Impact statement
Terrestrial large-bodied animals (megafauna) play important roles in ecosystems and human cultures. However, their diversity and abundance have declined severely across the last ~50,000 years. This late-Quaternary megafauna extinction pattern stands out from previous Cenozoic extinctions in three ways. (1) These losses were global and severe. (2) They were strongly biased toward larger-bodied species, with other organisms experiencing only very limited extinction in this period. Illustrating this pattern, only 11 out of 57 species of megaherbivores (mean body mass ≥1,000 kg) survived through to 1,000 AD. (3) This faunal simplification is unique on a ≥30-million-year time scale, with diverse megafauna guilds being the norm throughout this entire timeframe, excepting recent millennia. Further, temporal staggering is a defining feature of these losses, with extinctions concentrated in widely different time windows in different areas. The debate on the cause, or causes, of the late-Quaternary extinctions has been ongoing for over 200 years. Though most current work accepts at least a contributory role for modern humans, the topic remains controversial. We outline multiple characteristics of the late-Quaternary extinctions that, in order to merit support, any hypothesis needs to account for, and based thereon conclude the existing evidence strongly supports a dominant role of Homo sapiens and is inconsistent with climate as a substantial cause. We discuss the known and likely ecological consequences of the late-Quaternary megafauna extinctions, with the combined evidence indicating that the disappearance of so many large animal species constitutes a fundamental re-shaping of terrestrial ecosystem worldwide. Ecosystem effects can be grouped into trophic processes, physical environmental engineering and the transportation of energy and matter. Building thereon, we outline megafauna-based trophic rewilding as a key approach to restoration under global change. We also discuss the interplay of megafauna and human-driven biotic globalization and the ecological problems and potential in domestic megafauna, that is, livestock.
Introduction
There is a deep and ancient connection between humans and large animals (megafauna), which persists to this day (Berti and Svenning, Reference Berti and Svenning2020). This relationship has at times been adversarial, at others reverential, and at all times, due to the reliance of humans on animal-based resources, practical (e.g., Ben-Dor et al., Reference Ben-Dor, Gopher, Hershkovitz and Barkai2011; Ripple et al., Reference Ripple, Chapron, López-Bao, Durant, Macdonald, Lindsey, Bennett, Beschta, Bruskotter, Campos-Arceiz, Corlett, Darimont, Dickman, Dirzo, Dublin, Estes, Everatt, Galetti, Goswami, Hayward, Hedges, Hoffmann, Hunter, Kerley, Letnic, Levi, Maisels, Morrison, Nelson, Newsome, Painter, Pringle, Sandom, Terborgh, Treves, Valkenburgh, Vucetich, Wirsing, Wallach, Wolf, Woodroffe, Young and Zhang2016; Domínguez-Rodrigo et al., Reference Domínguez-Rodrigo, Baquedano, Organista, Cobo-Sánchez, Mabulla, Maskara, Gidna, Pizarro-Monzo, Aramendi, Galán, Cifuentes-Alcobendas, Vegara-Riquelme, Jiménez-García, Abellán, Barba, Uribelarrea, Martín-Perea, Diez-Martin, Maíllo-Fernández, Rodríguez-Hidalgo, Courtenay, Mora, Maté-González and González-Aguilera2021; Gaudzinski-Windheuser et al., Reference Gaudzinski-Windheuser, Kindler, MacDonald and Roebroeks2023; Smith et al., Reference Smith, Ruebens, Zavala, Sinet-Mathiot, Fewlass, Pederzani, Jaouen, Mylopotamitaki, Britton, Rougier, Stahlschmidt, Meyer, Meller, Dietl, Orschiedt, Krause, Schüler, McPherron, Weiss, Hublin and Welker2024). Respect for and value of the largest fauna by modern humans (Homo sapiens) is evident in prehistoric art across the world, which often predominantly depicts the largest animals that humans would have encountered (e.g., Malotki and Wallace, Reference Malotki and Wallace2011; Hussain and Floss, Reference Hussain and Floss2015; Zeller and Göttert, Reference Zeller and Göttert2021). Unfortunately, this importance to people would appear to have been historically detrimental, as the most massive animals consistently disappear following human arrival or intensified occupation (e.g., Martin, Reference Martin1967; Surovell et al., Reference Surovell, Waguespack and Brantingham2005; Koch and Barnosky, Reference Koch and Barnosky2006; Teng et al., Reference Teng, Xu, Teng and Svenning2020; Dembitzer et al., Reference Dembitzer, Barkai, Ben-Dor and Meiri2022). The ongoing loss of megafauna worldwide is not only a conservation issue but also an ecological issue, given the increasing evidence that large animals play important roles for biodiversity and in ecosystem functioning (e.g., Estes et al., Reference Estes, Terborgh, Brashares, Power, Berger, Bond, Carpenter, Essington, Holt, JBC, Marquis, Oksanen, Oksanen, Paine, Pikitch, Ripple, Sandin, Scheffer, Schoener, Shurin, ARE, Soulé, Virtanen and Wardle2011; Malhi et al., Reference Malhi, Doughty, Galetti, Smith, Svenning and Terborgh2016; Galetti et al., Reference Galetti, Moleón, Jordano, Pires, Guimarães, Pape, Nichols, Hansen, Olesen, Munk, de Mattos, Schweiger, Owen-Smith, Johnson, Marquis and Svenning2018; Enquist et al., Reference Enquist, Abraham, Harfoot, Malhi and Doughty2020). As such, there is an increasing focus on large animals in conservation, restoration and climate change mitigation and adaptation (e.g., Svenning et al., Reference Svenning, Pedersen, Donlan, Ejrnæs, Faurby, Galetti, Hansen, Sandel, Sandom, Terborgh and Vera2016, Reference Svenning, Buitenwerf and Le Roux2024; Cromsigt et al., Reference Cromsigt, te Beest, Kerley, Landman, le Roux and Smith2018; Malhi et al., Reference Malhi, Lander, le Roux, Stevens, Macias-Fauria, Wedding, Girardin, Kristensen, Sandom, Evans, Svenning and Canney2022). Despite this strong scientific interest in large-bodied animals, there is continued discussion around not just their role in the biosphere, but also their past and present relationship with humans.
One issue on which there is much discussion is the strong downsizing of terrestrial vertebrate assemblages across the last ~50,000 years, due to severe extinctions and extirpations of the larger species (Martin, Reference Martin1967; Smith et al., Reference Smith, Elliott Smith, Lyons and Payne2018). With the advent of 14C dating, it has become clear that Earth suffered widespread, severe extinctions among its terrestrial megafauna in recent prehistory, specifically during the Late Pleistocene (129,000–11,700 years BP) and Early to Middle Holocene (11,700–4,200 years BP), hereafter referred to as the late-Quaternary extinctions (e.g., Martin, Reference Martin1966). This pattern stands out from previous Cenozoic extinctions in three ways. First, the losses were global and severe: prior to this event, mainland faunas consistently harbored diverse assemblages of large mammals, while island systems had rich faunas of medium-large mammals, birds, and reptiles (e.g., Stuart, Reference Stuart2015). On the continents, communities shifted from highly diverse megafauna assemblages that included proboscideans and other megaherbivores (≥1,000 kg) as well as a range of megacarnivores (≥100 kg) to communities with few or no such species (e.g., Stuart, Reference Stuart2015; Malhi et al., Reference Malhi, Doughty, Galetti, Smith, Svenning and Terborgh2016). Second, other organisms were not similarly affected, with plants, invertebrates and small vertebrates experiencing only very limited extinction (e.g., Raffi et al., Reference Raffi, Stanley and Marasti1985; Stuart, Reference Stuart1991; Magri et al., Reference Magri, Di Rita, Aranbarri, Fletcher and González-Sampériz2017), the exception being megafauna-dependent organism groups such as scavenging birds and dung beetles (Galetti et al., Reference Galetti, Moleón, Jordano, Pires, Guimarães, Pape, Nichols, Hansen, Olesen, Munk, de Mattos, Schweiger, Owen-Smith, Johnson, Marquis and Svenning2018). Third, this simplification of the faunal community is unique on a 30-million-year or deeper time scale, with diverse megafauna guilds being the norm throughout this entire timeframe save for, depending on the region, the last 50,000–2,000 years (Smith et al., Reference Smith, Elliott Smith, Lyons and Payne2018). The cause of this extreme downsizing has been the subject of active study and debate since the 1960s (e.g., Martin, Reference Martin1966, Reference Martin1967; Koch and Barnosky, Reference Koch and Barnosky2006; Stuart, Reference Stuart2015). While there are multiple causal hypotheses, the emphasis has been on the evolution and expansion of modern humans (Homo sapiens) (Martin, Reference Martin1967; Sandom et al., Reference Sandom, Faurby, Sandel and Svenning2014a; Bartlett et al., Reference Bartlett, Williams, Prescott, Balmford, Green, Eriksson, Valdes, Singarayer and Manica2016; Araujo et al., Reference Araujo, Oliveira-Santos, Lima-Ribeiro, Diniz-Filho and Fernandez2017) and on climatic pressures associated with the last glacial–interglacial cycle (Cooper et al., Reference Cooper, Turney, Hughen, Brook, McDonald and Bradshaw2015; Carotenuto et al., Reference Carotenuto, Di Febbraro, Melchionna, Castiglione, Saggese, Serio, Mondanaro, Passaro, Loy and Raia2016; Stewart et al., Reference Stewart, Carleton and Groucutt2021).
Megafauna losses have continued up through the latter part of the Holocene to the present. Earth’s remaining megafauna are in quite a dire state, with ~47% of all living mammals weighing ≥10 kg listed as vulnerable, endangered or critically endangered by the International Union for the Conservation of Nature (IUCN), and an additional ~12% listed as near-threatened (calculated with PHYLACINE (Faurby et al., Reference Faurby, Davis, Pedersen, Schowanek, Antonelli and Svenning2018, Reference Faurby, Pedersen, Davis, Schowanek, Jarvie, Antonelli and Svenning2020a)). Defaunation is widespread and expanding in many parts of the Global South (Dirzo et al., Reference Dirzo, Young, Galetti, Ceballos, Isaac and Collen2014), often preceding habitat destruction. This is encapsulated by Empty Forest Syndrome, wherein forests are still standing but with major ecological dysfunction due to the extirpation or near-extirpation of their larger vertebrates (Redford, Reference Redford1992). Further, losses of megafauna in the most recent 1–5 millennia are widely reported, including severe declines in the ranges of many extant species. For example, China has seen strong range contractions in elephants (Elephas maximus), rhinoceroses (Dicerorhinus sumatrensis, Rhinoceros sondaicus) and tigers (Panthera tigris), as well as the global extinction of a water buffalo (Bubalus mephistopheles), an equid (Equus ovodovi) and a gibbon (Junzi imperialis) in recent millennia (Turvey et al., Reference Turvey, Bruun, Ortiz, Hansford, Hu, Ding, Zhang and Chatterjee2018; Teng et al., Reference Teng, Xu, Teng and Svenning2020). Similar declines in megafauna occurred in Europe (Crees and Turvey, Reference Crees and Turvey2014; Crees et al., Reference Crees, Carbone, Sommer, Benecke and Turvey2016) and the Middle East (Tsahar et al., Reference Tsahar, Izhaki, Lev-Yadun and Bar-Oz2009; Bar-Oz et al., Reference Bar-Oz, Zeder and Hole2011). The relationship between these losses and intensified human impact is widely accepted, yet classic conservation and restoration focus on a baseline set at or after 1500 CE, a time when ecosystems were already highly simplified or degraded (Martin, Reference Martin1967; Donlan et al., Reference Donlan, Berger, Bock, Bock, Burney, Estes, Foreman, Martin, Roemer, Smith, Soulé and Greene2006; Monsarrat and Svenning, Reference Monsarrat and Svenning2022).
The history of large-animal faunal dynamics is an increasingly practical consideration, both to inform and implement adequate conservation and restoration policies for endangered megafauna and to provide an informed basis for managing recovering and expanding populations of megafauna. An important counterpoint to widely continuing declines of large animals is the strong expansion of megafauna species observed in various, usually high-income regions. This dynamic is pronounced in Europe, reflecting increased societal tolerance, leading to expansions in most extant, wild-living megafauna species across recent decades (Ledger et al., Reference Ledger, Rutherford, Benham, Burfield, Deinet, Eaton, Freeman, Gray, Herrando and Puleston2022). This includes, for example, a >16,000% increase in Europe’s population of beaver (Castor fiber), a >300% increase in red deer (Cervus elaphus), a >300% increase in wild boar (Sus scrofa), a >16,000% in European bison (Bison bonasus) and a >1800% increase in gray wolf (Canis lupus) since 1960–1965 (Ledger et al., Reference Ledger, Rutherford, Benham, Burfield, Deinet, Eaton, Freeman, Gray, Herrando and Puleston2022). There have been similar expansions in North America, Japan and Australia (Kaji et al., Reference Kaji, Miyaki, Saitoh, Ono and Kaneko2000; Martin et al., Reference Martin, Chamaillé-Jammes and Waller2020; Read et al., Reference Read, Wilson, Coulson, Cooney, Paton, Moseby, Snape and Edwards2021). This recovery dynamic often includes alien megafauna as well (Lundgren et al., Reference Lundgren, Ramp, Ripple and Wallach2018), for example wild pigs (Sus scrofa) in much of the Americas (Vercauteren et al., Reference VerCauteren, Beasley, Ditchkoff, Mayer, Roloff and Strickland2020; Hegel et al., Reference Hegel, Faria, Ribeiro, Salvador, Rosa, Pedrosa, Batista, Sales, Wallau, Fornel and Aguiar2022). While these recoveries are receiving positive attention, they are also subject to controversy and calls for strong population control, as seen in debates around the overabundance of deer (Côté et al., Reference Côté, Rooney, Tremblay, Dussault and Waller2004; Martin et al., Reference Martin, Chamaillé-Jammes and Waller2020) and kangaroos (Read et al., Reference Read, Wilson, Coulson, Cooney, Paton, Moseby, Snape and Edwards2021), large carnivore comebacks (Martin et al., Reference Martin, Chamaillé-Jammes and Waller2020; von Hohenberg and Hager, Reference von Hohenberg and Hager2022), and alien ungulates (Lundgren et al., Reference Lundgren, Ramp, Ripple and Wallach2018). Finally, there is rising interest in the potential role of megafauna for ecosystem-level conservation and restoration efforts, notably in the concept of trophic rewilding (Svenning et al., Reference Svenning, Pedersen, Donlan, Ejrnæs, Faurby, Galetti, Hansen, Sandel, Sandom, Terborgh and Vera2016, Reference Svenning, Buitenwerf and Le Roux2024).
In this review, we appraise the current state of knowledge on the late-Quaternary extinctions, with focus on the patterns, drivers and consequences of megafauna disappearance as well as its relevancy for conservation and restoration. In palaeobiology, the definition of megafauna typically relies on a body weight threshold ≥45 kg, but other definitions and imprecise usages are common (Moleón et al., Reference Moleón, Sánchez-Zapata, Donázar, Revilla, Martín-López, Gutiérrez-Cánovas, Getz, Morales-Reyes, Campos-Arceiz, Crowder, Galetti, González-Suárez, He, Jordano, Lewison, Naidoo, Owen-Smith, Selva, Svenning, Tella, Zarfl, Jähnig, Hayward, Faurby, García, Barnosky and Tockner2020). Body mass is arguably the most important functional trait within animals, with strong effects on key aspects of their biology including life cycle, energy usage, and ecological impacts (e.g., Smith et al., Reference Smith, Payne, Heim, Balk, Finnegan, Kowalewski, Lyons, McClain, McShea and Novack-Gottshall2016; Enquist et al., Reference Enquist, Abraham, Harfoot, Malhi and Doughty2020; Fricke et al., Reference Fricke, Hsieh, Middleton, Gorczynski, Cappello, Sanisidro, Rowan, Svenning and Beaudrot2022). While the relationships between these factors and body mass are often non-linear, thresholds are mostly arbitrary and relative impacts can vary depending on biogeographic context with, for example, relatively small animals on isolated islands often functioning as megafauna (Hansen and Galetti, Reference Hansen and Galetti2009). Consequently, we use a broad definition of megafauna that generally includes all animal species with a typical adult body weight ≥10 kg, but with attention to body size effects and allowing for varying definitions between different studies. Furthermore, we focus on terrestrial megafauna, as Quaternary extinctions among marine megafauna are much fewer and clearly attributable to recent human impacts.
Extinction patterns
The broad-scale spatiotemporal patterns in the late-Quaternary extinctions are well described (Figure 1; e.g., Martin, Reference Martin1967; Koch and Barnosky, Reference Koch and Barnosky2006; Smith et al., Reference Smith, Elliott Smith, Lyons and Payne2018; Lemoine et al., Reference Lemoine, Buitenwerf and Svenning2023). Among terrestrial mammals, only 11 out of 57 species of megaherbivores (mean adult body mass ≥1,000 kg) survived through to 1,000 AD, that is, an 81% extinction rate (Table 1). The survivors include three species of elephant, four species of rhinoceros (the critically endangered fifth species, the Sumatran rhinoceros (Dicerorhinus sumatrensis), only has a 700–800 kg body mass), the common hippopotamus (Hippopotamus amphibius), and, at close to the megaherbivore size threshold, the giraffe (Giraffa camelopardalis s.l.) and two bovines. The extinct species include, for example, a variety of proboscideans and rhinoceroses, giant ground sloths and armadillos, rhinoceros-like toxodonts, and a giant marsupial. Many larger mesoherbivores (≥50 kg) also went extinct during this time including, for example, numerous equids, camelids, bovids, marsupials, ground sloths, and armadillos (Faurby et al., Reference Faurby, Davis, Pedersen, Schowanek, Antonelli and Svenning2018). Additionally, numerous large predators ≥50 kg went extinct, including all of the remaining saber-toothed cats (Smilodon spp., Homotherium latidens), steppe lions (Panthera atrox and P. spelaea), dire wolf (Aenocyon dirus), several bears, and a large hypercarnivorous marsupial (Thylacoleo carnifex) (Faurby et al., Reference Faurby, Davis, Pedersen, Schowanek, Antonelli and Svenning2018). Comparatively few smaller mammals went extinct during the late Quaternary, though non-insular exceptions in the 10–49 kg range include a collection of small ungulates (e.g., Antidorcas bondi), mid-sized marsupials and monotremes (e.g., Borungaboodie hatcheri, Megalibgwilia robustus), armadillos (e.g., Dasypus bellus), canids (e.g., Protocyon troglodytes), and large monkeys (e.g., Caipora bambuiorum) (Faurby et al., Reference Faurby, Davis, Pedersen, Schowanek, Antonelli and Svenning2018). Multiple large-scale extirpations also occurred among mammals ≥10 kg in the late Quaternary, with leopards (Panthera pardus) and dholes (Cuon alpinus) and several other species disappearing from Europe s.s. (Stuart, Reference Stuart2015; Taron et al., Reference Taron, Paijmans, Barlow, Preick, Iyengar, Drăgușin, Vasile, Marciszak, Roblíčková and Hofreiter2021). Extinctions in the 1–9 kg range were very few (Faurby et al., Reference Faurby, Davis, Pedersen, Schowanek, Antonelli and Svenning2018; Smith et al., Reference Smith, Elliott Smith, Lyons and Payne2018). The fragmented nature of the fossil record limits our ability to accurately estimate extinction rates with new species being discovered (Stinnesbeck et al., Reference Stinnesbeck, Frey, Olguín, Stinnesbeck, Zell, Mallison, González González, Aceves Núñez, Velázquez Morlet, Terrazas Mata, Benavente Sanvicente, Hering and Rojas Sandoval2017), shown to be invalid (Zurita et al., Reference Zurita, Gillette, Cuadrelli and Carlini2018), or identified in later time periods (Yang et al., Reference Yang, Li, Fostowicz-Frelik and Ni2019). Generally, however, these new findings show past extinction estimations to be too conservative. We also note the comparative ease of identifying extinctions in large mammals given their more robust bones, facilitating preservation. However, their relatively low population densities provide a counteracting effect. Due to its recency, the late-Quaternary inherently has a much more complete fossil record than earlier times, and its peculiar pattern of size-biased extinctions, relative to the rest of the Cenozoic, is very robust (e.g., Stuart, Reference Stuart1991; Smith et al., Reference Smith, Elliott Smith, Lyons and Payne2018).
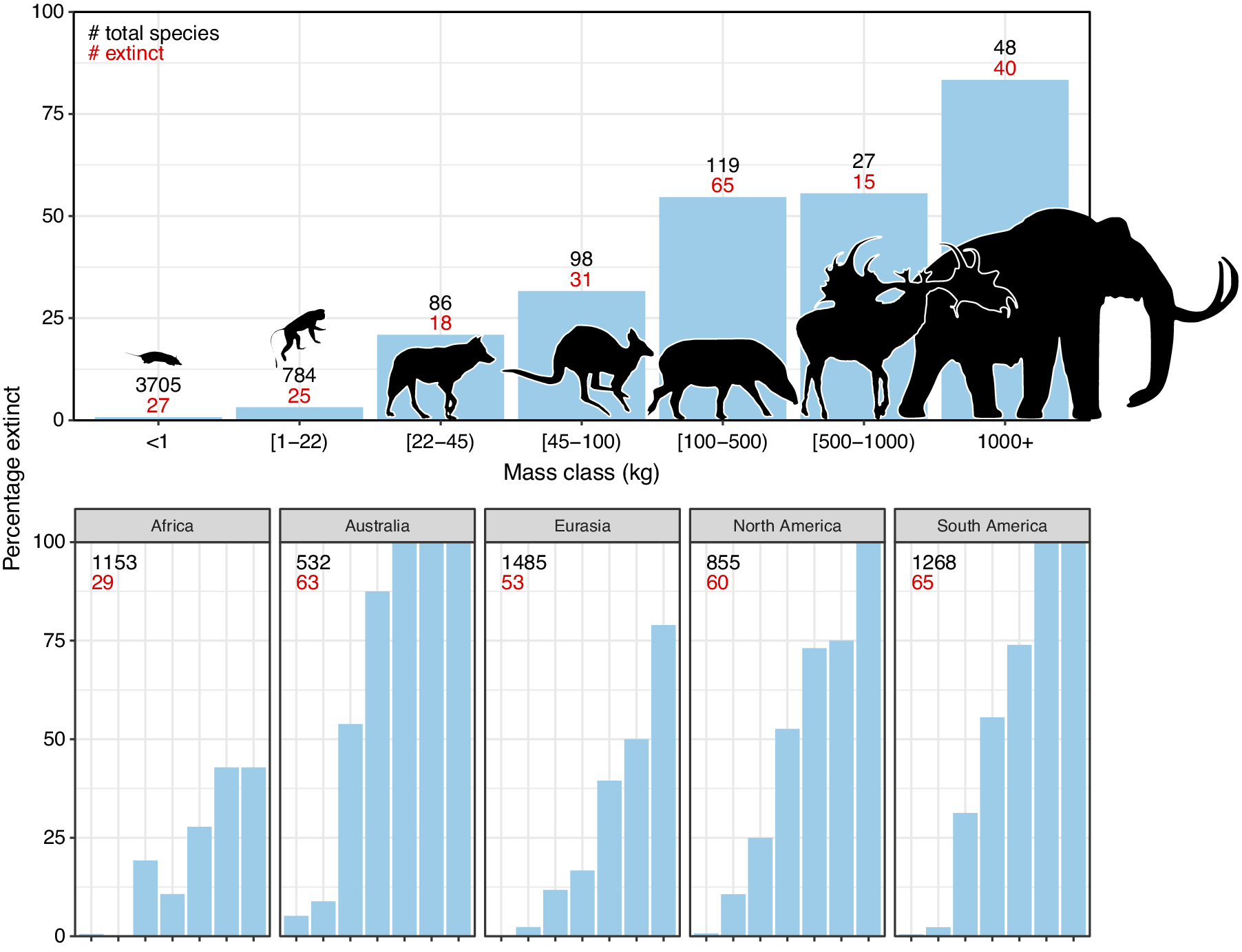
Figure 1. Late-Quaternary mammal extinctions as a function of body size. The global proportion of extinct species as a function of body size is shown at the top, and split per continent at the bottom. Black numbers are total late-Quaternary extant and extinct species counts, while red numbers are extinct species. We follow PHYLACINE 1.2.1 for mammal ranges and species list of all extant and extinct mammals throughout the last 129,000 years and include prehistorically extinct (EP), historically extinct (EX) and extinct in the wild (EW) as extinct. Continental extirpations are counted as extinctions in the bottom panels. The figure only includes non-marine species (i.e., sea cows, whales, seals, and marine otters are excluded), and also excludes humans (Homo spp.) and island endemics.
Table 1. Extant and extinct terrestrial megaherbivores (mean adult body weight ≥1,000 kg) from the late Quaternary

Note: Information from IUCN Red List (2023) for extant species and conservation status, and PHYLACINE 1.2.1 for extinct species (Faurby et al., Reference Faurby, Davis, Pedersen, Schowanek, Antonelli and Svenning2018, Reference Faurby, Pedersen, Davis, Schowanek, Jarvie, Antonelli and Svenning2020a), except as indicated in footnotes. IUCN Red List categories: CR, Critically Endangered; EN, Endangered; NT, Near Threatened; VU, Vulnerable. The table primarily follows the taxonomy in PHYLACINE 1.2.1 but deviates where the IUCN taxonomy has been updated since the latest PHYLACINE release, and where newer information is available for extinct species than PHYLACINE 1.2.1.
a All population sizes follow IUCN Red List (2023).
b Jukar et al. (Reference Jukar, Lyons, Wagner and Uhen2021).
c Body masses following Croft et al. (Reference Croft, Gelfo and López2020).
d Information from PHYLACINE 1.2.1 updated with McDonald (Reference McDonald2023).
e Loxodonta cyclotis is split from L. africana in latest IUCN taxonomy.
f Various Late Pleistocene populations of other Bovini species had populations with average adult body masses ≥1,000 kg, for example, Bison and Bos primigenius (e.g., Saarinen et al., Reference Saarinen, Eronen, Fortelius, Seppä and Lister2016).
g Multiple extinct llamas removed relative to PHYLACINE 1.2.1 due to updated body mass estimates.
h Family name following Delsuc et al. (Reference Delsuc, Gibb, Kuch, Billet, Hautier, Southon, Rouillard, Fernicola, Vizcaíno, MacPhee and Poinar2016).
i PHYLACINE 1.2.1 included Glyptotherium floridanum and G. cylindricum, but these taxa are now lumped (Zurita et al., Reference Zurita, Gillette, Cuadrelli and Carlini2018).
j All Palaeoloxodon species were listed as Elephas in PHYLACINE 1.2.1.
k Late Pleistocene Stegodon from India is often referred to a separate species, S. namadicus (Jukar et al., Reference Jukar, Lyons, Wagner and Uhen2021).
Extinctions in non-mammalian animals
In addition to mammalian megafauna, certain other groups suffered substantial extinctions during this period. These are mostly large-sized (≥10 kg body mass) or, if smaller, relatively large for their phylogenetic group, or species with links to megafauna, such as large dung beetles and a large vampire bat (Galetti et al., Reference Galetti, Moleón, Jordano, Pires, Guimarães, Pape, Nichols, Hansen, Olesen, Munk, de Mattos, Schweiger, Owen-Smith, Johnson, Marquis and Svenning2018; Tello et al., Reference Tello, Verdú, Rossini and Zunino2021). Among birds, multiple and mostly large to very large scavenging birds from the Americas (Galetti et al., Reference Galetti, Moleón, Jordano, Pires, Guimarães, Pape, Nichols, Hansen, Olesen, Munk, de Mattos, Schweiger, Owen-Smith, Johnson, Marquis and Svenning2018; Jones et al., Reference Jones, Rinderknecht, Alvarenga, Montenegro and Ubilla2018) and the only known vulture from Australia (Mather et al., Reference Mather, Lee and Worthy2022) went extinct. Also affected were various giant flightless birds such as an Asiatic ostrich (Pachystruthio anderssoni) (Buffetaut, Reference Buffetaut2023), the Australian mihirung (Genyornis newtoni) (Demarchi et al., Reference Demarchi, Stiller, Grealy, Mackie, Deng, Gilbert, Clarke, Legendre, Boano, Sicheritz-Pontén, Magee, Zhang, Bunce, Collins and Miller2022), and a late-surviving terror-bird from southern South America (Psilopterus sp.) (Jones et al., Reference Jones, Rinderknecht, Alvarenga, Montenegro and Ubilla2018). Other more moderately large birds went extinct on the continents as well, including an endemic Californian turkey (Meleagris californica) and a large coot (Bochenski and Campbell, Reference Bochenski and Campbell2006; Alarcón-Muñoz et al., Reference Alarcón-Muñoz, Labarca and Soto-Acuña2020). A large number of bird extinctions occurred on islands, biased toward larger and flightless species (Fromm and Meiri, Reference Fromm and Meiri2021), for example, huge fowl on Fiji and New Caledonia (Sylviornithidae) (Worthy et al., Reference Worthy, Mitri, Handley, Lee, Anderson and Sand2016), and truly giant flightless elephant birds (Aepyornithiformes) and moas (Dinornithiformes) on Madagascar and New Zealand, respectively (e.g., Koch and Barnosky, Reference Koch and Barnosky2006; Grealy et al., Reference Grealy, Miller, Phillips, Clarke, Fogel, Patalwala, Rigby, Hubbard, Demarchi, Collins, Mackie, Sakalauskaite, Stiller, Clarke, Legendre, Douglass, Hansford, Haile and Bunce2023). Among reptiles, a number of giant tortoises went extinct on the mainland during the Late Pleistocene with additional species on islands from this time onward (Rhodin et al., Reference Rhodin, Thomson, Georgalis, Karl, Danilov, Takahashi, de la Fuente, Bourque, Delfino, Bour, Iverson, Shaffer and van Dijk2015). In addition, multiple crocodiles (Ristevski et al., Reference Ristevski, Willis, Yates, White, Hart, Stein, Price and Salisbury2023), large monitor lizards (Varanus spp.) (Hocknull et al., Reference Hocknull, Piper, Van den Bergh, Due, Morwood and Kurniawan2009), a large snake (Wonambi naracoortensis), and a giant armored lizard (Thorn et al., Reference Thorn, Fusco, Hutchinson, Gardner, Clayton, Prideaux and Lee2023) went extinct in Australasia (Palci et al., Reference Palci, Hutchinson, Caldwell, Scanlon and Lee2018), and an enormous freshwater turtle disappeared in Amazonia (Ferreira et al., Reference Ferreira, Nascimento, Cadena, Cozzuol, Farina, Pacheco, Rizzutto and Langer2024).
Geographic patterns
The prehistoric late-Quaternary extinctions exhibit well-documented geographic contrasts, with moderate extinctions in the Afrotropics and Indomalaya, more severe extinctions in the Palaearctic, even more severe extinctions in the Nearctic and Neotropics, and near-total loss in Australasia (e.g., Martin, Reference Martin1966, Reference Martin1967; Koch and Barnosky, Reference Koch and Barnosky2006; Stuart, Reference Stuart2015; Figure 1). Importantly, there is a strong size basis in these extinctions even in the lesser affected regions (Figure 1). Further, many island environments also experienced total to near-total extinctions of their larger native fauna within this period or later (Stuart, Reference Stuart2015). Sub-Saharan Africa is often presented as having an intact megafauna, but actually lost a number of species in the late Quaternary, including an elephant species (Palaeoloxodon iolensis), a giant buffalo (Pelorovis antiquus), various antelopes (e.g., Rusingoryx atopocranion) and a giant warthog (Metridiochoerus compactus) (Faith, Reference Faith2014; Manthi et al., Reference Manthi, Sanders, Plavcan, Cerling and Brown2020; Kovarovic et al., Reference Kovarovic, Faith, Jenkins, Tryon and Peppe2021). Similarly, mainland southern Asia also lost an elephant (Palaeoloxodon namadicus), another proboscidean (Stegodon orientalis), a hippopotamus (Hexaprotodon sivalensis), an equid (Equus namadicus), several orangutans (Pongo spp.), and a giant tapir (Tapirus augustus), while the Indian aurochs (Bos primigenius namadicus) only survived in domesticated form (B. p. indicus) (Bacon et al., Reference Bacon, Westaway, Antoine, Duringer, Blin, Demeter, Ponche, Zhao, Barnes, Sayavonkhamdy, Thuy, Long, Patole-Edoumba and Shackelford2015; Jukar et al., Reference Jukar, Lyons, Wagner and Uhen2021). Northern Eurasia and North Africa also lost substantial numbers of megafauna during the Late Pleistocene and through the Holocene, for example, multiple species of elephant, rhinoceros (including the giant Elasmotherium sibiricum) and giant deer (e.g., Megaloceros giganteus), with the aurochs (B. primigenius primigenius) only surviving in domesticated form (B. p. taurus) (Faith, Reference Faith2014; Stuart, Reference Stuart2015; van der Plicht et al., Reference van der Plicht, Molodin, Kuzmin, Vasiliev, Postnov and Slavinsky2015; Kosintsev et al., Reference Kosintsev, Mitchell, Devièse, van der Plicht, Kuitems, Petrova, Tikhonov, Higham, Comeskey, Turney, Cooper, van Kolfschoten, Stuart and Lister2019). North and South America experienced more severe losses, including all proboscideans, ground sloths, glyptodonts, the endemic ungulate orders (Notoungulata, Litopterna), equids, and all but two camelids (e.g., Stuart, Reference Stuart2015). Australia lost almost all of its megafauna, with the complete extinction of all terrestrial species ≥50 kg, which included multiple species of short-faced kangaroo and several giant wombat relatives (Stuart, Reference Stuart2015). Extinctions of smaller megafauna occurred on essentially all major island systems at different times over the Late Pleistocene to the Late Holocene (e.g., Koch and Barnosky, Reference Koch and Barnosky2006; Slavenko et al., Reference Slavenko, Tallowin, Itescu, Raia and Meiri2016; Andermann et al., Reference Andermann, Faurby, Turvey, Antonelli and Silvestro2020; Fromm and Meiri, Reference Fromm and Meiri2021).
Megafauna losses occurred in a broad variety of biomes (Figure 2). Extinctions from cold, northerly biomes are well-documented, notably woolly mammoth (Mammuthus primigenius) and woolly rhinoceros (Coelodonta antiquitatis), alongside the extirpation of muskoxen (Ovibos moschatus) from Eurasia (e.g., Lorenzen et al., Reference Lorenzen, Nogués-Bravo, Orlando, Weinstock, Binladen, Marske, Ugan, Borregaard, Gilbert, Rs, Ho, Goebel, Graf, Byers, Stenderup, Rasmussen, Campos, Leonard, Koepfli, Froese, Zazula, Stafford, Aaris-Sorensen, Batra, Haywood, Singarayer, Valdes, Boeskorov, Burns, Davydov, Haile, Jenkins, Kosintsev, Kuznetsova, Lai, Martin, McDonald, Mol, Meldgaard, Munch, Stephan, Sablin, Sommer, Sipko, Scott, Suchard, Tikhonov, Willerslev, Wayne, Cooper, Hofreiter, Sher, Shapiro, Rahbek and Willerslev2011; Stuart, Reference Stuart2015). However, these species represent only very few of 134 known late-Quaternary extinctions among terrestrial mammal species ≥100 kg average adult body mass (Figure 1), hereunder 14 island endemics. The remainder occurred in tropical to temperate climates in ecosystems ranging from dense forests to open woodlands and savannahs to grasslands and deserts, and extinction occurred with similar severity across biomes (Figure 2). Importantly, a large proportion of the extinct species were clearly generalist in terms of climate, habitat, and diet (e.g., Price, Reference Price2008; França et al., Reference França, de Asevedo, Dantas, Bocchiglieri, Avilla, Lopes and da Silva2015; van Asperen and Kahlke, Reference van Asperen and Kahlke2015; Rivals et al., Reference Rivals, Semprebon and Lister2019). Even woolly mammoth and woolly rhinoceros occasionally occurred in temperate or boreal settings during the Late Pleistocene, for example in Iberia (Álvarez-Lao and García, Reference Álvarez-Lao and García2012) and north-eastern China (Ma et al., Reference Ma, Wang, Baryshnikov, Drucker, McGrath, Zhang, Bocherens and Hu2021).

Figure 2. Late-Quaternary mammal extinction as a function of body size and current biome. Black numbers are total late-Quaternary extant and extinct species counts, while red numbers are extinct species. The shaded part of the bars is the extinction fraction if we exclude Africa from the analysis – to illustrate how much its relatively numerous remaining megafauna influence the patterns. We follow PHYLACINE 1.2.1 for present-natural and current species ranges and the species list of all extant and extinct mammals throughout the last 129,000 years, counting species as extinct in a biome if it no longer occurs there. The figure only includes non-marine species (i.e., with sea cows, whales, seals, and marine otters excluded), and also excludes humans (Homo spp.) and island endemics. For biomes, we follow the Terrestrial Ecoregions of the World by the World Wildlife Fund.
Temporal patterns
Temporal staggering is a defining feature of the late-Quaternary megafauna extinctions, as already noted by Martin (Reference Martin1967), with extinctions concentrated in different time windows in different areas, extending from ~50,000 years ago until well into the Holocene, and often spread across thousands of years even within a given region. The extinctions of megafauna in Australia and New Guinea primarily occurred between 60–40 kya, whereas extinctions in the Americas began roughly 20–15 kya (e.g., Stuart, Reference Stuart2015) and continued until as late as 7–5 kya (Prado et al., Reference Prado, Martinez-Maza and Alberdi2015; Murchie et al., Reference Murchie, Monteath, Mahony, Long, Cocker, Sadoway, Karpinski, Zazula, MacPhee, Froese and Poinar2021). Extinctions in Eurasia occurred in different regions at different times during this 60–5 ky span (Stuart, Reference Stuart2015). Megafauna extinctions on islands exhibited similar staggering, albeit tending to happen later, from the end of the Pleistocene onward. Examples include Japan (25,000–16,000 ya) (Iwase et al., Reference Iwase, Hashizume, Izuho, Takahashi and Sato2012), the California Channel Islands (~13,000 ya) (Rick et al., Reference Rick, Hofman, Braje, Maldonado, Sillett, Danchisko and Erlandson2012), Sardinia (~7,500 ya) (Benzi et al., Reference Benzi, Abbazzi, Bartolomei, Esposito, Fassò, Fonzo, Giampieri, Murgia and Reyss2007), the Antilles (~4,000 ya) (Steadman et al., Reference Steadman, Martin, MacPhee, Jull, McDonald, Woods, Iturralde-Vinent and Hodgins2005), New Caledonia (~3,000 ya) (Anderson et al., Reference Anderson, Sand, Petchey and Worthy2010), Madagascar (~1,000 ya) (Hansford et al., Reference Hansford, Lister, Weston and Turvey2021), and New Zealand (~600 ya) (Perry et al., Reference Perry, Wheeler, Wood and and Wilmshurst2014). A notable example of survival deep into the Holocene is the widespread survival of woolly mammoth (Mammuthus primigenius) until the Middle Holocene in northern parts of continental Siberia and North America (Murchie et al., Reference Murchie, Monteath, Mahony, Long, Cocker, Sadoway, Karpinski, Zazula, MacPhee, Froese and Poinar2021; Wang et al., Reference Wang, Pedersen, Alsos, De Sanctis, Racimo, Prohaska, Coissac, Owens, Merkel, Fernandez-Guerra, Rouillard, Lammers, Alberti, Denoeud, Money, Ruter, McColl, Larsen, Cherezova, Edwards, Fedorov, Haile, Orlando, Vinner, Korneliussen, Beilman, Bjørk, Cao, Dockter, Esdale, Gusarova, Kjeldsen, Mangerud, Rasic, Skadhauge, Svendsen, Tikhonov, Wincker, Xing, Zhang, Froese, Rahbek, Nogues, Holden, Edwards, Durbin, Meltzer, Kjær, Möller and Willerslev2021). Similar late survival in Siberia is also noted for woolly rhinoceros (Coelodonta antiquitatis), steppe bison (Bison priscus), giant deer (Megaloceros giganteus), and the extant muskox (Ovibos moschatus) (van der Plicht et al., Reference van der Plicht, Molodin, Kuzmin, Vasiliev, Postnov and Slavinsky2015; Plasteeva et al., Reference Plasteeva, Gasilin, Devjashin and Kosintsev2020; Wang et al., Reference Wang, Pedersen, Alsos, De Sanctis, Racimo, Prohaska, Coissac, Owens, Merkel, Fernandez-Guerra, Rouillard, Lammers, Alberti, Denoeud, Money, Ruter, McColl, Larsen, Cherezova, Edwards, Fedorov, Haile, Orlando, Vinner, Korneliussen, Beilman, Bjørk, Cao, Dockter, Esdale, Gusarova, Kjeldsen, Mangerud, Rasic, Skadhauge, Svendsen, Tikhonov, Wincker, Xing, Zhang, Froese, Rahbek, Nogues, Holden, Edwards, Durbin, Meltzer, Kjær, Möller and Willerslev2021). East Asian examples include the non-caballine horse Equus ovodovi, a temperate water buffalo (Bubalus mephistopheles), and the ostrich Pachystruthio anderssoni (Janz et al., Reference Janz, Elston and Burr2009; Turvey et al., Reference Turvey, Bruun, Ortiz, Hansford, Hu, Ding, Zhang and Chatterjee2018; Cai et al., Reference Cai, Zhu, Gong, Zhang, Wen, Liang, Sun, Shao, Guo, Cai, Zheng, Zhang, Hu, Wang, Tian, Li, Liu, Yang, Yang, Wu, Orlando and Jiang2022). Other examples include European wild ass (Equus hydruntinus) (Crees and Turvey, Reference Crees and Turvey2014), giant buffalo (Pelorovis antiquus) in Africa (Faith, Reference Faith2014), the extant wild horse (Equus ferus) in North America (Murchie et al., Reference Murchie, Monteath, Mahony, Long, Cocker, Sadoway, Karpinski, Zazula, MacPhee, Froese and Poinar2021), and various megafauna species in South America, for example the proboscidean Notiomastodon platensis (Dantas et al., Reference Dantas, Liparini, Asevedo, LdM and Cherkinsky2022), the large ground sloth (Scelidotherium leptocephalum), and a giant armadillo Eutatus seguini (Prado et al., Reference Prado, Martinez-Maza and Alberdi2015). Some species that were more widespread in the Pleistocene or Early Holocene had their last stands in historic times, for example Steller’s sea cow (Hydrodamalis gigas) and a large, near-flightless cormorant (Urile perspicillatus), both of which ranged across the North Pacific during the Pleistocene and earlier Holocene (Crerar et al., Reference Crerar, Crerar, Domning and Parsons2014; Watanabe et al., Reference Watanabe, Matsuoka and Hasegawa2018). Tasmania held the last thylacines (Thylacinus cynocephalus) until European colonization, and still holds the last devils (Sarcophilus harrisii) and flightless nativehens (Tribonyx mortierii), with the disappearance of all three species in mainland Australia coinciding with the human introduction of dingoes (Canis lupus dingo) (Letnic et al., Reference Letnic, Fillios and Crowther2012).
A few species of megafauna went extinct in their originally wild form in the Late Holocene or recorded history, but survive as domesticates, such as cattle (B. primigenius taurus and B. primigenius indicus) (Ajmone-Marsan et al., Reference Ajmone-Marsan, Garcia and Lenstra2010), and one or both species of domestic camels (Camelus bactrianus and C. dromedarius) (Fitak et al., Reference Fitak, Mohandesan, Corander, Yadamsuren, Chuluunbat, Abdelhadi, Raziq, Nagy, Walzer, Faye and Burger2020). The historical record also documents many instances of Holocene range declines in extant megafauna species, for example, in the Middle East (Tsahar et al., Reference Tsahar, Izhaki, Lev-Yadun and Bar-Oz2009; Bar-Oz et al., Reference Bar-Oz, Zeder and Hole2011), China (Turvey et al., Reference Turvey, Crees, Li, Bielby and Yuan2017, Reference Turvey, Bruun, Ortiz, Hansford, Hu, Ding, Zhang and Chatterjee2018; Teng et al., Reference Teng, Xu, Teng and Svenning2020), and Europe (Crees et al., Reference Crees, Carbone, Sommer, Benecke and Turvey2016). A recent study on 139 species of extant terrestrial megafauna mammals from all continents (except Antarctica) using analyses of current genomes to estimate past demographics shows strong prehistoric population declines in >90% of these species and no significant increases (Bergman et al., Reference Bergman, Pedersen, Lundgren, Lemoine, Monsarrat, Pearce, Schierup and Svenning2023; Figure 3), in line with earlier studies on specific megafauna groups, for example rhinoceroses (Liu et al., Reference Liu, Westbury, Dussex, Mitchell, Sinding, Heintzman, Duchêne, Kapp, von Seth, Heiniger, Sánchez-Barreiro, Margaryan, André-Olsen, De Cahsan, Meng, Yang, Chen, van der Valk, Moodley, Rookmaaker, Bruford, Ryder, Steiner, Bruins-van Sonsbeek, Vartanyan, Guo, Cooper, Kosintsev, Kirillova, Lister, Marques-Bonet, Gopalakrishnan, Dunn, Lorenzen, Shapiro, Zhang, Antoine, Dalén and Gilbert2021) and elephants (Palkopoulou et al., Reference Palkopoulou, Lipson, Mallick, Nielsen, Rohland, Baleka, Karpinski, Ivancevic, To, Kortschak, Raison, Qu, Chin, Alt, Claesson, Dalén, MacPhee, Meller, Roca, Ryder, Heiman, Young, Breen, Williams, Aken, Ruffier, Karlsson, Johnson, Di Palma, Alfoldi, Adelson, Mailund, Munch, Lindblad-Toh, Hofreiter, Poinar and Reich2018). According to breakpoint analyses, these declines started at 76,000–32,000 years ago, at different times in different realms, and their severity increased with body mass (Bergman et al., Reference Bergman, Pedersen, Lundgren, Lemoine, Monsarrat, Pearce, Schierup and Svenning2023). Furthermore, many of the surviving megafauna species exhibit body size declines across the Late Pleistocene and Holocene. American bison (Bison bison), for example, had an average body size in the Late Pleistocene that was 37% greater than today (Martin et al., Reference Martin, Mead and Barboza2018). Other examples include jaguars (Panthera onca) in North and South America (Srigyan et al., Reference Srigyan, Schubert, Bushell, Santos, Figueiró, Sacco, Eizirik and and Shapiro2023), coyotes (Canis latrans) in North America (Meachen et al., Reference Meachen, Janowicz, Avery and Sadleir2014), European brown bears (Ursus arctos) (Marciszak et al., Reference Marciszak, Stefaniak, Mackiewicz and Ridush2015), European wild horse (E. ferus) (Forsten, Reference Forsten1993), and various kangaroos and other larger Australian marsupials (Marshall and Corruccini, Reference Marshall and Corruccini1978). Hence, we can generalize the prehistoric dynamics of surviving megafauna as a series of size-biased declines similar to the extinction patterns.

Figure 3. Genomic analyses show that surviving large mammals experienced strong population declines across the late Quaternary, in parallel to the global extinctions of many megafauna species. Effective population size dynamics were inferred from the whole genome nucleotide diversity of 139 terrestrial mammals (all >10 kg body mass) using the Pairwise Sequentially Markovian Coalescent method, adapted from Bergman et al. (Reference Bergman, Pedersen, Lundgren, Lemoine, Monsarrat, Pearce, Schierup and Svenning2023). Each gray step line represents a population size trajectory of a single species with the average population trend for each continent depicted in color. Both axes are log10-transformed.
Just as historic, Holocene, and Late Pleistocene extinction patterns can be difficult to separate, the Late Pleistocene extinctions also grade into earlier losses in some instances. There is evidence that megafauna extinctions without replacement and with a tendency towards body-size downgrading started somewhat earlier than the Late Pleistocene in some regions, specifically Africa and Eurasia (e.g., Martin, Reference Martin1966). The large carnivore guild in Sub-Saharan Africa underwent a drastic simplification including the loss of all machairodont cats already in the Early Pleistocene (Geraads, Reference Geraads, Gallotti and Mussi2018; Faurby et al., Reference Faurby, Silvestro, Werdelin and Antonelli2020b). Machairodont cats also went extinct or became rare (Homotherium latidens) in Eurasia from the early or middle Middle Pleistocene onward (Antón et al., Reference Antón, Galobart and Turner2005). Megaherbivores declined in diversity in Africa from the Early Pleistocene onward (Faith, Reference Faith2014; Bibi and Cantalapiedra, Reference Bibi and Cantalapiedra2023). More generally, a variety of large herbivores also went extinct before the Late Pleistocene seemingly without replacement in both Africa and southern Asia, as already noted by Martin (Reference Martin1966, Reference Martin1967), although this is not always clearcut to discern. Potential examples include the proboscidean Deinotherium, chalicotheres, robust giraffes (Sivatherium giganteum), the giant geleda Theropithecus oswaldi, and the giant terrestrial ape Gigantopithecus blacki (Surovell et al., Reference Surovell, Waguespack and Brantingham2005; Cerling et al., Reference Cerling, Andanje, Blumenthal, Brown, Chritz, Harris, Hart, Kirera, Kaleme, Leakey, Leakey, Levin, Manthi, Passey and Uno2015; Yan et al., Reference Yan, Wang, Zhu, Chen, Qin and Jin2016; Geraads, Reference Geraads, Gallotti and Mussi2018; Zhang et al., Reference Zhang, Westaway, Haberle, Lubeek, Bailey, Ciochon, Morley, Roberts, J-x, Duval, Dosseto, Pan, Rule, Liao, Gully, Lucas, Mo, Yang, Cai, Wang and Joannes-Boyau2024). A large beaver (Trogontherium cuvieri) also disappears from most of Eurasia at the same time (Yang et al., Reference Yang, Li, Fostowicz-Frelik and Ni2019). In Sub-Saharan Africa, these losses were strong enough to make large-herbivore faunas from 700,000 years ago and earlier functionally non-analog to extant faunas due to a greater richness of non-ruminants and megaherbivores (Faith et al., Reference Faith, Rowan, Du and Koch2018; also see Bibi and Cantalapiedra, Reference Bibi and Cantalapiedra2023).
Other potential patterns
While there is evidence of co-extinctions in non-megafauna species dependent on large vertebrates (Galetti et al., Reference Galetti, Moleón, Jordano, Pires, Guimarães, Pape, Nichols, Hansen, Olesen, Munk, de Mattos, Schweiger, Owen-Smith, Johnson, Marquis and Svenning2018), the possibility of co-extinction among megafauna merits consideration as well (e.g., Owen-Smith, Reference Owen-Smith1987). Extinction of the largest herbivores capable of preventing the dominance by woody plants or coarse grasses would have been to the disadvantage of smaller herbivores due to less nutrient-rich and spatially diverse vegetation (Owen-Smith, Reference Owen-Smith1987; also cf. Trepel et al., Reference Trepel, le Roux, Abraham, Buitenwerf, Kamp, Kristensen, Tietje, Lundgren and Svenning2024). In line with that possibility, several small, grassland-associated gazelles disappear in Africa (Faith, Reference Faith2014). Multiple pronghorns, including small and very small species, similarly went extinct in North America (e.g., Bravo-Cuevas et al., Reference Bravo-Cuevas, Jiménez-Hidalgo, Cabral-Perdomo and Priego Vargas2013), and the extant saiga (Saiga tatarica), a small antelope associated with open habitats, disappears from much of its Holarctic range (Jürgensen et al., Reference Jürgensen, Drucker, Stuart, Schneider, Buuveibaatar and Bocherens2017). Small, ruminant grazers require high-quality grasses and forbs, the maintenance of which often requires larger herbivores to remove more low-quality vegetation, as seen in the relationship between Thomson’s gazelle (Eudorcas thomsonii) and larger grazers like zebra (Equus quagga) and wildebeest (Connochaetes taurinus) in East Africa (Bell, Reference Bell1971; Anderson et al., Reference Anderson, Hepler, Holdo, Donaldson, Erhardt, Hopcraft, Hutchinson, Huebner, Morrison, Muday, Munuo, Palmer, Pansu, Pringle, Sketch and Packer2024).
Extinction drivers
The debate on the cause or causes of the late-Quaternary extinctions has been ongoing for over 200 years, but with greater rigor and focus from the 1960s onward (Martin, Reference Martin1967; Koch and Barnosky, Reference Koch and Barnosky2006). Though most current work accepts at least a contributory role for modern humans, the topic remains controversial. Competing alternative theories include an extra-terrestrial impact (reviewed and rejected by Holliday et al., Reference Holliday, Daulton, Bartlein, Boslough, Breslawski, Fisher, Jorgeson, Scott, Koeberl, Marlon, Severinghaus, Petaev and Claeys2023) and, more credibly, climate change.
To evaluate support for different drivers, there are a number of characteristics of the late-Quaternary extinctions that any hypothesis needs to account for. Firstly, the late-Quaternary extinction was a global event (Figure 1), largely constrained to the Last Glaciation and the Holocene, and unique for the whole of the Cenozoic (Smith et al., Reference Smith, Elliott Smith, Lyons and Payne2018; Figure 4). It occurred in all climate zones, with the majority of extinctions happening among temperate to tropical species (Figure 2). The late-Quaternary extinctions were extremely size-selective, with high and positively size-dependent extinction rates among large terrestrial vertebrates and very limited extinctions within the same time frame in smaller-sized terrestrial animals, marine vertebrates of any size, or plants (e.g., Smith et al., Reference Smith, Elliott Smith, Lyons and Payne2018). Further, the extinct species come from a large number of mammal families and orders and extend to a variety of only distantly related birds and reptiles. Additionally, severe extinctions penetrated to smaller animals on islands, but still with a bias toward the largest species there (e.g., Fromm and Meiri, Reference Fromm and Meiri2021). Finally, the extinctions were concentrated in different time windows in different continents, regions and islands, and extended from, for example, as early as ~50,000 years ago to the Middle and even late Holocene (e.g., Crees and Turvey, Reference Crees and Turvey2014; van der Plicht et al., Reference van der Plicht, Molodin, Kuzmin, Vasiliev, Postnov and Slavinsky2015; Andermann et al., Reference Andermann, Faurby, Turvey, Antonelli and Silvestro2020; Cai et al., Reference Cai, Zhu, Gong, Zhang, Wen, Liang, Sun, Shao, Guo, Cai, Zheng, Zhang, Hu, Wang, Tian, Li, Liu, Yang, Yang, Wu, Orlando and Jiang2022). These patterns mean that we can immediately discard explanations that do not have global scope or that hinge on a particular event such as a late-glacial extra-terrestrial impact or the loss of a specific ecosystem type like the mammoth steppe. Importantly, while detailed studies of range dynamics in single extinct species are valuable, explanatory models are only tenable if we can generalize them to the broader late-Quaternary extinct event. This leaves two broad potential drivers for serious consideration, namely the spread and cultural evolution of Homo sapiens and climate change during the late Quaternary. The possibility also exists for interaction between the two (e.g., Koch and Barnosky, Reference Koch and Barnosky2006). Here, we first discuss the evidence with respect to a climatic cause, and then with respect to Homo sapiens.
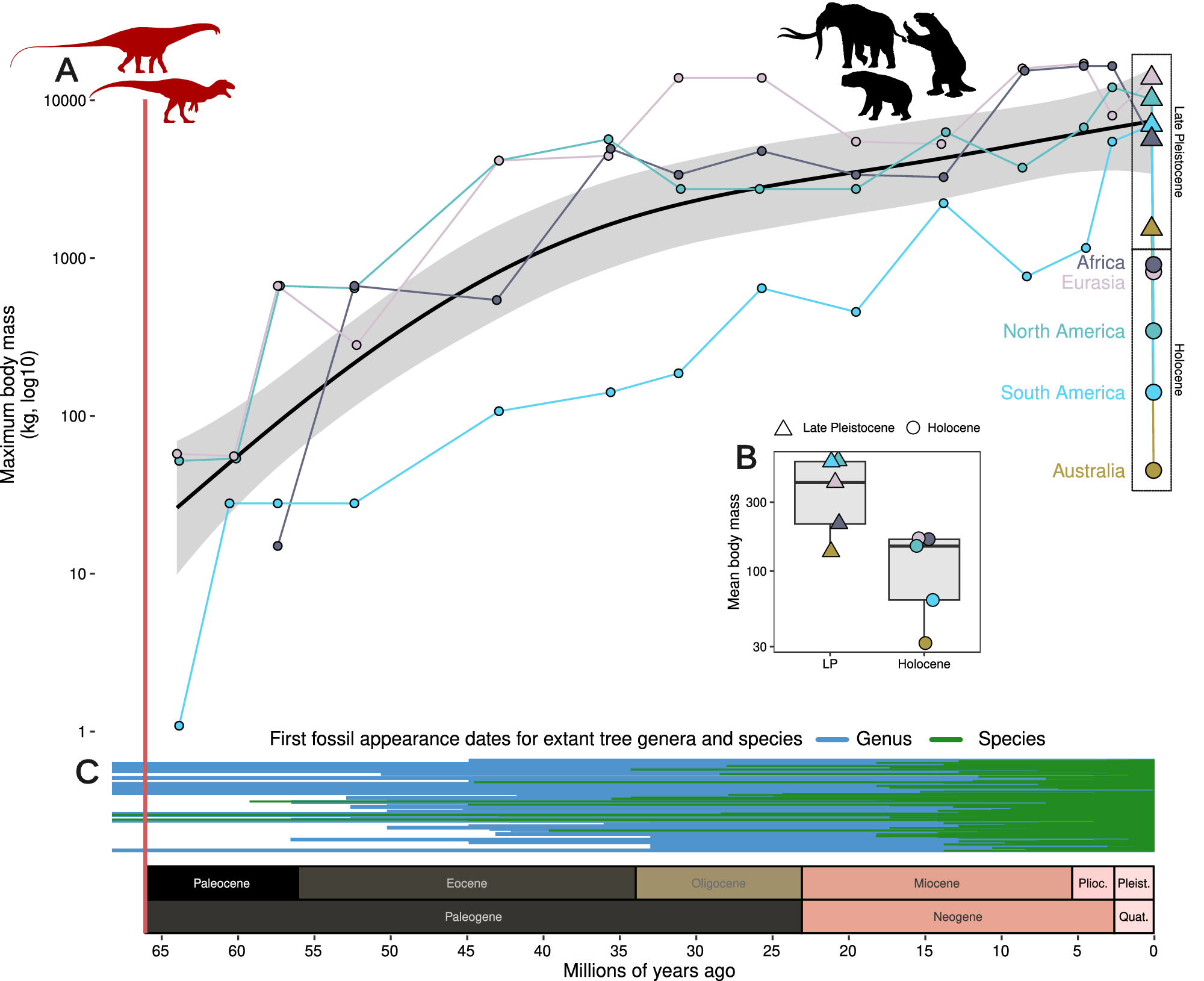
Figure 4. Mammalian body masses through the Cenozoic. (A) Maximum mammalian body mass (kg, log10 scale) increased steadily following the end-Mesozoic mass extinction (vertical red line) until the Late Pleistocene, but then declines precipitously. The X-axis indicates millions of years ago and is shared across panels A and C with geologic periods and epochs illustrated above axis text. Black line with gray error indicates Generalized Additive Model estimate across maximum values for four continents (data unavailable for Australia). Colored lines indicate the separate continental maximum body mass through time. Declines from the Late Pleistocene to the Holocene are highlighted with dashed boxes. (B) Mean body mass per continent during the Late Pleistocene and Holocene, after the megafauna extinctions. Data for A and B are from Smith et al. (Reference Smith, Elliott Smith, Lyons and Payne2018). (C) Megafauna was a pervasive presence during the evolution of modern-day terrestrial biota, here illustrated with first appearance dates of extant tree genera (blue) and species (green), shown as lines from the first fossil record to today (data from Paleodb, accessed 2023).
Climatic causation
The Late Pleistocene saw intense climatic shifts, which are often implicated as drivers of megafauna extinctions (e.g., Lorenzen et al., Reference Lorenzen, Nogués-Bravo, Orlando, Weinstock, Binladen, Marske, Ugan, Borregaard, Gilbert, Rs, Ho, Goebel, Graf, Byers, Stenderup, Rasmussen, Campos, Leonard, Koepfli, Froese, Zazula, Stafford, Aaris-Sorensen, Batra, Haywood, Singarayer, Valdes, Boeskorov, Burns, Davydov, Haile, Jenkins, Kosintsev, Kuznetsova, Lai, Martin, McDonald, Mol, Meldgaard, Munch, Stephan, Sablin, Sommer, Sipko, Scott, Suchard, Tikhonov, Willerslev, Wayne, Cooper, Hofreiter, Sher, Shapiro, Rahbek and Willerslev2011; Faith, Reference Faith2014; Stewart et al., Reference Stewart, Carleton and Groucutt2021). Various episodes of climatic stress or fast change have been proposed as causing the megafauna extinctions, for example: rapid Dansgaard-Oeschger warming events between 56,000–12,000 ya (Cooper et al., Reference Cooper, Turney, Hughen, Brook, McDonald and Bradshaw2015), regional longer-term climatic drying within the middle part of the last glaciation (Hocknull et al., Reference Hocknull, Lewis, Arnold, Pietsch, Joannes-Boyau, Price, Moss, Wood, Dosseto, Louys, Olley and Lawrence2020), but also even the relative stability of the Holocene itself (Mann et al., Reference Mann, Groves, Gaglioti and Shapiro2019).
A fundamental challenge for these explanations is that earlier severe climate instability did not lead to a similar pattern of extinction. Many earlier glacial cycles occurred during the Pleistocene, but only the most recent is associated with widespread and highly size-selective megafauna extinctions. Severe regional extinctions did occur earlier in the Pleistocene and were clearly climate-linked, but these affected a variety of non-megafauna organism groups, for example woody plants in Europe (Svenning, Reference Svenning2003; Magri et al., Reference Magri, Di Rita, Aranbarri, Fletcher and González-Sampériz2017; Martinetto et al., Reference Martinetto, Momohara, Bizzarri, Baldanza, Delfino, Esu and Sardella2017) and Australia (Mooney et al., Reference Mooney, Sniderman, Kershaw, Haberle, Roe and Keith2017), and mollusks in the Atlantic and the Mediterranean (Stanley and Campbell, Reference Stanley and Campbell1981; Raffi et al., Reference Raffi, Stanley and Marasti1985). These earlier extinctions did not lead to depauperate megafaunas and form a strong constrast to the megafauna-selective extinctions of the late Quaternary. This contrast extends even further back; earlier in the Cenozoic there were also major climate changes and associated extinctions, but these were also not size-selective and did not lead to depauperate megafaunas (Smith et al., Reference Smith, Elliott Smith, Lyons and Payne2018). An illustrative case concerns proboscideans in Europe and North America. Except for the last 0.01 million years, both continents have continuously harbored proboscideans throughout the last 18 and 16 million years, respectively, despite intense climate fluctuations (e.g., Fox and Fisher, Reference Fox and Fisher2004; Lucas and Morgan, Reference Lucas and Morgan2005; Haiduc et al., Reference Haiduc, Răţoi and Semprebon2018; von Koenigswald et al., Reference von Koenigswald, Widga and Göhlich2023). The contrast between these earlier non-size-selective extinction patterns and the strongly size-selective extinctions in the late Quaternary is a strong argument against a climatic causation.
An important consideration is if the last glacial cycle was somehow more severe than earlier ones, as this might then potentially explain its unique megafauna losses. There is, in fact, little support for such a scenario. Overall, Earth has had the same climate regime during the last ~1 million years, since the shift to deep, long (~100 kyr) glacial-interglacial cycles with the Mid-Pleistocene Climate Transition (Clark et al., Reference Clark, Archer, Pollard, Blum, Rial, Brovkin, Mix, Pisias and Roy2006; Herbert, Reference Herbert2023). Maximal Pleistocene ice sheet cover in the Northern Hemisphere was attained during multiple Middle Pleistocene glaciations rather than during the last glacial cycle (Batchelor et al., Reference Batchelor, Margold, Krapp, Murton, Dalton, Gibbard, Stokes, Murton and Manica2019). Similarly, multiple glacial cycles during the Middle Pleistocene and late Early Pleistocene led to more severe cooling and greater vegetation change (Margari et al., Reference Margari, Hodell, Parfitt, Ashton, Grimalt, Kim, Yun, Gibbard, Stringer, Timmermann and Tzedakis2023). Fast-paced, extreme climatic shifts similar to Heinrich events of the Last Glaciation are also documented from the earlier glacial cycles, having occurred since the Mid-Pleistocene Climate Transition (Naafs et al., Reference Naafs, Hefter and Stein2013). Further, strong millennial-scale climate variability similar to Dansgaard-Oeschger events of the last glaciation has been typical of glacial climates for at least the last 1.5 million years (Hodell et al., Reference Hodell, Crowhurst, Lourens, Margari, Nicolson, Rolfe, Skinner, Thomas, Tzedakis, Mleneck-Vautravers and Wolff2023; Margari et al., Reference Margari, Hodell, Parfitt, Ashton, Grimalt, Kim, Yun, Gibbard, Stringer, Timmermann and Tzedakis2023). Importantly, such frequent and persistent millennial climate instability prior to the Late Pleistocene has been shown to have had pronounced impacts on terrestrial ecosystems (e.g., vegetation) even within glacial refugial areas (Wilson et al., Reference Wilson, Frogley, Hughes, Roucoux, Margari, Jones, Leng and Tzedakis2021), but nevertheless did not elicit selective megafauna extinction episodes. Altogether, Quaternary climate history does not provide any obvious mechanism for the unique extinction pattern of the Late Pleistocene and Holocene.
On a global scale, megafauna extinction severity only poorly links to the severity of glacial maximum-present climate shift, with severe extinctions in many relatively stable regions such as California, southern Australia, and the pampas region of South America (Sandom et al., Reference Sandom, Faurby, Sandel and Svenning2014a; Lemoine et al., Reference Lemoine, Buitenwerf and Svenning2023). The continual climate changes throughout the late Quaternary mean that extinction-climate links may easily appear to be present in any restricted spatiotemporal window, that is, if the longer-term and broader geographic contexts are not considered. Further, strong climate change is predicted to elicit range and abundance responses in most species. In multiple cases, apparent regional population and community responses to climate have been suggested to support climate-driven extinction (e.g., Lorenzen et al., Reference Lorenzen, Nogués-Bravo, Orlando, Weinstock, Binladen, Marske, Ugan, Borregaard, Gilbert, Rs, Ho, Goebel, Graf, Byers, Stenderup, Rasmussen, Campos, Leonard, Koepfli, Froese, Zazula, Stafford, Aaris-Sorensen, Batra, Haywood, Singarayer, Valdes, Boeskorov, Burns, Davydov, Haile, Jenkins, Kosintsev, Kuznetsova, Lai, Martin, McDonald, Mol, Meldgaard, Munch, Stephan, Sablin, Sommer, Sipko, Scott, Suchard, Tikhonov, Willerslev, Wayne, Cooper, Hofreiter, Sher, Shapiro, Rahbek and Willerslev2011; Cooper et al., Reference Cooper, Turney, Hughen, Brook, McDonald and Bradshaw2015; Stewart et al., Reference Stewart, Carleton and Groucutt2021; Wang et al., Reference Wang, Pedersen, Alsos, De Sanctis, Racimo, Prohaska, Coissac, Owens, Merkel, Fernandez-Guerra, Rouillard, Lammers, Alberti, Denoeud, Money, Ruter, McColl, Larsen, Cherezova, Edwards, Fedorov, Haile, Orlando, Vinner, Korneliussen, Beilman, Bjørk, Cao, Dockter, Esdale, Gusarova, Kjeldsen, Mangerud, Rasic, Skadhauge, Svendsen, Tikhonov, Wincker, Xing, Zhang, Froese, Rahbek, Nogues, Holden, Edwards, Durbin, Meltzer, Kjær, Möller and Willerslev2021). However, such dynamics may reflect normal range responses to climate, as seen in numerous surviving species in response to the severe climatic changes of the period (e.g., Sommer et al., Reference Sommer, Kalbe, Ekström, Benecke and Liljegren2014; Cooper et al., Reference Cooper, Turney, Hughen, Brook, McDonald and Bradshaw2015). Further, other studies show contrasting patterns. For example, a sedimentary ancient DNA (sedaDNA) study from the Yukon shows strong megafauna decline between 21 and 14,500 years ago, prior to the loss of the mammoth steppe biome and the Younger Dryas (Murchie et al., Reference Murchie, Monteath, Mahony, Long, Cocker, Sadoway, Karpinski, Zazula, MacPhee, Froese and Poinar2021). In addition, as already mentioned, many extinct megafauna species have last occurrences in the Early or even Middle Holocene, that is, during the relatively stable climate of the Holocene, meaning that a climatic cause for their extinction is unlikely given their previous survival through numerous, massive climatic shifts throughout the Pleistocene, including long and warm interglacial periods.
An increasing number of studies look at local and regional dynamics in the overall abundance of large herbivores at high spatiotemporal resolution using dung-associated fungal spores. Many of these are able to pinpoint declines to timeframes where the climate was stable, for example, North America ~14–13,000 years ago, prior to the Younger Dryas cooling (Gill et al., Reference Gill, Williams, Jackson, Lininger and Robinson2009; Halligan et al., Reference Halligan, Waters, Perrotti, Owens, Feinberg, Bourne, Fenerty, Winsborough, Carlson, Fisher, Stafford and Dunbar2016; O’Keefe et al., Reference O’Keefe, Dunn, Weitzel, Waters, Martinez, Binder, Southon, Cohen, Meachen, DeSantis, Kirby, Ghezzo, Coltrain, Fuller, Farrell, Takeuchi, MacDonald, Davis and Lindsey2023), and 41,000 years ago in Australia at a time of no substantial climate change (Rule et al., Reference Rule, Brook, Haberle, Turney, Kershaw and Johnson2012; Adeleye et al., Reference Adeleye, Charles Andrew, Gallagher, van der Kaars, de Deckker, Hua and Haberle2023). In southeastern Brazil, the faecal spore decline spans from 15 to 11,000 years ago, overlapping a climatic wetting episode (Raczka et al., Reference Raczka, Bush and De Oliveira2018), and a similar coincidence is reported for a site at 3,000 m in the Peruvian Andes, between 16,800 and 15,800 years ago (Rozas-Davila et al., Reference Rozas-Davila, Valencia and Bush2016). However, these climate episodes were no more severe than the numerous others that occurred during the late Quaternary. Further, a 4,000 m site, also in the Peruvian Andes, finds that the decline occurs at 13–12,300 years ago and is not linked to any obvious climatic event (Rozas-Davila et al., Reference Rozas-Davila, Rodbell and Bush2023). In eastern North America strong, chronic spore declines occur during a relatively mild climate interval prior to the Younger Dryas, showing that this cooling episode cannot be the cause of the overall megafauna decline (e.g., Gill et al., Reference Gill, Williams, Jackson, Lininger and Robinson2009). Emerging sedaDNA studies are from high northern latitudes, where strong climate imprints on biotic dynamics are expected (Murchie et al., Reference Murchie, Monteath, Mahony, Long, Cocker, Sadoway, Karpinski, Zazula, MacPhee, Froese and Poinar2021; Wang et al., Reference Wang, Pedersen, Alsos, De Sanctis, Racimo, Prohaska, Coissac, Owens, Merkel, Fernandez-Guerra, Rouillard, Lammers, Alberti, Denoeud, Money, Ruter, McColl, Larsen, Cherezova, Edwards, Fedorov, Haile, Orlando, Vinner, Korneliussen, Beilman, Bjørk, Cao, Dockter, Esdale, Gusarova, Kjeldsen, Mangerud, Rasic, Skadhauge, Svendsen, Tikhonov, Wincker, Xing, Zhang, Froese, Rahbek, Nogues, Holden, Edwards, Durbin, Meltzer, Kjær, Möller and Willerslev2021). They report megafauna-climate relations, but with some megafauna survival deep into the relatively stable Holocene (Murchie et al., Reference Murchie, Monteath, Mahony, Long, Cocker, Sadoway, Karpinski, Zazula, MacPhee, Froese and Poinar2021; Wang et al., Reference Wang, Pedersen, Alsos, De Sanctis, Racimo, Prohaska, Coissac, Owens, Merkel, Fernandez-Guerra, Rouillard, Lammers, Alberti, Denoeud, Money, Ruter, McColl, Larsen, Cherezova, Edwards, Fedorov, Haile, Orlando, Vinner, Korneliussen, Beilman, Bjørk, Cao, Dockter, Esdale, Gusarova, Kjeldsen, Mangerud, Rasic, Skadhauge, Svendsen, Tikhonov, Wincker, Xing, Zhang, Froese, Rahbek, Nogues, Holden, Edwards, Durbin, Meltzer, Kjær, Möller and Willerslev2021) and sometimes with major declines prior to shifts in climate and vegetation (Murchie et al., Reference Murchie, Monteath, Mahony, Long, Cocker, Sadoway, Karpinski, Zazula, MacPhee, Froese and Poinar2021). Altogether, the detailed spatiotemporal resolution offered by the increasing number of fungal and sedaDNA studies does not support a strong role of climate in the chronic declines in megafauna.
Range modeling has been used to test if extinction is explainable from reduced climatic suitability through the late Quaternary, for example estimating strong reductions in climatically suitable areas for woolly mammoth (Fordham et al., Reference Fordham, Brown, Akçakaya, Brook, Haythorne, Manica, Shoemaker, Austin, Blonder, Pilowsky, Rahbek and Nogues-Bravo2022). However, such models estimate the realized niche, that is, the conditions under which the species occurred during the period where the calibration data come from, including pressure from humans, and hence might potentially estimate climatic refugia from Paleolithic hunting (cf. Pitulko et al., Reference Pitulko, Tikhonov, Pavlova, Nikolskiy, Kuper and Polozov2016). Since most of the late-Quaternary extinctions affected temperate to tropical species (Figure 2), late- and post-glacial declines in climatic suitability would not be expected for most species. Accordingly, range models for many extinct megafauna species have indicated stable, rising or at least large remaining areas of climatically suitable conditions into the Holocene (Martinez-Meyer et al., Reference Martinez-Meyer, Peterson and Hargrove2004; Varela et al., Reference Varela, Lobo, Rodríguez and Batra2010; Lima-Ribeiro et al., Reference Lima-Ribeiro, Nogués-Bravo, Terribile, Batra and Diniz-Filho2013; Villavicencio et al., Reference Villavicencio, Corcoran and Marquet2019). These results can be seen as having been empirically validated by the successful reintroductions of species that suffered prehistoric regional extirpations, notably horse (Equus ferus) in North America and muskox (Ovibos moschatus) in Eurasia (Lundgren et al., Reference Lundgren, Ramp, Ripple and Wallach2018).
An important piece of evidence that counts against any climate explanation for the late-Quaternary extinctions concerns the evidence for broad climatic tolerances, broad dietary niches, and persistent food availability for many of the extinct megafauna species. Large carnivores exemplify these patterns well, with broad climatic ranges and dietary flexibility, for example scimitar cats (Homotherium latidens), which in the Late Pleistocene in North America spanned from Alaska to Texas and had a diet varying from generalist foraging on large herbivores to more focus on juvenile large grazers, and short-faced bears (Arctodus simus) with a similar distribution and a foraging strategy including various ungulates as well as plants (Bocherens, Reference Bocherens2015; DeSantis et al., Reference DeSantis, Feranec, Antón and Lundelius2021; Smith et al., Reference Smith, Elliott Smith, Villaseñor, Tomé, Lyons and Newsome2022). Occurrence across widely varying climatic conditions and high levels of dietary flexibility were common for late-Quaternary herbivores (e.g., Price, Reference Price2008; França et al., Reference França, de Asevedo, Dantas, Bocchiglieri, Avilla, Lopes and da Silva2015; van Asperen and Kahlke, Reference van Asperen and Kahlke2015). Tooth-wear and isotope studies indicate high dietary variability among conspecific populations and individuals, from mainly grazing to mainly browsing, in extinct proboscideans from Eurasia and the Americas such as Mammuthus columbi, Palaeoloxodon antiquus, Notiomastodon platensis and Cuvieronius hyodon (González-Guarda et al., Reference González-Guarda, Petermann-Pichincura, Tornero, Domingo, Agustí, Pino, Abarzúa, Capriles, Villavicencio, Labarca, Tolorza, Sevilla and Rivals2018; Haiduc et al., Reference Haiduc, Răţoi and Semprebon2018; Rivals et al., Reference Rivals, Semprebon and Lister2019; Smith and DeSantis, Reference Smith and DeSantis2020; Dantas et al., Reference Dantas, Liparini, Asevedo, LdM and Cherkinsky2022). For example, the South American proboscidean Notiomastodon platensis occurred in multiple ecosystem types and varied in diet from grazer to mixed-feeder or browser depending on locality (e.g., Asevedo et al., Reference Asevedo, Winck, Mothé and Avilla2012; González-Guarda et al., Reference González-Guarda, Petermann-Pichincura, Tornero, Domingo, Agustí, Pino, Abarzúa, Capriles, Villavicencio, Labarca, Tolorza, Sevilla and Rivals2018; Pérez-Crespo et al., Reference Pérez-Crespo, Prado, Alberdi, Arroyo-Cabrales and Johnson2020; Asevedo et al., Reference Asevedo, Ranzi, Kalliola, Pärssinen, Ruokolainen, Cozzuol, ERd, Negri, Souza-Filho, Cherkinsky and Trindade Dantas2021; Dantas et al., Reference Dantas, Liparini, Asevedo, LdM and Cherkinsky2022). The American mastodon (Mammut americanum) exhibited a narrower signal as a consistent browser, but consumed a rich diversity of trees, shrubs, lianas, vines and herbs in Florida to spruce (Picea) and sedge-swamp plants in northern areas (Newsom and Mihlbachler, Reference Newsom, Mihlbachler and Webb2006; Green et al., Reference Green, DeSantis and Smith2017; Birks et al., Reference Birks, van Geel, Fisher, Grimm, Kuijper, van Arkel and van Reenen2019), leading to the conclusion that regional populations were capable of maintaining their dietary niche despite climate change (Green et al., Reference Green, DeSantis and Smith2017). Furthermore, studies of mastodon tusks indicate that a decreasing age in maturation over the last 1,000 years before their extinction, indicating that they were not experiencing nutritional stress (Fisher, Reference Fisher and Haynes2009), as was also concluded based on the rich diversity of plants – most still regionally present –in their diet close to their extinction (Newsom and Mihlbachler, Reference Newsom, Mihlbachler and Webb2006). Similarly, toothwear studies in extinct large carnivores also do not indicate food shortage toward their extinction in the terminal Pleistocene (DeSantis et al., Reference DeSantis, Schubert, Scott and and Ungar2012).
Altogether, while it is clear that megafauna populations responded to the climate dynamics of the late Quaternary, like numerous other species, the combined evidence strongly challenges climate-based causal explanations for the late-Quaternary megafauna losses (Table 2).
Table 2. Major arguments against a climatic causation of the prehistoric late-Quaternary megafauna extinctions and corresponding arguments for a human-caused explanation
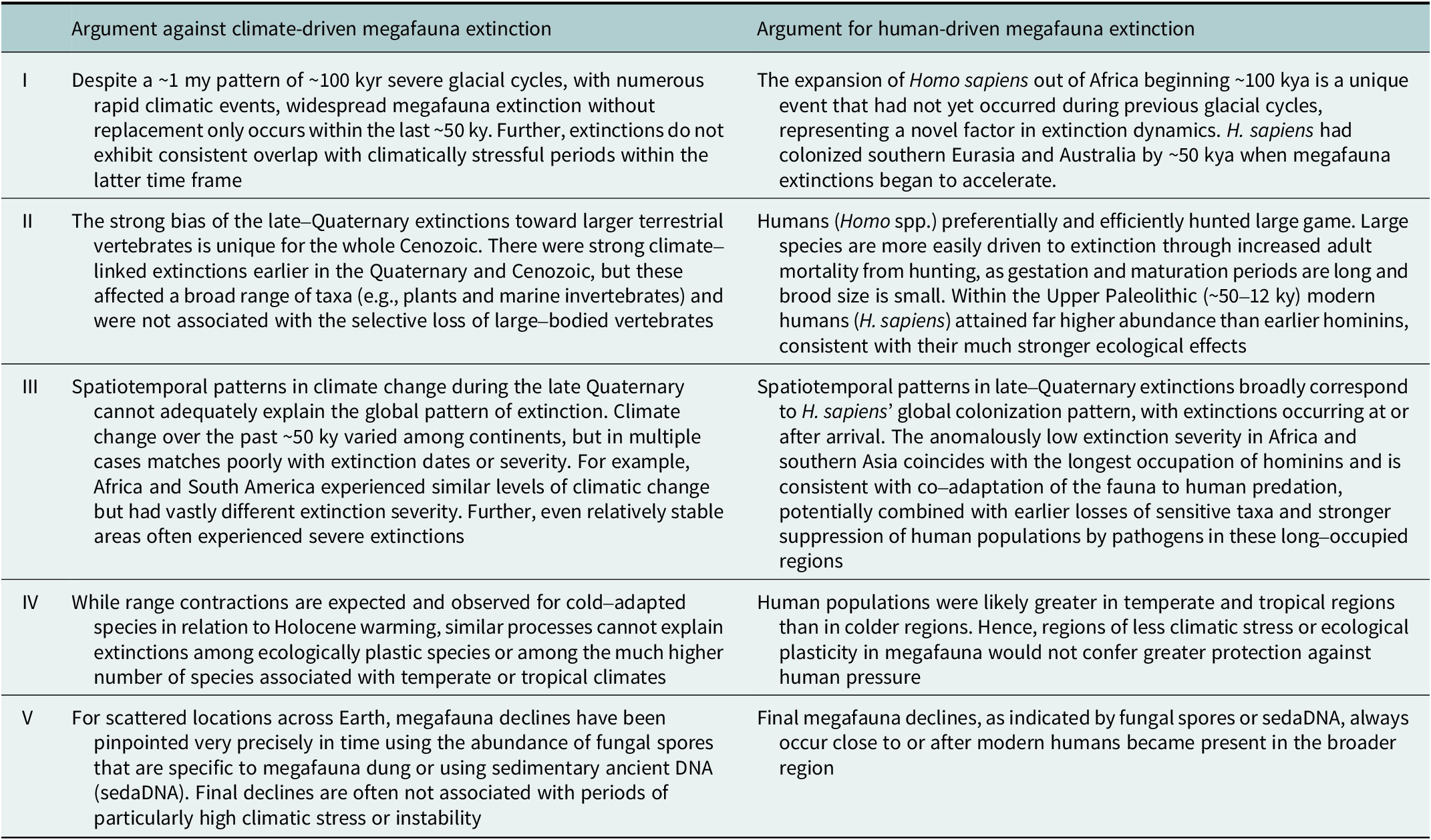
Note: The table summarizes the arguments outlined in the text, where the supporting references are provided.
Human causation
In contrast to the substantial arguments against climate as a major cause of the late-Quaternary megafauna extinctions, their linkage to the rise and expansion of behaviourally modern humans during the Late Pleistocene and Holocene is well supported by many types of evidence, offering an explanation for why the global loss of megafauna, unique for the whole Cenozoic, happened at this time (Smith et al., Reference Smith, Elliott Smith, Lyons and Payne2018; Figure 4), as the emergence and global dispersal of H. sapiens was itself a unique event in Earth history, given our species’ exceptional capabilities (e.g., Ellis, Reference Ellis2015). Notably, in areas with no pre-sapiens hominins, well-dated extinctions always occur around or after colonization by H. sapiens; additionally, extinction severity is strongly linked to human biogeography, with severe extinctions where H. sapiens was most novel (i.e., the first hominin present) and more moderate extinctions in areas of long-term human evolution (i.e., Africa and southern Asia), all discussed further below. Hence, the explanation most consistent with the extinction pattern is that expanding modern humans exploited megafauna at levels that were unsustainable in the short- or long-term.
There is widespread evidence that Late Pleistocene Homo sapiens populations targeted large game and were sophisticated, efficient megafauna hunters in the varied environments they colonized, for example: mammoths in northern Siberia as early as 45,000 years ago (Pitulko et al., Reference Pitulko, Tikhonov, Pavlova, Nikolskiy, Kuper and Polozov2016), diverse large mammals at the initial colonization of Europe north of the Alps at the same time (Smith et al., Reference Smith, Ruebens, Zavala, Sinet-Mathiot, Fewlass, Pederzani, Jaouen, Mylopotamitaki, Britton, Rougier, Stahlschmidt, Meyer, Meller, Dietl, Orschiedt, Krause, Schüler, McPherron, Weiss, Hublin and Welker2024), and a diversity of gomphotheres, giant ground sloths, giant armadillos, equids, bears, cervids, and camelids across South America (Bampi et al., Reference Bampi, Barberi and Lima-Ribeiro2022). In fact, numerous megafauna kill sites for a large number of extinct species exist in Africa (Kovarovic et al., Reference Kovarovic, Faith, Jenkins, Tryon and Peppe2021), Eurasia (Shipman, Reference Shipman2015; Pitulko et al., Reference Pitulko, Tikhonov, Pavlova, Nikolskiy, Kuper and Polozov2016), North America (Sanchez et al., Reference Sanchez, Holliday, Gaines, Arroyo-Cabrales, Martínez-Tagüeña, Kowler, Lange, Hodgins, Mentzer and Sanchez-Morales2014; Waters et al., Reference Waters, Stafford, Kooyman and Hills2015), and South America (Bampi et al., Reference Bampi, Barberi and Lima-Ribeiro2022). Kill sites are so far missing from the limited record for Australia and New Guinea (Hocknull et al., Reference Hocknull, Lewis, Arnold, Pietsch, Joannes-Boyau, Price, Moss, Wood, Dosseto, Louys, Olley and Lawrence2020). Many of the extinct megaherbivores (Table 1) likely had no predators as adults and are expected to have been vulnerable to the use of projectiles, traps, and fire (Agam and Barkai, Reference Agam and Barkai2018). Moreover, severe size-biased extinctions are also predicted from mechanistic modeling of human hunting and impacts on prey populations due to well-established general relationships of demographic characteristics to body mass (Alroy, Reference Alroy2001). Apart from hunting, other mechanisms of human-mediated megafauna extinctions have also been considered, notably habitat alteration, introduced predators (such as dogs), and introduced pathogens (Koch and Barnosky, Reference Koch and Barnosky2006). However, these mechanisms cannot explain the size-selectivity, nor the timing and generality of the extinctions (Koch and Barnosky, Reference Koch and Barnosky2006). Further, as discussed earlier, co-extinctions - rather than direct human persecution - help explain the extinctions that did occur among smaller species, clearly playing a role for directly megafauna-dependent species, but potentially also more widely. Notably, extinctions among various moderately sized species may potentially be linked to the disappearance of many or all of the largest herbivores (Owen-Smith, Reference Owen-Smith1987).
Human biogeography provides a logical explanation for both the striking global spatiotemporal patterns in the late-Quaternary megafauna extinctions and for their severity. The lower severity and longer time frame of the extinctions in Africa and southern Asia matches the core areas of evolution of Homo sapiens as well as that of hominins broadly, as already noted by Martin (Reference Martin1966, Reference Martin1967). A number of macroecological analyses have shown that the expansion of H. sapiens in the context of the broader human biogeographic history provides high statistical explanatory power for the global spatial and spatiotemporal patterns in the megafauna extinctions, whereas climate offers little such explanatory power (Sandom et al., Reference Sandom, Faurby, Sandel and Svenning2014a; Bartlett et al., Reference Bartlett, Williams, Prescott, Balmford, Green, Eriksson, Valdes, Singarayer and Manica2016; Araujo et al., Reference Araujo, Oliveira-Santos, Lima-Ribeiro, Diniz-Filho and Fernandez2017; Andermann et al., Reference Andermann, Faurby, Turvey, Antonelli and Silvestro2020; Lemoine et al., Reference Lemoine, Buitenwerf and Svenning2023). The same is true for severe population declines in numerous extant megafauna species over the last ~50,000 years, estimated based on current genomes (Bergman et al., Reference Bergman, Pedersen, Lundgren, Lemoine, Monsarrat, Pearce, Schierup and Svenning2023). When analyzing human and climatic predictors together, these global analyses all identify a dominant role for human biogeography, with climate providing a small (Sandom et al., Reference Sandom, Faurby, Sandel and Svenning2014a; Bartlett et al., Reference Bartlett, Williams, Prescott, Balmford, Green, Eriksson, Valdes, Singarayer and Manica2016) or negligible contribution (Araujo et al., Reference Araujo, Oliveira-Santos, Lima-Ribeiro, Diniz-Filho and Fernandez2017; Andermann et al., Reference Andermann, Faurby, Turvey, Antonelli and Silvestro2020; Bergman et al., Reference Bergman, Pedersen, Lundgren, Lemoine, Monsarrat, Pearce, Schierup and Svenning2023; Lemoine et al., Reference Lemoine, Buitenwerf and Svenning2023). Hence, the global analyses strongly support human causation, and even indicate little or no interaction with climate change, neither in the severity of extinction and decline nor in the timing. Further, the detailed spatiotemporal resolution offered by the increasing number of fungal and sedaDNA studies consistently shows that final megafauna declines occur close to or after the arrival of H. sapiens, for example in the arctic (Murchie et al., Reference Murchie, Monteath, Mahony, Long, Cocker, Sadoway, Karpinski, Zazula, MacPhee, Froese and Poinar2021; Wang et al., Reference Wang, Pedersen, Alsos, De Sanctis, Racimo, Prohaska, Coissac, Owens, Merkel, Fernandez-Guerra, Rouillard, Lammers, Alberti, Denoeud, Money, Ruter, McColl, Larsen, Cherezova, Edwards, Fedorov, Haile, Orlando, Vinner, Korneliussen, Beilman, Bjørk, Cao, Dockter, Esdale, Gusarova, Kjeldsen, Mangerud, Rasic, Skadhauge, Svendsen, Tikhonov, Wincker, Xing, Zhang, Froese, Rahbek, Nogues, Holden, Edwards, Durbin, Meltzer, Kjær, Möller and Willerslev2021), north-eastern (Gill et al., Reference Gill, Williams, Jackson, Lininger and Robinson2009), south-eastern (Halligan et al., Reference Halligan, Waters, Perrotti, Owens, Feinberg, Bourne, Fenerty, Winsborough, Carlson, Fisher, Stafford and Dunbar2016), and south-western North America (O’Keefe et al., Reference O’Keefe, Dunn, Weitzel, Waters, Martinez, Binder, Southon, Cohen, Meachen, DeSantis, Kirby, Ghezzo, Coltrain, Fuller, Farrell, Takeuchi, MacDonald, Davis and Lindsey2023), eastern South America (Raczka et al., Reference Raczka, Bush and De Oliveira2018) and the Andes (Rozas-Davila et al., Reference Rozas-Davila, Valencia and Bush2016, Reference Rozas-Davila, Rodbell and Bush2023), and northern (Rule et al., Reference Rule, Brook, Haberle, Turney, Kershaw and Johnson2012) and southern Australia (Adeleye et al., Reference Adeleye, Charles Andrew, Gallagher, van der Kaars, de Deckker, Hua and Haberle2023). Also, severe megafauna losses in areas with relatively mild, stable climates are consistent with human causation, as human populations were likely greater in temperate and tropical regions than in colder regions (e.g., Ordonez and Riede, Reference Ordonez and Riede2022). Hence, less climatic stress or ecological plasticity in megafauna would not confer greater protection against human pressure in these areas.
While the human-megafauna link is clear, the mechanisms involved are less so. It has been hypothesized that, because Homo originated in Africa and was present in much of southern Eurasia from an early date, local megafauna would have had time to adapt to gradually intensifying hominin predation over a long period of time, whereas in Australia, the Americas, or on islands megafauna would have faced highly developed groups of H. sapiens with no prior adaptation, leading to severe extinctions (e.g., Martin, Reference Martin1967; Sandom et al., Reference Sandom, Faurby, Sandel and Svenning2014a). It is unclear what form such adaptation would take, but such responses are seen in modern “human predator” systems, for example decreased body size and increased reproductive capacity (Darimont et al., Reference Darimont, Carlson, Kinnison, Paquet, Reimchen and Wilmers2009). Traits conferring vulnerability to hunting, such as slow movement, might also have been more common outside Africa and Eurasia (Johnson, Reference Johnson2009). Here, such susceptible species might have been driven to extinction much earlier (e.g., Martin, Reference Martin1966), potentially allowing for diversification in more human-tolerant groups, such as smaller bovids. As mentioned earlier, there are Early-Middle Pleistocene simplification dynamics involving both global extinctions and regional extirpations in megafaunas in Eurasia and Africa. These have been linked to climate change (e.g., Faith et al., Reference Faith, Rowan, Du and Koch2018; Bibi and Cantalapiedra, Reference Bibi and Cantalapiedra2023; Zhang et al., Reference Zhang, Westaway, Haberle, Lubeek, Bailey, Ciochon, Morley, Roberts, J-x, Duval, Dosseto, Pan, Rule, Liao, Gully, Lucas, Mo, Yang, Cai, Wang and Joannes-Boyau2024), but also, alternatively, to pre-sapiens hominin impacts beginning as far back as 2–4 million years ago, through the evolutionary insertion of hominins into the large-carnivore guild (e.g., Martin, Reference Martin1966; Antón et al., Reference Antón, Galobart and Turner2005; Faurby et al., Reference Faurby, Silvestro, Werdelin and Antonelli2020b; Domínguez-Rodrigo et al., Reference Domínguez-Rodrigo, Baquedano, Organista, Cobo-Sánchez, Mabulla, Maskara, Gidna, Pizarro-Monzo, Aramendi, Galán, Cifuentes-Alcobendas, Vegara-Riquelme, Jiménez-García, Abellán, Barba, Uribelarrea, Martín-Perea, Diez-Martin, Maíllo-Fernández, Rodríguez-Hidalgo, Courtenay, Mora, Maté-González and González-Aguilera2021; Dembitzer et al., Reference Dembitzer, Barkai, Ben-Dor and Meiri2022; Plummer et al., Reference Plummer, Oliver, Finestone, Ditchfield, Bishop, Blumenthal, Lemorini, Caricola, Bailey, Herries, Parkinson, Whitfield, Hertel, Kinyanjui, Vincent, Li, Louys, Frost, Braun, Reeves, EDG, Onyango, Lamela-Lopez, Forrest, He, Lane, Frouin, Nomade, Wilson, Bartilol, Rotich and Potts2023). Among these earlier extinctions, there is evidence of butchery by hominins, for example Sivatherium (Organista et al., Reference Organista, Domínguez-Rodrigo, Yravedra, Uribelarrea, Arriaza, Ortega, Mabulla, Gidna and Baquedano2017), Deinotherium (Surovell et al., Reference Surovell, Waguespack and Brantingham2005), and giant geladas (Shipman et al., Reference Shipman, Bosler, Davis, Behrensmeyer, Dunbar, Groves, Thackeray, Couvering and Stucky1981) in Africa and the large beaver Trogontherium cuvieri in Europe (Yang et al., Reference Yang, Li, Fostowicz-Frelik and Ni2019). Similar patterns are also seen in the extinction of large and giant tortoises across the globe. While not strictly megafauna by some definitions, these large reptiles are obviously sensitive to human predation and have a long history of hominin procurement (Rhodin et al., Reference Rhodin, Thomson, Georgalis, Karl, Danilov, Takahashi, de la Fuente, Bourque, Delfino, Bour, Iverson, Shaffer and van Dijk2015). The largest tortoises disappear first in Africa during the Early Pleistocene, followed quickly by mainland Eurasia, then experience severe extinctions during the late Quaternary in Australia and the Americas, followed by many islands in the Holocene and in recorded history (Rhodin et al., Reference Rhodin, Thomson, Georgalis, Karl, Danilov, Takahashi, de la Fuente, Bourque, Delfino, Bour, Iverson, Shaffer and van Dijk2015). Additional factors may also have played a role in generating the human-megafauna link, for example higher disease load for Homo populations in areas long occupied by hominins due to coevolved parasites and pathogens (Koch and Barnosky, Reference Koch and Barnosky2006). A potentially underexplored theme is the influence of habitat on modulating human-linked extinction patterns. It has, for example, been suggested that less human-accessible habitats potentially explain some survivals (Johnson, Reference Johnson2002).
Human causation has been questioned based on the hypothesized rapidity of extinctions by relatively small groups of humans in North America (Martin, Reference Martin1973). While there is clear evidence of rapid, permanent declines in many localities (e.g., Gill et al., Reference Gill, Williams, Jackson, Lininger and Robinson2009; Rule et al., Reference Rule, Brook, Haberle, Turney, Kershaw and Johnson2012; Adeleye et al., Reference Adeleye, Charles Andrew, Gallagher, van der Kaars, de Deckker, Hua and Haberle2023), this so-called “blitzkrieg” version of human overkill is clearly not adequate, with evidence for declines and extinctions occurring over many millenia, particularly at broader geographic scales (e.g., Koch and Barnosky, Reference Koch and Barnosky2006). Often there is evidence for continent-level overlap between extinct megafauna species and H. sapiens across very extended time periods (e.g., Andermann et al., Reference Andermann, Faurby, Turvey, Antonelli and Silvestro2020), with megafauna kill sites in South America spanning ~10,000 years, for example (Bampi et al., Reference Bampi, Barberi and Lima-Ribeiro2022). Such overlaps have long been recognized in Eurasia, given the late survival and eventual human-driven extinction and extirpation of taxa such as aurochs (Bos primigenius primigenius) (e.g., Crees et al., Reference Crees, Carbone, Sommer, Benecke and Turvey2016) and northern populations of Asiatic elephant (Elephas maximus) and other megafauna in East Asia (Teng et al., Reference Teng, Xu, Teng and Svenning2020). These patterns are further supported by emerging evidence of later survival in relict megafauna populations than formerly thought, for example in northern Eurasia and North America (Murchie et al., Reference Murchie, Monteath, Mahony, Long, Cocker, Sadoway, Karpinski, Zazula, MacPhee, Froese and Poinar2021; Wang et al., Reference Wang, Pedersen, Alsos, De Sanctis, Racimo, Prohaska, Coissac, Owens, Merkel, Fernandez-Guerra, Rouillard, Lammers, Alberti, Denoeud, Money, Ruter, McColl, Larsen, Cherezova, Edwards, Fedorov, Haile, Orlando, Vinner, Korneliussen, Beilman, Bjørk, Cao, Dockter, Esdale, Gusarova, Kjeldsen, Mangerud, Rasic, Skadhauge, Svendsen, Tikhonov, Wincker, Xing, Zhang, Froese, Rahbek, Nogues, Holden, Edwards, Durbin, Meltzer, Kjær, Möller and Willerslev2021). Long-extended extinction processes are in fact expected under a human impact model given the progressive increase in human population density and socio-technological capabilities (e.g., Ellis, Reference Ellis2015), as well-documented for the mass killing, progressive decline, and eventual near-extinction of ungulates in the Holocene Levant (Bar-Oz et al., Reference Bar-Oz, Zeder and Hole2011). Similar dynamics in population density and culture across the transition from archaic to modern humans also offer an explanation for why only the expansion of the latter caused severe, wide-scale megafauna extinction (aside from the exceptions discussed above), despite the former also being capable megafauna hunters (e.g., Domínguez-Rodrigo et al., Reference Domínguez-Rodrigo, Baquedano, Organista, Cobo-Sánchez, Mabulla, Maskara, Gidna, Pizarro-Monzo, Aramendi, Galán, Cifuentes-Alcobendas, Vegara-Riquelme, Jiménez-García, Abellán, Barba, Uribelarrea, Martín-Perea, Diez-Martin, Maíllo-Fernández, Rodríguez-Hidalgo, Courtenay, Mora, Maté-González and González-Aguilera2021; Gaudzinski-Windheuser et al., Reference Gaudzinski-Windheuser, Kindler, MacDonald and Roebroeks2023).
Other points of criticism regarding human causation include a perceived rarity of kill sites and the amount of waste that would be necessary for small human populations to have exterminated megafauna, but both are expected based on taphonomic bias, modern hunter-gatherer analogs, and the relative scarcity of relevant archeological sites (Alroy, Reference Alroy2001, Koch and Barnosky, Reference Koch and Barnosky2006; Surovell and Waguespack, Reference Surovell and Waguespack2008; Ben-Dor et al., Reference Ben-Dor, Gopher, Hershkovitz and Barkai2011; Wolfe and Broughton, Reference Wolfe and Broughton2020). In summary, while many details of a human-driven model are subject to further investigation, it remains highly consistent with the total evidence (Table 2).
Ecological consequences
Current megafauna-poor ecosystems are ecologically novel relative to evolutionary baselines since the majority of modern taxa (e.g., plants, invertebrates) originated and evolved with diverse, abundant large-mammal assemblages (e.g., Martin, Reference Martin1967; Donlan et al., Reference Donlan, Berger, Bock, Bock, Burney, Estes, Foreman, Martin, Roemer, Smith, Soulé and Greene2006; Fløjgaard et al., Reference Fløjgaard, Pedersen, Sandom, Svenning and Ejrnæs2022; Figure 4). Since large mammals often have disproportionate impacts on ecosystem structure and processes (Bakker et al., Reference Bakker, Gill, Johnson, Vera, Sandom, Asner and Svenning2016; Enquist et al., Reference Enquist, Abraham, Harfoot, Malhi and Doughty2020; Pringle et al., Reference Pringle, Abraham, Anderson, Coverdale, Davies, Dutton, Gaylard, Goheen, Holdo, Hutchinson, Kimuyu, Long, Subalusky and Veldhuis2023), the loss of megafauna likely has had strong consequences for many ecosystems (Johnson, Reference Johnson2009; Malhi et al., Reference Malhi, Lander, le Roux, Stevens, Macias-Fauria, Wedding, Girardin, Kristensen, Sandom, Evans, Svenning and Canney2022) and has led to major shifts in biotic community assembly (Carotenuto et al., Reference Carotenuto, Di Febbraro, Melchionna, Castiglione, Saggese, Serio, Mondanaro, Passaro, Loy and Raia2016; Lyons et al., Reference Lyons, Amatangelo, Behrensmeyer, Bercovici, Blois, Davis, DiMichele, Du, Eronen, Faith, Graves, Jud, Labandeira, Looy, McGil, Miller, Patterson, Pineda-Munoz, Potts, Riddle, Terry, Tóth, Ulrich, Villaseñor, Wing, Anderson, Anderson, Waller and Gotelli2016; Tóth et al., Reference Tóth, Lyons, Barr, Behrensmeyer, Blois, Bobe, Davis, Du, Eronen, Faith, Fraser, Gotelli, Graves, Jukar, Miller, Pineda-Munoz, Soul, Villaseñor and Alroy2019; Smith et al., Reference Smith, Elliott Smith, Hedberg, Lyons, Pardi and Tomé2023). Importantly, the effects of large mammals cannot be fully compensated for by smaller species (e.g.,Lundgren et al., Reference Lundgren, Bergman, Trepel, Le Roux, Monsarrat, Kristensen, Pedersen, Pereyra, Tietje and Svenning2024; Trepel et al., Reference Trepel, le Roux, Abraham, Buitenwerf, Kamp, Kristensen, Tietje, Lundgren and Svenning2024) and as such, the changes in impact are expected to have been amplified by the bias in extinctions toward the largest megafauna species (Smith et al., Reference Smith, Elliott Smith, Lyons and Payne2018, Smith et al., Reference Smith, Elliott Smith, Hedberg, Lyons, Pardi and Tomé2023; Figure 1).
The consequences of losing large-bodied species are intuitive since many ecological processes scale with body size, for example metabolic rate and energy consumption (Enquist et al., Reference Enquist, Abraham, Harfoot, Malhi and Doughty2020), forage selectivity (Bell, Reference Bell1971; Jarman, Reference Jarman1974; Pansu et al., Reference Pansu, Guyton, Potter, Atkins, Daskin, Wursten, Kartzinel and Pringle2019), movement capacity (Berti and Svenning, Reference Berti and Svenning2020), and associated processes like seed (Fricke et al., Reference Fricke, Hsieh, Middleton, Gorczynski, Cappello, Sanisidro, Rowan, Svenning and Beaudrot2022) and nutrient dispersal (le Roux et al., Reference Le Roux, van Veenhuisen, Kerley and Cromsigt2020). However, we have a poorer understanding of other organismal traits, life-history strategies, and behavioral expressions that have been lost and may have had large impacts on community and ecosystem processes (Pringle, Reference Pringle2020). For example, proboscideans, which suffered particularly high extinction rates, have prehensile trunks that, in combination with large body size, have the potential to exert unique impacts on plants and vegetation structure (Haynes, Reference Haynes2012). Another example is seasonal mega-herd migrations of medium to large-bodied herbivores, now only existing in a few places on Earth, where they have overriding impacts on ecosystem dynamics (e.g., Subalusky et al., Reference Subalusky, Dutton, Rosi and Post2017; Owen-Smith et al., Reference Owen-Smith, Hopcraft, Morrison, Chamaillé-Jammes, Hetem, Bennitt and Van Langevelde2020; Anderson et al., Reference Anderson, Hepler, Holdo, Donaldson, Erhardt, Hopcraft, Hutchinson, Huebner, Morrison, Muday, Munuo, Palmer, Pansu, Pringle, Sketch and Packer2024). Such migrations were much more common before human-driven extirpations (e.g., Spinage, Reference Spinage1992; Bar-Oz et al., Reference Bar-Oz, Zeder and Hole2011).
In this section, we evaluate how megafauna affected and continue to affect ecosystem and community processes, drawing on direct evidence from both the past and from contemporary ecosystems. In doing so, we discuss the known and likely ecological consequences of the late-Quaternary megafauna extinctions, with the evidence together indicating that strong losses of large-bodied animals constitute a fundamental re-shaping of terrestrial ecosystem structure and functioning worldwide (Figure 5; see also Smith et al., Reference Smith, Elliott Smith, Hedberg, Lyons, Pardi and Tomé2023). The processes by which megafauna affect ecosystems can be grouped within three broad categories: (1) trophic processes that enforce a top-down control on lower trophic levels and ecosystem processes, (2) physical engineering of the abiotic and biotic environment and processes, and (3) the transportation of energy and matter, including nutrients, plant seeds, and smaller organisms.

Figure 5. Potential impacts of megafauna extinctions on ecosystems. Apart from downsizing faunal biomass, loss of vegetation heterogeneity, and reducing trophic complexity across biomes, megafauna extinctions can also be linked with contemporary ecosystem functioning. Here, three potential cases, all supported by the literature. (A) Across the Americas, big carcasses from the high abundance of proboscideans and other megafauna supported large scavengers (Galetti et al., Reference Galetti, Moleón, Jordano, Pires, Guimarães, Pape, Nichols, Hansen, Olesen, Munk, de Mattos, Schweiger, Owen-Smith, Johnson, Marquis and Svenning2018). (B) Megafauna extinction can be linked to extinctions and extirpations of large scavengers in the region and may have driven changes to the vector-borne pathogen regime (Doughty et al., Reference Doughty, Prys‐Jones, Faurby, Abraham, Hepp, Leshyk, Fofanov, Nieto, Svenning and Galetti2020). (C) In the tropical America, large herbivores such as ground sloths and glyptodonts likely dispersed large-seeded plants long distances and promoted greater vegetation openness through the consumption of plant biomass (Janzen and Martin, Reference Janzen and Martin1982; Doughty et al., Reference Doughty, Faurby and Svenning2016a). (D) The extinction of megafauna from tropical Americas can be linked to the reduced dispersal of large-seeded plants and structural homogenization of vegetation cover (Doughty et al., Reference Doughty, Faurby and Svenning2016a). (E) In Australia, giant kangaroos and diprotodons extensively fed on a wide variety of plants, likely contributing to the maintenance of vegetation diversity. (F) Megafauna extinctions in Australia can be linked to intensified fire regimes in some areas and associated declines in fire-sensitive plant taxa (Rule et al., Reference Rule, Brook, Haberle, Turney, Kershaw and Johnson2012). (Images: A, HodariNundu; C, D, Bogdanov, WolfmanSF Naturhistorisches Museum Wien [Wikimedia Commons]; E, Dmitry Bogdanov [Wikimedia Commons], Queensland Museum).
Trophic processes
There is a wealth of literature on direct local- to landscape-scale effects of extant large herbivores on vegetation, including vegetation biomass and structure (Asner and Levick, Reference Asner and Levick2012; Davies et al., Reference Davies, Gaylard and Asner2018; Lundgren et al., Reference Lundgren, Bergman, Trepel, Le Roux, Monsarrat, Kristensen, Pedersen, Pereyra, Tietje and Svenning2024; Trepel et al., Reference Trepel, le Roux, Abraham, Buitenwerf, Kamp, Kristensen, Tietje, Lundgren and Svenning2024) as well as plant community composition (Bakker et al., Reference Bakker, Ritchie, Olff, Milchunas and Knops2006; Bakker et al., Reference Bakker, Pagès, Arthur and Alcoverro2016; Staver et al., Reference Staver, Abraham, Hempson, Karp and Faith2021). Herbivore pressure can shift plant species assemblages in various ways. Species-specific dietary preferences will see some species being consumed more frequently than others and shift species composition in favour of the less palatable plant species. However, some plant species are better equipped to deal with herbivore pressure, either by being able to avoid consumption or by being able to tolerate it (Archibald et al., Reference Archibald, Hempson and Lehmann2019). Herbivores may also shape community composition in more indirect ways, where they can keep the growth of dominant species in check and thereby provide the opportunity for competitively inferior species to keep a foothold in the community. This disruption of plant competitive interactions links trophic impact to high plant diversity (Koerner et al., Reference Koerner, Smith, Burkepile, Hanan, Avolio, Collins, Knapp, Lemoine, Forrestel and Eby2018), particularly when the feeding disturbance is from large, indiscriminate feeders that are forced to target quantity over quality (Lundgren et al., Reference Lundgren, Bergman, Trepel, Le Roux, Monsarrat, Kristensen, Pedersen, Pereyra, Tietje and Svenning2024; Trepel et al., Reference Trepel, le Roux, Abraham, Buitenwerf, Kamp, Kristensen, Tietje, Lundgren and Svenning2024).
Given their current vegetation relations, the late-Quaternary megafauna extinctions are estimated to have had strong effects on vegetation structure and dynamics, not least due to declines in herbivory levels (e.g., Bakker et al., Reference Bakker, Gill, Johnson, Vera, Sandom, Asner and Svenning2016; Doughty et al., Reference Doughty, Faurby and Svenning2016a, Pedersen et al., Reference Pedersen, Faurby and Svenning2023; Davoli et al., Reference Davoli, Monsarrat, Pedersen, Scussolini, Karger, Normand and Svenning2024; Figure 5). There are palaeoecological studies consistent with strong herbivore impacts on vegetation prior to the late-Quaternary megafauna extinctions, for example high amounts of open and semi-open vegetation alongside high densities of large herbivores in Last Interglacial Britain (Sandom et al., Reference Sandom, Ejrnæs, Hansen and Svenning2014b). Disturbance-linked vegetation types occurred widely across Europe in the same time frame (Pearce et al., Reference Pearce, Mazier, Normand, Fyfe, Andrieu, Bakels, Balwierz, Bińka, Boreham, Borisova, Brostrom, de Beaulieu, Gao, González-Sampériz, Granoszewski, Hrynowiecka, Kołaczek, Kuneš, Magri, Malkiewicz, Mighall, Milner, Möller, Nita, Noryśkiewicz, Pidek, Reille, Robertsson, Salonen, Schläfli, Schokker, Scussolini, Šeirienė, Strahl, Urban, Winter and Svenning2023), despite the generally warm, productive climate, consistent with high estimated densities of large herbivores (Davoli et al., Reference Davoli, Monsarrat, Pedersen, Scussolini, Karger, Normand and Svenning2024). There are also palaeoecological studies of other ecosystem types, for example the mammoth steppe, supporting at least some direct role of the late-Quaternary megafauna losses in the collapse of this forb- and grass-rich biome type (e.g., Murchie et al., Reference Murchie, Monteath, Mahony, Long, Cocker, Sadoway, Karpinski, Zazula, MacPhee, Froese and Poinar2021; Magyari et al., Reference Magyari, Gasparik, Major, Lengyel, Pál, Virág, Korponai, Haliuc, Szabó and Pazonyi2022). Other cases of vegetation transformations associated with megafauna extinctions come from eastern North America (Gill et al., Reference Gill, Williams, Jackson, Lininger and Robinson2009) and Australia (Rule et al., Reference Rule, Brook, Haberle, Turney, Kershaw and Johnson2012), but strong changes do not always seem to have happened (Johnson et al., Reference Johnson, Rule, Haberle, Kershaw, McKenzie and Brook2016), and their generality, detailed characteristics, and the mechanisms involved need to become better understood.
Large carnivores can have strong impacts on the population dynamics (e.g., Le Roux et al., Reference Le Roux, Marneweck, Clinning, Druce, Kerley and Cromsigt2019) and behaviour (e.g., Le Roux et al., Reference Le Roux, Kerley and Cromsigt2018) of specific herbivore species. However, predation in many contexts does not reduce overall herbivore biomass (Mduma et al., Reference Mduma, Sinclair and Hilborn2001; Le Roux et al., Reference Le Roux, Marneweck, Clinning, Druce, Kerley and Cromsigt2019) or, by extension, consumption of plant biomass (Beschta et al., Reference Beschta, Ripple, Kauffman and Painter2020; Hobbs et al., Reference Hobbs, Johnston, Marshall, Wolf and Cooper2024). Notably, megaherbivores (body mass ≥1,000 kg) are essentially immune to predation due to their large size and strength, have strong impacts on vegetation, and were omnipresent across all continents except Antarctica until the end-Pleistocene and Early Holocene (Owen-Smith, Reference Owen-Smith1987). Although dietary reconstruction suggests that machairodont cats in some cases likely hunted juvenile megaherbivores (DeSantis et al., Reference DeSantis, Feranec, Antón and Lundelius2021; Smith et al., Reference Smith, Elliott Smith, Villaseñor, Tomé, Lyons and Newsome2022), they also indicate that megaherbivores often formed only a small proportion of the diet (e.g., Coltrain et al., Reference Coltrain, Harris, Cerling, Ehleringer, Dearing, Ward and Allen2004; Bocherens, Reference Bocherens2015), and population limitation was unlikely (but see Van Valkenburgh et al., Reference Van Valkenburgh, Hayward, Ripple, Meloro and Roth2016). One contributing factor may have been that various megaherbivores also attained larger body sizes than their present-day relatives, for example proboscideans (Larramendi, Reference Larramendi2016). Macroecological analyses support a dominant role of megaherbivores in megafauna community assembly relative to carnivores and mesoherbivores, alongside carnivore effects on the latter (Mondanaro et al., Reference Mondanaro, Castiglione, Melchionna, Di Febbraro, Vitagliano, Serio, Vero, Carotenuto and Raia2017).
Research from Africa shows that even restoring megacarnivores such as lions (Panthera leo) does not lead to decreases in overall herbivore biomass, but instead shifts it to the larger species, including the bigger non-megaherbivores (Le Roux et al., Reference Le Roux, Marneweck, Clinning, Druce, Kerley and Cromsigt2019). The wolf reintroduction to Yellowstone National Park provides a similar case. Here, the re-establishment of wolves, alongside resurging numbers of other large carnivores, coincided with a decline in the elk population (Cervus canadensis), albeit likely enhanced by human hunting (Hobbs et al., Reference Hobbs, Johnston, Marshall, Wolf and Cooper2024). However, this decline is now increasingly compensated by rising numbers of American bison (Bison bison), a species rarely predated by wolves, despite high levels of culling (Beschta et al., Reference Beschta, Ripple, Kauffman and Painter2020; Hobbs et al., Reference Hobbs, Johnston, Marshall, Wolf and Cooper2024). Along similar lines, the largest surviving wild herbivore in Europe, European bison (B. bonasus) does not experience significant predation by wolves or other carnivores where they co-occur in eastern Europe (Okarma, Reference Okarma1995). Importantly, while overall herbivory may not be down-regulated by predation in assemblages with diverse megafaunas, such predation-linked shifts toward larger herbivore species are expected to lead to changes in forage species selection and spatial patterns of impact. Smaller species are more likely to exhibit spatial responses to predation risk (e.g., Davies et al., Reference Davies, Cromsigt, Tambling, le Roux, Vaughn, Druce, Marneweck and Asner2021; Lundgren et al., Reference Lundgren, Ramp, Middleton, Wooster, Kusch, Balisi, Ripple, Hasselerharm, Sanchez, Mills and Wallach2022), while larger species are more likely to include low-quality food in their diet (e.g., Daskin et al., Reference Daskin, Becker, Kartzinel, Potter, Walker, Eriksson, Buoncore, Getraer, Long and Pringle2023). Predation-driven shifts toward larger herbivore species, whose bulk requirements force them to feed more indiscriminately, tend to promote local plant diversity (Lundgren et al., Reference Lundgren, Bergman, Trepel, Le Roux, Monsarrat, Kristensen, Pedersen, Pereyra, Tietje and Svenning2024; Trepel et al., Reference Trepel, le Roux, Abraham, Buitenwerf, Kamp, Kristensen, Tietje, Lundgren and Svenning2024), as mentioned earlier.
Beyond herbivory and predation, megafauna also cause other trophic interactions via their generation of various biotic microhabitats and resources, notably their dung, living bodies, and carcasses, with numerous dependent biota (e.g., Galetti et al., Reference Galetti, Moleón, Jordano, Pires, Guimarães, Pape, Nichols, Hansen, Olesen, Munk, de Mattos, Schweiger, Owen-Smith, Johnson, Marquis and Svenning2018). Reflecting this, such dependent groups have seen extinctions as well as declines, for example scavenging birds and dung beetles (e.g., Galetti et al., Reference Galetti, Moleón, Jordano, Pires, Guimarães, Pape, Nichols, Hansen, Olesen, Munk, de Mattos, Schweiger, Owen-Smith, Johnson, Marquis and Svenning2018; Schweiger and Svenning, Reference Schweiger and Svenning2018). In addition to interactions between trophic levels, species also interact within trophic levels in megafauna communities through competition and facilitation, which have cascading impacts on lower trophic levels. A classic example is the successive migration of herbivores in the Serengeti, where smaller animals follow larger animals to benefit from nutritious regrowth (Bell, Reference Bell1971; Herrik et al., Reference Herrik, Svenning and Buitenwerf2023; Anderson et al., Reference Anderson, Hepler, Holdo, Donaldson, Erhardt, Hopcraft, Hutchinson, Huebner, Morrison, Muday, Munuo, Palmer, Pansu, Pringle, Sketch and Packer2024). Intra-trophic level interactions among large herbivores have also been inferred from the Cenozoic fossil record (e.g., Mondanaro et al., 2017). Overall, it is clear that the extinction and extirpation of megafauna in the late Quaternary must have directly and severely reduced trophic interactions within mammal communities (Fricke et al., Reference Fricke, Ordonez, Rogers and Svenning2022) and with other organism groups (e.g., Galetti et al., Reference Galetti, Moleón, Jordano, Pires, Guimarães, Pape, Nichols, Hansen, Olesen, Munk, de Mattos, Schweiger, Owen-Smith, Johnson, Marquis and Svenning2018), for example through strongly reduced vegetation consumption (Alroy, Reference Alroy2001; Pedersen et al., Reference Pedersen, Faurby and Svenning2023; Davoli et al., Reference Davoli, Monsarrat, Pedersen, Scussolini, Karger, Normand and Svenning2024).
Aside from direct trophic impacts on other organisms, megafauna have potentially large indirect effects on ecosystems through the manipulation of natural fire regimes, with large-scale implications for vegetation structure and functioning, potentially even affecting biome distributions and biogeochemical fluxes with Earth-system relevance (e.g., Archibald and Hempson, Reference Archibald and Hempson2016; Foster et al., Reference Foster, Banks, Cary, Johnson, Lindenmayer and Valentine2020; Schmitz et al., Reference Schmitz, Sylvén, Atwood, Bakker, Berzaghi, Brodie, Cromsigt, Davies, Leroux, Schepers, Smith, Stark, Svenning, Tilker and Ylänne2023; Figure 5). By consuming plant biomass herbivores reduce fuel loads, often reducing local fire frequency and intensity (e.g., Foster et al., Reference Foster, Banks, Cary, Johnson, Lindenmayer and Valentine2020). That fire-herbivore interactions can indeed dramatically affect vegetation states is directly evident from the palaeoecological record, which shows several cases across at least three continents where Late Pleistocene losses of megafauna appear to have enhanced fire regimes, in some cases resulting in large vegetation changes (Gill et al., Reference Gill, Williams, Jackson, Lininger and Robinson2009; Rule et al., Reference Rule, Brook, Haberle, Turney, Kershaw and Johnson2012; Karp et al., Reference Karp, Faith, Marlon and Staver2021). Expectedly, the impact was context-dependent (cf. Foster et al., Reference Foster, Banks, Cary, Johnson, Lindenmayer and Valentine2020), and there are regions where the effects on fire regimes were not apparent (Adeleye et al., Reference Adeleye, Charles Andrew, Gallagher, van der Kaars, de Deckker, Hua and Haberle2023).
Physical habitat engineering
Megafauna species play important roles as physical habitat engineers, partly due to their direct and indirect trophic effects (as outlined above), but also via causing non-trophic disturbances and other activities. In the terrestrial realm, there are many examples of large animals that, by engineering their environment, generate habitats or unlock resources for other species. The digging of water wells, for example, is ubiquitous among elephants and equids (Haynes, Reference Haynes2012; Lundgren et al., Reference Lundgren, Ramp, Stromberg, Wu, Nieto, Sluk, Moeller and Wallach2021). Elephants, specifically due to their large size in combination with a prehensile trunk, have a large potential for engineering environments in additional ways, for example creating hollows by breaking off branches and so generating habitat for hollow-dependent animals (Gordon et al., Reference Gordon, Greve, Henley, Bedetti, Allin and Svenning2023). Other physical engineering actions that are common among ungulates and other large herbivores, and therefore widespread in areas with large-herbivore communities, include the creation of mud wallows, dust pits, and animal trails, with effects on arthropod communities (e.g., Nickell et al., Reference Nickell, Varriano, Plemmons and Moran2018), fire patterns (Foster et al., Reference Foster, Banks, Cary, Johnson, Lindenmayer and Valentine2020), and river ecosystem functioning (Naiman and Rogers, Reference Naiman and Rogers1997). There is direct paleobiological evidence for such engineering effects by extinct late-Quaternary megafauna, for example proboscidean trackways and large burrows made by ground sloths and, potentially, giant armadillos (Haynes, Reference Haynes2012; Lopes et al., Reference Lopes, Frank, Buchmann and Caron2017). A likely widespread and understudied form of physical engineering is soil compaction by large herbivores, which, in interaction with soil disturbance, may shape plant communities (Howison et al., Reference Howison, Olff, van de Koppel and Smit2017; Trepel et al., Reference Trepel, le Roux, Abraham, Buitenwerf, Kamp, Kristensen, Tietje, Lundgren and Svenning2024). Positive feedbacks between repeated grazing and subsequent soil compaction and nutrient input from dung, alternating with ungrazed patches, where bioturbation promotes taller and less palatable plant communities, may drive shifting local mosaics of alternative vegetation states (Howison et al., Reference Howison, Olff, van de Koppel and Smit2017). The impacts of these and other biogenic modifications can be highly persistent, enhancing the impact of such ecosystem engineering (Albertson et al., Reference Albertson, Sklar, Tumolo, Cross, Collins and Woods2024). Further, little is known about the potential impacts of megafauna herbivores at natural densities on soil erosion, what the consequences may be for sediment and nutrient loads in rivers, coastal systems and oceans, and how those loadings may affect downstream communities, primary productivity and other ecological processes at local to global scales (e.g., Subalusky and Post, Reference Subalusky and Post2019).
Transportation of organism and materials
Roughly half of the world’s plant species are dispersed by animals, mainly birds and mammals, which have the capacity to transport even large seeds (Fricke et al., Reference Fricke, Hsieh, Middleton, Gorczynski, Cappello, Sanisidro, Rowan, Svenning and Beaudrot2022). Large mammals are also important for smaller-seeded herbaceous plants, including graminoids, with seeds transported endo- and ectozoochorously (e.g., Baltzinger et al., Reference Baltzinger, Karimi and Shukla2019). Plants have evolved obvious adaptations to cling to animal fur or skin, a clear example of how megafauna have acted as a major force on plant evolution. More subtle adaptations may also exist, for example in many herbs (including grasses) foliage serves to attract herbivores that inadvertently ingest and disperse seeds (Janzen, Reference Janzen1984). The most striking example of plant dispersal adaptation to megafauna are the so-called “megafauna fruits,” with seeds that are too large to be swallowed or carried by smaller animals or with very large fruits with numerous small seeds (Janzen and Martin, Reference Janzen and Martin1982; Guimarães et al., Reference Guimarães, Galetti and Jordano2008). Where their megafauna dispersers are being lost at present, such species experience reduced regeneration and population declines (Galetti et al., Reference Galetti, Moleón, Jordano, Pires, Guimarães, Pape, Nichols, Hansen, Olesen, Munk, de Mattos, Schweiger, Owen-Smith, Johnson, Marquis and Svenning2018). There are many species with megafauna fruits for which their late-Quaternary partner(s) appear to be extinct, and which may consequently have suffered range contractions or even extinctions (e.g., Janzen and Martin, Reference Janzen and Martin1982; Doughty et al., Reference Doughty, Wolf, Morueta-Holme, Jørgensen, Sandel, Violle, Boyle, Kraft, Peet, Enquist, Svenning, Blake and Galetti2016c; Galetti et al., Reference Galetti, Moleón, Jordano, Pires, Guimarães, Pape, Nichols, Hansen, Olesen, Munk, de Mattos, Schweiger, Owen-Smith, Johnson, Marquis and Svenning2018; Onstein et al., Reference Onstein, Baker, Couvreur, Faurby, Herrera-Alsina, Svenning and Kissling2018), as also directly indicated in the Late Pleistocene ecosystem dynamics of southern Australia (Adeleye et al., Reference Adeleye, Charles Andrew, Gallagher, van der Kaars, de Deckker, Hua and Haberle2023; Figure 5). Fossil dung provides direct evidence that megafauna species such as American mastodon (Mammut americanum) dispersed megafauna fruits and many other plant species (e.g., Newsom and Mihlbachler, Reference Newsom, Mihlbachler and Webb2006). Adaptation to dispersal by extinct megafauna is also supported by observations of plants whose regeneration and distribution are promoted primarily by introduced cattle and horses in the Americas (e.g., Janzen and Martin, Reference Janzen and Martin1982; Brown and Archer, Reference Brown and Archer1988).
In addition to being the sole bearers of megafauna fruit seeds and transporting large numbers of smaller seeds, the distance by which these seeds can be moved by megafauna is important, not least given the importance of long-distance dispersal events in modulating range and metapopulation dynamics (e.g., Higgins and Richardson, Reference Higgins and Richardson1999). Potential dispersal distance by terrestrial animals scales with body size, as larger animals have greater stride length, larger home ranges, and longer gut retention times (Berti and Svenning, Reference Berti and Svenning2020; Berti et al., Reference Berti, Davoli, Buitenwerf, Dyer, Hansen, Hirt, Svenning, Terlau, Brose and Vollrath2021; Fricke et al., Reference Fricke, Hsieh, Middleton, Gorczynski, Cappello, Sanisidro, Rowan, Svenning and Beaudrot2022). Similar impacts may apply to the transportation of small-bodied organisms, including parasites such as ticks (Galetti et al., Reference Galetti, Moleón, Jordano, Pires, Guimarães, Pape, Nichols, Hansen, Olesen, Munk, de Mattos, Schweiger, Owen-Smith, Johnson, Marquis and Svenning2018) but also microorganisms, including disease agents, dung-associated fungi, and potentially mycorrhizal fungi (e.g., Doughty et al., Reference Doughty, Prys‐Jones, Faurby, Abraham, Hepp, Leshyk, Fofanov, Nieto, Svenning and Galetti2020). As a result, movement rates for plants as well as gut-transported microorganisms are estimated to have been strongly reduced by the late-Quaternary megafauna extinctions (Berti and Svenning, Reference Berti and Svenning2020; Doughty et al., Reference Doughty, Prys‐Jones, Faurby, Abraham, Hepp, Leshyk, Fofanov, Nieto, Svenning and Galetti2020; Fricke et al., Reference Fricke, Hsieh, Middleton, Gorczynski, Cappello, Sanisidro, Rowan, Svenning and Beaudrot2022).
The potential for size-dependent transportation rates and distances by megafauna herbivores also extends to abiotic elements including carbon, a primary resource for arthropods and microorganisms, and plant nutrients such as nitrogen, phosphorus, and essential micro-nutrients. These types of effects are estimated to have declined severely with the late-Quaternary megafauna extinctions (Doughty et al., Reference Doughty, Roman, Faurby, Wolf, Haque, Bakker, Malhi, Dunning and Svenning2016b). Megafauna contribute to shaping nutrient landscapes by increasing nutrient availability and redistributing them spatially. By consuming plant material, herbivorous megafauna liberate nutrients and reduce the amount of nutrients locked up in structural tissue, which can boost ecosystem productivity (McNaughton et al., Reference McNaughton, Ruess and Seagle1988; Augustine et al., Reference Augustine, McNaughton and Frank2003). The ability of larger animals to digest and extract nutrients from large amounts of lower-quality material enables them to access nutrients locked away in more recalcitrant plant tissue and thus release them in forms more readily available for plant uptake (McNaughton et al., Reference McNaughton, Ruess and Seagle1988). Importantly, megafauna-driven nutrient distribution can create fundamentally different spatiotemporal patterns from those created by abiotic forces (McInturf et al., Reference McInturf, Pollack, Yang and Spiegel2019). For example, gravity propels water to accumulate nutrients in low-lying areas, gradually transporting it to coastlines, whereas mammals can move nutrients in dung against the forces of gravity uphill and back toward continental interiors (Doughty et al., Reference Doughty, Roman, Faurby, Wolf, Haque, Bakker, Malhi, Dunning and Svenning2016b). As a noteworthy case, hippopotamus dung is responsible for 76% of total silicon flux along East African rivers, thereby strongly affecting downstream primary production and communities (Schoelynck et al., Reference Schoelynck, Subalusky, Struyf, Dutton, Unzué-Belmonte, Van de Vijver, Post, Rosi, Meire and Frings2019). In addition to depositing dung, the nutrient composition of an animal’s body is notably distinct from the ratios in which they exist in the environment. Carcasses can therefore generate distinct local nutrient hotspots, with impacts on ecological processes such as plant productivity (Towne, Reference Towne2000) and carrion-associated species (van Klink et al., Reference van Klink, van Laar-Wiersma, Vorst and Smit2020). These smaller-scale patterns and processes have the potential to translate to larger scales and affect, for example, the climate system, but this remains poorly understood (Malhi et al., Reference Malhi, Doughty, Galetti, Smith, Svenning and Terborgh2016; Pringle et al., Reference Pringle, Abraham, Anderson, Coverdale, Davies, Dutton, Gaylard, Goheen, Holdo, Hutchinson, Kimuyu, Long, Subalusky and Veldhuis2023; Smith et al., Reference Smith, Elliott Smith, Hedberg, Lyons, Pardi and Tomé2023).
Large-scale effects
While there exists a wealth of evidence for smaller-scale patterns and processes with potential to translate to larger scales and affect, for example, the climate system, the occurrence of such effects remains incompletely understood, both in relation to the late-Quaternary extinctions and in context of potential, broad-scale megafauna restoration (Malhi et al., Reference Malhi, Doughty, Galetti, Smith, Svenning and Terborgh2016; Pringle et al., Reference Pringle, Abraham, Anderson, Coverdale, Davies, Dutton, Gaylard, Goheen, Holdo, Hutchinson, Kimuyu, Long, Subalusky and Veldhuis2023; Smith et al., Reference Smith, Elliott Smith, Hedberg, Lyons, Pardi and Tomé2023). Of particular interest is the degree to which megafauna-mediated processes maintain biodiversity across scales, and especially how these processes are maintained in a rapidly changing world where both climate and other environmental conditions are moving into novel territory, as well as accelerating human-driven redistribution of species. Also important is the magnitude of impact by megafauna on climate through atmospheric carbon, soot and methane (Archibald and Hempson, Reference Archibald and Hempson2016), above- and below-ground carbon stocks (Wigley et al., Reference Wigley, Augustine, Coetsee, Ratnam and Sankaran2020; Kristensen et al., Reference Kristensen, Svenning, Georgiou and Malhi2022; Malhi et al., Reference Malhi, Lander, le Roux, Stevens, Macias-Fauria, Wedding, Girardin, Kristensen, Sandom, Evans, Svenning and Canney2022; Schmitz et al., Reference Schmitz, Sylvén, Atwood, Bakker, Berzaghi, Brodie, Cromsigt, Davies, Leroux, Schepers, Smith, Stark, Svenning, Tilker and Ylänne2023; Smith et al., Reference Smith, Elliott Smith, Hedberg, Lyons, Pardi and Tomé2023), land-surface albedo (Cromsigt et al., Reference Cromsigt, te Beest, Kerley, Landman, le Roux and Smith2018; Malhi et al., Reference Malhi, Lander, le Roux, Stevens, Macias-Fauria, Wedding, Girardin, Kristensen, Sandom, Evans, Svenning and Canney2022), the extent and depth of permafrost (Macias-Fauria et al., Reference Macias-Fauria, Jepson, Zimov and Malhi2020), and modulation of fire regimes (Malhi et al., Reference Malhi, Lander, le Roux, Stevens, Macias-Fauria, Wedding, Girardin, Kristensen, Sandom, Evans, Svenning and Canney2022). Although these studies offer insights into the potential influence of megafauna on climate, accurately assessing their collective effects on the Earth’s system—across past, present, and future timelines—continues to be a complex challenge.
Megafauna management under global change
The recent, severe losses of megafauna worldwide and the associated ecological consequences have driven attention to the fact that current ecosystems, even in perceived wilderness areas, are in functionally novel, compromised states relative to a deep-time norm, but also to implications for land management in the Anthropocene (e.g., Martin, Reference Martin1967; Donlan et al., Reference Donlan, Berger, Bock, Bock, Burney, Estes, Foreman, Martin, Roemer, Smith, Soulé and Greene2006; Svenning, Reference Svenning2020; Svenning et al., Reference Svenning, Buitenwerf and Le Roux2024). In this section, we discuss three important such issues, namely the integration of megafauna into restoration actions, the management of alien and perceived alien megafauna, and ecological impacts of livestock. All are important not just for the future of Earth’s megafauna, but also broadly for biodiversity and ecosystem functioning in the Anthropocene.
Megafauna-based trophic rewilding as a restoration approach
Trophic rewilding is defined as a restoration approach that aims to reestablish self-sustaining complex, biodiverse ecosystems by restoring top-down trophic processes, with emphasis on the reestablishment of large animals given their high ecological importance, but widespread elimination (Svenning et al., Reference Svenning, Pedersen, Donlan, Ejrnæs, Faurby, Galetti, Hansen, Sandel, Sandom, Terborgh and Vera2016, Reference Svenning, Buitenwerf and Le Roux2024). Rewilding more broadly focuses on restoring ecological processes alongside reducing human pressures (e.g., Perino et al., Reference Perino, Pereira, Navarro, Fernández, Bullock, Ceauu, Cortés-Avizanda, van Klink, Kuemmerle, Lomba, Pe’er, Plieninger, Rey Benayas, Sandom, J-C and Wheeler2019). Rewilding is usually conceptualized as an open-ended, forward-looking approach aiming to promote trajectories of high functionality for biodiversity as opposed to the common focus on fixed ecological targets in conventional restoration (e.g., Perino et al., Reference Perino, Pereira, Navarro, Fernández, Bullock, Ceauu, Cortés-Avizanda, van Klink, Kuemmerle, Lomba, Pe’er, Plieninger, Rey Benayas, Sandom, J-C and Wheeler2019; Svenning et al., Reference Svenning, Buitenwerf and Le Roux2024). The need to restore self-sustaining and resilient, yet dynamic ecosystems is especially pertinent given ongoing global change, not least climate change and rising human-mediated globalization (Svenning et al., Reference Svenning, Buitenwerf and Le Roux2024).
As trophic rewilding is a young field the number of empirical studies on self-identified rewilding efforts remains limited, albeit strongly rising (Svenning et al., Reference Svenning, Pedersen, Donlan, Ejrnæs, Faurby, Galetti, Hansen, Sandel, Sandom, Terborgh and Vera2016; Hart et al., Reference Hart, Haigh and Ciuti2023). However, there are many empirical studies on the ecosystem effects and biodiversity outcomes of de facto trophic rewilding, especially in Europe, North America, and southern Africa, often under names such as year-round or extensive grazing, or reintroductions, e.g., with many showing positive biodiversity effects (especially for plants) attributable to bison (e.g, Ratajczak et al., Reference Ratajczak, Collins, Blair, Koerner, Louthan, Smith, Taylor and Nippert2022) or feral and semi-feral cattle and horses (e.g., Konvička et al., Reference Konvička, Ričl, Vodičková, Beneš and Jirků2021; Dvorský et al., Reference Dvorský, Mudrák, Doležal and Jirků2022; Bonavent et al., Reference Bonavent, Olsen, Ejrnæs, Fløjgaard, Hansen, Normand, Svenning and Bruun2023; Köhler et al., Reference Köhler, Schmidt, Hölzel, Baasch and Tischew2023). Further, several long-term cases of megafauna recovery that did not necessarily emerge out of rewilding principles show how trophic rewilding can result in large-scale top-down effects on ecosystems, for example vegetation changes in response to the reestablishment of white rhinoceros (Ceratotherium simum) (Cromsigt and te Beest, Reference Cromsigt and te Beest2014) and African savanna elephants (Gordon et al., Reference Gordon, Greve, Henley, Bedetti, Allin and Svenning2023). Further, there is evidence that conserving or restoring large-herbivore assemblages helps protect against negative impacts of global change on biodiversity. Examples include limiting warming-induced woody plant dominance to the benefit of low-growing tundra plants, lichens, and fungi (Post et al., Reference Post, Kaarlejärvi, Macias-Fauria, Watts, Bøving, Cahoon, Higgins, John, Kerby, Pedersen, Post and Sullivan2023), and reducing invasive alien plant abundance to the benefit of native plant diversity (Guyton et al., Reference Guyton, Pansu, Hutchinson, Kartzinel, Potter, Coverdale, Daskin, da Conceição, Peel, Stalmans and Pringle2020; Mungi et al., Reference Mungi, Jhala, Qureshi, Le Roux and Svenning2023; Svenning et al., Reference Svenning, Buitenwerf and Le Roux2024). In addition to generating ecological effects and benefitting other species, trophic rewilding can also play a role in the conservation and restoration of wild megafauna, which are among the most endangered functional groups on the planet (Ripple et al., Reference Ripple, Chapron, López-Bao, Durant, Macdonald, Lindsey, Bennett, Beschta, Bruskotter, Campos-Arceiz, Corlett, Darimont, Dickman, Dirzo, Dublin, Estes, Everatt, Galetti, Goswami, Hayward, Hedges, Hoffmann, Hunter, Kerley, Letnic, Levi, Maisels, Morrison, Nelson, Newsome, Painter, Pringle, Sandom, Terborgh, Treves, Valkenburgh, Vucetich, Wirsing, Wallach, Wolf, Woodroffe, Young and Zhang2016; Atwood et al., Reference Atwood, Valentine, Hammill, McCauley, Madin, Beard and Pearse2020).
Functional effects of non-native megafauna
While the impact of humans on megafauna has generally been a driver of declines and extinctions, a surprising counter-current has occurred over the last couple of centuries through inadvertent megafauna introductions. At least 22 megafauna species (≥100 kg) have established wild populations around the world, representing 29% of the total remaining megafauna species (Lundgren et al., Reference Lundgren, Ramp, Ripple and Wallach2018). Fifty percent of these introduced megafauna are threatened or extinct in their native ranges, suggesting novel albeit contentious conservation opportunities (Lundgren et al., Reference Lundgren, Ramp, Ripple and Wallach2018). Collectively, these introductions have restored as much as 15–67% of lost late-Quaternary megafauna species richness in each continent (Lundgren et al., Reference Lundgren, Ramp, Ripple and Wallach2018). and have restored 39% of lost functional richness, producing functional compositions more similar to those of the Late Pleistocene than assemblages composed only of native species (Lundgren et al., Reference Lundgren, Ramp, Rowan, Middleton, Schowanek, Sanisidro, Carroll, Davis, Sandom, Svenning and Wallach2020). Indeed, many introduced megafauna are close functional analogs of extinct species (e.g., introduced wild boar [Sus scrofa] bear some similarities to extinct peccaries like Platygonus compressus) or are conspecific or nearly so with extirpated species (introduced horses – Equus ferus – in the Americas) (Lundgren et al., Reference Lundgren, Ramp, Rowan, Middleton, Schowanek, Sanisidro, Carroll, Davis, Sandom, Svenning and Wallach2020). Other introduced megafauna are phylogenetically distinct from any prehistoric megafauna but still share trait resemblance, for example for Australia there is no species more ecologically similar to the extinct marsupial Palorchestes azael than the introduced dromedary Camelus dromedarius, based on available functional traits (Lundgren et al., Reference Lundgren, Ramp, Rowan, Middleton, Schowanek, Sanisidro, Carroll, Davis, Sandom, Svenning and Wallach2020).
Despite their similarity to extinct species, the effects of introduced megafauna are generally considered ecologically harmful and aberrant relative to native megafauna, thereby justifying eradication and control programs, even when introduced megafauna are the same species as Late Pleistocene native species (e.g., wild horses in North America). However, meta-analyses of introduced and native megafauna impacts have found no evidence that nativeness shapes their effects on plants (Lundgren et al., Reference Lundgren, Bergman, Trepel, Le Roux, Monsarrat, Kristensen, Pedersen, Pereyra, Tietje and Svenning2024) or on other dimensions of ecosystems, such as small mammals and birds or soil and plant nutrients (Trepel et al., Reference Trepel, le Roux, Abraham, Buitenwerf, Kamp, Kristensen, Tietje, Lundgren and Svenning2024). This suggests that our understanding of introduced organisms would benefit from critical re-assessment and increased transparency about how we assign “harm” to the effects of organisms and that a dogmatic emphasis on nativeness may be unhelpful to broader conservation goals.
Further research on the impacts of introduced megafauna, especially at broader scales (Trepel et al., Reference Trepel, le Roux, Abraham, Buitenwerf, Kamp, Kristensen, Tietje, Lundgren and Svenning2024) is necessary to understand if and how these organisms restore lost or novel beneficial ecological processes. Some initial research in this domain supports that introduced megafauna may have positive ecological effects. For example, a long-term before-after control-impact study on introduced wild water buffalo in Northern Australia found that buffalo grazing reduced wildfire frequency and severity and increased tree establishment and growth rates (Werner, Reference Werner2005). Feral pigs, among the most widely introduced and persecuted megafauna species, can increase native plant species richness by controlling competitive dominants (Cushman et al., Reference Cushman, Tierney and Hinds2004; Cuevas et al., Reference Cuevas, Campos, Ojeda and Jaksic2020; Hensel et al., Reference Hensel, Silliman, Hensel and Byrnes2022); increase food availability for avifauna (Natusch et al., Reference Natusch, Mayer, Lyons and Shine2017) and increase nutrient availability and thus tree growth rates (Lacki and Lancia, Reference Lacki and Lancia1986). Feral equids increase water availability in drylands through well-digging (Lundgren et al., Reference Lundgren, Ramp, Stromberg, Wu, Nieto, Sluk, Moeller and Wallach2021) and maintain open water habitats in desert wetlands, with their removal linked to the extinction of endemic fish populations (Kodric-Brown and Brown, Reference Kodric-Brown and Brown2007).
Studies on the effects of introduced megafauna should take widespread predator persecution into account, which can modulate the impacts of small- to medium-sized large herbivores, native or non-native (Wallach et al., Reference Wallach, Ripple and Carroll2015). In North America, protected cougar populations are major predators of feral horses (Andreasen et al., Reference Andreasen, Stewart, Longland and Beckmann2021) and can limit population growth if in habitat with sufficient ambush cover (Turner and Morrison, Reference Turner and Morrison2001). Likewise, feral donkeys respond to cougar predation by reducing their activity rates at desert wetlands, with associated reductions in their impacts on wetland vegetation (Lundgren et al., Reference Lundgren, Ramp, Middleton, Wooster, Kusch, Balisi, Ripple, Hasselerharm, Sanchez, Mills and Wallach2022). While conservationists readily recognize that the undesirable impacts of native megafauna, such as heavy browsing by deer (Côté et al., Reference Côté, Rooney, Tremblay, Dussault and Waller2004; Martin et al., Reference Martin, Chamaillé-Jammes and Waller2020) are a result of apex predator extirpation or persecution, conservation has been slow to accept that the same could be true for introduced megafauna. However, conversely, it is important to avoid anthropogenic low-density baselines for large herbivores (Fløjgaard et al., Reference Fløjgaard, Pedersen, Sandom, Svenning and Ejrnæs2022). Further, it is important to note that under non-defaunated circumstances there are usually herbivores present which do not experience top-down regulation but are bottom-up regulated by food resources and abiotic stressors (as discussed earlier).
Functionality of domestic livestock
Global declines of wild megafauna have been followed by large increases in domestic livestock densities (Bar-On et al., Reference Bar-On, Phillips and Milo2018). Further, in areas such as the Amazon and Chaco regions clearing of natural vegetation to make space for cattle production is rampant to this day. In defaunated systems domestic megafauna may, under certain conditions, replace some of the ecological functions and processes previously provided by wild megafauna. However, there is a need to establish greater clarity on the ecological functionality of cattle (and other livestock) and the ecological contexts under which they may manifest, not least because a global livestock farming lobby claims high-density and production-focused cattle ranching as a means to ecosystem restoration and climate change mitigation (Hawkins et al., Reference Hawkins, Venter and Cramer2022). Importantly, there are key differences between wild megafauna and domestic livestock. First, the effects of domestic livestock are a function of husbandry practices, in addition to essential aspects of the animal’s biology. Modern husbandry practices, particularly in affluent countries where the cost of labor is high, generally involve hands-off approaches to grazing including the removal of apex predators, fencing, the maintenance of female-only herds, yearly removals of livestock to feedlots, and inoculation against disease. Notably, when livestock is kept indoors or in small, densely packed pens in industrial agriculture, any natural ecological functionality is lost. Even in more extensive settings, such as with year-round, low-density grazing on unimproved pastures, the situation is complex. High stocking densities (>1 livestock/ha) are associated with generalized negative effects on animal biodiversity (Sartorello et al., Reference Sartorello, Pastorino, Bogliani, Ghidotti, Viterbi and Cerrato2020). Husbandry practices can lead to stationary herds of livestock, for example, by providing supplementary feed, water or mineral licks at fixed locations. Movement is often further restricted by fencing, which can fragment other wild megafauna populations (Jakes et al., Reference Jakes, Jones, Paige, Seidler and Huijser2018) and may prevent livestock from performing key megafauna functions such as nutrient and seed dispersal. Finally, modern livestock production also involves shipping animals off-site for slaughter and processing and the disposal of carcasses. This may constitute a form of nutrient-mining in grazed landscapes where the amount of minerals removed may parallel primary sources like atmospheric deposition or weathering rates (Abraham et al., Reference Abraham, Webster, Prys-Jones, le Roux, Smith, McFayden, de Jager, Clauss and Doughty2021). In regions with low nutrient deposition or low weathering rates, this could have deleterious effects on potential primary productivity, even if there is appropriate management for grazing impacts.
Despite the differences between livestock ranching and free-roaming wildlife, livestock in combination with other human impacts may maintain certain ecological processes that were formerly sustained by wild megafauna (Gordon et al., Reference Gordon, Pérez-Barbería and Manning2021). For example, traditional extensive livestock practices in Europe were able to generate and maintain open vegetation and associated biodiversity in the Holocene (Navarro and Pereira, Reference Navarro and Pereira2012) and can still do so both there (Troiano et al., Reference Troiano, Buglione, Petrelli, Belardinelli, De Natale, Svenning and Fulgione2021) and elsewhere, for example in the Argentinean Pampas (Aranda et al., Reference Aranda, Tognetti, Mochi and Mazía2023) and Brazilian Cerrado (Durigan et al., Reference Durigan, Pilon, Souza, Melo, Ré and Souza2022). This can result in reduced dominance of competitive plant species, resulting in greater stability in grassland productivity (Campana et al., Reference Campana, Tognetti and Yahdjian2022) and increased diversity in plants and other taxa such as birds (Frutos et al., Reference Frutos, Ronchi-Virgolini, Giraudo and Piña2020). Similar to wild megafauna, livestock can also reduce fire frequency, intensity, and size in grassy ecosystems around the world (Probert et al., Reference Probert, Parr, Holdo, Anderson, Archibald, Courtney Mustaphi, Dobson, Donaldson, Hopcraft, Hempson, Morrison and Beale2019; Durigan et al., Reference Durigan, Pilon, Souza, Melo, Ré and Souza2022), and likely also in Mediterranean ecosystems. It has been known for decades that livestock can disperse plant seeds adapted to dispersal by wild megafauna (e.g., Janzen and Martin, Reference Janzen and Martin1982; Bruun and Fritzbøger, Reference Bruun and Fritzbøger2002; Auffret et al., Reference Auffret, Schmucki, Reimark and Cousins2012). However, different livestock species can have different effects on, for example, plant species richness, and more importantly, domestic populations may benefit ecosystem heterogeneity and species diversity less than feral populations, as has been shown for horses (Mutillod et al., Reference Mutillod, Buisson, Tatin, Mahy, Dufrêne, Mesléard and Dutoit2024). While it is clear that single-species livestock systems do not generate the same diversity of ecosystem impacts as a diverse wild megafauna assemblage, these results suggest that extensive livestock practices, under certain conditions, can partly replace lost ecosystem functionality (Gordon et al., Reference Gordon, Pérez-Barbería and Manning2021).
At the same time it is important to recognize that while traditional herding practices can be compatible with some forms of wildlife conservation, especially with smaller herbivores (e.g., Fynn et al., Reference Fynn, Augustine, Peel and de Garine-Wichatitsky2016; Herrik et al., Reference Herrik, Svenning and Buitenwerf2023; Simba et al., Reference Simba, te Beest, Hawkins, Larson, Palmer, Sandström, Smart, Kerley and Cromsigt2024), larger wildlife species such as buffalo and elephant are likely to experience competition from livestock grazing (Herrik et al., Reference Herrik, Svenning and Buitenwerf2023), especially at high cattle densities (Wells et al., Reference Wells, Crego, Ekadeli, Namoni, Kimuyu, Odadi, Porensky, Dougill, Stringer and Young2022). Finally, driven by socioeconomic dynamics, extensive livestock production is itself disappearing in many parts of the world, with a tendency toward commercialization and intensification of the remaining livestock production. For example, in the Mediterranean region of Europe, grazing land has been steadily abandoned over recent decades (e.g., Navarro and Pereira, Reference Navarro and Pereira2012). To replace livestock-mediated ecosystem services such as reducing wildfire risk, a re-expansion of wild megafauna has been advocated (San Miguel-Ayanz et al., Reference San Miguel-Ayanz, García-Calvo and García-Olalla2010).
Identifying ecosystem restoration approaches for pastoralist and livestock ranching systems is likely to become increasingly important as meat consumption is expected to increase due to rising affluence and human population (Revell, Reference Revell2015), despite a strong potential for sustainability in shifting toward highly plant-based diets (e.g., Sun et al., Reference Sun, Scherer, Tukker, Spawn-Lee, Bruckner, Gibbs and Behrens2022). Moreover, societies in semi-arid and arid landscapes that are unsuitable for crop production are likely to maintain dependence on livestock as a source of subsistence and income, which is likely a more ecologically friendly method of food production than irrigation agriculture in these environments. Therefore, an important avenue of future research – complementing research on how to reduce meat consumption globally – would be on how to preserve and rejuvenate modern husbandry practices that facilitate natural megafauna functions while mitigating negative impacts on biodiversity (including wild megafauna) and ecosystems, for example, by mixing extensive livestock grazing with wildlife (e.g., Herrik et al., Reference Herrik, Svenning and Buitenwerf2023; Simba et al., Reference Simba, te Beest, Hawkins, Larson, Palmer, Sandström, Smart, Kerley and Cromsigt2024), as already suggested by Martin (Reference Martin1967). Further, replacing ruminant livestock with non-ruminants would also substantially reduce greenhouse gas emissions (Cromsigt et al., Reference Cromsigt, te Beest, Kerley, Landman, le Roux and Smith2018).
Conclusion
The severe losses of large-bodied animals in the near prehistory, notably the Late Pleistocene and Early to Middle Holocene, have stimulated interest and scientific debate for decades and continue to do so. However, much evidence on patterns and drivers has emerged in the last 20 years, and our understanding is now much clearer (Table 2). The strong, size-biased megafauna extinctions across the last ~50,000 years are a global pattern, even affecting sub-Saharan Africa albeit less strongly than elsewhere (Figure 1), and affecting all major biomes, from the Arctic to the tropics (Figure 2). Further, it is a unique event for the whole Cenozoic, that is, the last 66 million years (Figure 4), demanding a cause distinctive to the late Quaternary. As it affected different landmasses at different times, occurred across an extended time frame within larger regions, and affected groups that were phylogenetically very disparate, explanations relying on an extraterrestrial impact or a disease are untenable (Koch and Barnosky, Reference Koch and Barnosky2006; Holliday et al., Reference Holliday, Daulton, Bartlein, Boslough, Breslawski, Fisher, Jorgeson, Scott, Koeberl, Marlon, Severinghaus, Petaev and Claeys2023). The Late Pleistocene saw massive climate changes, and these continue to be put forward as a potential explanation for the megafauna extinctions. However, these changes were no more severe than earlier in the Pleistocene, with the earlier climatic dynamics not causing selective, global megafauna losses. Further, our review shows that there is weak or no support for any major influence of climate on the late-Quaternary extinctions, neither by coarse-grained macroecological patterns nor by detailed spatial/temporal and mechanistic evidence, with much evidence directly against a climatic cause. Conversely, there is strong, cumulative support for direct and indirect pressures from behaviourally modern humans as the key driver. The global expansion of Homo sapiens is indeed unique to the late Quaternary, and there is much evidence for the sophisticated megafauna hunting capabilities in even early behaviourally modern humans in various regions. Crucially, the extinctions exhibit strong links in severity to general human biogeography and are spatiotemporally linked to modern humans at the global level and, with increasing evidence also at finer scales, with extinction concentrated at the arrival of H. sapiens or happening later, as expected from ongoing socio-technological development (cf. Ellis, Reference Ellis2015). Interestingly, there is emerging evidence that the initial onset of the extinctions may have occurred prior to the Late Pleistocene in regions where pre-sapiens hominins would also have exerted pressure on megafauna, albeit to a lesser extent. However, this remains contested, and in the context of the broader Plio-Pleistocene, it is certainly likely that climatic stress and associated declining vegetation productivity played a role in these limited early megafauna losses (e.g., Bibi and Cantalapiedra, Reference Bibi and Cantalapiedra2023), as also seen in other organism groups in various regions (e.g., Raffi et al., Reference Raffi, Stanley and Marasti1985; Svenning, Reference Svenning2003; Magri et al., Reference Magri, Di Rita, Aranbarri, Fletcher and González-Sampériz2017; Mooney et al., Reference Mooney, Sniderman, Kershaw, Haberle, Roe and Keith2017).
A broad range of empirical and theoretical evidence shows that the late-Quaternary extinctions have or must have elicited profound changes to the structure and functioning of terrestrial ecosystems worldwide. As such, the late-Quaternary extinctions represent an early, human-driven environmental transformation at a large geographic scale and hence a progenitor of the Anthropocene, where humans have emerged as a major player in planetary functioning. We further conclude that even partial megafauna restoration – notably as implemented via trophic rewilding (Svenning et al., Reference Svenning, Pedersen, Donlan, Ejrnæs, Faurby, Galetti, Hansen, Sandel, Sandom, Terborgh and Vera2016, Reference Svenning, Buitenwerf and Le Roux2024) – is likely to have positive effects on biodiversity and ecosystem functioning, given that most extant species have evolved in ecosystems with rich large-animal faunas and given the general functional effects of large animals. Still, outcomes are expected to exhibit context- and scale-dependence and be modulated by the functional composition of the fauna, notably its functional trait and trophic composition (e.g., Le Roux et al., Reference Le Roux, Marneweck, Clinning, Druce, Kerley and Cromsigt2019; Lundgren et al., Reference Lundgren, Bergman, Trepel, Le Roux, Monsarrat, Kristensen, Pedersen, Pereyra, Tietje and Svenning2024). Further, the existing evidence indicates positive potential for biodiversity and ecosystem functioning in wild-living, non-native megafauna in many circumstances as well as, in the context of facilitating biodiversity in production landscapes (land sharing), in non-intensive husbandry practices, designed to facilitate natural megafauna functions. We note that the potential benefits of megafauna restoration remain, regardless of whether the late-Quaternary extinctions were caused by H. sapiens or, contrary to the totality of the evidence, past climate stress.
The overall pattern and amount of evidence for H. sapiens playing the dominant role in the megafauna extinctions is perhaps one of the clearest, well-supported patterns in ecology (Table 2). However, key open questions concern the detailed mechanisms involved, for example, the role of coextinctions and the role of pre-sapiens hominins in limited prior megafauna losses, and much remains to be learnt on the ecological impacts of the extinctions (Figure 5). Further, while there is already much empirical work on trophic rewilding, much more – in differing ecological and societal contexts and via large, well-designed experiments – would be valuable. A key point of attention here should be on trophic rewilding in context of the global emergence of novel ecosystems (Svenning et al., Reference Svenning, Buitenwerf and Le Roux2024) due to anthropogenic climate change, alien species introductions, and other human environmental transformations (e.g., Guyton et al., Reference Guyton, Pansu, Hutchinson, Kartzinel, Potter, Coverdale, Daskin, da Conceição, Peel, Stalmans and Pringle2020; Mungi et al., Reference Mungi, Jhala, Qureshi, Le Roux and Svenning2023; Post et al., Reference Post, Kaarlejärvi, Macias-Fauria, Watts, Bøving, Cahoon, Higgins, John, Kerby, Pedersen, Post and Sullivan2023).
Open peer review
To view the open peer review materials for this article, please visit http://doi.org/10.1017/ext.2024.4.
Data availability statement
No new data are reported in this review.
Author contribution
All authors contributed substantially to the conceptualization and writing of the manuscript.
Financial support
We received economic support from VILLUM FONDEN via JCS’ VILLUM Investigator project “Biodiversity Dynamics in a Changing World” (grant 16549), the Danish National Research Foundation via Center for Ecological Dynamics in a Novel Biosphere (ECONOVO) (grant DNRF173 to J.-C.S.) and Independent Research Fund Denmark | Natural Sciences via the MegaComplexity project (grant 0135-00225B to J.-C.S.).
Competing interest
The authors declare no competing interests exist.
Comments
Dear editors,
I’m happy to submit our commissioned review on the late-Quaternary megafauna extinctions. Thanks a lot for the opportunity to senior editor S. Kathleen Lyons and the rest of the team. I hope you and the reviewers will like it - it has certainly been a pleasure writing it and am convinced that it will be of interest and utility to ecologists, evolutionary biologists, paleobiologists, and conservation biologists broadly.
Yours sincerely,
Jens-Christian Svenning
PS: I do not think we have any competing interests to declare. I am a pro bono member of the supervisory board for the NGO rewilding Europe, and I and others in the team are also involved with providing our best scientific advice to various other conservation-related organizations, but I don’t think such efforts fall into this category.