissue sulfur derived from sedimentary sulfide (Frederiksen et al., Reference Frederiksen, Holmer, Borum and Kennedy2006).
Thus far, the literature on sulfide intrusion in seagrasses shows relatively few measurements for species besides Zostera marina, Thalassia testudinum, and Posidonia oceanica (Holmer & Hasler-Sheetal, Reference Holmer and Hasler-Sheetal2014). The objective of this study was to use stable isotope analysis to examine H2S intrusion in the seagrass Halodule wrightii, a semi-tropical species found throughout the Gulf of Mexico, Caribbean, and parts of both the eastern and western Atlantic coasts (Green & Short, Reference Green and Short2003). We also estimated the proportion of total sulfur derived from sedimentary sulfide in the root, rhizome, and leaf tissues. Our goal was to obtain a more complete picture of sulfide uptake and distribution in this species, including its influence on the sulfur content in major plant organs.
Methods
Forty-eight H. wrightii, 10 sediment, and five seawater samples were collected from Oso Bay, Corpus Christi, TX, near the campus of Texas A&M University-Corpus Christi (Figure 1). Leaf, rhizome, and root tissues were separated from each plant sample, oven-dried and ground to a fine powder using a ball mill. Sediment sulfide was extracted and precipitated as Ag2S using a modified total reduced inorganic sulfur (TRIS) distillation method based on Backlund et al. (Reference Backlund, Boman, Frojdo and Astrom2005) and Fossing and Jørgensen (Reference Fossing and Jørgensen1989). A detailed protocol is available at https://doi.org/10.17504/protocols.io.b2b8qarw. Seawater sulfate was precipitated as barium sulfate (BaSO4) under acidic conditions (Grasshoff et al., Reference Grasshoff, Kremling and Ehrhardt1999). Tissue, Ag2S, and BaSO4 samples were sent to the Stable Isotopes for Biosphere Science (SIBS) Laboratory at Texas A&M University (College Station) for analysis of stable sulfur isotope ratios (δ34S; per mil (‰) units) and total sulfur content (TS; % dw) using elemental analyzer combustion continuous flow isotope ratio mass spectroscopy. δ34S represents the deviation in the ratio of 34S/32S from a particular sample relative to an international standard, and is defined as

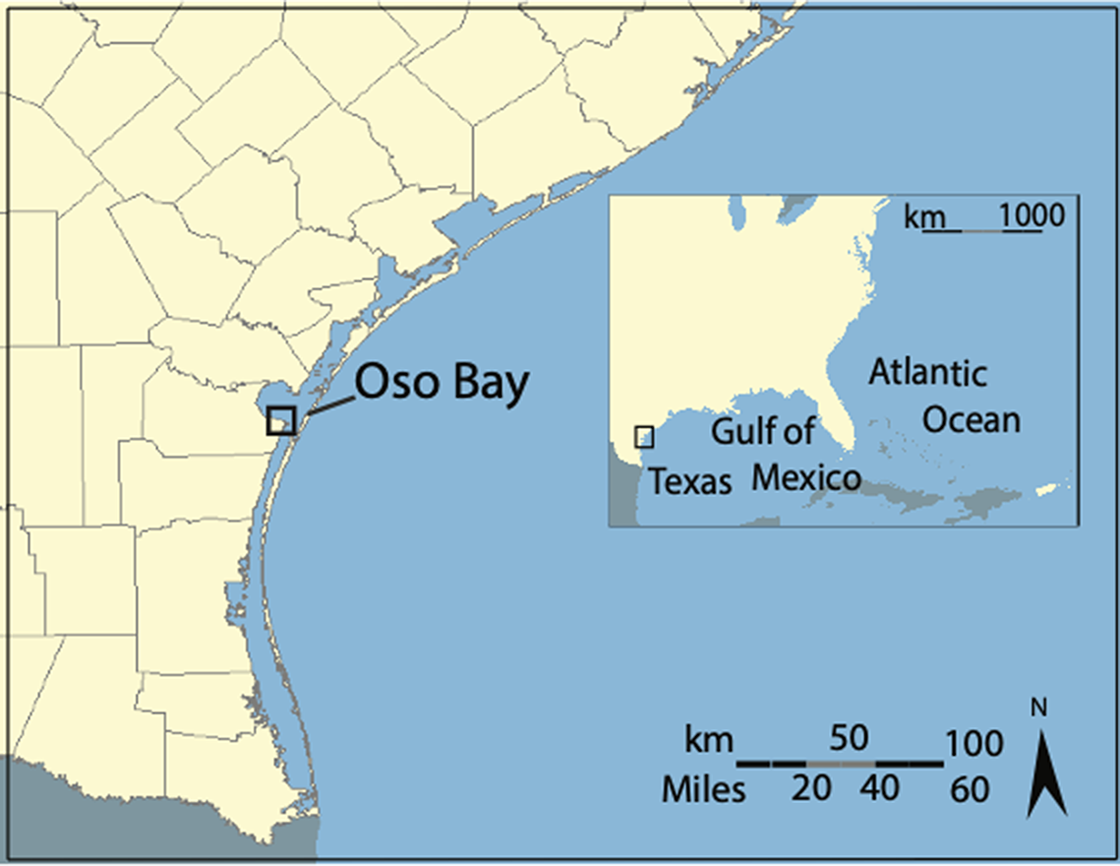
Figure 1. Sampling site in Oso Bay, Corpus Christi, TX.
where R represents the 34S/32S ratio and VCDT corresponds to the Vienna-Canyon Diablo Troilite international standard. δ34S values can be negative or positive depending on whether a sample is depleted or enriched, respectively, for the S34 isotope compared to the standard.
δ34S values from seagrass tissues (root, rhizome, leaf), sediment sulfide, and seawater sulfate were used to calculate the F sulfide parameter, an estimate of the percentage of the total sulfur content within a tissue that is derived from sedimentary sulfide (Frederiksen et al., Reference Frederiksen, Holmer, Borum and Kennedy2006):

Results
The mean δ34S value from 10 sediment samples was −27.38 ± 1.41‰, while that of the seawater sulfate samples was +21.11 ± 0.76‰ (Table 1). Mean δ34S values for seagrass tissues ranged from −5.58 ± 3.73‰ for roots to +13.58 ± 2.04‰ for leaves (Table 2). F sulfide values ranged from 15.51 ± 4.2% of the total sulfur content in leaves to 55.02 ± 7.68% in roots (Table 2).
Table 1. δ34S values of sulfur sources (sediment TRIS or seawater sulfate) from H. wrightii bed in Oso Bay, Corpus Christi, TX

Note. Values are given as sample mean ± SD. N = number of observations.
Abbreviation: TRIS, total reduced inorganic sulfur.
Table 2. Total sulfur (TS) and δ34S values for H. wrightii leaf, rhizome, and root samples from Oso Bay, Corpus Christi, TX

Note. Values are given as mean ± SD. Sample sizes for each tissue were N leaf = 47, N rhizome = 48, and N root = 48.
While the proportion of sulfur derived from sediment sulfide varied, the total sulfur content (%TS) across tissues was similar (Table 2). Mean TS values ranged from 0.49 ± 0.18% in rhizomes to 0.55 ± 0.23% in roots. Variation in mean δ34S, F sulfide, and TS values among tissues was assessed with a one-way analysis of variance (ANOVA) test. Results showed significant differences for F sulfide and δ34S across seagrass tissues, but no statistically significant difference for TS (Figure 2).
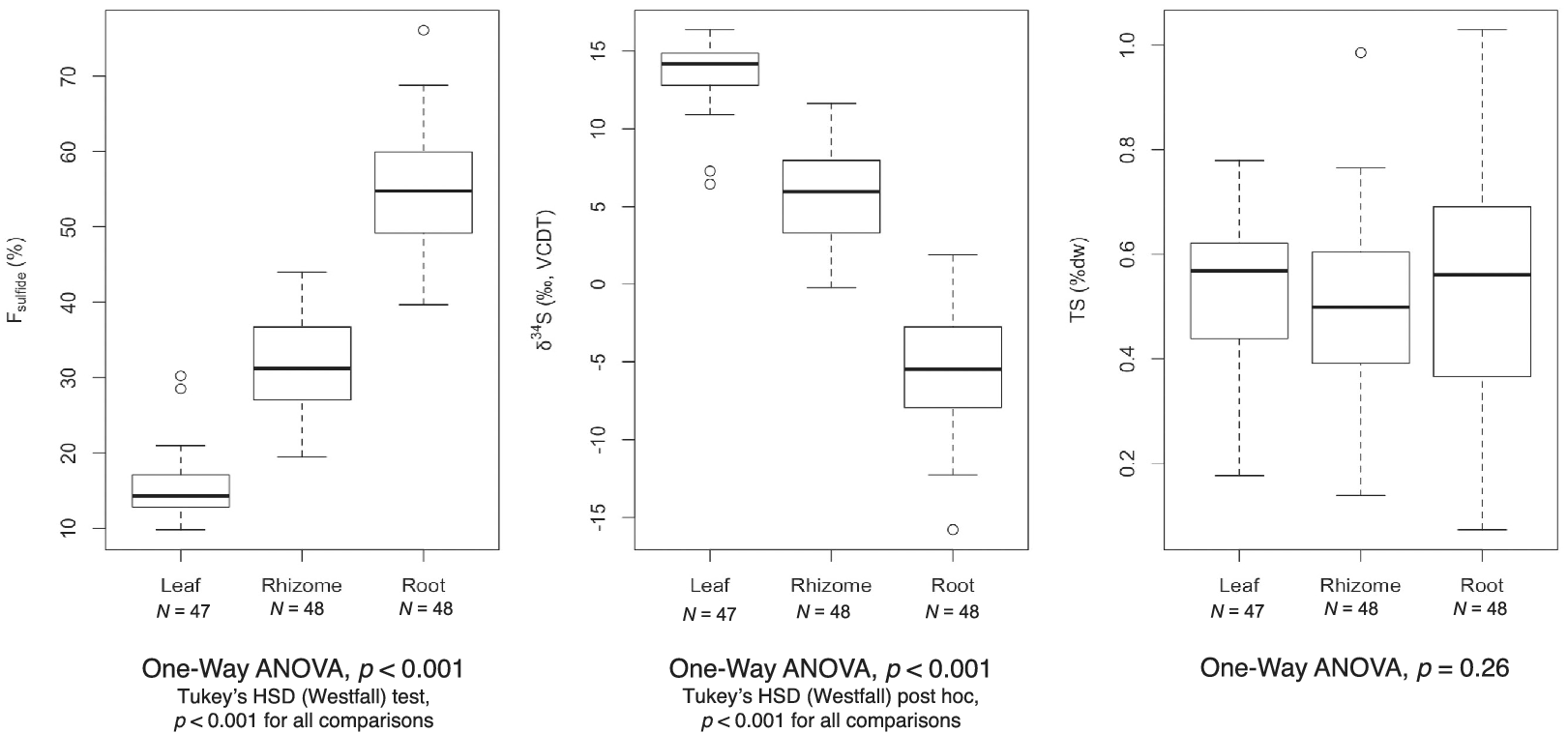
Figure 2. Boxplots representing variation among F sulfide (a), δ34S (b), and total sulfur (c) values for H. wrightii tissues. p-values from one-way ANOVAs are included below each graph. Individual dots represent outliers, as defined by any number larger than 3rd Quantile (Q3) + 1.5 interquartile range (IQR) or smaller than 1st Quantile (Q1) – 1.5 IQR. Lines outside the box (whiskers) extend to the smallest and largest non-outliers.
Discussion
The mean δ34S values for H. wrightii leaf and root tissues were higher than those previously reported for this species (+9.3‰ and −7.4‰, respectively, Holmer & Hasler-Sheetal, Reference Holmer and Hasler-Sheetal2014) but the high level of variation for these measurements across studies suggests the differences may not be significant. Although we could find no previous report for H. wrightii rhizomes, the mean δ34S value for this tissue was similar to one calculated across a number of seagrass species (+5.1‰, Holmer & Hasler-Sheetal, Reference Holmer and Hasler-Sheetal2014).
We found a gradient in δ34S values from roots to leaves, suggesting that H2S enters the roots and then passes up to the rhizome and leaf tissue, either as sulfide itself or in an oxidized or metabolized form. This was quantified as F sulfide, which estimated that approximately 55, 32, and 15% of the total sulfur content in roots, rhizomes, and leaves, respectively, came from sediment-derived H2S. The range of values suggests a mixing of the sulfur pools (seawater sulfate and sedimentary sulfide) in the various tissues, similar to other species. A comparable gradient, however, was not observed for total sulfur content, which remained similar across tissue types. This could suggest that, while H2S can intrude and become distributed throughout the plant, the level does not exceed H. wrightii’s normal metabolic requirements for sulfur.
Conclusion
Our findings suggest that significant sedimentary H2S intrusion can occur in H. wrightii, entering through the roots and then becoming distributed throughout the plant. The results verified a trend previously observed for H. wrightii, and seagrasses in general. They also represent the first report we are aware of for rhizome tissue from this species. The relatively high proportions of total sulfur content derived from sedimentary sulfide in root, rhizome, and leaf tissue suggest H. wrightii is able to convert H2S into non-toxic forms that can accumulate and mix with other sulfur-containing compounds derived from seawater sulfate, as demonstrated in Z. marina (Hasler-Sheetal & Holmer, Reference Hasler-Sheetal and Holmer2015). Diverse levels of intrusion, however, did not translate into differences in total sulfur content among tissues, suggesting that H2S-derived products may constitute a normal part of their sulfur budget.
Acknowledgments
We thank Richard Coffin, Paula Rose, and Hao Yu for assistance with preparation of samples for isotopic analysis.
Supplementary Materials
To view supplementary material for this article, please visit http://doi.org/10.1017/exp.2022.15.
Data availability statement
The isotope results used in this study are available in the Supplementary Materials (Supplementary Table S1).
Funding statement
This research was supported by a Graduate Research Award from the Texas Sea Grant program, a Hans and Patricia Suter Endowment Award from Texas A&M University-Corpus Christi, and by the Welch Foundation.
Conflict of interest
The authors have no conflicts of interest to declare.
Authorship contributions
S.R.-R. and P.D.L. designed the study and collected the samples for analysis. S.R.-R. conducted the data collection and statistical analyses. S.R.-R. and P.D.L. wrote the article.
Comments
Comments to the Author: Nice short paper. Minor comments. L.9-10,L.22: These are natural processes that can be intensified by human influence. Sulfide intrusion is not anthropogenic per se and not unusual in (most) seagrass species. Coastal sediments are generally anoxic below a couple cm regardless of eutrophication status. L.21-22: Citation(s). L. 31-32: Perhaps more accurate to say that relatively few measurements are available except in those species. Holmer&Hasler-Sheetal2014 (HHS) compiles TS/d34S data from many species, including 4 pubs with d34S data in Halodule wrightii (Hw). True, there are no d34S data in Hw rhizomes or TS/Fsulfide for any tissue for Hw. L.31-37, discussion/conclusion: Consider elaborating slightly on motivation and contributions of study. L.13-14,L.82-87: Could use context – as implied in L.94 but not cited, this pattern/process is expected in many seagrass species from data in HHS and elsewhere. L.80-81: Why not compare to the Hw-specific data in HHS? Also not sure I agree with “most”. Per HHS and Holmer&Kendrick2013, smaller species often exhibit greater belowground sulfide intrusion. Discussion: Any ideas why d34S but not TS can proxy for Fsulfide here, in contrast to HHS, Holmeretal.2009 (Thalassia, but see Syringodium), etc.? L.15-17,L.92: Perhaps “suggests” rather than “indicates”. Form and complete conversion cannot be determined from TS/d34S/Fsulfide. Figure2,text: Consider post-hoc tests; consider giving p-value as e.g.p<0.001 (2.2E-16 is the low default in base R); consider giving sample sizes on figure in addition to tables. General: Very minor proofs, e.g. BaSO4, Fsulfide formatting; define all scientific names and acronyms e.g. VCDT, IQR, Thalassia, Zostera.