Scientific Classification
Domain: Eukaryota
Kingdom: Plantae
Phylum: Spermatophyta
Subphylum: Angiospermae
Class: Dicotyledonae
Order: Gentianales
Family: Apocynaceae
Subfamily: Asclepiadoideae
Tribe: Asclepiadeae
Subtribe: Tylophorinae
Genus: Vincetoxicum Wolf
Species 1: nigrum (L.) Moench
Synonyms: Cynanchum louiseae Kartesz & Gandhi, Cynanchum nigrum (L.) Pers.
EPPO Code: CYKNI
Species 2: rossicum (Kleopow) Barbarich
Synonyms: Cynanchum rossicum Kleopow, Cynanchum rossicum (Kleopow) Borhidi, Vincetoxicum officinale var. rossicum (Kleopow) Grodz.
EPPO Code: VNCRO
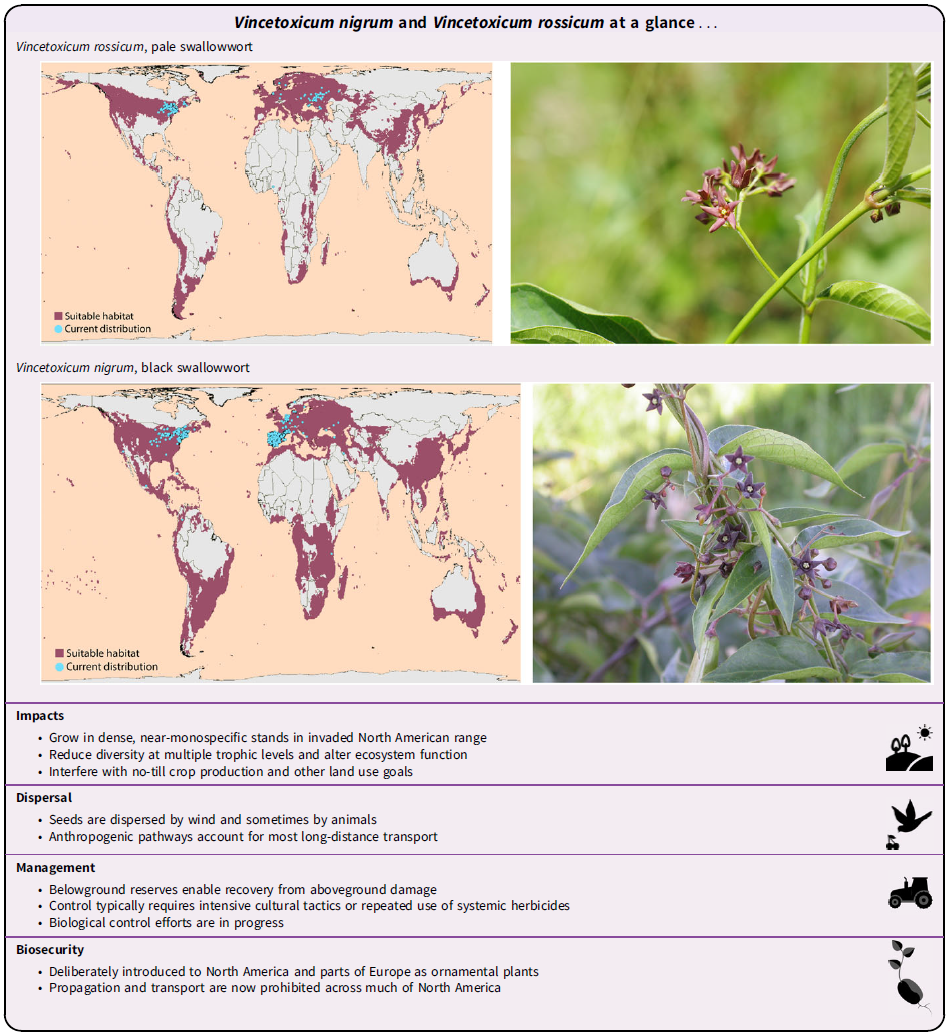
Name and Taxonomy
Vincetoxicum comes from the Latin vinco (to conquer or subdue) + toxicum (poison), for the supposed use of these plants as an antidote for poison (DiTommaso et al. Reference DiTommaso, Lawlor and Darbyshire2005b). The specific epithet rossicum is a Latinized reference to the presumed origin of V. rossicum in Russia, whereas nigrum is Latin for “black” in reference to the flower color of V. nigrum. The identities of North American specimens of V. nigrum and V. rossicum were confirmed by Gaina Konechnaya and Nikolai Tsvelev, Institute of Botany, Russian Academy of Sciences, St Petersburg, Russia (personal communication). In addition to the two invasive Vincetoxicum species, a third species, Vincetoxicum hirundinaria Medik. [syn.: Cynanchum vincetoxicum (L.) Pers., Vincetoxicum officinale Moench], occupies a large native range in Eurasia but appears only to be a rare garden escape elsewhere, not showing invasive tendencies like V. rossicum and V. nigrum. Some workers have considered V. rossicum to be a dark-flowered form of the V. hirundinaria species complex (Gleason and Cronquist Reference Gleason and Cronquist1991; Lauvanger and Borgen Reference Lauvanger and Borgen1998) as hybrids between the two are known (Markgraf Reference Markgraf1971).
The common name pale swallowwort (or the more widely used hyphenated form “pale swallow-wort,” both used in the United States) for V. rossicum may be confusing because the pink or maroon flowers of V. rossicum are paler than the dark purple flowers of V. nigrum, but darker than the cream-colored flowers of V. hirundinaria (DiTommaso et al. Reference DiTommaso, Lawlor and Darbyshire2005b). Alternate common names for V. rossicum include European swallowwort (for its region of origin), dog-strangling vine (for its ability to form dense mats, Canada), dompte-venin de Russie (Canada), and russesvalerot (Norway). Common names for V. nigrum include black swallowwort (or black swallow-wort, United States), Louise’s swallowwort (based on one of its synonyms), black dog-strangling vine (Canada), and dompte-venin noir (Canada). In addition to white swallowwort, common names for V. hirundinaria include German ipecac, poison-rope swallowwort (Canada), white dog-strangling vine (Canada), and common vincetoxicum (Belgium) (references in Global Biodiversity Information Facility 2021, 2022a, 2022b).
The genus Vincetoxicum N.M. Wolf (1776) is placed within the subtribe Tylophorinae in the tribe Asclepiadeae in the subfamily Asclepiadoideae in the family Apocynaceae. The incorporation of the former family Asclepiadaceae into the Apocynaceae was required to make the Apocynaceae monophyletic (Endress and Bruyns Reference Endress and Bruyns2000). The family Asclepiadaceae was not reduced to a tribe or subfamily, as erroneously reported (DiTommaso et al. Reference DiTommaso, Lawlor and Darbyshire2005b). Instead, the five subfamilies in the revised family Apocynaceae include three subfamilies (Periplocoideae, Secamonoideae, and Asclepiadoideae) that were formerly included in the Asclepiadaceae and two subfamilies (Rauvolfioideae and Apocynoideae) that were already present in the Apocynaceae (Endress et al. Reference Endress, Liede-Schumann and Meve2007; Endress and Bruyns Reference Endress and Bruyns2000). Within the Asclepiadoideae (tribe Asclepiadeae), the subtribe Tylophorinae is closely related to the subtribe Cynanchinae, which contains the genus Cynanchum L. (Fishbein et al. Reference Fishbein, Livshultz, Straub, Simões, Boutte, McDonnell and Foote2018). The relationship between Vincetoxicum and Cynanchum has historically elicited controversy (DiTommaso et al. Reference DiTommaso, Lawlor and Darbyshire2005b; Liede Reference Liede1996), but it is now clear that these two genera should be separated (Endress et al. Reference Endress, Liede-Schumann and Meve2007, Reference Endress, Liede-Schumann and Meve2014).
The subtribe Tylophorinae initially included several genera (Endress et al. Reference Endress, Liede-Schumann and Meve2007; Liede et al. Reference Liede, Täuber and Schneidt2002), most of which were subsequently merged with Vincetoxicum (Endress et al. Reference Endress, Liede-Schumann and Meve2014; Liede-Schumann and Meve Reference Liede-Schumann and Meve2018). The combined group, Vincetoxicum sensu lato, contains at least 140 species native to Africa, Asia, and Europe (Liede-Schumann et al. Reference Liede-Schumann, Khanum, Mumtaz, Gherghel and Pahlevani2016; Liede-Schumann and Meve Reference Liede-Schumann and Meve2018). Over evolutionary time, the species V. rossicum, V. nigrum, and V. hirundinaria emerged from a clade (Vincetoxicum sensu stricto) that expanded northwest along the Asian mountain chains to Europe (Liede-Schumann et al. Reference Liede-Schumann, Khanum, Mumtaz, Gherghel and Pahlevani2016).
The duplicate genus Vincetoxicum T. Walter (1788) (Bullock Reference Bullock1967) was erected for North American species, resulting in several illegitimate synonyms under the name Vincetoxicum. These species are properly placed in the genera Matelea Aubl. and Gonolobus Michx. within the subtribe Gonolobinae (Endress et al. Reference Endress, Liede-Schumann and Meve2014; USDA-NRCS 2021).
Description
Unless otherwise noted, the following information is drawn from the descriptions of North American V. rossicum and V. nigrum presented by DiTommaso et al. (Reference DiTommaso, Lawlor and Darbyshire2005b). These species are perennial herbs or small vines (Figure 1). Although not rhizomatous (Averill et al. Reference Averill, DiTommaso, Mohler and Milbrath2011), they have short, horizontal, semi-woody rootstalks and slightly fleshy roots (Figure 1). Root-to-shoot ratios are often high, particularly in V. rossicum (Milbrath Reference Milbrath2008). Stems are usually 60- to 200-cm long in V. rossicum and 40- to 200-cm long in V. nigrum, but occasionally longer (particularly when climbing up shrubs). Stems are pubescent with the hairs in longitudinal bands (especially in V. rossicum, which also has denser hairs on peduncles and pedicels). Leaves are largest in the middle of the stem, opposite, entire-margined, and pubescent on margins and major veins of the lower surface. Leaves of V. rossicum are ovate to elliptic, acute at the tip, and typically 7 to 12 by 5 to 7 cm with 5- to 20-mm petioles. Leaf bases are often truncate or slightly cuneate. Leaves of V. nigrum are oblong to ovate and typically 5 to 12 by 2 to 6.5 cm with 10- to 15-mm petioles. Leaf bases are often emarginate or cordate in V. nigrum.

Figure 1. (A) Seedlings and small juveniles of Vincetoxicum rossicum. (B) Individual Vincetoxicum rossicum plant. (C) Individual Vincetoxicum nigrum plant. (D) Cross-section of a Vincetoxicum rossicum root system. (E) Two connected rootstocks of Vincetoxicum nigrum (southeastern New York, USA). (Photo credits: (A–C and E) Jeromy Biazzo; (D) Scott Morris.)
Reproductive traits show much more interspecific variation than vegetative traits. Vincetoxicum rossicum flower buds are ovoid to conoidal with pointed apices, whereas V. nigrum flower buds are globose with rounded apices. Petals are twisted before opening in V. rossicum. Flowers are produced in umbelliform cymes in leaf axils, with 5 to 20 flowers in V. rossicum and 4 to 10 flowers in V. nigrum. Peduncles of the inflorescences are pubescent and longer (1.5 to 4.5 cm) in V. rossicum than in V. nigrum (0.5 to 1.5 cm). Flowers are five-parted with diameters of 5 to 7 mm (V. rossicum) or 5 to 8 mm (V. nigrum). Calyx segments (1 to 1.5 mm) are more straplike in V. rossicum and closer to triangular in V. nigrum. Vincetoxicum rossicum and V. nigrum are usually easy to distinguish by corolla characteristics, especially color: pink, red-brown, or maroon in V. rossicum and dark purple to blackish in V. nigrum (Figure 2A and C). However, white-flowered individuals of V. rossicum are known from Ontario, Canada, and New York State, USA (S Darbyshire and L Milbrath, personal observation; Figure 2A). Vincetoxicum rossicum petals (3- to 5-mm long) are ovate-lanceolate to lanceolate, hairless, and only slightly fleshy. In contrast, V. nigrum petals (1.5- to 3-mm long) are ovate to deltoid, finely hairy on the inner surface, and fleshy. Both species have fleshy coronas that typically share the corolla color. The V. rossicum corona is more deeply lobed. The gynostegium (anthers fused with the stigmatic disk) is pale yellow to pale green. Fruits are smooth follicles, 4- to 7-cm long in V. rossicum and 4- to 8-cm long in V. nigrum. Vincetoxicum rossicum follicles are slender, and V. nigrum follicles may be slender or plump (Figure 2B and D). Two follicles may be formed per flower (more frequently in V. rossicum). The seeds of V. rossicum (4 to 6.5 by 2.4 to 3.1 mm) are light to dark brown, obovoid to oblong, and convex on one side. Vincetoxicum nigrum seeds are larger (6 to 8 by 3 to 4.7 mm), dark brown, ovoid to obovoid, and flattened on both sides. Seeds have membranous marginal wings (wider in V. rossicum, up to 0.25 mm) and a large coma (2 to 3 cm). Seedlings of V. rossicum and V. nigrum are not easily distinguishable. The cotyledons and first leaves are ovate to elliptic and may have slightly pointed apices. Cotyledons are not typically visible (Figure 3). Because V. nigrum has larger seeds, V. nigrum seedlings are initially larger than V. rossicum seedlings (Milbrath Reference Milbrath2008).

Figure 2. (A) Vincetoxicum rossicum typically has pink, red-brown, or maroon flowers but also occurs in a white-flowered mutant form. (B) Vincetoxicum rossicum follicles are slender. (C) Vincetoxicum nigrum flowers are dark purple to blackish. (D) Vincetoxicum nigrum follicles may be slender or plump. (Photo credits: (A) Stephen Darbyshire; (B) Larissa Smith; (C and D) Jeromy Biazzo.)

Figure 3. Cotyledons of Vincetoxicum nigrum (and V. rossicum) do not typically emerge from the seed. At the right, a seed has been manually dissected. (Photo credit: Scott Morris.)
Vincetoxicum hirundinaria, a widespread species in Eurasia and possible occasional garden escape in North America, is most easily distinguished from V. rossicum and V. nigrum by its white, yellow, or greenish-white flower petals (except in the case of white-flowered V. rossicum mutants). Petals may or may not be hairy (Cullen et al. Reference Cullen, Knees and Cubey2011). Otherwise, V. hirundinaria appears similar to V. rossicum and V. nigrum, according to descriptions from Eurasia (Bojnanský and Fargašová Reference Bojnanský and Fargašová2007; Cullen et al. Reference Cullen, Knees and Cubey2011). Disagreements arise as to what constitutes a species or subspecies within the highly variable and widespread V. hirundinaria complex (Markgraf Reference Markgraf1972; Pobedimova Reference Pobedimova1952, Reference Pobedimova1978). As noted earlier (see “Name and Taxonomy”), V. rossicum has been included under the name V. hirundinaria (Gleason and Cronquist Reference Gleason and Cronquist1991; Lauvanger and Borgen Reference Lauvanger and Borgen1998). These taxonomic disagreements, combined with the white-flowered mutant form of V. rossicum, have likely contributed to erroneous reports of invasive V. hirundinaria in North America.
In North America, V. rossicum is diploid (2n = 2x = 22) and V. nigrum is tetraploid (2n = 4x = 44) (Bon et al. Reference Bon, Guermache, Rodier-Goud, Bakry, Bourge, Dolgovskaya, Volkovitsh, Sforza, Darbyshire and Milbrath2013); hybridization between the two species in North America is therefore considered unlikely. Vincetoxicum rossicum is also reported to be diploid in its native range (Russia), and V. nigrum to be tetraploid in its native range (southern France; Bon et al. Reference Bon, Guermache, Rodier-Goud, Bakry, Bourge, Dolgovskaya, Volkovitsh, Sforza, Darbyshire and Milbrath2013). However, V. nigrum has been reported to be diploid in Spain (references in DiTommaso et al. Reference DiTommaso, Lawlor and Darbyshire2005b). Vincetoxicum hirundinaria is diploid in its native range (Guermache et al. Reference Guermache, Bon, Jones and Milbrath2010). Genome sizes (mean 2C values ± STD) are reported to be 0.71 ± 0.02 pg for V. rossicum and 1.44 ± 0.03 pg for V. nigrum and appear to be similar between the native and introduced ranges (Bon et al. Reference Bon, Guermache, Rodier-Goud, Bakry, Bourge, Dolgovskaya, Volkovitsh, Sforza, Darbyshire and Milbrath2013). For V. rossicum and V. nigrum, genetic diversity is much lower in North America than in Europe (Bon et al. Reference Bon, Jeanneau, Jones, Milbrath, Sforza and Dolgovskaya2010; Douglass Reference Douglass2008), with the majority of North American populations apparently derived from one major European genotype per species (Bon et al. Reference Bon, Jeanneau, Jones, Milbrath, Sforza and Dolgovskaya2010). The source population for V. rossicum has been identified in Ukraine (Sforza et al. Reference Sforza, Augé, Jeanneau, Poidatz, Reznik, Simonot, Volkovitch and Milbrath2013a).
Importance
Vincetoxicum rossicum and V. nigrum are listed as noxious or prohibited weeds across much of the northeastern United States and eastern Canada (Supplementary Table S1). In North America and other invaded regions, such as Norway, these weeds threaten both unmanaged and managed ecosystems by outcompeting resident and desirable species (Bjureke Reference Bjureke2007; DiTommaso et al. Reference DiTommaso, Lawlor and Darbyshire2005b). They can grow in dense near-monospecific stands (Figure 4), altering ecosystem structure and function (DiTommaso et al. Reference DiTommaso, Lawlor and Darbyshire2005b; Douglass et al. Reference Douglass, Weston and DiTommaso2009). In such stands, stem densities are often 100 to 200 stems m−2 (>10-cm tall) and >1,400 stems m−2 (<10 cm) under high light (Milbrath et al. Reference Milbrath, Davis and Biazzo2018; Sheeley Reference Sheeley1992; Smith et al. Reference Smith, DiTommaso, Lehmann and Greipsson2006). In southern Ontario, Livingstone et al. (Reference Livingstone, Isaac and Cadotte2020a) found that invasion by V. rossicum significantly decreased plant community diversity. Invasion also increased total productivity; however, productivity tended to increase with the richness of the remaining resident community. Changes to species diversity are associated with changes to functional diversity (Livingstone et al. Reference Livingstone, Isaac and Cadotte2020a; Sodhi et al. Reference Sodhi, Livingstone, Carboni and Cadotte2019). Vincetoxicum rossicum reduces plant community diversity by limiting resource availability to all residents and severely restricting resource availability to residents in particular niches (Sodhi et al. Reference Sodhi, Livingstone, Carboni and Cadotte2019). That is, V. rossicum acts as an ecological filter, causing the nonrandom exclusion of some resident species (Sodhi et al. Reference Sodhi, Livingstone, Carboni and Cadotte2019). In addition to competing with resident species, V. rossicum may drive plant diversity loss through ecosystem modification (such as changes to soil characteristics; Carboni et al. Reference Carboni, Livingstone, Isaac and Cadotte2021). Vincetoxicum invasion is particularly concerning in rare habitats such as alvar ecosystems (Kricsfalusy and Miller Reference Kricsfalusy and Miller2010; O’Brien et al. Reference O’Brien, Holzworth, Otis, Belding and Robert2010) and not necessarily prevented by high initial biodiversity in the invaded community (Kricsfalusy and Miller Reference Kricsfalusy and Miller2010). Dibble et al. (Reference Dibble, White and Lebow2007) included V. nigrum in the complex of introduced species that may enhance fire risks in forests and overgrown fields of the northeastern United States.

Figure 4. (A) Field infestation of Vincetoxicum rossicum in New York, USA. (B) Forest infestation of Vincetoxicum rossicum in New York, USA. (C) Emerging shoots of Vincetoxicum nigrum in New York, USA. (Photo credits: (A) Jeromy Biazzo; (B and C) Kristine Averill.)
In addition to reshaping resident plant communities, Vincetoxicum invasion effects changes at other trophic levels. For example, V. rossicum can have significant impacts on soil fungal and bacterial communities (Bongard et al. Reference Bongard, Butler and Fulthorpe2013a; Bugiel et al. Reference Bugiel, Livingstone, Isaac, Fulthorpe and Martin2018; Greipsson and DiTommaso Reference Greipsson and DiTommaso2006). Malloch et al. (Reference Malloch, Tatsumi, Seibold, Cadotte and MacIvor2020) reported interactive effects of V. rossicum invasion and urbanization on microarthropod communities in leaf litter. Ernst and Cappuccino (Reference Ernst and Cappuccino2005) reported reduced arthropod communities on invasive V. rossicum compared with native old-field plants and mixed grass communities, accounting for both stem- and ground-dwelling species of all feeding guilds. This finding is consistent with reports that V. rossicum and V. nigrum are seldom damaged by phytophagous arthropods in eastern North America (Carpenter and Cappuccino Reference Carpenter and Cappuccino2005; Milbrath Reference Milbrath2010). Livingstone (Reference Livingstone2018) suggested that pollinator richness and abundance are lower in ecosystems highly invaded by V. rossicum. Because invasive Vincetoxicum harbor very few arthropods, they are unlikely to be a reservoir for arthropod crop pests. Vincetoxicum species may be more problematic as reservoirs for fungal pathogens. For example, research in northern and southern Europe has demonstrated that V. nigrum and V. hirundinaria can serve as alternate hosts for Cronartium flaccidum (Alb. & Schwein.) G. Winter, a rust fungus that causes disease in pines (Pinus spp.) (Bon and Guermache Reference Bon and Guermache2012; Kaitera et al. Reference Kaitera, Hiltunen and Samils2012, Reference Kaitera, Hiltunen, Kauppila and Hantula2017). In an outdoor experiment in Switzerland, leaves of V. rossicum and V. nigrum became infected with the fungal pathogens Ascochyta sp. and Cercospora sp. (Ascomycota), respectively (Weed et al. Reference Weed, Gassmann and Casagrande2011a). There is some evidence that the fungal communities associated with V. rossicum in North America could have positive effects on V. rossicum and negative effects on native plants (Day et al. Reference Day, Dunfield and Antunes2016; Dickinson et al. Reference Dickinson, Bourchier, Fulthorpe, Shen, Jones and Smith2021).
Vincetoxicum infestations may harm monarch butterfly (Danaus plexippus L.) populations by acting as an oviposition sink or by competing with milkweeds. If D. plexippus females oviposit on these plants rather than on milkweeds (Asclepias spp.), the larvae do not survive (Haribal and Renwick Reference Haribal and Renwick1998). This mortality risk is reduced by the preference of D. plexippus females for Asclepias over Vincetoxicum hosts. Some experiments have shown a very strong preference for Asclepias, although this outcome may have resulted from using laboratory-reared insects (Alred et al. Reference Alred, Haan, Landis and Szűcs2022a; DiTommaso and Losey Reference DiTommaso and Losey2003; Mattila and Otis Reference Mattila and Otis2003). Other work has shown some to substantial oviposition on Vincetoxicum plants under field conditions (Alred et al. Reference Alred, Haan, Landis and Szűcs2022a; Casagrande and Dacey Reference Casagrande and Dacey2007; Milbrath Reference Milbrath2010). If oviposition on Vincetoxicum occurs at a significant rate, this rate likely depends on the ratio of Vincetoxicum to Asclepias plants at a landscape level. It has been suggested that Vincetoxicum species may do more damage to D. plexippus populations by outcompeting desirable Asclepias hosts than by serving as oviposition sinks (DiTommaso et al. Reference DiTommaso, Lawlor and Darbyshire2005b; DiTommaso and Losey Reference DiTommaso and Losey2003). As the two genera are related (in the same tribe, Asclepiadeae), and Vincetoxicum species tend to suppress native vegetation, the competitive effects of Vincetoxicum species on Asclepias species are likely to be substantial; however, these effects are difficult to measure in natural systems (MacIvor et al. Reference MacIvor, Roberto, Sodhi, Onuferko and Cadotte2017). Jackson and Amatangelo (Reference Jackson and Amatangelo2021) suggested that V. rossicum is a poor competitor against common milkweed (Asclepias syriaca L.) on an individual basis at low densities but gains a competitive advantage as its density increases (Allee effect). Because the effects of Vincetoxicum invasion on D. plexippus oviposition and Asclepias survival and performance appear to be context dependent, it is not entirely clear how the negative effects of Vincetoxicum species on D. plexippus compare with other threats to this species (Malcolm Reference Malcolm2018; Wilcox et al. Reference Wilcox, Flockhart, Newman and Norris2019).
Vincetoxicum rossicum and V. nigrum can be problematic in North American agricultural systems with low disturbance. For example, these species have been reported in no-till field crops and Christmas tree farms (DiTommaso et al. Reference DiTommaso, Lawlor and Darbyshire2005b; Douglass et al. Reference Douglass, Weston and DiTommaso2009; Lawlor Reference Lawlor2003). Horse pasture owners have also reported severe infestations, sometimes leading to abandonment of the pasture (DiTommaso et al. Reference DiTommaso, Lawlor and Darbyshire2005b; Douglass et al. Reference Douglass, Weston and DiTommaso2009). In addition to competing with desirable species, Vincetoxicum plants may overgrow and thereby reduce the effectiveness of electric fences (Tewksbury et al. Reference Tewksbury, Casagrande and Gassmann2002). These species are thought to be toxic to mammals (DiTommaso et al. Reference DiTommaso, Lawlor and Darbyshire2005b; Hess Reference Hess2014; Weston et al. Reference Weston, Barney and DiTommaso2005), although grazing by cattle and sheep has been reported (DiTommaso et al. Reference DiTommaso, Lawlor and Darbyshire2005b; Tewksbury et al. Reference Tewksbury, Casagrande and Gassmann2002). Because established Vincetoxicum populations are difficult to eradicate, severe infestations can decrease the land value of fields and pastures (AD, personal observation). Vincetoxicum infestations may also interfere with land use goals in areas managed for nonagricultural purposes. For example, vines of V. rossicum have been observed to weigh down small trees planted at restoration sites in Ontario (Christensen Reference Christensen1998). The number of breeding grassland birds decreased with increasing V. rossicum cover in a habitat managed for grassland birds in New York (DiTommaso et al. Reference DiTommaso, Lawlor and Darbyshire2005b). The foliage of Vincetoxicum species is typically unpalatable to herbivores native to North America, such as white-tailed deer (Odocoileus virginianus Zimmermann) (Lawlor Reference Lawlor2003). Most visitors to an urban park felt that a V. rossicum infestation detracted from the aesthetic value of the park (Livingstone et al. Reference Livingstone, Cadotte and Isaac2018). In its introduced North American range, V. rossicum colonizes a variety of habitats within the urban matrix, ranging from lawns and gardens to remnants of natural ecosystems (Cadotte et al. Reference Cadotte, Yasui, Livingstone and MacIvor2017; Potgieter and Cadotte Reference Potgieter and Cadotte2020). Control of this aggressive weed is a major issue in Toronto, Ontario (Potgieter and Cadotte Reference Potgieter and Cadotte2020; Potgieter et al. Reference Potgieter, Shrestha and Cadotte2021).
Vincetoxicum plants offer some benefits. For example, they have traditionally been used for medicinal purposes in Europe. Vincetoxicum hirundinaria has been administered as an emetic, diuretic, or anti-tumor agent (Duke Reference Duke2002, p. 325; Tanner and Wiegrebe Reference Tanner and Wiegrebe1993; Uphof 1968, p. 168). Extracts from Vincetoxicum species, including V. hirundinaria, exhibit antifeedant activity against the phytophagous larvae of the Colorado potato beetle (Leptinotarsa decemlineata Say) and Egyptian cotton leafworm (Spodoptera littoralis Boisduval) (Guzel et al. Reference Guzel, Pavela and Kokdil2015; Pavela Reference Pavela2010). Despite these possible uses, Vincetoxicum invasion should generally be considered deleterious.
Distribution
Vincetoxicum rossicum is native to Ukraine and southwestern Russia (DiTommaso et al. Reference DiTommaso, Lawlor and Darbyshire2005b; Figure 5A). This species has been anthropogenically introduced to other areas of Europe (see “Invasion History”; Markgraf Reference Markgraf1971). It is naturalized in Norway (Bjureke Reference Bjureke2007; Lauvanger and Borgen Reference Lauvanger and Borgen1998). Introductions to Belgium, Denmark, Finland, Germany, the Netherlands, and Sweden have also been reported (Gassmann 2022b; Global Biodiversity Information Facility 2022b). In North America, V. rossicum has been reported in the U.S. states of Connecticut, Indiana, Massachusetts, Michigan, Missouri, New Hampshire, New Jersey, New York, and Pennsylvania (Gassmann 2022b; USDA-NRCS 2021). It has been reported in the Canadian provinces of British Columbia (but not persisting), Ontario, and Quebec (Gassmann 2022b; USDA-NRCS 2021). There has been one report of V. rossicum in Nigeria (Global Biodiversity Information Facility 2022b).
Vincetoxicum nigrum is native to southwestern Europe, including Portugal, Spain, France, and Italy (DiTommaso et al. Reference DiTommaso, Lawlor and Darbyshire2005b; Figure 5B). Naturalized in the Netherlands (Markgraf Reference Markgraf1972), this species is also present in Belgium (Gassmann 2022a; Global Biodiversity Information Facility 2022a). There are occasional reports from Albania, the Bahamas, Bulgaria, Colombia, Croatia, the Czech Republic, Denmark, Germany, Iran, Mexico, Norway, Russia, Sweden, Tanzania, Turkey, and Ukraine (Global Biodiversity Information Facility 2022a; Petrova Reference Petrova2010). In North America, V. nigrum has been reported in the U.S. states of California, Connecticut, Illinois, Indiana, Kansas, Kentucky, Maine, Maryland, Massachusetts, Michigan, Minnesota, Missouri, Nebraska, New Hampshire, New Jersey, New York, Ohio, Pennsylvania, Rhode Island, Vermont, and Wisconsin (Gassmann 2022a; USDA-NRCS 2021). The single population reported in California has apparently died out (Milbrath and Biazzo Reference Milbrath and Biazzo2016), so the current distribution in the United States likely extends no farther west than Kansas and Nebraska. Vincetoxicum nigrum is present in the Canadian provinces of Ontario and Quebec (Gassmann 2022a; USDA-NRCS 2021).
Vincetoxicum hirundinaria is widespread in its native Eurasian range but not strongly invasive like V. rossicum and V. nigrum. Records of V. hirundinaria in North America are often misidentifications: many of these specimens are correctly identified as V. rossicum. The misidentifications reflect confusion over nomenclature or potentially the white-flowered mutant of V. rossicum (see “Name and Taxonomy”; “Description”). Although V. hirundinaria has been reported in New York and Michigan, USA, and Ontario, Canada (USDA-NRCS 2021), it is likely that most of these reports represent garden specimens with the exception of a 1904 collection involving a garden escape (Pringle Reference Pringle1973; Sheeley and Raynal Reference Sheeley and Raynal1996).
Habitat
According to the Köppen-Geiger classification, V. rossicum most frequently inhabits Dfa (cold, no dry season, hot summer) and Dfb (cold, no dry season, warm summer) climate types (Supplementary Figure S1A). Vincetoxicum nigrum most frequently inhabits BSk (arid, steppe, cold), Csa (temperate, dry summer, hot summer), Csb (temperate, dry summer, warm summer), Cfa (temperate, no dry season, hot summer), Cfb (temperate, no dry season, warm summer), Dfa, and Dfb climate types (Supplementary Figure S1B).
In their introduced North American ranges, V. rossicum and V. nigrum behave as generalists with respect to climate and habitat type. Kricsfalusy and Miller (Reference Kricsfalusy and Miller2010) characterized V. rossicum’s North American range as similar in temperature but wetter than its native range in Ukraine and Russia. In both ranges, V. rossicum tends to inhabit semi-open scrub or woodland habitats on calcareous soils (Kricsfalusy and Miller Reference Kricsfalusy and Miller2010). However, V. rossicum populations in North America occupy a wider variety of light environments and soil types (Kricsfalusy and Miller Reference Kricsfalusy and Miller2010). Vincetoxicum nigrum may inhabit cooler and wetter habitats in North America than in southwestern Europe (DiTommaso et al. Reference DiTommaso, Lawlor and Darbyshire2005b). In both species, cold temperatures are tolerated well but typically associated with phenological delays (see “Growth and Development: Stress Tolerance”; “Growth and Development: Phenology”). Vincetoxicum species tolerate a wide range of moisture levels, but permanently waterlogged soils are not suitable (see “Growth and Development: Stress Tolerance”). These species primarily inhabit upland habitats, including exposed habitats with highly variable water availability (DiTommaso et al. Reference DiTommaso, Lawlor and Darbyshire2005b). Based on a survey of V. rossicum populations in Ontario, Dickinson et al. (Reference Dickinson, Bourchier, Fulthorpe, Shen, Jones and Smith2021) reported that aboveground biomass production was positively correlated with precipitation but not strongly associated with habitat type or abiotic soil variables.
Vincetoxicum rossicum is often found on calcareous soils, including shallow soils over limestone bedrock as well as deeper soils (DiTommaso et al. Reference DiTommaso, Lawlor and Darbyshire2005b; Kricsfalusy and Miller Reference Kricsfalusy and Miller2010; Weston et al. Reference Weston, Barney and DiTommaso2005). In New York, Douglass (Reference Douglass2008) reported that soils colonized by V. rossicum (pH 5.2 to 7.6) had higher levels of calcium (Ca) and aluminum (Al) than soils colonized by V. nigrum (pH 5.2 to 7.2). Soils colonized by V. rossicum had a slightly higher average pH compared with V. nigrum (6.8 relative to 6.0). In a study combining soil measurements with data retrieved from the Soil Survey Geographic Database, Magidow et al. (Reference Magidow, DiTommaso, Westbrook, Kwok, Ketterings and Milbrath2022) found that V. rossicum colonized soils with higher mean pH than soils colonized by V. nigrum in the northeastern United States and southeastern Canada. However, soil characteristics (pH, fertility, texture, and taxonomy) varied widely across the ranges of both species (Magidow et al. Reference Magidow, DiTommaso, Westbrook, Kwok, Ketterings and Milbrath2022). Common garden and growth chamber experiments have provided only weak support for the hypothesis that V. rossicum and V. nigrum perform best on different soils (Magidow et al. Reference Magidow, DiTommaso, Ketterings, Mohler and Milbrath2013). Soil factors do not appear to limit range expansion in V. rossicum (Sanderson et al. Reference Sanderson, Day and Antunes2015).
At smaller spatial scales, light and disturbance are important influences on species distributions. Vincetoxicum rossicum and V. nigrum both inhabit high-light environments, including fields, habitat edges, and waste areas (DiTommaso et al. Reference DiTommaso, Lawlor and Darbyshire2005b). Vincetoxicum rossicum is also frequently found in forest understories, whereas V. nigrum is less common in understory habitats (Alred et al. Reference Alred, Hufbauer and Szűcs2022b; Averill et al. Reference Averill, DiTommaso, Mohler and Milbrath2011; Milbrath et al. Reference Milbrath, Davis and Biazzo2017), even though both species have the photosynthetic capacity to tolerate low light (Averill et al. Reference Averill, DiTommaso, Whitlow and Milbrath2016). Vincetoxicum nigrum often occupies shaded environments in its native European range (Averill et al. Reference Averill, DiTommaso, Mohler and Milbrath2011). In both species, light limitation can reduce establishment, growth, and reproductive potential (see “Growth and Development: Ecophysiology”). Vincetoxicum species are not problematic in highly disturbed habitats like tilled crop fields (DiTommaso et al. Reference DiTommaso, Lawlor and Darbyshire2005b). Vincetoxicum populations cannot survive under frequent, extreme disturbance because these perennials may remain vegetative for several years before flowering (see “Life-Form and Life History”). Averill et al. (Reference Averill, DiTommaso, Mohler and Milbrath2010) tested V. rossicum establishment and growth in two old fields under strong disturbance treatments (herbicide or herbicide plus tillage) or weak disturbance treatments (mowing or no disturbance). At one site, establishment was increased under weak disturbance relative to strong disturbance. However, total biomass at both sites was increased under strong disturbance relative to weak disturbance. These data suggest a possible trade-off between initial establishment and subsequent growth, in addition to illustrating the effects of small-scale habitat characteristics on Vincetoxicum competitiveness within the introduced range.
Invasion History
Vincetoxicum nigrum, V. rossicum, and V. hirundinaria are all native to Europe, with the native distribution of V. hirundinaria also including parts of Asia and Africa (see “Distribution”; Royal Botanic Gardens, Kew 2021). In addition, V. nigrum and V. rossicum have invaded areas of Europe to which they are not native (see “Distribution”). For example, a population of V. nigrum (native to southwestern Europe) was found to be established in Bulgaria in 2009 (Petrova Reference Petrova2010). It is naturalized in the Netherlands and is considered a garden escape in Belgium (Global Biodiversity Information Facility 2022a; Markgraf Reference Markgraf1972; Ronse Reference Ronse2013). Markgraf (Reference Markgraf1971) noted that V. rossicum has been grown in gardens in Central and Western Europe since at least the 1800s, with some escapes reported. Vincetoxicum rossicum was anthropogenically introduced to Norway before 1865, although misidentified as V. hirundinaria, and is now considered invasive there (Bjureke Reference Bjureke2007; Lauvanger and Borgen Reference Lauvanger and Borgen1998).
The early history of Vincetoxicum in North America is obscured by inconsistent nomenclature and the fact that escapes did not immediately lead to explosive population growth. Vincetoxicum nigrum appears to have been introduced first, with most early herbarium specimens (beginning in Massachusetts, USA, in 1854) collected in or near gardens (Sheeley and Raynal Reference Sheeley and Raynal1996). The fifth edition of Gray’s Manual of Botany (Gray Reference Gray1868, p. 399) described V. nigrum as “a weed escaping from gardens” around Cambridge, MA. It may have been brought into Ontario as early as 1861 from the Botanic Garden at Cambridge, MA (Pringle Reference Pringle1973). Early herbarium records and descriptions of V. rossicum (mistakenly labeled Cynanchum medium R. Br. in early years) (Sheeley and Raynal Reference Sheeley and Raynal1996) suggest a similar invasion pathway. These records include the Canadian provinces of British Columbia in 1885 (which did not persist) and Ontario in 1889, and New York, USA, in 1897 (Moore Reference Moore1959; Sheeley and Raynal Reference Sheeley and Raynal1996). Reports of V. rossicum in Canada and the northeastern United States remained sporadic until the second half of the 20th century (Sheeley and Raynal Reference Sheeley and Raynal1996). The third species, V. hirundinaria, has generated fewer records in North America. The seventh edition of Gray’s Manual of Botany (Gray Reference Gray, Robinson and Fernald1908, p. 667) reported that this species [as Cynanchum vincetoxicum (L.) Pers.] had escaped from cultivation in southern Ontario, although it apparently has not persisted (Pringle Reference Pringle1973). The earliest herbarium specimen found by Sheeley and Raynal (Reference Sheeley and Raynal1996) was collected in New York in 1916.
Following their initial introductions, V. rossicum and V. nigrum did not become major problems in North America for many decades. This long lag phase has been followed by a period of rapid expansion. Kricsfalusy and Miller (Reference Kricsfalusy and Miller2008) formalized these observations for V. rossicum in the Toronto, Ontario, area. They found that the lag phase lasted nearly a century and that V. rossicum invasion followed an exponential trajectory from 2000 to 2005. More qualitative observations of larger-scale trends indicate that V. rossicum and V. nigrum were well established in some areas (but not particularly common) by the mid-20th century (Sheeley and Raynal Reference Sheeley and Raynal1996). These species became increasingly prevalent in subsequent decades, especially at the end of the 20th century and in the 21st century (DiTommaso et al. Reference DiTommaso, Lawlor and Darbyshire2005b; Douglass et al. Reference Douglass, Weston and DiTommaso2009). Several factors may have contributed to increasing range and population expansion rates. Positive associations between population size and expansion rate (e.g., larger populations produce more seeds) should occur when resources are available and environmental conditions are suitable. During the lag phase, Vincetoxicum species may have increased their ability to compete for resources and thrive under North American environmental conditions. This process may have involved evolutionary changes to the genotypes introduced from Europe (Douglass et al. Reference Douglass, Weston and DiTommaso2009). Adaptive evolution could help explain the wide climatic ranges occupied by Vincetoxicum species in North America (see “Habitat”). Because V. rossicum and V. nigrum were already well established in North America by the time that their rapid expansion began attracting major concern, research and management efforts have focused on limiting environmental and economic injury rather than pursuing eradication, except in Minnesota, USA (Minnesota Department of Agriculture 2021).
Life-Form and Life History
Vincetoxicum species are herbaceous perennial vines that reproduce by seed (Figure 6). Based on North American studies, single-stemmed seedlings typically emerge in spring, although autumn germination can also occur from the seedbank (DiTommaso et al. Reference DiTommaso, Lawlor and Darbyshire2005b; Milbrath et al. Reference Milbrath, Davis and Biazzo2017). Survival rates for seedlings (and older plants) may be high, especially outside of heavily shaded forest habitats (Milbrath et al. Reference Milbrath, Davis and Biazzo2017). High survival rates may be facilitated by resource allocation strategies that initially emphasize resource acquisition and storage. Although it is possible for flowering and follicle production to occur in the first or second years under semi-natural conditions (Averill et al. Reference Averill, DiTommaso, Mohler and Milbrath2010; Magidow et al. Reference Magidow, DiTommaso, Ketterings, Mohler and Milbrath2013), flowering typically does not occur for several years (see “Reproduction: Population Dynamics”; Milbrath et al. Reference Milbrath, Davis and Biazzo2017). During the pre-flowering juvenile period, V. rossicum plants grow slowly and store resources belowground (Averill et al. Reference Averill, DiTommaso, Mohler and Milbrath2010; McKague and Cappuccino Reference McKague and Cappuccino2005; Milbrath et al. Reference Milbrath, Davis and Biazzo2017). Gradual resource accumulation may be particularly important in shaded or otherwise low-resource environments, but the long juvenile period is not unique to a particular habitat type (Averill et al. Reference Averill, DiTommaso, Mohler and Milbrath2010). In any competitive environment, V. rossicum may benefit from delaying reproduction until energy reserves are sufficient. Prolonged juvenile periods also occur in V. nigrum, although young V. nigrum plants are more likely to flower and produce follicles than young V. rossicum plants (Magidow et al. Reference Magidow, DiTommaso, Ketterings, Mohler and Milbrath2013; Milbrath Reference Milbrath2008; Milbrath et al. Reference Milbrath, Davis and Biazzo2017). The interspecific difference in time to flowering appears to be associated with increased root allocation in V. rossicum relative to V. nigrum (Milbrath et al. Reference Milbrath, Davis and Biazzo2017). Once a plant begins flowering, it may continue for many years. Individual plants are long-lived (life span unknown; Milbrath et al. Reference Milbrath, Davis and Biazzo2017), and populations may persist indefinitely (>70 yr; Sheeley and Raynal Reference Sheeley and Raynal1996).

Figure 6. (A) Seeds of Vincetoxicum nigrum (left) and Vincetoxicum rossicum (right). (B) Seedlings (with seed coats) and older vegetative juveniles of V. rossicum. (C) Vegetative juveniles of Vincetoxicum rossicum. (D) Individual Vincetoxicum rossicum plant with multiple stems. (E) Senesced Vincetoxicum rossicum stems and spring regrowth. (F) Follicle dehiscence in Vincetoxicum rossicum. (Photo credits: (A and D) Scott Morris; (B) Kristine Averill; (C and E) Jeromy Biazzo; (F) Larissa Smith.)
Each year, Vincetoxicum stems senesce in late summer or early autumn (DiTommaso et al. Reference DiTommaso, Lawlor and Darbyshire2005b). Belowground organs overwinter. In the next growing season, one to several new stems sprout from buds on the root crown (DiTommaso et al. Reference DiTommaso, Lawlor and Darbyshire2005b; Milbrath et al. Reference Milbrath, Davis and Biazzo2017). In productive environments, older plants produce more stems than young plants (Milbrath et al. Reference Milbrath, Davis and Biazzo2017). Averill et al. (Reference Averill, DiTommaso, Mohler and Milbrath2011) demonstrated this phenomenon in both V. rossicum and V. nigrum clumps (i.e., single genets or multiple genets in the case of polyembryony; see “Reproduction: Seed Production and Dispersal”). Stem numbers increased more quickly in old fields (−0.02 to 2.1 stems clump−1 yr−1 for V. rossicum and −0.01 to 4.6 stems clump−1 yr−1 for V. nigrum) than in forests (−0.01 to 0.8 stems clump−1 yr−1 for V. rossicum). Older plants may have up to 25 stems (Milbrath et al. Reference Milbrath, DiTommaso, Biazzo and Morris2016, Reference Milbrath, Davis and Biazzo2017). However, under high densities, individual plants may only possess a single stem (LRM, unpublished data). Increases in stem number can facilitate proportional increases in per-plant biomass or seed production (Averill et al. Reference Averill, DiTommaso, Mohler and Milbrath2011), or these trends can be decoupled (e.g., under disturbance; Milbrath et al. Reference Milbrath, DiTommaso, Biazzo and Morris2016). Depending on their length and the availability of supports, Vincetoxicum stems may be erect or scrambling, twining, or climbing (DiTommaso et al. Reference DiTommaso, Lawlor and Darbyshire2005b). In hedgerow and woodland-edge habitats, V. rossicum can reportedly climb to heights of 2 to 3 m (Cappuccino et al. Reference Cappuccino, Mackay and Eisner2002). The climbing habit aids seed dispersal and increases the competitive (i.e., shading) and physical effects of vines on support plants.
Invasive Vincetoxicum species are effective competitors and tolerant of interspecific competition. Their life cycles are relatively slow. They tolerate only moderate disturbance and stress. For these reasons, they are best classified as Competitors in the C-S-R (Competitor, Stress tolerator, Ruderal) framework (Averill et al. Reference Averill, DiTommaso, Mohler and Milbrath2010; Grime Reference Grime1977).
Dispersal and Establishment
Vincetoxicum rossicum and V. nigrum reproduce exclusively by producing seeds, which are primarily wind dispersed. Wind-dispersed seeds typically travel short to moderate distances. Cappuccino et al. (Reference Cappuccino, Mackay and Eisner2002) found that V. rossicum seeds traveled up to 18 m, with 50% of seeds traveling less than 2.5 m from the release point (release height: 1.5 m; windspeed: 11.2 km h−1). Seed weight was negatively associated with dispersal distance. Large seeds sometime achieved better germination and growth than small seeds; however, the relationships between seed weight and dispersibility or quality are weak enough that any trade-off between dispersibility and quality is probably unimportant at the local scale (Ladd and Cappuccino Reference Ladd and Cappuccino2005). Release height is a more important influence on dispersal distance. Seeds released from 0.75 m landed 4.4 ± 3.3 m (mean ± SD) from the release point in V. nigrum and 4.7 ± 3.5 m from the release point in V. rossicum, whereas seeds released from 2 m landed an average of 12.6 ± 9.6 m and 17.1 ± 12.1 m from the release point, respectively (DiTommaso et al. Reference DiTommaso, Stokes, Cordeau, Milbrath and Whitlow2018). The maximum dispersal distances (2-m release height) were 72.1 m for V. nigrum and 79.6 m for V. rossicum. This interspecific difference reflected the fact that V. nigrum seeds are heavier. Long-distance wind dispersal of V. nigrum seeds could require the combination of a high release point and a strong wind (DiTommaso et al. Reference DiTommaso, Stokes, Cordeau, Milbrath and Whitlow2018). Other seed-dispersal mechanisms are less common than wind dispersal. There are many anecdotal reports of animal dispersal, including dispersal by white-tailed deer (O. virginianus; DiTommaso et al. Reference DiTommaso, Stokes, Cordeau, Milbrath and Whitlow2018) and dispersal along hiking trails (Sandilands Reference Sandilands2013). Longer-distance transport is mostly anthropogenic. Vincetoxicum species have historically been transported deliberately as ornamentals and presumably continue to be transported inadvertently (see “Invasion Pathways”).
Within their bioclimatic envelopes (see “Habitat”), V. rossicum and V. nigrum are limited by competition from resident plant species. Greenhouse and growth chamber experiments have shown that competition against A. syriaca, Canada goldenrod (Solidago canadensis L.), and quackgrass [Elymus repens (L.) Gould] can substantially reduce Vincetoxicum performance (Blanchard et al. Reference Blanchard, Barney, Averill, Mohler and DiTommaso2010; Jackson and Amatangelo Reference Jackson and Amatangelo2021; Milbrath et al. Reference Milbrath, Biazzo, DiTommaso and Morris2019a; Sanderson and Antunes Reference Sanderson and Antunes2013). Research on V. nigrum has demonstrated that competitive responses are shaped by competitive environments over evolutionary time (Atwood and Meyerson Reference Atwood and Meyerson2011). Competitive environments also matter at smaller, more ecological spatiotemporal scales: in general, plant community invasibility depends on numerous determinants of resource supply and demand (Davis et al. Reference Davis, Grime and Thompson2000). However, some habitats invaded by V. rossicum and V. nigrum lack characteristics likely to promote resource surpluses, such as high disturbance levels (Averill et al. Reference Averill, DiTommaso, Mohler and Milbrath2010; DiTommaso et al. Reference DiTommaso, Lawlor and Darbyshire2005b).
Several factors may be helpful in explaining why V. rossicum and V. nigrum are so invasive. First, these species can survive and reproduce in low-resource environments (see “Life-Form and Life History”; “Growth and Development: Ecophysiology”). Second, positive density-dependent effects (e.g., plants twining together into a light-extinguishing mat) may help suppress allospecific competitors (see “Reproduction: Population Dynamics”). Third, their fitness is typically not limited by herbivores or pathogens in North America (enemy release; see “Management Options: Biological”). Vincetoxicum species contain phenanthroindolizidine alkaloids, notably (−)-antofine, in their seeds, leaves/stems, and roots (Capo and Saa Reference Capo and Saa1989; Gibson et al. Reference Gibson, Krasnoff, Biazzo and Milbrath2011, Reference Gibson, Vaughan and Milbrath2015; Mogg et al. Reference Mogg, Petit, Cappuccino, Durst, McKague, Foster, Yack, Arnason and Smith2008; Stærk et al. Reference Stærk, Christensen, Lemmich, Duus, Olsen and Jaroszewski2000). Vincetoxicum rossicum contains higher concentrations of (−)-antofine than V. nigrum; roots have higher concentrations than stems; and seeds, seedlings, and young plants of V. rossicum contain higher concentrations than adult plant roots (Gibson et al. Reference Gibson, Krasnoff, Biazzo and Milbrath2011, Reference Gibson, Vaughan and Milbrath2015). These compounds are highly cytotoxic, as demonstrated in experiments with human cancer cells (Stærk et al. Reference Stærk, Christensen, Lemmich, Duus, Olsen and Jaroszewski2000, Reference Stærk, Lykkeberg, Christensen, Budnik, Abe and Jaroszewski2002; Tanner and Wiegrebe Reference Tanner and Wiegrebe1993). Phenanthroindolizidine alkaloids, which appear to be rare in the Asclepiadoideae, could contribute to invasiveness by reducing palatability to herbivores in the introduced range (Liede-Schumann et al. Reference Liede-Schumann, Khanum, Mumtaz, Gherghel and Pahlevani2016). Mogg et al. (Reference Mogg, Petit, Cappuccino, Durst, McKague, Foster, Yack, Arnason and Smith2008) and Gibson et al. (Reference Gibson, Krasnoff, Biazzo and Milbrath2011) found that (−)-antofine from V. rossicum extracts exhibited antibacterial and especially antifungal activity. Mogg et al. (Reference Mogg, Petit, Cappuccino, Durst, McKague, Foster, Yack, Arnason and Smith2008) also reported insect antifeedant activity, which was not attributable to (−)-antofine. Similarly, other Vincetoxicum species contain alkaloids that deter feeding by nonspecialist insects (Kathuria and Kaushik Reference Kathuria and Kaushik2005; Verma et al. Reference Verma, Ramakrishnan, Mulchandani and Chadha1986).
Allelopathy has been proposed as a fourth explanation for successful Vincetoxicum invasion of occupied niches. Cappuccino (Reference Cappuccino2004) indicated that V. rossicum extracts inhibited radish (Raphanus sativus L.) seed germination in addition to exhibiting antifungal activity. Such data suggest that tissue leachates or root exudates from Vincetoxicum invaders could affect native plants both directly (inhibition) and indirectly (altered rhizosphere communities). The cytotoxic alkaloids may act as “novel weapons” (sensu Callaway and Ridenour Reference Callaway and Ridenour2004), promoting invasiveness. Similarly, Douglass et al. (Reference Douglass, Weston and Wolfe2011) found that V. rossicum and V. nigrum root exudates could reduce the germination and growth of some indicator species in laboratory tests (in one case, root exudates increased growth). Vincetoxicum tissue leachates had inhibitory, neutral, or stimulatory effects, depending on the indicator species (Douglass et al. Reference Douglass, Weston and Wolfe2011). Gibson et al. (Reference Gibson, Krasnoff, Biazzo and Milbrath2011) found that native Apocynaceae were more sensitive to (−)-antofine than V. rossicum and V. nigrum. However, Gibson et al. (Reference Gibson, Vaughan and Milbrath2015) reported that (−)-antofine degrades rapidly under ambient conditions and its allelopathic activity is greatly reduced in nonsterile soil. In addition, the concentrations of (−)-antofine in V. rossicum and V. nigrum root exudates were too low for significant biological activity, although the authors did not rule out (−)-antofine accumulation in the rhizosphere (Gibson et al. Reference Gibson, Vaughan and Milbrath2015). While high concentrations of (−)-antofine in seeds and seedlings might contribute to establishment, it is not clear that allelopathy plays a role in Vincetoxicum invasions.
Invasion Risk
Foster et al. (Reference Foster, Kharouba and Smith2022) developed MaxEnt and spatial dispersal models for V. rossicum across its invasion history in North America (10-yr intervals, 1890 to 2020). This analysis suggested that the North American distribution of V. rossicum was largely a function of dispersal in the early stages of invasion. Environmental constraints played a more important role in later stages. Foster et al. (Reference Foster, Kharouba and Smith2022) concluded that V. rossicum may have reached environmental equilibrium and that its geographic distribution may be stabilizing, but noted caveats related to the period of reference, spatial scale, and potential for dispersal limitation or local adaptation.
To our knowledge, potential distributions of V. rossicum and V. nigrum have not previously been modeled at a global scale. We developed CLIMEX models to identify regions of the world that are potentially suitable for V. rossicum and V. nigrum persistence under current climate. CLIMEX software (v. 4, Hearne Software, Melbourne, Australia) determines potential species distributions based on model parameters that describe how the species respond to climate. Model parameters reflect experimental data (when available) and species occurrence records. Our models were based on responses to temperature and precipitation; we did not explicitly account for factors such as land use or biotic constraints. Modeling methods are described in Supplementary Appendix S1, and model parameters are given in Supplementary Tables S2 and S3.
The CLIMEX models showed that the potential distributions of V. rossicum and V. nigrum are much larger than their current distributions (Figure 7). For example, the projected distribution of V. rossicum includes the northern half of the continental United States, and the projected distribution of V. nigrum includes most of the continental United States. Most areas of Europe are potentially suitable for both species. These results suggest that substantial range expansions are possible for both species.

Figure 7. Climatic suitability for (A) Vincetoxicum rossicum and (B) Vincetoxicum nigrum. CLIMEX models were based on temperature and precipitation (see Supplementary Appendix S1). According to these models, unshaded areas are not suitable for Vincetoxicum population growth, yellow areas are somewhat suitable, and red areas are highly suitable. Current species distributions are also shown with blue dots (Global Biodiversity Information Facility 2022a, 2022b).
Any discrepancies between our conclusions and those of Foster et al. (Reference Foster, Kharouba and Smith2022) could reflect the different goals, methods, and spatiotemporal scales of the two analyses. As different approaches provide different insights, it is useful to construct diverse models to develop a consensus picture of invasion risk. Further modeling efforts should also seek to develop high-resolution maps that account for additional influences on distribution (e.g., biotic constraints) and future climate change.
Invasion Pathways
Many reported introductions of V. rossicum, V. nigrum, and V. hirundinaria to new continents and regions have been deliberate (see “Invasion History”). These species were primarily introduced to North America as ornamental plants that escaped from gardens repeatedly and in multiple locations (Sheeley and Raynal Reference Sheeley and Raynal1996). Cultivation is now uncommon and sometimes prohibited in North America (Supplementary Table S1). Garden escapes have occurred to a lesser extent in Europe (Lauvanger and Borgen Reference Lauvanger and Borgen1998; Ronse Reference Ronse2013), where Vincetoxicum species are less likely to have large advantages over competitors. Long-distance Vincetoxicum transport may also occur inadvertently. Contamination in the nursery trade is one possibility (DiTommaso et al. Reference DiTommaso, Lawlor and Darbyshire2005b). This mode of transport could easily bridge continents, although frequent intercontinental transport seems unlikely to have occurred given the reduced genetic diversity of V. rossicum and V. nigrum in North America relative to Europe (Bon et al. Reference Bon, Jeanneau, Jones, Milbrath, Sforza and Dolgovskaya2010). Other plausible vectors for seed transport include vehicles, machinery, and hay (Cadotte et al. Reference Cadotte, Yasui, Livingstone and MacIvor2017; Plant Conservation Alliance’s Alien Plant Working Group 2006). Although non-anthropogenic mechanisms like wind dispersal usually operate over short or medium distances (see “Dispersal and Establishment”), they could allow infrequent long-distance transport. Seeds of horseweed [Conyza canadensis (L.) Cronquist] may travel very long distances by entering the planetary boundary layer (Shields et al. Reference Shields, Dauer, VanGessel and Neumann2006). Seeds of V. rossicum and V. nigrum might likewise achieve occasional long-distance transport when release height and wind speed are high (DiTommaso et al. Reference DiTommaso, Stokes, Cordeau, Milbrath and Whitlow2018). Given that V. rossicum is considered rare and even endangered in some parts of its native range, it is unlikely that the Russia–Ukraine conflict will affect its invasiveness in the region.
Growth and Development
Morphology
The competitiveness of Vincetoxicum species is influenced by resource allocation patterns. Both V. rossicum and V. nigrum rely on root reserves for overwintering and recovery from damage (DiTommaso et al. Reference DiTommaso, Lawlor and Darbyshire2005b). Root allocation allows gradual resource accumulation and thereby supports competitiveness and fitness (see “Life-Form and Life History”). Root-to-shoot ratios are typically higher in V. rossicum than in V. nigrum (Milbrath et al. Reference Milbrath, DiTommaso, Biazzo and Morris2016). For V. rossicum, Averill et al. (Reference Averill, DiTommaso, Mohler and Milbrath2010) reported root-to-shoot ratios of 2.6 and 3.5 (two sites, two seasons after sowing). McKague and Cappuccino (Reference McKague and Cappuccino2005) reported root-to-shoot ratios of nearly 1 in mature V. rossicum and greater than 3 in seedlings.
Root-to-shoot ratios are sensitive to abiotic and biotic environments. Milbrath (Reference Milbrath2008) reported high root-to-shoot ratios in V. rossicum (1.9 in mature plants and greater than 3 in seedlings), relative to consistently low ratios in V. nigrum (less than 1) under high light (488 to 916 μmol s−1 m−2). Under low light (12 to 25 μmol s−1 m−2), there was no interspecific difference. DiTommaso et al. (Reference DiTommaso, Averill, Qin, Ho, Westbrook and Mohler2021) reported a root-to-shoot ratio of 1.4 in V. rossicum and 0.5 for V. nigrum under sufficient soil moisture. Soil moisture deficit increased these root-to-shoot ratios to 3.0 in V. rossicum and 1.2 in V. nigrum. Cappuccino (Reference Cappuccino2004) reported that root-to-shoot ratios were marginally greater in smaller patches of V. rossicum relative to larger patches (plants in larger patches produced more vegetative biomass). Root-to-shoot ratios in V. rossicum may be higher under interspecific competition than intraspecific competition (Blanchard et al. Reference Blanchard, Barney, Averill, Mohler and DiTommaso2010). Sanderson and Antunes (Reference Sanderson and Antunes2013) found that competition for nutrients against S. canadensis increased the root-to-shoot ratio of V. rossicum by 40% (1.72 to 2.41) relative to V. rossicum grown alone. Conversely, competition against V. rossicum did not significantly increase the root-to-shoot ratio of S. canadensis. Taken together, these data indicate that plasticity in resource allocation represents an important component of V. rossicum’s response to competition.
Stress Tolerance
Vincetoxicum rossicum and V. nigrum overwinter as seeds and by perennating buds on the root crown (DiTommaso et al. Reference DiTommaso, Lawlor and Darbyshire2005b). Overwintering belowground allows V. rossicum to survive very cold temperatures, including minimum temperatures of −35 C in Ontario (Dickinson and Royer Reference Dickinson and Royer2014, p. 40; Dickinson et al. Reference Dickinson, Bourchier, Fulthorpe, Shen, Jones and Smith2021). Crown buds sprout at the beginning of the growing season or after aboveground damage, such as from spring frosts or stem removal (DiTommaso et al. Reference DiTommaso, Lawlor and Darbyshire2005b; McKague and Cappuccino Reference McKague and Cappuccino2005; LRM, personal observation). Regrowth from axillary buds also allows for tolerance to damage, especially under high light (see “Management Options: Cultural”).
Vincetoxicum species can tolerate seasonal (not prolonged) flooding, but do not occupy wetlands (DiTommaso et al. Reference DiTommaso, Lawlor and Darbyshire2005b; Lawlor Reference Lawlor2002). Vincetoxicum rossicum establishment may be reduced in poorly drained soil (Averill et al. Reference Averill, DiTommaso, Mohler and Milbrath2010). In a greenhouse experiment, V. rossicum height and V. nigrum biomass were reduced by recurring drought (Joline and DiTommaso Reference Joline and DiTommaso2016). Another greenhouse experiment showed that drought reduced growth (height, number of nodes, and biomass production) and reproduction, especially in V. rossicum (DiTommaso et al. Reference DiTommaso, Averill, Qin, Ho, Westbrook and Mohler2021). Vincetoxicum nigrum maintained higher rates of shoot growth and reproduction under drought (DiTommaso et al. Reference DiTommaso, Averill, Qin, Ho, Westbrook and Mohler2021). In a field study of V. hirundinaria in Sweden, supplemental watering (324 mm in addition to 130-mm rainfall) increased follicle (but not flower) production in the treatment year and the following year (Ågren et al. Reference Ågren, Ehrlén and Solbreck2008). Like water availability, soil pH does not narrowly constrain Vincetoxicum distributions (see “Habitat”) but can affect performance. For example, some authors have reported reduced biomass under low pH (Magidow et al. Reference Magidow, DiTommaso, Ketterings, Mohler and Milbrath2013; Sanderson et al. Reference Sanderson, Day and Antunes2015). The presence of Vincetoxicum species in urban environments and along roadsides (Cadotte et al. Reference Cadotte, Yasui, Livingstone and MacIvor2017; DiTommaso et al. Reference DiTommaso, Lawlor and Darbyshire2005b; Potgieter and Cadotte Reference Potgieter and Cadotte2020) might be facilitated by a tolerance to salinity or chemical toxins, but this hypothesis requires experimental testing.
Ecophysiology
Although both V. rossicum and V. nigrum are physiologically capable of tolerating low-light conditions, V. nigrum mostly occupies high-light sites (Averill et al. Reference Averill, DiTommaso, Mohler and Milbrath2011, Reference Averill, DiTommaso, Whitlow and Milbrath2016). Vincetoxicum rossicum is found in a variety of environments, but generally achieves higher survival, growth, and reproduction under high light (see “Habitat”; “Reproduction: Seed Production and Dispersal”; Hotchkiss et al. Reference Hotchkiss, DiTommaso, Brainard and Mohler2008; Milbrath Reference Milbrath2008; Milbrath et al. Reference Milbrath, Davis and Biazzo2017). Ambient light levels help determine specific leaf area in V. rossicum (Rochette Reference Rochette2019; Yasui Reference Yasui2016). Smith et al. (Reference Smith, DiTommaso, Lehmann and Greipsson2006) reported that V. rossicum plants grown under low light exhibited a classic shade phenotype (taller, thicker stems and larger, thinner leaves) that resulted in an increased tendency to climb over nearby plants. More generally, tall stems and high specific leaf areas may help V. rossicum invade and dominate existing plant communities (Livingstone et al. Reference Livingstone, Isaac and Cadotte2020a).
Vincetoxicum rossicum adopts a “sit-and-wait” strategy in low-light sites under forest canopies (Averill et al. Reference Averill, DiTommaso, Mohler and Milbrath2011, Reference Averill, DiTommaso, Whitlow and Milbrath2016; Hotchkiss et al. Reference Hotchkiss, DiTommaso, Brainard and Mohler2008). These plants can photosynthesize efficiently and store resources belowground until canopy disturbance increases light penetration, enabling substantial aboveground growth and seed production. This strategy can be understood as a special application of the long juvenile phase characteristic of Vincetoxicum species and especially V. rossicum (see “Life-Form and Life History”). The waiting strategy of V. rossicum has been observed at both plant and population scales: a forest population can persist at low density until disturbance releases additional resources (Averill et al. Reference Averill, DiTommaso, Mohler and Milbrath2011; Sheeley Reference Sheeley1992).
Phenology
In eastern Canada and the northeastern United States, seed germination and seedling emergence of V. rossicum and V. nigrum usually occur in a spring flush (often beginning in May) with germination and emergence continuing at decreasing rates through summer and autumn (often ending in October) (Averill et al. Reference Averill, DiTommaso, Mohler and Milbrath2010; DiTommaso et al. Reference DiTommaso, Lawlor and Darbyshire2005b; Milbrath et al. Reference Milbrath, Davis and Biazzo2017). Although not all newly dispersed seeds exhibit dormancy (DiTommaso et al. Reference DiTommaso, Brainard and Webster2005a, 2005b), germination and emergence are rare in the autumn relative to the spring (Milbrath et al. Reference Milbrath, Davis and Biazzo2017). Most autumn-germinating seeds germinate from the seedbank (i.e., they are more than 1 yr old; Milbrath et al. Reference Milbrath, Davis and Biazzo2017). After the establishment year, new shoots often emerge in late April or early May (DiTommaso et al. Reference DiTommaso, Lawlor and Darbyshire2005b). In northern New York, V. rossicum plants attained their maximum heights in late June or early July (Smith et al. Reference Smith, DiTommaso, Lehmann and Greipsson2006); Averill et al. (Reference Averill, DiTommaso, Mohler and Milbrath2011) indicated that maximum stem lengths for both species occurred by August across New York sites.
Flowering and follicle development overlap (DiTommaso et al. Reference DiTommaso, Lawlor and Darbyshire2005b). A generic phenology for V. rossicum and V. nigrum in the northeastern United States would predict most flowering and follicle development between June and early autumn, with seed dispersal beginning in August. The exact timing depends on species, climate, and habitat characteristics. In central and northern New York, flowering of V. rossicum can begin in mid- to late May and peak from late May to late June (Lawlor Reference Lawlor2000; Sheeley Reference Sheeley1992; Smith et al. Reference Smith, DiTommaso, Lehmann and Greipsson2006). Flowering may peak approximately 5 wk after the emergence of shoots (Sheeley Reference Sheeley1992). Follicle production may level off between mid-June and early August, remaining high until early September (Smith et al. Reference Smith, DiTommaso, Lehmann and Greipsson2006). In New York, seed dispersal in open habitats often begins in late July or early August (Lawlor Reference Lawlor2003). In Ontario, V. rossicum flowers from late May or June to August, although plants that resprout after being damaged may flower until the first frost (Christensen Reference Christensen1998; St. Denis and Cappuccino Reference St. Denis and Cappuccino2004). Christensen (Reference Christensen1998) reported that follicle development in southern Ontario occurs between mid-June and mid-August, seed dispersal begins by late August, and stems are dead by the end of September (Christensen Reference Christensen1998). Farther north near Ottawa, Ontario, follicle dehiscence may not begin until September, with seed release continuing through November (Cappuccino et al. Reference Cappuccino, Mackay and Eisner2002). Seed maturation, follicle dehiscence, and senescence are sometimes delayed (2 wk up to 1 mo) in shaded populations of V. rossicum relative to populations under high light (Christensen Reference Christensen1998; Lawlor Reference Lawlor2000; Livingstone et al. Reference Livingstone, Smith, Bourchier, Ryan, Roberto and Cadotte2020b; Milbrath et al. Reference Milbrath, Davis and Biazzo2017).
Within a growing season, the flowering period may be longer in V. nigrum than V. rossicum. Unlike V. rossicum, which may stop flowering as soon as early August in New York, V. nigrum can continue flowering until the first hard frost (Milbrath et al. Reference Milbrath, DiTommaso, Biazzo and Morris2016). Otherwise, phenology is generally similar between the two species. In southeastern New York, flowering of V. nigrum typically begins in mid- to late May, peaks in mid- to late June, and tapers off in July (Lumer and Yost Reference Lumer and Yost1995). Within this region, flowering tends to begin earlier at more southern sites and continue later (into August) at shadier sites (Lumer and Yost Reference Lumer and Yost1995). In the northeastern United States (New York and New England states), V. nigrum seed broadcast begins in late July or early to mid-August but may continue as late as October (Lumer and Yost Reference Lumer and Yost1995; URI CELS Outreach Center n.d.).
Mycorrhiza and Bacterial Symbionts
Vincetoxicum species associate with arbuscular mycorrhizal fungi (AMF), which may improve performance in their introduced range. For example, Smith et al. (Reference Smith, DiTommaso, Lehmann and Greipsson2008) measured high rates of AMF root colonization in V. rossicum field-collected in New York, relative to co-occurring species such as S. canadensis and A. syriaca. Greenhouse experiments showed that soil containing AMF from the field site facilitated V. rossicum survival (relative to sterilized soil) and growth (relative to sterilized soil, sterilized soil reamended with an AMF-free microbial wash, or soil inoculated with an AMF isolate from Alabama, USA). Greipsson and DiTommaso (Reference Greipsson and DiTommaso2006) also observed high rates of root colonization in V. rossicum. Soil invaded by V. rossicum contained four times as many AMF spores as nearby non-invaded soil and had a higher mycorrhizal infectivity potential. Vincetoxicum rossicum has been described as a fungal generalist, associating with AMF (e.g., Rhizophagus spp. and Funneliformis spp.) with which nearby native plants may not associate (Bongard et al. Reference Bongard, Navaranjan, Yan and Fulthorpe2013b). Although V. rossicum may be capable of associating with diverse AMF, observed AMF communities are not always diverse. Dickinson et al. (Reference Dickinson, Bourchier, Fulthorpe, Shen, Jones and Smith2021) observed a low diversity of AMF colonizing V. rossicum roots across 54 sites in southern Ontario: only three species appeared at more than 5% of sites, two of which appeared at all sites. The authors suggested that these AMF species might resist antagonism by other fungal partners and might also resist allelochemicals such as (−)-antofine. Bongard et al. (Reference Bongard, Butler and Fulthorpe2013a) reported that native plants living in close association with V. rossicum were colonized at higher densities and by different AMF communities than native plants living without V. rossicum. Changes to soil AMF communities due to V. rossicum likely develop over multiple growing seasons (Day et al. Reference Day, Antunes and Dunfield2015a). Although V. rossicum often exerts a powerful influence on AMF communities, this influence is not always dominant. Bongard and Fulthorpe (Reference Bongard and Fulthorpe2013) found that garlic mustard [Alliaria petiolata (M. Bieb.) Cavara & Grande] could decrease the density of AMF on V. rossicum, but V. rossicum had less effect on A. petiolata.
Vincetoxicum–soil feedbacks are not exclusively mediated by AMF. Dickinson et al. (Reference Dickinson, Bourchier, Fulthorpe, Shen, Jones and Smith2021) identified 30 endophytes, including 12 known pathogens, whose abundances were positively correlated with aboveground biomass production in V. rossicum. In addition, aboveground biomass was positively correlated with the percentage of the fungal community represented by these 12 known pathogens. The authors suggested that fungal pathogens might promote V. rossicum invasion by negatively affecting competing plants. The authors also noted the presence of dark septate endophytes colonizing V. rossicum; like AMF, dark septate endophytes may provide their hosts with water and nutrients. The results of this field study (Dickinson et al. Reference Dickinson, Bourchier, Fulthorpe, Shen, Jones and Smith2021) were consistent with an earlier report (Day et al. Reference Day, Dunfield and Antunes2016) that V. rossicum can host fungi that increase its total biomass but do not facilitate native species and might even contribute to inhibitory effects on S. canadensis. In S. canadensis, unlike other native species, rhizosphere biota from V. rossicum–invaded soil appear to have a stronger negative effect on biomass than rhizosphere biota from uninvaded soils (Dukes et al. Reference Dukes, Koyama, Dunfield and Antunes2019). In its North American range, V. rossicum does not yet appear to be strongly inhibited by pathogenic fungi (see “Dispersal and Establishment”; “Management Options: Biological”). Despite the presence of pathogenic fungi, soil biota from invaded soils may have a net positive effect on V. rossicum with no evidence of pathogen accumulation over long time periods (100 yr; Day et al. Reference Day, Dunfield and Antunes2015b).
Fewer research projects have focused on bacteria. Thompson et al. (Reference Thompson, Bell and Kao-Kniffin2018) reported that invasion by V. rossicum did not explain much variation in bacterial community composition (unlike fungal community composition), soil respiration, or available soil nitrogen (N). In contrast, Bugiel et al. (Reference Bugiel, Livingstone, Isaac, Fulthorpe and Martin2018) reported that invasion by V. rossicum explained a significant portion of variation in bacterial community composition. Plant–soil feedbacks might also be mediated by the availability of nitrogen and other nutrients, but understanding these feedback mechanisms will require further research on nutrient dynamics in communities invaded by Vincetoxicum species (Liu Reference Liu2020).
Reproduction
Floral Biology
Vincetoxicum rossicum, V. nigrum, and V. hirundinaria (in Finland) can produce seeds by insect-mediated cross-pollination or self-pollination (Leimu Reference Leimu2004; Lumer and Yost Reference Lumer and Yost1995; St. Denis and Cappuccino Reference St. Denis and Cappuccino2004). These species, like other members of the subfamily Asclepiadoideae, have complex floral structures that facilitate insect pollination. Pollen is dispersed in a structure called a pollinarium, which consists of pollinia (sac-shaped masses of pollen grains) from two adjacent anthers connected by sterile structures called the translator (Kunze Reference Kunze1991). A notch in the center of the translator enables pollinarium dispersal by catching onto the proboscis (or perhaps leg) of an insect as the proboscis is removed from a floral nectary (DiTommaso et al. Reference DiTommaso, Lawlor and Darbyshire2005b; Kunze Reference Kunze1991).
In Ontario, up to 25% of naturally growing V. rossicum flowers had been visited by insects, as evidenced by the removal of pollinaria (St. Denis and Cappuccino Reference St. Denis and Cappuccino2004). In New York, Lumer and Yost (Reference Lumer and Yost1995) reported that V. nigrum plants were cross-pollinated exclusively by flies. The flies—mostly small, unspecialized species with short tongues—were apparently attracted by the dark color and strong odor of V. nigrum flowers. At least 14 species visited the flowers, six of which carried pollinia. Although observations of floral visitors in Ontario and New York are typically rare, other researchers have reported visits by flies, ants, bees, wasps, and beetles (Christensen Reference Christensen1998; Maclvor et al. Reference MacIvor, Roberto, Sodhi, Onuferko and Cadotte2017; Milbrath Reference Milbrath2010; St. Denis and Cappuccino Reference St. Denis and Cappuccino2004). Nocturnal visitors are possible but have not been documented if present. In Europe, V. hirundinaria is mainly pollinated by large flies, moths, and bees (Leimu Reference Leimu2004). Further research is needed to clarify which (if any) insects are important pollinators of invasive Vincetoxicum species and how pollinator availability affects population dynamics.
Self-pollination (autogamy) is a rare trait in Vincetoxicum sensu lato and elsewhere in the Asclepiadoideae (Liede-Schumann et al. Reference Liede-Schumann, Khanum, Mumtaz, Gherghel and Pahlevani2016). This trait has been reported in only 5 of 68 Vincetoxicum representatives surveyed by Liede-Schumann et al. (Reference Liede-Schumann, Khanum, Mumtaz, Gherghel and Pahlevani2016), including V. rossicum, V. nigrum, and V. hirundinaria. Self-compatibility tends to facilitate establishment in uncolonized areas after long-distance dispersal events (“Baker’s law”; Baker Reference Baker1955, Reference Baker1967). Consequently, self-compatibility could promote invasiveness in diverse taxa (Pannell et al. Reference Pannell, Auld, Brandvain, Burd, Busch, Cheptou, Conner, Goldberg, Grant, Grossenbacher, Hovick, Igic, Kalisz, Petanidou and Randle2015), including groups within the Asclepiadoideae (Ward et al. Reference Ward, Johnson and Zalucki2012). Disadvantages of self-pollination are often—but not always—insignificant in the short term. In a glasshouse study of V. hirundinaria in Finland, cross-pollination increased fruit set (both initiated and mature, despite increased abortion) relative to self-pollination (Leimu Reference Leimu2004). These differences were not observed in an analysis including only self-fertile individuals. A field study in Finland found that V. hirundinaria reproduces primarily by outcrossing, perhaps due to inbreeding depression among the offspring of self-pollinated plants (Muola et al. Reference Muola, Scheepens, Laukkanen, Kalske, Mutikainen and Leimu2021). Evidence for inbreeding depression in V. hirundinaria is mixed, partially due to substantial methodological differences between studies (Leimu Reference Leimu2004; Leimu and Mutikainen Reference Leimu and Mutikainen2005; Muola et al. Reference Muola, Mutikainen, Laukkanen, Lilley and Leimu2011). In a greenhouse study of V. rossicum in Ontario, pollination method (self-pollination or cross-pollination) did not affect fruit set, seed production, seed weight, or embryony (St. Denis and Cappuccino Reference St. Denis and Cappuccino2004). Similarly, pollination method did not affect the germination rate of V. nigrum seeds in New York, which was measured at 40% for seeds produced by self-pollination and 49% for seeds produced by open pollination (Lumer and Yost Reference Lumer and Yost1995).
Seed Production and Dispersal
Vincetoxicum seeds are primarily wind-dispersed, with some animal dispersal and anthropogenic transport over long distances (see “Dispersal and Establishment”). Adaptations for wind dispersal include a membranous marginal wing and a large coma (DiTommaso et al. Reference DiTommaso, Lawlor and Darbyshire2005b). Viable seed production per follicle is reported to be 5 to 13 (mean of 8) in V. rossicum and 7 to 12 (mean of 10) in V. nigrum (Averill et al. Reference Averill, DiTommaso, Mohler and Milbrath2011). Follicle size parameters, especially weight, are correlated with the number of seeds per follicle in V. rossicum (Sheeley Reference Sheeley1992). In a high-light greenhouse environment, mature V. nigrum produced more follicle dry mass, seeds per follicle, and seeds per plant than mature V. rossicum (Milbrath Reference Milbrath2008). Because V. nigrum seeds are heavier than V. rossicum seeds (20.3 vs. 7.2 mg; Milbrath Reference Milbrath2008), total seed mass would be larger in V. nigrum even if seed numbers were similar. In agreement with the fact that V. nigrum begins reproducing earlier than V. rossicum (see “Life-Form and Life History”), nondefoliated V. nigrum seedlings produced follicles (4 plant−1) and seeds (27 plant−1) under high light, whereas V. rossicum seedlings typically did not reproduce (Milbrath Reference Milbrath2008). Under low light, seedlings did not flower and mature plants of both species flowered but did not produce follicles or seed (Milbrath Reference Milbrath2008). Similarly, in a common garden experiment in central New York, more than 12% of V. nigrum seedlings but less than 1% of V. rossicum seedlings produced follicles in their first growing season (Magidow et al. Reference Magidow, DiTommaso, Ketterings, Mohler and Milbrath2013). Follicle production does not typically occur in the first year under natural levels of stress and competition.
Among Vincetoxicum plants, maturity and site may explain more variation in fecundity than species. In a study of six New York populations of V. rossicum (forest and field) and V. nigrum (field only), Milbrath et al. (Reference Milbrath, Davis and Biazzo2017) measured fecundity (viable seeds per plant) in small (one to two stems) and large (three or more stems) flowering plants. For small flowering V. rossicum, fecundities were 4 and 67 seeds plant−1 at forest sites, compared with 95 and 177 seeds plant−1 at field sites. Large flowering V. rossicum had fecundities of 221 to 1,051 seeds plant−1. Vincetoxicum nigrum fecundities were 17 and 98 seeds plant−1 at the small flowering stage, then 48 and 963 seeds plant−1 at the large flowering stage. Although the effect of species on field population fecundity was significant for small flowering plants, these data are a stronger illustration of between-site variation. In central and southeastern New York, V. rossicum and V. nigrum produced similar numbers of viable seeds (130 and 100 seeds stem−1 yr−1, respectively) in old-field habitats (Averill et al. Reference Averill, DiTommaso, Mohler and Milbrath2011). Vincetoxicum rossicum usually produced very few viable seeds in forests (fewer than 25 seeds stem−1 yr−1) but produced up to 170 seeds stem−1 yr−1 at a higher-light forest site (Averill et al. Reference Averill, DiTommaso, Mohler and Milbrath2011). The authors suggested that the establishment and maintenance of low-light (forest) populations sometimes depends on propagule pressure from nearby high-light (field) populations. If so, management of high-light populations should limit the expansion of low-light populations as well.
Seed production per unit area depends largely on environmental conditions and plant density. Smith et al. (Reference Smith, DiTommaso, Lehmann and Greipsson2006) measured follicle and seed densities in shade (2,000 follicles m−2, 28,000 seeds m−2), intermediate sun (2,250 follicles m−2, 32,000 seeds m−2), and full sun (1,600 follicles m−2, 25,000 seeds m−2) plots at a site densely infested with V. rossicum. Seeds produced in full sun had higher viability, less dormancy, and higher rates of polyembryony. End-of-season seedling densities under high light were 1,136 and 1,654 seedlings m−2 in the 2 study years. Seed production per unit area may be much lower in less dense patches. Sheeley (Reference Sheeley1992) reported that V. rossicum populations occupying shaded and open sites produced 1,330 seeds m−2 and 2,090 seeds m−2, respectively. Mean seed production per flowering ramet was 15 in the shaded site and 85 in the open site (ramet density was higher at the shaded site).
Vincetoxicum seeds exhibit high rates of polyembryony (DiTommaso et al. Reference DiTommaso, Lawlor and Darbyshire2005b; Figure 8). Polyembryony in V. rossicum appears to be adventitious (additional embryos arising from maternal tissue rather than cleavage of the fertilized embryo), but this ontogeny has not been definitively proven or studied in most other members of the Vincetoxicum genus (Blanchard et al. Reference Blanchard, Barney, Averill, Mohler and DiTommaso2010; Hotchkiss et al. Reference Hotchkiss, DiTommaso, Brainard and Mohler2008). In V. rossicum, Sheeley (Reference Sheeley1992) reported that 22% of seeds contained one embryo, 35% contained two embryos, 40% contained three embryos, and 3% contained four embryos. These rates were comparable to an existing data set in which 13% of V. rossicum seeds contained one embryo, 39% contained two embryos, 35% contained three embryos, 8% contained four embryos, and 5% contained five embryos (von Hausner 1976 as cited by DiTommaso et al. Reference DiTommaso, Lawlor and Darbyshire2005b). Smith et al. (Reference Smith, DiTommaso, Lehmann and Greipsson2006) reported up to eight radicles emerging from a V. rossicum seed, although most seeds only had one or two radicles. Light availability had a positive effect on mean embryony in 1 of 2 yr (Smith et al. Reference Smith, DiTommaso, Lehmann and Greipsson2006). According to other estimates, nearly (Ladd and Cappuccino Reference Ladd and Cappuccino2005) or more than (Cappuccino et al. Reference Cappuccino, Mackay and Eisner2002; St. Denis and Cappuccino Reference St. Denis and Cappuccino2004) half of V. rossicum seeds are polyembryonic and produce multiple seedlings. Seed weight sometimes increases with embryony in V. rossicum, but this effect is not consistent (Cappuccino et al. Reference Cappuccino, Mackay and Eisner2002; DiTommaso et al. Reference DiTommaso, Brainard and Webster2005a). Other Vincetoxicum species have lower rates of polyembryony, with 62% to 78% (V. nigrum) and 95% (V. hirundinaria) of seeds containing a single embryo (DiTommaso et al. Reference DiTommaso, Milbrath, Morris, Mohler and Biazzo2017; von Hausner 1976 as cited by Sheeley Reference Sheeley1992). Douglass et al. (Reference Douglass, Weston and DiTommaso2009) indicated that a V. rossicum seed was 10 times more likely to be polyembryonic than a V. nigrum seed.

Figure 8. Vincetoxicum rossicum seedlings emerging from a polyembryonic seed. (Photo credit: Scott Morris.)
Polyembryony may mitigate risks associated with environmental variability and increase the likelihood that a seed that undergoes long-distance dispersal will found a new population (DiTommaso et al. Reference DiTommaso, Lawlor and Darbyshire2005b). Although an individual embryo in a polyembryonic seed may be less likely to survive than an embryo in a monoembryonic seed, a polyembryonic seed may be more likely to produce at least one surviving seedling (Ladd and Cappuccino Reference Ladd and Cappuccino2005). Averill et al. (Reference Averill, DiTommaso, Mohler and Milbrath2010) reported that polyembryony increased emergence and survival in old fields. Staggered embryo emergence times (up to 20-d difference) may increase the likelihood that at least one seedling will avoid acute stresses (Ladd and Cappuccino Reference Ladd and Cappuccino2005). When multiple seedlings survive, the clump of several seedlings may be more fit or more competitive than an isolated seedling (Ladd and Cappuccino Reference Ladd and Cappuccino2005; Sheeley Reference Sheeley1992). However, Cappuccino et al. (Reference Cappuccino, Mackay and Eisner2002) found that polyembryonic V. rossicum seeds produced greater total seedling weights than monoembryonic seeds only in the absence of competition. Embryony did not affect total seedling weight under competition from grasses. Similarly, Blanchard et al. (Reference Blanchard, Barney, Averill, Mohler and DiTommaso2010) reported that polyembryonic individuals seldom outperformed monoembryonic individuals under intraspecific or interspecific competition. This finding suggests that polyembryony is not an adequate explanation for V. rossicum invasion of established plant communities, although it may provide some advantages. For example, seedlings from a single seed may share the costs of allelochemical production and rhizosphere priming (Blanchard et al. Reference Blanchard, Barney, Averill, Mohler and DiTommaso2010). Hotchkiss et al. (Reference Hotchkiss, DiTommaso, Brainard and Mohler2008) found that polyembryony did not increase survival or long-term growth in V. rossicum grown in forest gaps or full shade. The authors suggested that polyembryony might be more useful in high-light environments such as old fields. Taken together, these studies provide stronger evidence for polyembryony as a bet-hedging strategy in disturbed environments than polyembryony as an adaptation for increased competitiveness.
Seedbanks, Seed Viability, and Germination
Seed viability has been reported at 56% to 71% in V. rossicum, depending on light availability, and 68% in V. nigrum (Milbrath Reference Milbrath2008; Smith et al. Reference Smith, DiTommaso, Lehmann and Greipsson2006). In V. rossicum, seeds produced under low light were more likely to be nonviable (unfilled; Smith et al. Reference Smith, DiTommaso, Lehmann and Greipsson2006). After discarding unfilled seeds, Magidow et al. (Reference Magidow, DiTommaso, Ketterings, Mohler and Milbrath2013) reported viability rates of 88% and 86% for seeds of V. rossicum and V. nigrum, respectively, collected in August and viability rates of 100% and 93% for seeds of V. rossicum and V. nigrum, respectively, collected in October. Milbrath et al. (Reference Milbrath, Davis and Biazzo2017) measured initial viabilities of 93% to 99% among filled seeds of V. rossicum and V. nigrum.
The mechanistic basis of seed dormancy in Vincetoxicum is not well understood, but most seeds exhibit dormancy in addition to environmental requirements for germination. Most seeds germinate after overwintering, although some nondormant seeds are produced each autumn (DiTommaso et al. Reference DiTommaso, Brainard and Webster2005a, 2005b; Lumer and Yost Reference Lumer and Yost1995; Milbrath et al. Reference Milbrath, Davis and Biazzo2017). Milbrath et al. (Reference Milbrath, Davis and Biazzo2017) measured emergence rates of V. rossicum and V. nigrum in New York by sowing seeds in August or October and counting emerged seedlings over several seasons. Seed survival was highly variable (14% to 74%) and contributed to variation in estimated germination rates (emergence rates divided by seed survival rates). Germination and emergence were typically concentrated in the spring after sowing, with lower rates in the second spring. At one site, emergence was higher in the second spring, perhaps because water was limiting in the first year. In field populations (V. rossicum and V. nigrum), autumn emergence was very rare in the sowing year (before the first winter) and moderately rare in the following year. Autumn emergence was not observed in forest populations (V. rossicum). Across species, habitat, and sowing time, emergence in the first year after sowing varied from 5% to 39%.
Seed characteristics and environmental conditions influence germination and emergence rates. In V. rossicum, germination rates have been reported to vary between 45% and 95% and increase under alternating temperature and light intensities (Dickinson and Royer Reference Dickinson and Royer2014, p. 40). Sheeley (Reference Sheeley1992) tested the germination of V. rossicum seeds from New York under growth chamber and greenhouse conditions. Seeds were collected in October and stored under dark, cold conditions for approximately 1 mo (growth chamber) or 7 mo (greenhouse). The growth chamber experiment included a dark treatment and a light treatment (14-h photoperiod) with temperatures fluctuating in daily cycles (13 to 21 C early in the trial and 20 to 27 C subsequently). Germination rates were 36% in the dark treatment and 46% in the light treatment. Under greenhouse conditions (range: 25 to 31 C), the germination rate was only 23%. DiTommaso et al. (Reference DiTommaso, Brainard and Webster2005a) reported that the site of seed production, seed harvest date, and period of seed storage affected seed germinability in V. rossicum from New York. For example, fresh seeds (no storage period) collected from old-field sites in August had the highest germination rates (30% to 50%) of all groups tested. Fresh seeds collected from old-field sites in November had lower germination rates. Cold-dry storage had a large negative effect on germination in seeds collected in August relative to seeds collected in November. Among fresh seeds collected in August, seeds produced in a forest were more dormant (germinated at a lower rate) and heavier than seeds produced in an adjacent old field. The production of dormant V. rossicum seeds under low light has been reported elsewhere (Smith et al. Reference Smith, DiTommaso, Lehmann and Greipsson2006). Magidow et al. (Reference Magidow, DiTommaso, Ketterings, Mohler and Milbrath2013) reported much higher germination rates for V. rossicum and V. nigrum in a growth chamber experiment (seeds from New York, cold-dry storage followed by germination at 0.6 cm soil depth, 25/17 C, 14-h photoperiod). Percentage germination (emergence) was higher on a Wassaic silt loam (75%) than a Hollis gravelly loam (66%) with no difference between species or soil pH levels. Species, soil type, and soil pH sometimes interacted to influence germination speed. Cold-wet stratification for at least 3 mo usually results in >95% germination (LRM, unpublished data).
There is mixed evidence for an effect of V. rossicum seed size on germination or emergence (Cappuccino et al. Reference Cappuccino, Mackay and Eisner2002; Douglass et al. Reference Douglass, Weston and DiTommaso2009). Ladd and Cappuccino (Reference Ladd and Cappuccino2005) reported that larger V. rossicum seeds were slightly more likely to emerge under field conditions (seeds were sown in April in Ontario and emergence was tracked for 3 yr). Burial depth was a more important influence on emergence, with V. rossicum emerging at total (multiyear) rates of 51% for seeds sown on the surface and 72% for seeds buried at 1 cm (Ladd and Cappuccino Reference Ladd and Cappuccino2005). Surface-sown seeds were more likely to remain dormant for at least 1 yr before emerging. In an outdoor experiment in central New York, seeds were surface-sown in November into outdoor pots (various soils), and seedlings began to emerge in May (Magidow et al. Reference Magidow, DiTommaso, Ketterings, Mohler and Milbrath2013). Seedling emergence rates were higher in V. rossicum than V. nigrum across soil types and pH levels. In the same geographic area, Averill et al. (Reference Averill, DiTommaso, Mohler and Milbrath2010) found that emergence of V. rossicum (seeds sown at 2 mm) was typically lower and more dependent on disturbance treatment at a poorly drained site relative to a better-drained site.
Vincetoxicum rossicum and V. nigrum do not persist long in the seedbank. In a 4-yr pot experiment in an old field in central New York, most seedlings (92%) emerged in the first year after burial, and no seedlings of either species emerged in the third or fourth years (DiTommaso et al. Reference DiTommaso, Milbrath, Morris, Mohler and Biazzo2017). No viable seeds were recovered after the second growing season, and seeds were badly deteriorated after the third growing season. Other reports have indicated that V. rossicum emergence is concentrated in the first year and very low by the third year (Averill et al. Reference Averill, DiTommaso, Mohler and Milbrath2010; Ladd and Cappuccino Reference Ladd and Cappuccino2005; Milbrath et al. Reference Milbrath, Davis and Biazzo2017) and that V. rossicum seeds remain viable for 3 to 4 yr under field conditions (Dickinson and Royer Reference Dickinson and Royer2014, p. 40). DiTommaso et al. (Reference DiTommaso, Milbrath, Morris, Mohler and Biazzo2017) also tested the effect of burial depth (0, 1, 5, or 10 cm) on emergence. Vincetoxicum rossicum, the smaller-seeded species, achieved its highest emergence rate (37%) at the 1-cm burial depth. Other burial depths resulted in lower emergence (11% at 0 cm, 6% at 5 cm, and 0.05% at 10 cm). Vincetoxicum nigrum, the larger-seeded species, had higher emergence rates (16% at 0 cm, 71% at 1 cm, 66% at 5 cm, and 26% at 10 cm). Burial at 5 cm (V. rossicum) or 10 cm (both species) often resulted in fatal germination, suggesting that Vincetoxicum recruitment could be limited by deep seed burial. However, it may be more practical to focus on preventing new additions to the seedbank.
Vegetative Reproduction
Invasive Vincetoxicum species do not appear to reproduce vegetatively. Plants can increase their stem number over time (see “Life-Form and Life History”), but all stems sprout from the same root crown. Early reports of V. nigrum reproduction by rhizomes (Lumer and Yost Reference Lumer and Yost1995) have not been confirmed (Averill et al. Reference Averill, DiTommaso, Mohler and Milbrath2011). The rhizome-like structure observed connecting V. nigrum root crowns at one location in southeastern New York remains to be properly described (12% of sampled plants; LRM, unpublished data; Figure 1E).
Population Dynamics
The establishment and persistence of invasive Vincetoxicum populations depend on high survival rates, gradual resource accumulation, high seed production in mature individuals under good conditions, and long life spans. Milbrath et al. (Reference Milbrath, Davis and Biazzo2017, Reference Milbrath, Davis and Biazzo2018) parameterized a demographic matrix model to identify the reductions in survival, maturation, and reproduction (vital rates) necessary to prevent population growth in lower-density patches of V. rossicum and V. nigrum in New York. Annual seed survival and seedling emergence were highly variable (see “Reproduction: Seedbanks, Seed Viability, and Germination”). Survival rates were typically high for seedlings and juveniles (72% to 100%, exceptions included a survival rate of 33% for V. rossicum seedlings at a heavily shaded forest site) and very high (98% to 100%) for flowering plants (Milbrath et al. Reference Milbrath, Davis and Biazzo2017). Similarly, Ladd and Cappuccino (Reference Ladd and Cappuccino2005) reported 71% to 100% survival for first-year seedlings of V. rossicum, and Averill et al. (Reference Averill, DiTommaso, Mohler and Milbrath2011) reported 100% survival for established clumps of V. rossicum and V. nigrum. Seedling densities can decline up to approximately 80% within a season under very high densities (Smith et al. Reference Smith, DiTommaso, Lehmann and Greipsson2006). Some V. nigrum plants became reproductive as early as their third year of growth (Milbrath et al. Reference Milbrath, Davis and Biazzo2017), whereas V. rossicum typically requires 6 or more years to begin flowering (LRM and AD, unpublished data). Few large flowering plants (three or more stems) of V. rossicum were observed in forests (Milbrath et al. Reference Milbrath, Davis and Biazzo2017), supporting observations that vegetative expansion occurs slowly, if at all, in established forest clumps of V. rossicum (Averill et al. Reference Averill, DiTommaso, Mohler and Milbrath2011). Based on these data, Milbrath et al. (Reference Milbrath, Davis and Biazzo2018) projected that disruptions of some individual survival, growth, or fecundity rates could prevent population growth in slower-growing populations (λ < 1.6). However, control of rapidly growing populations (λ = 1.6 to 2.5) would require very large and simultaneous reductions in multiple vital rates (e.g., reductions in both seedling and adult survival).
A severe Vincetoxicum infestation interferes with the growth of native plants by forming a dense mat of twining stems (DiTommaso et al. Reference DiTommaso, Lawlor and Darbyshire2005b; Livingstone et al. Reference Livingstone, Isaac and Cadotte2020a). Vincetoxicum rossicum may establish stands that are larger and have higher Vincetoxicum cover than V. nigrum stands (Magidow et al. Reference Magidow, DiTommaso, Westbrook, Kwok, Ketterings and Milbrath2022). However, both species can establish near-monospecific stands (DiTommaso et al. Reference DiTommaso, Lawlor and Darbyshire2005b; Figure 4). This ability reflects their growth habit and their potential for high stand densities, typically 100 to 200 stems m−2 under high light with up to 400 stems m−2 at some locations (Milbrath et al. Reference Milbrath, Davis and Biazzo2018; Sheeley Reference Sheeley1992; Smith et al. Reference Smith, DiTommaso, Lehmann and Greipsson2006). It is probably difficult for other species to survive in the dark understory under a mat of V. rossicum (Cappuccino Reference Cappuccino2004). Suppression of interspecific competitors is one reason that V. rossicum plants may perform better in large patches than in small patches or isolation (an Allee effect; Cappuccino Reference Cappuccino2004; Jackson and Amatangelo Reference Jackson and Amatangelo2021). This hypothesis is consistent with greenhouse data showing increased V. rossicum performance under intraspecific competition relative to interspecific competition (Blanchard et al. Reference Blanchard, Barney, Averill, Mohler and DiTommaso2010; Jackson and Amatangelo Reference Jackson and Amatangelo2021). However, field studies using potted V. nigrum and V. hirundinaria plants in France have not fully supported an Allee effect (Maguire et al. Reference Maguire, Sforza and Smith2011). The size and fecundity of Vincetoxicum patches depend not only on competitive dynamics but also on climate factors. For example, patches of V. hirundinaria in Sweden grew larger as the growing season lengthened in recent decades (Solbreck Reference Solbreck2012; Solbreck and Knape Reference Solbreck and Knape2017). Climate factors also influenced patterns of seed production and therefore seed predation (Solbreck and Knape Reference Solbreck and Knape2017). These findings could provide insight into the potential effects of climate change on Vincetoxicum species in their introduced North American ranges.
Management Options
Phytosanitary Measures
Phytosanitary measures are not a major focus of Vincetoxicum management efforts. The propagation and transport of these weeds are prohibited across much of the North American range (Supplementary Table S1). However, no specific programs or recommendations have been developed to limit accidental transport.
Chemical
Herbicide applications generally provide good Vincetoxicum control. Lawlor and Raynal (Reference Lawlor and Raynal2002) found that foliar spray applications of glyphosate (3.1 or 7.8 kg ae ha−1) or triclopyr (1.9 kg ae ha−1) provided better V. rossicum control than cut-stem applications of glyphosate (3.1 kg ae ha−1) or triclopyr (1.4 kg ae ha−1). In another experiment, they found that cut-stem glyphosate applications (3.1 or 6.2 kg ae ha−1) outperformed cut-stem triclopyr applications (1.4, 2.8, or 5.6 kg ae ha−1) at all application rates. No treatment prevented V. rossicum regrowth and new seedling emergence, so the authors concluded that long-term control would require retreatment (Lawlor and Raynal Reference Lawlor and Raynal2002). Two- or three-application programs of glyphosate or triclopyr can achieve good V. rossicum control (Cain and Irvine Reference Cain and Irvine2011; Cain and Mervosh Reference Cain and Mervosh2012; Christensen Reference Christensen1998; Mervosh and Boettner Reference Mervosh and Boettner2009). In some areas, single applications of triclopyr or imazapyr may suppress V. rossicum for up to two growing seasons (Averill et al. Reference Averill, DiTommaso and Morris2008; Cain and Irvine Reference Cain and Irvine2011; Cain and Mervosh Reference Cain and Mervosh2012). Other herbicides that have been tested on V. rossicum include aminocyclopyrachlor, aminopyralid, imazapic, and metsulfuron (Gover and Rung Reference Gover and Rung2015; Mervosh and Boettner Reference Mervosh and Boettner2009).
Vincetoxicum rossicum is more easily controlled with herbicides than with entirely nonchemical approaches. For example, Mervosh and Gumbart (Reference Mervosh and Gumbart2015) performed chemical or nonchemical control annually for 2 yr. In the third year, V. rossicum cover and vigor were lower in glyphosate treatments (foliar spray, 2.2 kg ai ha−1, or cut-stem) than hand pulling or cutting treatments. A cut-stem triclopyr treatment provided an intermediate level of suppression, but a triclopyr foliar spray (1.7 kg ai ha−1) was not superior to hand pulling or cutting. In a 2-yr study, Averill et al. (Reference Averill, DiTommaso and Morris2008) compared the effects of triclopyr (1.9 kg ae ha−1, first year only), clipping (once or twice per year), and triclopyr plus clipping (1.9 kg ae ha−1 plus one clipping in the first year, one clipping in the second year) on V. rossicum cover and density. The triclopyr and triclopyr plus clipping treatments were not significantly different. Both treatments were superior to the single-clipping and double-clipping treatments. At an old-field site, DiTommaso et al. (Reference DiTommaso, Milbrath, Bittner and Wesley2013) found that V. rossicum was effectively suppressed by combination treatments (one cutting plus one herbicide application per year) but not by cutting alone (twice per year). Glyphosate (3.65 kg ae ha−1) suppressed both V. rossicum and non-target species. For this reason, treatments with a high rate of triclopyr salt (4.87 vs. 0.93 kg ae ha−1) or triclopyr ester (4.87 vs. 2.99 kg ae ha−1) were considered most successful. DiTommaso et al. (Reference DiTommaso, Milbrath, Bittner and Wesley2013) also tested the combination and cutting-only treatments in a forest understory (herbicides were applied at lower rates at this site because V. rossicum density was lower). At the forest site, most combination treatments reduced V. rossicum cover, but no treatment reduced V. rossicum stem density relative to the control. These findings underscore the influence of habitat on Vincetoxicum management outcomes. Only one chemical control study has been conducted with V. nigrum. Glyphosate greatly reduced V. nigrum biomass, but mowing several weeks before spraying did not always increase the effectiveness of herbicide treatments. Triclopyr was ineffective, but additional approaches including higher rates or greater frequency of application should be investigated (Milbrath et al. Reference Milbrath, Biazzo, Morris and DiTommaso2022).
Cultural
Vincetoxicum plants are difficult to kill by mowing or clipping because they recover from aboveground damage by drawing on root reserves. Annual or repeated mowing over 1 to 2 yr typically does not reduce V. rossicum density or cover and may even facilitate establishment by limiting interspecific competition for light (Averill et al. Reference Averill, DiTommaso and Morris2008, Reference Averill, DiTommaso, Mohler and Milbrath2010; Christensen Reference Christensen1998; DiTommaso et al. Reference DiTommaso, Milbrath, Bittner and Wesley2013). In a 7-yr study in New York, Biazzo and Milbrath (Reference Biazzo and Milbrath2019) found that mowing V. rossicum three times per growing season reduced V. rossicum stem density, root crown density, and percent cover relative to an unmowed control, but these effects were only observed after 3 to 5 yr of treatment. Mowing six times per season provided no additional benefit. The authors concluded that mowing three times per growing season could suppress V. rossicum but would not eliminate existing patches. McKague and Cappuccino (Reference McKague and Cappuccino2005) determined that a properly timed, single clipping of V. rossicum at ground level could prevent seed production. However, if mowed at a standard 8-cm height, V. nigrum and V. rossicum can quickly regrow from axillary buds. Although two mowings or clippings per season greatly reduce that year’s seed production, three mowings are needed to consistently eliminate it (Averill et al. Reference Averill, DiTommaso and Morris2008; Biazzo and Milbrath Reference Biazzo and Milbrath2019; Milbrath et al. Reference Milbrath, DiTommaso, Biazzo and Morris2016).
Among treatments other than mowing and clipping, the most effective solutions are often the most labor-intensive. Manual removal of V. rossicum follicles can limit seed rain, but maturing follicles must be removed throughout the late summer and early fall (DiTommaso et al. Reference DiTommaso, Lawlor and Darbyshire2005b). Digging V. rossicum out of the soil is more effective than hand pulling, which usually fails to remove the root crown (DiTommaso et al. Reference DiTommaso, Lawlor and Darbyshire2005b; Lawlor Reference Lawlor2002). Once dug up, root crowns must be removed from the site because they can regrow if left on the ground. Vincetoxicum rossicum might even survive cultivation by regrowth from root crown fragments, although regularly tilled fields are not suitable habitats (DiTommaso et al. Reference DiTommaso, Lawlor and Darbyshire2005b; Lawlor Reference Lawlor2002). Trampling and burning may suppress V. rossicum but should not be expected to provide long-term control (DiTommaso et al. Reference DiTommaso, Lawlor and Darbyshire2005b; Lawlor Reference Lawlor2002). Light-excluding mulches (e.g., black plastic) can suppress V. rossicum if they are sufficiently durable (Christensen Reference Christensen1998; Miller and Kricsfalusy Reference Miller and Kricsfalusy2008). Many of these cultural methods are appropriate for controlling Vincetoxicum in small areas, but cannot be applied across larger, less intensively managed areas.
Biological
Vincetoxicum rossicum and V. nigrum harbor few arthropods, mainly with little to no damage, in their introduced North American range (see “Importance”; Carpenter and Cappuccino Reference Carpenter and Cappuccino2005; Ernst and Cappuccino Reference Ernst and Cappuccino2005; Milbrath Reference Milbrath2010; Milbrath and Biazzo Reference Milbrath and Biazzo2012). In contrast, V. hirundinaria and other Vincetoxicum species host multiple herbivores in Europe and Asia, many of which were considered promising for biological control (Tewksbury et al. Reference Tewksbury, Casagrande and Gassmann2002; Weed et al. Reference Weed, Gassmann, Leroux and Casagrande2011b; LRM, unpublished data). As a guide to eventual biological control releases, artificial herbivory studies were initially conducted in North America. A study using natural infestations of V. rossicum in Ontario indicated that increasing damage intensity (0% to 90% of either leaf or root removal) reduced V. rossicum seed production in sunny sites up to 80% (Doubleday and Cappuccino Reference Doubleday and Cappuccino2011). Root damage was slightly more effective than leaf removal, but repeated leaf removal (twice vs. once) did not further reduce seed production (Doubleday and Cappuccino Reference Doubleday and Cappuccino2011). In contrast to this study, defoliation treatments (50% or 100% defoliation, once or twice per growing season for 6 yr) had little effect on growth or reproduction of V. rossicum and V. nigrum in a common garden experiment under full-sun conditions in New York (Milbrath et al. Reference Milbrath, DiTommaso, Biazzo and Morris2016). The results of Milbrath et al. (Reference Milbrath, DiTommaso, Biazzo and Morris2016) also contradicted a greenhouse study in which repeated defoliation (100%, twice) was suggested to be valuable in reducing biomass and seed production in high-light environments (Milbrath Reference Milbrath2008). Defoliation (100%) under low light, simulating a heavily shaded forest understory, caused high mortality of seedlings and mature plants of both Vincetoxicum species (Milbrath Reference Milbrath2008). Thus, a combination of stressors might help manage forest infestations of these species, but tolerance to aboveground damage may hamper biological control efforts in open-field infestations.
The leaf-feeding moth Hypena opulenta Christoph (Lepidoptera: Erebidae) was collected in a forested area from V. rossicum and Vincetoxicum scandens Sommier & Levier in Ukraine (Weed and Casagrande Reference Weed and Casagrande2010). It was found to successfully develop only on Vincetoxicum species in no-choice tests, and it is expected to produce two generations per summer (Hazlehurst et al. Reference Hazlehurst, Weed, Tewksbury and Casagrande2012; Weed and Casagrande Reference Weed and Casagrande2010). Laboratory and greenhouse impact assessments showed that H. opulenta significantly reduced aboveground biomass and seed production in mature V. rossicum and V. nigrum plants, with greater reductions occurring with greater frequency of defoliation and increased shading of the plants (Milbrath and Biazzo Reference Milbrath and Biazzo2016; Weed and Casagrande Reference Weed and Casagrande2010). Defoliation by H. opulenta also reduced seedling growth and killed V. nigrum seedlings that were completely defoliated twice under low light (Milbrath and Biazzo Reference Milbrath and Biazzo2016).
Hypena opulenta was first released in Ottawa, Ontario beginning in 2013 and has successfully established at this site, producing two generations per summer (Bourchier et al. Reference Bourchier, Cappuccino, Rochette, des Rivières, Smith, Tewksbury and Casagrande2019). In the United States, H. opulenta has been annually released since 2017 (Connecticut, Massachusetts, Michigan, New York, Rhode Island), but establishment has yet to be reported for these release sites or additional Canadian sites (Alred Reference Alred2021; Alred et al. Reference Alred, Hufbauer and Szűcs2022b; Livingstone et al. Reference Livingstone, Smith, Bourchier, Ryan, Roberto and Cadotte2020b; LRM, personal observation). Although larvae will feed nocturnally on V. rossicum in high-light (meadow) environments in which they are released, they appear to prefer the shaded conditions of low-light (forest understory) habitats (Livingstone et al. Reference Livingstone, Smith, Bourchier, Ryan, Roberto and Cadotte2020b; Rochette Reference Rochette2019). A field cage study showed that H. opulenta defoliation of V. nigrum was higher in a shaded environment, relative to a sunny environment, but the low levels of defoliation did not reduce V. nigrum fitness in either environment (Alred et al. Reference Alred, Hufbauer and Szűcs2022b). Hypena opulenta seems likely to be most useful in controlling slow-growing forest populations of V. rossicum and any similar populations of V. nigrum (forest populations of V. nigrum are not as common; Milbrath et al. Reference Milbrath, Davis and Biazzo2018). Adult females lay single eggs, and the larvae do not aggregate, reducing the amount of feeding damage inflicted on individual plants unless H. opulenta populations reach high levels throughout an area (Bourchier et al. Reference Bourchier, Cappuccino, Rochette, des Rivières, Smith, Tewksbury and Casagrande2019). Pupal diapause is induced in H. opulenta at a threshold photoperiod of 15 h 35 min (Jones et al. Reference Jones, Seehausen, Bourchier and Smith2020). Two generations per summer have been shown to occur at release sites >42°N latitude when H. opulenta was experimentally released by late June (daylength of 15 h 20 min or more; Alred et al. Reference Alred, Hufbauer and Szűcs2022b; Jones et al. Reference Jones, Seehausen, Bourchier and Smith2020). However, the threshold photoperiod may result in only one generation per summer at more southern latitudes in North America, reducing the potential impact of H. opulenta on Vincetoxicum infestations. Other factors influencing establishment and population increase of the moth may include substantial pupal mortality, resulting from a combination of late-summer predation and overwintering mortality, and the availability of adult food sources (Jones et al. Reference Jones, Smith and Bourchier2022; Seehausen et al. Reference Seehausen, Timm, Jones, Bourchier and Smith2019).
Like H. opulenta, the European leaf-feeding moth Abrostola asclepiadis (Denis & Schiffermüller) (Lepidoptera: Noctuidae) can only successfully develop on Vincetoxicum species (Hazlehurst Reference Hazlehurst2011). This moth is likely to be univoltine if released in North America, limiting its potential impact on Vincetoxicum species (Milbrath et al. Reference Milbrath, Dolgovskaya, Volkovitsh, Sforza and Biazzo2019b). Under greenhouse conditions, defoliation by A. asclepiadis and other stressors (shading or competition with a grass) had additive effects on growth and reproduction of V. rossicum and V. nigrum (Milbrath et al. Reference Milbrath, Biazzo, DiTommaso and Morris2019a). However, a single defoliation by A. asclepiadis had no measurable impact on either species (plant growth measured 1 yr after defoliation) in an outdoor experiment and is not projected to control most populations of V. rossicum or V. nigrum (Milbrath et al. Reference Milbrath, Davis and Biazzo2018, Reference Milbrath, Biazzo, DiTommaso and Morris2019a; Weed et al. Reference Weed, Gassmann and Casagrande2011a). The potential for competition with H. opulenta is also a concern because, while A. asclepiadis is found in sunny habitats, it prefers shaded patches of its typical host (V. hirundinaria) in Europe (Förare Reference Förare1995; Förare and Engqvist Reference Förare and Engqvist1996). No release petition has been submitted for this moth to date.
The European beetle Chrysochus asclepiadeus (Pallas) (syn.: Eumolpus asclepiadeus Pallas) (Coleoptera: Chrysomelidae) feeds on leaves as an adult and on roots as a larva. It was considered a promising Vincetoxicum biological control agent particularly due to its ability to significantly reduce root biomass and disrupt water and nutrient uptake (Maguire et al. Reference Maguire, Sforza and Smith2011; Sforza et al. Reference Sforza, Towmey, Maguire, Riquier, Augé and Smith2013b; Weed et al. Reference Weed, Gassmann and Casagrande2011a, Reference Weed, Gassmann, Leroux and Casagrande2011b). Screening of C. asclepiadeus was halted for several years because larvae can develop on some non-target species, including North American Asclepias species (Bourchier et al. Reference Bourchier, Weed, Casagrande, Gassmann, Smith and Cappuccino2013). However, a recent study advocated reevaluating C. asclepiadeus as a potential agent (deJonge et al. Reference deJonge, Jones, Bourchier and Smith2020). European field tests have been underway since 2018 to better characterize whether it will attack non-target species under field conditions (LRM, personal observation).
The seed-feeding fly Euphranta connexa (Fabricius) (Diptera: Tephritidae), also from Europe, can successfully attack V. rossicum and V. nigrum in laboratory tests (Weed et al. Reference Weed, Gassmann, Leroux and Casagrande2011b) and should tolerate North American temperatures at most potential release sites (Leroux et al. Reference Leroux, Gassmann and Holliday2016). Larval feeding usually destroys all seed within a follicle and potentially all seed within patches of V. hirundinaria (Leimu and Lehtilä Reference Leimu and Lehtilä2006; Solbreck and Sillén-Tullberg Reference Solbreck and Sillén-Tullberg1986). It is projected to be an effective biological control agent if approved, potentially with greater impact than defoliating agents (Leimu and Lehtilä Reference Leimu and Lehtilä2006; Milbrath et al. Reference Milbrath, Davis and Biazzo2018). However, this species may occasionally oviposit on non-target species in laboratory tests (Bourchier et al. Reference Bourchier, Weed, Casagrande, Gassmann, Smith and Cappuccino2013). Additional screening has been halted in recent years in part due to logistical difficulties and to focus on C. asclepiadeus (LRM, personal observation).
Other insects from Europe and Asia that have been rejected as biological control agents due to a lack of host specificity or likely ineffectiveness include the leaf-feeding beetle Chrysolina aurichalcea asclepiadis (Villa) (Coleoptera: Chrysomelidae); the root-feeding beetles Chrysochus chinensis Baly, Chrysochus goniostoma Weise, and Chrysochus globicollis Lefèvre (Coleoptera: Chrysomelidae); and the gall-forming midges Contarinia asclepiadis (Giraud) and Contarinia vincetoxici Kieffer (Diptera: Cecidomyiidae) (Dolgovskaya et al. Reference Dolgovskaya, Volkovitsh, Reznik, Moseyko and Milbrath2016; Weed and Casagrande Reference Weed and Casagrande2011; Widenfalk et al. Reference Widenfalk, Gyllenstrand, Sylvén and Solbreck2002; Widenfalk and Solbreck Reference Widenfalk and Solbreck2005). Recent studies with native North American Chrysochus species have not shown significant utilization of V. rossicum (deJonge et al. Reference deJonge, Bourchier and Smith2017, Reference deJonge, Bourchier, Jones and Smith2019).
Plant pathogens were not noted on Vincetoxicum species in North America during early research on these invasive species (DiTommaso et al. Reference DiTommaso, Lawlor and Darbyshire2005b), although some pathogens have been reported from Europe (see “Importance”). More recently, a Cercospora leaf-spot fungus has been reported infecting V. nigrum in Michigan and Rhode Island, USA, resulting in significant defoliation, and a similar unidentified leaf-spot disease was reported on V. nigrum in eastern New York (Alred Reference Alred2021; Alred et al. Reference Alred, Hufbauer and Szűcs2022b; LRM, personal observation). Athelia rolfsii (Curzi) C.C. Tu & Kimbr. (Southern blight, syn.: Sclerotium rolfsii Sacc., Atheliaceae) was observed causing V. rossicum mortality in a New York meadow (Gibson et al. Reference Gibson, Castrillo, Giuliano Garisto Donzelli and Milbrath2012). In growth chamber experiments, A. rolfsii caused 60% to 100% mortality in 4- to 8-wk-old seedlings of both V. rossicum and V. nigrum; the pathogen was also virulent to adult plants of both species (Gibson et al. Reference Gibson, Castrillo, Giuliano Garisto Donzelli and Milbrath2012, Reference Gibson, Vaughan, Biazzo and Milbrath2014; Pethybridge et al. Reference Pethybridge, Murphy, Sharma, Biazzo and Milbrath2021). This fungus was not affected by (−)-antofine (Gibson et al. Reference Gibson, Vaughan, Biazzo and Milbrath2014). Although several species of broadleaf plants appear to be susceptible to this pathogen strain, it was suggested as a potential mycoherbicide for use in select habitats (Gibson et al. Reference Gibson, Vaughan, Biazzo and Milbrath2014). However, further development of A. rolfsii was abandoned, in part due to increased reports of its infection of New York table beet (Beta vulgaris L. subsp. vulgaris) fields (Pethybridge et al. Reference Pethybridge, Murphy, Sharma, Biazzo and Milbrath2021). The pathogen Colletotrichum lineola Corda (Glomerellaceae) was collected on the related species V. scandens in Russia (Berner et al. Reference Berner, Cavin, Mukhina and Kassanelly2011). Vincetoxicum rossicum and V. nigrum exhibited disease symptoms when inoculated with C. lineola, but host range tests were not conducted, as the impact of C. lineola on the plants would likely be minimal (D Berner, personal communication).
General Outlook
Vincetoxicum rossicum and V. nigrum have invaded some regions of North America, particularly the northeastern United States and southeastern Canada, and some locations in Europe outside their native distributions (e.g., Norway). Continued spread within North America is very likely. Spread within Europe may be somewhat constrained by biotic factors. In North America, V. rossicum and V. nigrum can establish near-monocultures and produce large quantities of wind-dispersed seeds. Because both species tolerate a wide range of environmental conditions with potentially high survival of all life stages, they colonize diverse habitats, including cities. Our CLIMEX models suggest that many uninvaded regions of the world may be climatologically suitable for Vincetoxicum species. However, long-distance movement of these species appears minimal, with the greatest threat coming from deliberate introductions of V. rossicum or V. nigrum into new areas for horticultural purposes, as happened in North America and Europe. Regulatory measures to prevent importation will be crucial. Cultural management of existing infestations of V. rossicum and V. nigrum is impeded by substantial belowground reserves. Management programs that include herbicides may be more effective, although repeated herbicide applications are typically necessary. Biological control may be a useful approach for these weeds of primarily natural areas. However, the biological control agent H. opulenta has only recently been released in Canada and the United States, and few additional agents may be available. Future research should focus on the improvement of management programs, the identification of biotic and abiotic factors constraining Vincetoxicum distributions, and the development of potential distribution models at a resolution useful to land managers.
Supplementary material
To view supplementary material for this article, please visit https://doi.org/10.1017/inp.2023.7
Acknowledgments
We thank Darren Kriticos (CSIRO) for his assistance with the CLIMEX model and DiTommaso Lab members Scott Morris and Ariadne Filothei Vazenios for their contributions to the text and figures. No conflicts of interest have been declared. Mention of trade names or commercial products in this article is solely for the purpose of providing specific information and does not imply recommendation or endorsement by the U.S. Department of Agriculture. USDA is an equal opportunity provider and employer.